- 1Department of Orthopaedics, LongHua Hospital, Shanghai University of Traditional Chinese Medicine, Shanghai, China
- 2Department of Orthopaedics, Spine Disease Institute, LongHua Hospital, Shanghai University of Traditional Chinese Medicine, Shanghai, China
Background: To critically evaluate the neurological recovery effects and antioxidant effects of erythropoietin (EPO) in rat models of spinal cord injury (SCI).
Methods: The PubMed, EMBASE, MEDLINE, ScienceDirect, and Web of Science were searched for animal experiments applying EPO to treat SCI to January 2022. We included studies which examined neurological function by the Basso, Beattie, and Bresnahan (BBB) scale, as well as cavity area and spared area, and determining the molecular-biological analysis of antioxidative effects by malondialdehyde (MDA) levels in spinal cord tissues. Meta-analysis were performed with Review Manager 5.4 software.
Results: A total of 33 studies were included in this review. The results of the meta-analysis showed that SCI rats receiving EPO therapy showed a significant locomotor function recovery after 14 days compared with control, then the superiority of EPO therapy maintained to 28 days from BBB scale. Compared with the control group, the cavity area was reduced [4 studies, weighted mean difference (WMD) = −16.65, 95% CI (−30.74 to −2.55), P = 0.02] and spared area was increased [3 studies, WMD =11.53, 95% CI (1.34 to 21.72), P = 0.03] by EPO. Meanwhile, MDA levels [2 studies, WMD = −0.63 (−1.09 to −0.18), P = 0.007] were improved in the EPO treatment group compared with control, which indicated its antioxidant effect. The subgroup analysis recommended 5,000 UI/kg is the most effective dose [WMD = 4.05 (2.23, 5.88), P < 0.0001], although its effect was not statistically different from that of 1,000 UI/kg. Meanwhile, the different rat strains (Sprague-Dawley vs. Wistar), and models of animals, as well as administration method (single or multiple administration) of EPO did not affect the neuroprotective effect of EPO for SCI.
Conclusions: This systematic review indicated that EPO can promote the recovery of the locomotor function of SCI rats. The mechanism exploration of EPO needs to be verified by experiments, and then carefully designed randomized controlled trials are needed to explore its neural recovery effects.
Introduction
Spinal cord injury (SCI) is an injury to the spinal cord caused by trauma or disease, which may lead to alterations to the normal motor, sensory or autonomic function of the spinal cord (1, 2). Traumatic SCI is a disastrous event associated with high morbidity and mortality (3). With the development of the modern transportation and construction industries, incidence of SCI has sharply risen up (4, 5). The incidence of SCI is ~180,000 cases per year worldwide (6). Half of the people with SCI have a huge impact on their daily lives, such as limb paralysis and urine obstacles (7). In the last 20 years, drug therapy, Chinese herbal medicine, and stem cell transplantation have been studied widely across the world and have been the focus of a significant effort.
At present, the main methods for the therapy of SCI include surgical treatment, drug treatment, hyperbaric oxygen therapy, and physical therapy. During the SCI window, the main strategies used to restrict secondary damage are surgical decompression, therapeutic hypothermia, and the administration of high doses of glucocorticoids (8, 9). However, there may be an increased risk of gastrointestinal hemorrhage and respiratory tract infection after high-dose methylprednisolone treatment early after SCI (10). Surgical treatment of patients with spinal cord injury is not satisfactory, and it is usually related to poor prognosis (11). At present, the drugs studied include resveratrol, Gangliosides, quercetin, and mesenchymal stem cells, which have certain effects on the treatment of SCI, but there are still limitations (12–15).
Erythropoietin (EPO), an evolutionarily conserved hormone specially produced within the kidney, has been properly documented for its quintessential role in erythropoiesis. EPO belongs to the type 1 cytokine superfamily and has 165 amino acids forming four α helices 1 (16). EPO binds to a homodimeric EPO receptor, which induces phosphorylation of numerous tyrosine residues within the intracellular domain of the receptor, which ultimately ends in the activation of prosurvival, proliferation, and prodifferentiation genes in the progenitor cells (17). In recent years, numerous studies have shown that EPO acts far beyond erythropoiesis. In hypoxia, trauma, or inflammation, many tissues produce EPO at the borders surrounding injury sites; EPO plays a central role in tissue protection and restoration. This may include the mechanisms of producing anti-apoptotic factors, limiting oxidative stress, inhibiting the production of nitric oxide, stimulating angiogenesis, reducing glutamate toxicity, and relieving inflammation by activating antioxidant enzymes and inhibiting lipid peroxidation (18–23). However, a few studies have shown that EPO has no positive effect on the recovery of motor function, the reduction of lesion volume, and the increase in the number of axons (24, 25). Moreover, the demonstration of EPO and its analogs' wide neuroprotective results in animal models of cord lesion and traumatic brain injury, and human trials like stroke, ought to inspire scientists and clinicians to design clinical trials assessing the efficacy of these pharmacological compounds on SCI (26–28). Therefore, the neurological recovery and antioxidant effects of EPO for SCI should be evaluated.
Therefore, our aim was to verify the hypothesis that EPO was more effective than placebo on locomotor function recovery in the rat SCI model. A systematic review and analysis was performed to assess the neurological recovery and anti-oxidative results of EPO in SCI rats.
Data and methods
Search strategy
Literature retrieval was conducted from English databases, including PubMed, EMBASE, MEDLINE, ScienceDirect, and Web of Science. Relevant studies were found using the following search terms “EPO,” “epoetin,” “erythropoietin,” “Procrit,” “epogen,” “erupt,” “Biopoin,” “Eporatio,” “darbepoetin,” “DARB,” “ARANESP,” “NESP,” “Neorecormon,” “erythropoietin stimulating proteins,” “erythropoietin stimulating agents,” “ESA,” “epoetin alfa,” “darbepoetin alfa,” “spinal cord injury,” “spinal cord injuries,” “traumatic spinal cord injury,” and “spinal cord repair.” In addition, the bibliographies of all included articles and important conference papers were searched for additional relevant studies. Retrieval of literature was carried out from the inception dates of the databases to January 2022. No language restriction was used in the literature search, and the search was limited to studies in rats.
Study selection
Two reviewers (YYZ and MY) evaluated each article separately for preliminary screening, according to the title and abstract, then read through the full text for secondary screening. Disagreements were resolved by agreement and discussion with a third party (JHX).
Inclusion and exclusion criteria
Trials were included if they met the following criteria for participants, interventions, comparisons, outcomes, and study design (PICOS) criteria: (1) Participants: Experimental rat studies that involved at any age or gender with traumatic SCI, including contusion, impactor damage, crush and compression injury; (2) Intervention: The dose and the administration method of EPO, duration of treatment, and follow-up time were unrestricted; (3) Comparison: Physiological saline, vehicle, or no treatment were included in the control groups; (4) Outcomes: The Basso, Beattie, and Bresnahan (BBB) score was prioritized for collection as the primary outcome (29). Cavity area and spared area was also used to evaluate the neurological recovery of EPO, as secondary outcomes, as well as malondialdehyde (MDA) to antioxidant effect. Articles containing other outcome measures were also included for the final mechanism summary. (5) Study design: Comparision of EPO with control in SCI rats.
