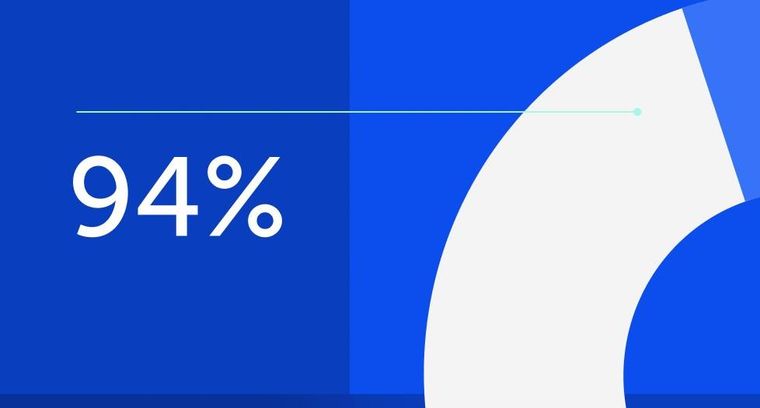
94% of researchers rate our articles as excellent or good
Learn more about the work of our research integrity team to safeguard the quality of each article we publish.
Find out more
REVIEW article
Front. Neurol., 07 July 2022
Sec. Pediatric Neurology
Volume 13 - 2022 | https://doi.org/10.3389/fneur.2022.922322
This article is part of the Research TopicInsights in Pediatric Neurology: 2021View all 20 articles
We provide evidence to support the contention that many aspects of Autistic Spectrum Disorder (ASD) are related to interregional brain functional disconnectivity associated with maturational delays in the development of brain networks. We think a delay in brain maturation in some networks may result in an increase in cortical maturation and development in other networks, leading to a developmental asynchrony and an unevenness of functional skills and symptoms. The paper supports the close relationship between retained primitive reflexes and cognitive and motor function in general and in ASD in particular provided to indicate that the inhibition of RPRs can effect positive change in ASD.
The term “primitive reflex” was first used by Buckley (1). Primitive or infantile reflexes are sensory/motor reflexes that are present at birth. Most of these reflexes are present in the womb (2), and one of their functions is to help the child “birth itself.” The primary function of primitive reflexes is to allow the infant to move and react to their environment leading to the maturation of the motor system (3, 4). Children need to move, feed, protect, and orient to engage their senses and muscles and create sensory and motor feedback that will activate genes allowing the brain to be built from the bottom up (3–5). The control of these reflexes arises from multiple brainstem regions. The lower reflexes in the medulla are thought to be active first, followed by reflex control associated with the pons and mesencephalon (6–10).
Nevertheless, if there is a delay or disruption to this bottom-up projection known as “bottom