Studies with one or more of the following conditions were excluded: (1) Nontraumatic injury, penetrating injury, and complete transverse spinal cord injury of SCI rat models were excluded. (2) The study of clinical case reports, genetically modified rats, and EPO combined with other intervention treatments was excluded. (3) Review, duplicated, and not related articles were excluded.
Data extraction
The information was independently extracted from papers by two authors. The following data were extracted: first author, publication year, animal gender, days of rats, weight of rats, number of rats per group, model of SCI, SCI level, type of intervention, timing of intervention, duration of intervention, the daily dose of EPO and outcomes. The mean ± standard deviation (SD) of each outcome was also extracted for pooled analysis. Disagreements were resolved by discussion, and a third reviewer's opinion was asked for when necessary. If the data were missing or incomplete, numerical values were requested from the authors via email, or GetData Graph Digitizer 2.24 was used to estimate numerical values from the graphs (http://getdata-graph-digitizer.com/download.php).
Assessment of risk of bias in included studies
The Collaborative Approach to Meta Analysis and Review of Animal Data From Experimental Stroke (CAMARADES) 10-item checklist were used to assess the quality and design of the studies by two independent investigators (30). The CAMARADES list includes the following: (1) peer-reviewed journal; (2) temperature control; (3) animals were randomly allocated; (4) blind established model; (5) blinded outcome assessment; (6) anesthetics used without marked intrinsic neuroprotective properties; (7) animal model (diabetic, advanced age or hypertensive); (8) calculation of sample size; (9) statement of compliance with animal welfare regulations; (10) possible conflicts of interest.
Two reviewers assessed the risk of bias. Bias was marked as high or low risk, as well as “unclear” indicated that the risk of bias was unclear. The symbol “+” was used to marked low risk, and it was also recorded as the point of quality score. Disagreements were resolved by discussion, and a third reviewer's opinion was asked for when necessary.
Statistical analysis
Statistical analyses were performed with version 5.4 of the Cochrane Collaboration Review Manager (RevMan). Data from all EPO groups were pooled to compare with the SCI groups; data were pooled if outcomes were reported by at least two studies and continuous variables were expressed as weighted mean difference (WMD) or standardized mean difference (SMD), both with 95% confidence interval (CI). A chi-square-based Q test was used to measure between-study heterogeneity. Statistical significance was defined at P < 0.05. I2 statistic was calculated to quantify the proportion of the total variation across studies due to heterogeneity (31). The fixed effect models were adopted, if the heterogeneity was not obvious (P > 0.1; I2 ≤ 50%); when P ≤ 0.1; I2 > 50%, random effect models were used (32, 33). Subgroup analysis was performed according to different conditions, such as rat strain, modeling method, dosage and time of administration. If we included at least 10 studies in a meta-analysis related to primary outcomes, funnel plots were used to test the potential risk of publication bias (34).
Results
Search results
Among the 746 articles found in the initial search strategy, 649 similar and duplicated studies were removed. Fifty-four articles were ruled out by the titles and abstracts; 43 studies were retained. After evaluating the full texts, 10 articles were excluded, 33 independent studies were chosen for the final meta-analysis after evaluation. The flow chart of the study selection is summarized in Figure 1.
Characteristics of included studies
Characteristics of the studies included in this systematic review are shown in Table 1. Of the 33 articles that met the inclusion criteria, all were published in English. Sprague-Dawley rats were used in 25 studies and Wistar rats were used in eight studies. Nineteen studies used male rats, nine studies were conducted using female rats and the other studies had no gender information. Only two studies had age information (57, 59). Studies used aneursym clip, impactor, aortic occlusion, deep hypothermic circulatory arrest or bulldog clamp to induce SCI in rats.
In most studies EPO was administered to SCI rats immediately afterwards, either intraperitoneally or intravenously, at doses ranging from 100 to 5,000 UI/kg. The negative control was saline in most cases. All studies reported results by functional assessment or biochemical analysis.
Bias analysis of included studies
The risks of bias for all 33 independent studies are shown in Table 2. The overall methodological quality of studies methodology is good. Thirty-three studies contained a statement of the peer-reviewed journal, blind established model, and anesthetics used without marked intrinsic neuroprotective properties. None of the studies have described whether animal models are affected by diabetes, advanced age, or high blood pressure. Ninteen studies described the allocation concealment and 17 studies described temperature control. Blinded assessment of the outcome was described in 15 studies. Thirty-two studies described the statement of compliance with animal welfare regulations and only two studies described calculation of sample size. Twenty-three studies contained a statement of potential conflicts of interest.
The effects of EPO intervention on BBB score in rats with SCI
The meta-analysis indicated that SCI rats receiving EPO therapy showed a significant locomotor function recovery in all studies (1 d to 28 d, P < 0.01; Figure 2). After the intervention of EPO, the BBBs score increased [1 d, 21 studies (18, 25, 35–37, 41, 43–46, 48, 49, 51, 54, 59, 61, 63–65), WMD = 0.69, 95% CI [0.22, 1.15], P = 0.004; 3 d, 17 studies (18, 25, 35, 42, 43, 46, 48, 49, 58, 63, 66–70), WMD = 2.50, 95% CI [1.69, 3.31], P < 0.00001; 7 d, 24 studies (18, 25, 35, 36, 42, 43, 45, 46, 48, 49, 54, 58, 59, 63, 65, 67–69, 71–74), WMD = 3.31, 95% CI [2.21, 4.41], P < 0.00001; 14 d, 20 studies (18, 25, 35, 42, 43, 45, 46, 48, 49, 58, 59, 63, 65, 67–69, 73, 74), WMD = 3.80, 95% CI [2.50, 5.09], P < 0.00001; 21 d, 18 studies (18, 25, 35, 42, 43, 45, 46, 48, 49, 58, 59, 63, 65, 67, 68, 73), WMD = 4.06, 95% CI [2.89, 5.88], P < 0.00001; 28 d, 19 studies (18, 25, 35, 37, 42, 43, 45, 46, 49, 58, 59, 63, 65, 67, 68, 73, 75), WMD = 4.88, 95% CI [3.57, 6.18], P < 0.00001;] in a random-effects model. The meta-analysis indicated that SCI rats receiving EPO therapy showed a significant locomotor function recovery after 14 days compared with control, then the superiority of EPO therapy maintained to 28 days (Figure 2).
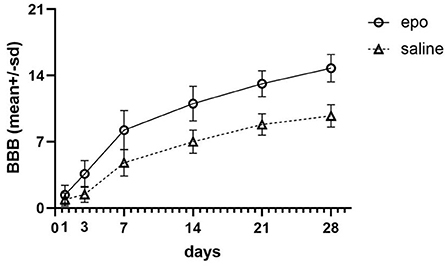
Figure 2. Evolution of the scores on the BBB of the two groups including the six (1, 3, 7, 14, 21, and 28 days) evolution periods. BBB, Basso-Beattie-Bresnahan locomotor rating.
Subgroup analysis was carried out according to the different animal models and species, as well as the different EPO administration. While, there was no difference between different animal models and species, then the subgroup analysis recommended 5,000 UI/kg is the more effective dose [7 d, 11 studies (18, 35, 36, 41, 44, 45, 54, 59, 61, 64, 65), WMD = 4.05, 95% CI (2.23, 5.88), P < 0.0001; Table 3], although its effect was not statistically different from that of 1,000 UI/kg [7 d, nine studies (18, 25, 43, 45, 46, 48, 49, 51, 63), WMD = 3.13, 95% CI (1.05, 5.21), P = 0.003; Table 3]. Then, the different administration methods of animals, single or multiple administration after SCI dis not affect the neuroprotective effect of EPO for SCI (Table 3).
The effects of EPO intervention on cavity area and spared area in rats with SCI
Four studies reported cavity area and three studies reported spared area as an outcome. Compared with the control group, the cavity area was reduced [four studies (37, 45, 54, 65), WMD = −16.65, 95% CI (−30.74 to −2.55), P = 0.02; Figure 3] and spared area was increased by EPO [three studies (36, 37, 45), WMD = 11.53, 95% CI (1.34 to 21.72), P = 0.03; Figure 4].
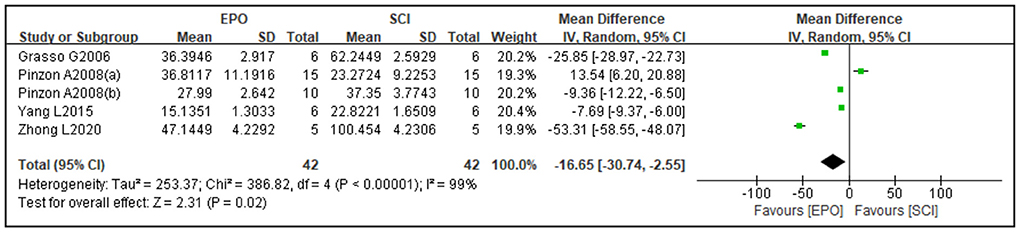
Figure 3. Forest plot for the effects of EPO intervention on cavity area in rats with SCI. EPO, erythropoietin; SCI, spinal cord injury.
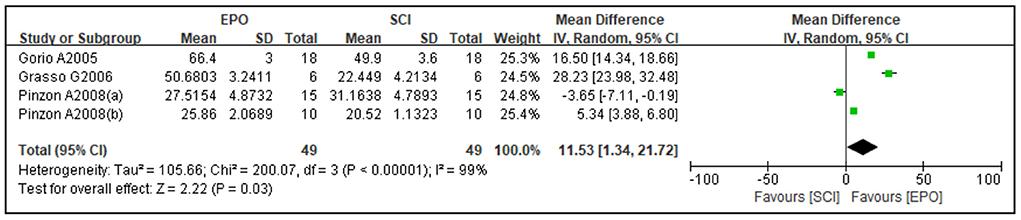
Figure 4. Forest plot for the effects of EPO intervention on spared area in rats with SCI. EPO, erythropoietin; SCI, spinal cord injury.
The effects of EPO intervention on MDA in rats with SCI
Antioxidative effects were measured in three studies. MDA has been widely used as a convenient biomarker for lipid peroxidation of omega-3 and omega-6 fatty acids due to its easy reaction with thiobarbituric acid (66, 67). Three studies measured MDA levels after SCI and found that MDA levels were significantly lower in the EPO group than the matched group of controls [2 studies (42, 58), WMD = −0.63, 95% CI (−1.09 to −0.18), P = 0.007; Figure 5] to detect its antioxidative effect.

Figure 5. Forest plot for the effects of EPO intervention on MDA in rats with SCI. EPO, erythropoietin; MDA, malondialdehyde.
Publication bias
The funnel plot of the BBB score was essentially symmetrical, suggesting a low risk of publication bias (Figure 6).
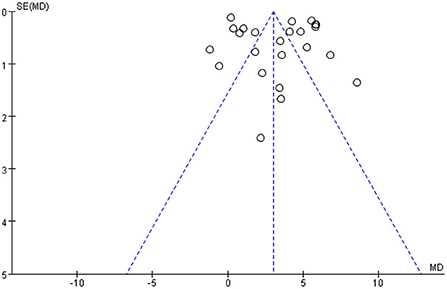
Figure 6. Funnel plots of publication bias for BBB score. BBB, Basso-Beattie-Bresnahan locomotor rating.
Discussion
Summary of main results
Currently, this is the first meta-analysis of animal experiments where EPO improves neurological outcomes after SCI. A total of 33 studies were included. The overall methodological quality of the studies were rated as “good.” Twenty-five of 33 studies scored over six after quality assessment, which meant over 75% researches were advanced in methodology. Results showed that EPO had been closely associated with improving BBB score and spared area, as well as decreasing cavity area and MDA after injury in vivo animal studies, which indicated its inducing neurological recovery and antioxidant effects. Meanwhile, subgroup analysis showed that a single injection of 5,000 UI/kg after injury promoted the recovery of spinal cord function. Meanwhile, the differences of species, animal models and administration of EPO were insignificant on the neuroprotective effect of EPO against SCI. Moreover, the funnel plot is essentially symmetrical while the accuracy of the measurement results is robust.
The possible mechanism for the effect of EPO in SCI
According to the pathology of SCI, it can be categorized into primary and secondary SCI. Primary injury is caused mainly by mechanical damage resulting in the destruction of the local neural tissue. Secondary injury develops gradually on the basis of the primary injury and includes oxidative stress, apoptosis, and autophagy (68–73). The potential mechanism for the effect of EPO in SCI is summarized in Figure 7 and Table 4.
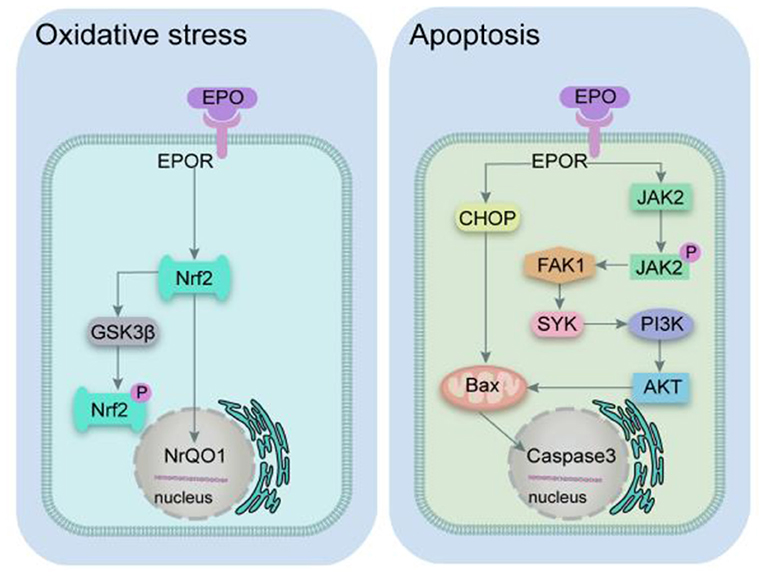
Figure 7. Mechanism diagram of EPO pathway. EPO, erythropoietin; EPOR, erythropoietin receptor; Nrf2, nuclear factor 2; GSK3β, glycogen synthase kinase 3 beta; NQO1, (NAD(P)H quinone dehydrogenase 1; CHOP, C/Ebp-homologous protein; BAX, Bcl2-associated x protein; JAK2, janus activated kinase 2; FAK1, focal adhesion kinase 1; SYK, spleen tyrosine kinase; PI3K, phosphatidylinositol 3-kinase; AKT, AKT serine/threonine kinase.
Protective effect of EPO related to oxidative stress
SCI is mainly a physical injury, which releases a lot of reactive oxygen species (ROS) and causes oxidative stress reactions in cells. It is widely believed that oxidative stress injury causes cell and tissue damage after SCI. One of the mechanisms of EPO treating SCI is inhibiting oxidative toxicity of cells (74, 75). This study showed that EPO against SCI mainly attributed to reducing MDA levels which was an important indicator of oxidative stress. Recent studies have demonstrated that nuclear factor 2 (Nrf2) is the core transcription factor of antioxidant response of exogenous stimuli. Under the conditions of oxidative stress, Nrf2 goes through the cytoplasm and enters the nucleus, which regulates the expression of cytoprotective enzymes such as nicotinamide adenine dinucleotide phosphate quinone oxidoreductase 1 (NrQO1) and glutathione s-transferase (GST), thus reducing cell oxidation and inflammation (77). Oxidative stress causes endoplasmic reticulum (ER) stress which disrupts protective signals thus leading to glycogen synthase kinase 3 beta (GSK-3β) phosphorylation and increasing in mitochondrial GSK-3β. This dual mechanism aggravates ER stress inhibiting EPO-induced suppression (78). The exogenous administration of recombinant EPO can activate Nrf2 signal pathway. Therefore, it was suspected that EPO inhibits oxidative stress through the classical Nrf2 signaling pathway in the SCI model.
Protective effect of EPO related to apoptosis
The C/Ebp-homologous Protein (CHOP) is a symbolic gene of endoplasmic reticulum stress-induced apoptosis, and cells involved in endoplasmic reticulum stress-regulated apoptosis and deficient expression of CHOP gene significantly reduce cell death induced by endoplasmic reticulum stress. Continuous activation of CHOP can down-regulate the anti-apoptotic protein b cell lymphoma 2 (Bcl-2), and then activate the mitochondrial apoptosis pathway regulated by Bcl2-associated x protein (Bax) (79). The anti-apoptotic mechanisms of phosphatidylinositol 3-kinase/serine/threonine kinase (PI3K/Akt) signaling pathway are mainly due to the direct regulation of Bad kinase, the transcription factor Forkhead, and the inhibition of mitochondrial apoptosis factor release (80–83). SCI leads to local inflammatory reaction and releases a variety of cytokines, which can regulate cell apoptosis by activating janus activated kinase 2/signal transducer and activator of transcription (JAK-STAT) signaling pathway (84). STAT3 inhibits neuronal apoptosis by inducing the expression of genes related to cell growth and repair, such as Bcl-2 and Bcl-xl (85). However, STAT1 played a role in vinegar cell apoptosis by inhibiting the expressions of Bcl-2 and Bcl-xl and up-regulating the expressions of caspase-1, caspase-2 and caspase-3 (86–88). Exogenous administration of EPO can reduce tissue damage and apoptosis by improving the expression of anti-apoptotic genes and telomerase activity (89). EPO can activate PI3K/Akt, JAK/STAT signaling pathways and improve ERS, thus enhancing cell repair capacity and resisting apoptosis (90–92).
Strengths and limitation of evidence
In this study, the advantages of systematic and experimental evaluation were combined, and the summarized data indicated that EPO was a promising drug for the recovery of neurological function. Meanwhile the action mechanism of EPO was summarized. An in-depth understanding of the mechanism of EPO treatment for SCI will not only contribute to the exploration of the pathological mechanism of SCI, but also was conductive to exploring new and effective drugs for further development and related transformation research. Among the limitations of the evidence, MDA and spared area only have two to four studies, with relatively low evidence strength. Rats of other ages or other kinds of experimental animals lack a certain quantity and quality of research, so comprehensive evidence cannot be provided. Inevitably, this systematic review has some limitations. First, since we were unable to obtain data on individual animals, we could only conduct a meta-analysis of the overall level of each study. Although we used the standardized mean difference to reduce the length of the statistical effect, we did not completely eliminate the bias, which is also an important factor affecting the quality of the study. Different SCI models were used in each study, for example, administration method (single or multiple administration). A simple hybrid approach is not the most appropriate approach, but we cannot layer-analyze all inconsistencies.
Conclusions
Based on the results of this meta-analysis, we demonstrated that EPO intervention could improve neurological recovery and antioxidative effects in rat models of SCI. Our results show that the mechanism of EPO preventing neuronal apoptosis is mainly through the alleviation of oxidative stress. The results of this meta-analysis must be interpreted and applied with an appropriate degree of caution because some factors such as the failure to use more novel research methods may overestimate the efficacy of treatment. Even so, EPO could be a promising drug to treat SCI. In the future, it deserves a larger study that takes into account the design features recommended in the discussion of this meta-analysis.
Data availability statement
The original contributions presented in the study are included in the article/supplementary material, further inquiries can be directed to the corresponding authors.
Author contributions
Y-yZ and MY designed the study and did the data analysis. KZ and R-rX collected the data. Y-yZ, MY, and J-hX wrote the manuscript. X-jC and WM revised the manuscript and decided to submit the manuscript for publication. All authors contributed to the article and approved the submitted version.
Funding
The financial support for this work are provided by the Research Project of Shanghai Science and Technology Commission (19401901100 and 21S21900500), Shanghai Municipal Health Commission (No. 2021LPTD-008), The Fifth Batch of Scholars of Longhua Hospital Affiliated to Shanghai University of TCM (KC2022006), The program for Longhua Medical Scholar (No. YM2021007), National Natural Science Foundation of China (No. 82174409 for MY and No. 82074454 for X-jC), China Association of Traditional Chinese Medicine Youth Talent Promotion Project (No. CACM-2021-QNRC2-B23 for MY), and Shanghai Natural Science Foundation (No. 20ZR1459000 for MY and 22ZR1461700 for X-jC).
Conflict of interest
The authors declare that the research was conducted in the absence of any commercial or financial relationships that could be construed as a potential conflict of interest.
Publisher's note
All claims expressed in this article are solely those of the authors and do not necessarily represent those of their affiliated organizations, or those of the publisher, the editors and the reviewers. Any product that may be evaluated in this article, or claim that may be made by its manufacturer, is not guaranteed or endorsed by the publisher.
References
1. Hendrix S, Sanchez S, Ventriglia E, Lemmens S. HDAC8 inhibition reduces lesional Iba-1+ cell infiltration after spinal cord injury without effects on functional recovery. Int J Mol Sci. (2020) 21. doi: 10.3390/ijms21124539
3. Liu J, Yi L, Xiang Z, Zhong J, Zhang H, Sun T. Resveratrol attenuates spinal cord injury-induced inflammatory damage in rat lungs. Int J Clin Exp Pathol. (2015) 8:1237–46.
4. Shrestha RP, Qiao JM, Shen FG, Bista KB, Zhao ZN, Yang J. Intra-spinal bone marrow mononuclear cells transplantation inhibits the expression of nuclear factor-kappaB in acute transection spinal cord injury in rats. J Korean Neurosurg Soc. (2014) 56:375–82. doi: 10.3340/jkns.2014.56.5.375
5. Selvarajah S, Hammond ER, Schneider EB. Trends in traumatic spinal cord injury. JAMA. (2015) 314:1643. doi: 10.1001/jama.2015.11194
6. Lee BB, Cripps RA, Fitzharris M, Wing PC. The global map for traumatic spinal cord injury epidemiology: update 2011, global incidence rate. Spinal Cord. (2014) 52:110–6. doi: 10.1038/sc.2012.158
7. Goulet J, Richard-Denis A, Thompson C, Mac-Thiong JM. Relationships between specific functional abilities and health-related quality of life in chronic traumatic spinal cord injury. Am J Phys Med Rehabil. (2019) 98:14–9. doi: 10.1097/PHM.0000000000001006
8. Chen S, Levi AD. Restorative treatments for spinal cord injury. Neurosurg Clin N Am. (2017) 28:63–71. doi: 10.1016/j.nec.2016.08.004
9. Zhang Q, Huang C, Tang T, Shi Q, Yang H. Comparative neuroprotective effects of methylprednisolone and rosiglitazone, a peroxisome proliferator-activated receptor-gamma following spinal cord injury. Neurosciences. (2011) 16:46–52.
10. Liu Z, Yang Y, He L, Pang M, Luo C, Liu B, et al. High-dose methylprednisolone for acute traumatic spinal cord injury: a meta-analysis. Neurology. (2019) 93:e841–50. doi: 10.1212/WNL.0000000000007998
11. Fehlings MG, Tetreault LA, Wilson JR, Kwon BK, Burns AS, Martin AR, et al. A clinical practice guideline for the management of acute spinal cord injury: introduction, rationale, and scope. Global Spine J. (2017) 7:84S−94S. doi: 10.1177/2192568217703387
12. Geisler FH, Coleman WP, Grieco G, Poonian D. The Sygen multicenter acute spinal cord injury study. Spine. (2001) 26:S87–98. doi: 10.1097/00007632-200112151-00015
13. Xu BP, Yao M, Li ZJ, Tian ZR, Ye J, Wang YJ, et al. Neurological recovery and antioxidant effects of resveratrol in rats with spinal cord injury: a meta-analysis. Neural Regen Res. (2020) 15:482–90. doi: 10.4103/1673-5374.266064
14. Wang Y, Xiong M, Wang M, Chen H, Li W, Zhou X. Quercetin promotes locomotor function recovery and axonal regeneration through induction of autophagy after spinal cord injury. Clin Exp Pharmacol Physiol. (2021) 48:1642–52. doi: 10.1111/1440-1681.13573
15. Cofano F, Boido M, Monticelli M, Zenga F, Ducati A, Vercelli A, et al. Mesenchymal stem cells for spinal cord injury: current options, limitations, and future of cell therapy. Int J Mol Sci. (2019) 20:2698. doi: 10.3390/ijms20112698
16. Brines M, Cerami A. The receptor that tames the innate immune response. Mol Med. (2012) 18:486–96. doi: 10.2119/molmed.2011.00414
17. McGraw K, List A. Erythropoietin receptor signaling and lipid rafts. Vitam Horm. (2017) 105:79–100. doi: 10.1016/bs.vh.2017.02.002
18. Gorio A, Gokmen N, Erbayraktar S, Yilmaz O, Madaschi L, Cichetti C, et al. Recombinant human erythropoietin counteracts secondary injury and markedly enhances neurological recovery from experimental spinal cord trauma. Proc Natl Acad Sci USA. (2002) 99:9450–5. doi: 10.1073/pnas.142287899
19. Solaroglu I, Solaroglu A, Kaptanoglu E, Dede S, Haberal A, Beskonakli E, et al. Erythropoietin prevents ischemia-reperfusion from inducing oxidative damage in fetal rat brain. Childs Nerv Syst. (2003) 19:19–22. doi: 10.1007/s00381-002-0680-2
20. Shingo T, Sorokan ST, Shimazaki T, Weiss S. Erythropoietin regulates the in vitro and in vivo production of neuronal progenitors by mammalian forebrain neural stem cells. J Neurosci. (2001) 21:9733–43. doi: 10.1523/JNEUROSCI.21-24-09733.2001
21. Masuda S, Nagao M, Takahata K, Konishi Y, Gallyas FJ, Tabira T, et al. Functional erythropoietin receptor of the cells with neural characteristics. Comparison with receptor properties of erythroid cells. J Biol Chem. (1993) 268:11208–16. doi: 10.1016/S0021-9258(18)82112-3
22. Digicaylioglu M, Lipton SA. Erythropoietin-mediated neuroprotection involves cross-talk between Jak2 and NF-kappaB signalling cascades. Nature. (2001) 412:641–7. doi: 10.1038/35088074
23. Ribatti D, Presta M, Vacca A, Ria R, Giuliani R, Dell'Era P, et al. Human erythropoietin induces a pro-angiogenic phenotype in cultured endothelial cells and stimulates neovascularization in vivo. Blood. (1999) 93:2627–36. doi: 10.1182/blood.V93.8.2627
24. Rink S, Manthou ME, Arnold J, Grigo M, Dicken P, Abdulla D, et al. Motor, sensitive, and vegetative recovery in rats with compressive spinal-cord injury after combined treatment with erythropoietin and whole-body vibration. Restor Neurol Neurosci. (2021) 39:85–100. doi: 10.3233/RNN-201120
25. de Mesquita CP, Cristante AF, de Barros FT, Ferreira R, Dos SG. Effects of tacrolimus and erythropoietin in experimental spinal cord lesion in rats: functional and histological evaluation. Spinal Cord. (2016) 54:439–44. doi: 10.1038/sc.2015.172
26. Carelli S, Marfia G, Di Giulio AM, Ghilardi G, Gorio A. Erythropoietin: recent developments in the treatment of spinal cord injury. Neurol Res Int. (2011) 2011:1–8. doi: 10.1155/2011/453179
27. Gatto R, Chauhan M, Chauhan N. Anti-edema effects of rhEpo in experimental traumatic brain injury. Restor Neurol Neurosci. (2015) 33:927–41. doi: 10.3233/RNN-150577
28. Chauhan NB, Gatto R. Synergistic benefits of erythropoietin and simvastatin after traumatic brain injury. Brain Res. (2010) 1360:177–92. doi: 10.1016/j.brainres.2010.09.010
29. Basso DM, Beattie MS, Bresnahan JC. A sensitive and reliable locomotor rating scale for open field testing in rats. J Neurotrauma. (1995) 12:1–21. doi: 10.1089/neu.1995.12.1
30. Macleod MR, O'Collins T, Howells DW, Donnan GA. Pooling of animal experimental data reveals influence of study design and publication bias. Stroke. (2004) 35:1203–8. doi: 10.1161/01.STR.0000125719.25853.20
31. Higgins JP, Thompson SG, Deeks JJ, Altman DG. Measuring inconsistency in meta-analyses. BMJ. (2003) 327:557–60. doi: 10.1136/bmj.327.7414.557
32. Mantel N, Haenszel W. Statistical aspects of the analysis of data from retrospective studies of disease. J Natl Cancer Inst. (1959) 22:719–48.
33. DerSimonian R, Laird N. Meta-analysis in clinical trials. Control Clin Trials. (1986) 7:177–88. doi: 10.1016/0197-2456(86)90046-2
34. Higgins JP. Cochrane handbook for systematic reviews of interventions. Version 5.1. 0. The Cochrane Collaboration (2011). Available online at: www.cochrane-handbook.org
35. Leist M, Ghezzi P, Grasso G, Bianchi R, Villa P, Fratelli M, et al. Derivatives of erythropoietin that are tissue protective but not erythropoietic. Science. (2004) 305:239–42. doi: 10.1126/science.1098313
36. Gorio A, Madaschi L, Di Stefano B, Carelli S, Di Giulio AM, De Biasi S, et al. Methylprednisolone neutralizes the beneficial effects of erythropoietin in experimental spinal cord injury. Proc Natl Acad Sci U S A. (2005) 102:16379–84. doi: 10.1073/pnas.0508479102
37. Grasso G, Sfacteria A, Erbayraktar S, Passalacqua M, Meli F, Gokmen N, et al. Amelioration of spinal cord compressive injury by pharmacological preconditioning with erythropoietin and a nonerythropoietic erythropoietin derivative. J Neurosurg Spine. (2006) 4:310–8. doi: 10.3171/spi.2006.4.4.310
38. Choi UH, Ha Y, Huang X, Park SR, Chung J, Hyun DK, et al. Hypoxia-inducible expression of vascular endothelial growth factor for the treatment of spinal cord injury in a rat model. J Neurosurg Spine. (2007) 7:54–60. doi: 10.3171/SPI-07/07/054
39. Okutan O, Solaroglu I, Beskonakli E, Taskin Y. Recombinant human erythropoietin decreases myeloperoxidase and caspase-3 activity and improves early functional results after spinal cord injury in rats. J Clin Neurosci. (2007) 14:364–8. doi: 10.1016/j.jocn.2006.01.022
40. Vitellaro-Zuccarello L, Mazzetti S, Madaschi L, Bosisio P, Gorio A, De Biasi S. Erythropoietin-mediated preservation of the white matter in rat spinal cord injury. Neuroscience. (2007) 144:865–77. doi: 10.1016/j.neuroscience.2006.10.023
41. Vitellaro-Zuccarello L, Mazzetti S, Madaschi L, Bosisio P, Fontana E, Gorio A, et al. Chronic erythropoietin-mediated effects on the expression of astrocyte markers in a rat model of contusive spinal cord injury. Neuroscience. (2008) 151:452–66. doi: 10.1016/j.neuroscience.2007.11.004
42. Yazihan N, Uzuner K, Salman B, Vural M, Koken T, Arslantas A. Erythropoietin improves oxidative stress following spinal cord trauma in rats. Injury. (2008) 39:1408–13. doi: 10.1016/j.injury.2008.03.010
43. Fumagalli F, Madaschi L, Brenna P, Caffino L, Marfia G, Di Giulio AM, et al. Single exposure to erythropoietin modulates nerve growth factor expression in the spinal cord following traumatic injury: comparison with methylprednisolone. Eur J Pharmacol. (2008) 578:19–27. doi: 10.1016/j.ejphar.2007.09.021
44. Mann C, Lee JH, Liu J, Stammers AM, Sohn HM, Tetzlaff W, et al. Delayed treatment of spinal cord injury with erythropoietin or darbepoetin–a lack of neuroprotective efficacy in a contusion model of cord injury. Exp Neurol. (2008) 211:34–40. doi: 10.1016/j.expneurol.2007.12.013
45. Pinzon A, Marcillo A, Pabon D, Bramlett HM, Bunge MB, Dietrich WD, et al. A re-assessment of erythropoietin as a neuroprotective agent following rat spinal cord compression or contusion injury. Exp Neurol. (2008) 213:129–36. doi: 10.1016/j.expneurol.2008.05.018
46. Fang XQ, Fang M, Fan SW, Gu CL. Protection of erythropoietin on experimental spinal cord injury by reducing the expression of thrombospondin-1 and transforming growth factor-beta. Chin Med J. (2009) 122:1631–5.
47. Kontogeorgakos VA, Voulgaris S, Korompilias AV, Vekris M, Polyzoidis KS, Bourantas K, et al. The efficacy of erythropoietin on acute spinal cord injury. An experimental study on a rat model. Arch Orthop Trauma Surg. (2009) 129:189–94. doi: 10.1007/s00402-008-0594-x
48. Huang H, Fan S, Ji X, Zhang Y, Bao F, Zhang G. Recombinant human erythropoietin protects against experimental spinal cord trauma injury by regulating expression of the proteins MKP-1 and p-ERK. J Int Med Res. (2009) 37:511–9. doi: 10.1177/147323000903700227
49. Hong Z, Hong H, Chen H, Wang Z, Hong D. Investigation of the protective effect of erythropoietin on spinal cord injury in rats. Exp Ther Med. (2011) 2:837–41. doi: 10.3892/etm.2011.285
50. Hwang J, Huh J, Kim J, Jeon Y, Cho S, Han S. Pretreatment with erythropoietin attenuates the neurological injury after spinal cord ischemia. Spinal Cord. (2012) 50:208–12. doi: 10.1038/sc.2011.136
51. Hong Z, Hong H, Chen H, Wang Z, Hong D. Protective effects of erythropoietin in experimental spinal cord injury by reducing the C/EBP-homologous protein expression. Neurol Res. (2012) 34:85–90. doi: 10.1179/1743132811Y.0000000026
52. Jin W, Ming X, Hou X, Zhu T, Yuan B, Wang J, et al. Protective effects of erythropoietin in traumatic spinal cord injury by inducing the Nrf2 signaling pathway activation. J Trauma Acute Care Surg. (2014) 76:1228–34. doi: 10.1097/TA.0000000000000211
53. Freitag MT, Márton G, Pajer K, Hartmann J, Walder N, Rossmann M, et al. Monitoring of short-term erythropoietin therapy in rats with acute spinal cord injury using manganese-enhanced magnetic resonance imaging. J Neuroimaging. (2015) 25:582–9. doi: 10.1111/jon.12202
54. Yang L, Yan X, Xu Z, Tan W, Chen Z, Wu B. Delayed administration of recombinant human erythropoietin reduces apoptosis and inflammation and promotes myelin repair and functional recovery following spinal cord compressive injury in rats. Restor Neurol Neurosci. (2015) 34:647–63. doi: 10.3233/RNN-150498
55. Wu MF, Zhang SQ, Gu R, Liu JB, Li Y, Zhu QS. Transplantation of erythropoietin gene-modified neural stem cells improves the repair of injured spinal cord. Neural Regen Res. (2015) 10:1483–90. doi: 10.4103/1673-5374.165521
56. Marcon RM, Cristante AF, de Barros TF, Ferreira R, Dos SG. Effects of ganglioside G(M1) and erythropoietin on spinal cord lesions in rats: functional and histological evaluations. Clinics (São Paulo). (2016) 71:351–60. doi: 10.6061/clinics/2016(06)11
57. Zhao L, Jing Y, Qu L, Meng X, Cao Y, Tan H. Expression of adrenomedullin in rats after spinal cord injury and intervention effect of recombinant human erythropoietin. Exp Ther Med. (2016) 12:3680–4. doi: 10.3892/etm.2016.3832
58. Kökoglu Ç, Delen E, Arslantaş A, Arslantaş D, Kökoglu B, Özbek Z, et al. Therapeutic efficacy of tadalafil and eriythropoietin in experimental spinal cord injury. Ulus Travma Acil Cerrahi Derg. (2016) 22:521–5. doi: 10.5505/tjtes.2016.37571
59. Li J, Guo W, Xiong M, Zhang S, Han H, Chen J, et al. Erythropoietin facilitates the recruitment of bone marrow mesenchymal stem cells to sites of spinal cord injury. Exp Ther Med. (2017) 13:1806–12. doi: 10.3892/etm.2017.4182
60. Ozkunt O, Sariyilmaz K, Gemalmaz HC, Gürgen SG, Yener U, Dikici F. Investigation of efficacy of treatment in spinal cord injury: Erythropoietin versus methylprednisolone. J Orthop Surg (Hong Kong). (2017) 25:2309499017739481. doi: 10.1177/2309499017739481
61. Zhang H, Fang X, Huang D, Luo Q, Zheng M, Wang K, et al. Erythropoietin signaling increases neurogenesis and oligodendrogenesis of endogenous neural stem cells following spinal cord injury both in vivo and in vitro. Mol Med Rep. (2018) 17:264–72. doi: 10.3892/mmr.2017.7873
62. Wang P, Xie ZD, Xie CN, Lin CW, Wang JL, Xuan LN, et al. AMP-activated protein kinase-dependent induction of autophagy by erythropoietin protects against spinal cord injury in rats. Cns Neurosci Ther. (2018) 24:1185–95. doi: 10.1111/cns.12856
63. Barros A, Cristante AF, Santos G, Natalino R, Ferreira R, Barros-Filho T. Evaluation of the effects of erythropoietin and interleukin-6 in rats submitted to acute spinal cord injury. Clinics. (2019) 74:e674. doi: 10.6061/clinics/2019/e674
64. Li Y, Wang H, Ding X, Shen J, Zhou H, Jiang D, et al. Human brain-derived neurotrophic factor gene-modified bone marrow mesenchymal stem cells combined with erythropoietin can improve acute spinal cord injury. Dose Response. (2020) 18:1559325820910930. doi: 10.1177/1559325820910930
65. Zhong L, Zhang H, Ding ZF, Li J, Lv JW, Pan ZJ, et al. Erythropoietin-induced autophagy protects against spinal cord injury and improves neurological function via the extracellular-regulated protein kinase signaling pathway. Mol Neurobiol. (2020) 57:3993–4006. doi: 10.1007/s12035-020-01997-0
66. Esterbauer H, Cheeseman KH. Determination of aldehydic lipid peroxidation products: malonaldehyde and 4-hydroxynonenal. Methods Enzymol. (1990) 186:407–21. doi: 10.1016/0076-6879(90)86134-H
67. Pryor WA. On the detection of lipid hydroperoxides in biological samples. Free Radic Biol Med. (1989) 7:177–8. doi: 10.1016/0891-5849(89)90010-5
68. Zhou X, He X, Ren Y. Function of microglia and macrophages in secondary damage after spinal cord injury. Neural Regen Res. (2014) 9:1787–95. doi: 10.4103/1673-5374.143423
69. Luo Y, Fu C, Wang Z, Zhang Z, Wang H, Liu Y. Mangiferin attenuates contusive spinal cord injury in rats through the regulation of oxidative stress, inflammation and the Bcl2 and Bax pathway. Mol Med Rep. (2015) 12:7132–8. doi: 10.3892/mmr.2015.4274
70. Liu J, Wu Y. Electro-acupuncture-modulated miR-214 prevents neuronal apoptosis by targeting Bax and inhibits sodium channel Nav13 expression in rats after spinal cord injury. Biomed Pharmacother. (2017) 89:1125–35. doi: 10.1016/j.biopha.2017.02.077
71. Wang Y, Li C, Gao C, Li Z, Yang J, Liu X, et al. Effects of hyperbaric oxygen therapy on RAGE and MCP-1 expression in rats with spinal cord injury. Mol Med Rep. (2016) 14:5619–25. doi: 10.3892/mmr.2016.5935
72. Geng CK, Cao HH, Ying X, Zhang HT Yu HL. The effects of hyperbaric oxygen on macrophage polarization after rat spinal cord injury. Brain Res. (2015) 1606:68–76. doi: 10.1016/j.brainres.2015.01.029
73. Sun Y, Liu D, Su P, Lin F, Tang Q. Changes in autophagy in rats after spinal cord injury and the effect of hyperbaric oxygen on autophagy. Neurosci Lett. (2016) 618:139–45. doi: 10.1016/j.neulet.2016.02.054
74. Shiehmorteza M, Ahmadi A, Abdollahi M, Nayebpour M, Mohammadi M, Hamishehkar H, et al. Recombinant human erythropoietin reduces plasminogen activator inhibitor and ameliorates pro-inflammatory responses following trauma. Daru. (2011) 19:159–65.
75. Arishima Y, Setoguchi T, Yamaura I, Yone K, Komiya S. Preventive effect of erythropoietin on spinal cord cell apoptosis following acute traumatic injury in rats. Spine. (2006) 31:2432–8. doi: 10.1097/01.brs.0000239124.41410.7a
76. Simon FH, Erhart P, Vcelar B, Scheuerle A, Schelzig H, Oberhuber A. Erythropoietin preconditioning improves clinical and histologic outcome in an acute spinal cord ischemia and reperfusion rabbit model. J Vasc Surg. (2016) 64:1797–804. doi: 10.1016/j.jvs.2015.10.011
77. Hu Q, Li T, Wang L, Xie Y, Liu S, Bai X, et al. Neuroprotective effects of a smoothened receptor agonist against early brain injury after experimental subarachnoid hemorrhage in rats. Front Cell Neurosci. (2016) 10:306. doi: 10.3389/fncel.2016.00306
78. Miki T, Miura T, Hotta H, Tanno M, Yano T, Sato T, et al. Endoplasmic reticulum stress in diabetic hearts abolishes erythropoietin-induced myocardial protection by impairment of phospho-glycogen synthase kinase-3beta-mediated suppression of mitochondrial permeability transition. Diabetes. (2009) 58:2863–72. doi: 10.2337/db09-0158
79. Tabas I, Ron D. Integrating the mechanisms of apoptosis induced by endoplasmic reticulum stress. Nat Cell Biol. (2011) 13:184–90. doi: 10.1038/ncb0311-184
80. Dibble CC, Cantley LC. Regulation of mTORC1 by PI3K signaling. Trends Cell Biol. (2015) 25:545–55. doi: 10.1016/j.tcb.2015.06.002
81. Nakano N, Matsuda S, Ichimura M, Minami A, Ogino M, Murai T, et al. PI3K/AKT signaling mediated by G proteincoupled receptors is involved in neurodegenerative Parkinson's disease (Review). Int J Mol Med. (2017) 39:253–60. doi: 10.3892/ijmm.2016.2833
82. Sangawa A, Shintani M, Yamao N, Kamoshida S. Phosphorylation status of Akt and caspase-9 in gastric and colorectal carcinomas. Int J Clin Exp Pathol. (2014) 7:3312–7.
83. Ma H, Ma JX, Xue P, Gao Y, Li YK. Osteoblast proliferation is enhanced upon the insulin receptor substrate 1 overexpression via PI3K signaling leading to down-regulation of NFkappaB and BAX pathway. Exp Clin Endocrinol Diabetes. (2015) 123:126–31. doi: 10.1055/s-0034-1390422
84. Ahn YH, Lee G, Kang SK. Molecular insights of the injured lesions of rat spinal cords: Inflammation, apoptosis, cell survival. Biochem Biophys Res Commun. (2006) 348:560–70. doi: 10.1016/j.bbrc.2006.07.105
85. Dziennis S, Alkayed NJ. Role of signal transducer and activator of transcription 3 in neuronal survival and regeneration. Rev Neurosci. (2008) 19:341–61. doi: 10.1515/REVNEURO.2008.19.4-5.341
86. Chin YE, Kitagawa M, Kuida K, Flavell RA, Fu XY. Activation of the STAT signaling pathway can cause expression of caspase 1 and apoptosis. Mol Cell Biol. (1997) 17:5328–37. doi: 10.1128/MCB.17.9.5328
87. Kumar A, Commane M, Flickinger TW, Horvath CM, Stark GR. Defective TNF-alpha-induced apoptosis in STAT1-null cells due to low constitutive levels of caspases. Science. (1997) 278:1630–2. doi: 10.1126/science.278.5343.1630
88. Takagi Y, Harada J, Chiarugi A, Moskowitz MA. STAT1 is activated in neurons after ischemia and contributes to ischemic brain injury. J Cereb Blood Flow Metab. (2002) 22:1311–8. doi: 10.1097/01.WCB.0000034148.72481.F4
89. Akisu M, Durmaz B, Koroglu OA, Unlubay S, Yalaz M, Akin H, et al. The effects of IGF-1 and erythropoietin on apoptosis and telomerase activity in necrotizing enterocolitis model. Pediatr Res. (2021) 90:559–64. doi: 10.1038/s41390-020-01195-7
90. Asker ME, Ali SI, Mohamed SH, Abdelaleem R, Younis NN. The efficacy of bone marrow-derived mesenchymal stem cells and/or erythropoietin in ameliorating kidney damage in gamma irradiated rats: Role of non-hematopoietic erythropoietin anti-apoptotic signaling. Life Sci. (2021) 275:119388. doi: 10.1016/j.lfs.2021.119388
91. Zhang Y, Wang Q, Liu A, Wu Y, Liu F, Wang H, et al. Erythropoietin Derived Peptide Improved Endoplasmic Reticulum Stress and Ischemia-Reperfusion Related Cellular and Renal Injury. Front Med. (2020) 7:5. doi: 10.3389/fmed.2020.00005
Keywords: erythropoietin, spinal cord injury, neurological recovery, antioxidant, meta-analysis
Citation: Zhang Y-y, Yao M, Zhu K, Xue R-r, Xu J-h, Cui X-j and Mo W (2022) Neurological recovery and antioxidant effect of erythropoietin for spinal cord injury: A systematic review and meta-analysis. Front. Neurol. 13:925696. doi: 10.3389/fneur.2022.925696
Received: 22 April 2022; Accepted: 27 June 2022;
Published: 19 July 2022.
Edited by:
Hua Feng, Army Medical University, ChinaReviewed by:
Rodolfo Gabriel Gatto, Mayo Clinic, United StatesSarah C. Hellewell, Curtin University, Australia
Copyright © 2022 Zhang, Yao, Zhu, Xue, Xu, Cui and Mo. This is an open-access article distributed under the terms of the Creative Commons Attribution License (CC BY). The use, distribution or reproduction in other forums is permitted, provided the original author(s) and the copyright owner(s) are credited and that the original publication in this journal is cited, in accordance with accepted academic practice. No use, distribution or reproduction is permitted which does not comply with these terms.
*Correspondence: Xue-jun Cui, MTM5MTc3MTU1MjRAMTM5LmNvbQ==; Jin-hai Xu, amluaGFpeHVAMTI2LmNvbQ==; Wen Mo, bG9uZ2h1YWdza0AxNjMuY29t
†These authors have contributed equally to this work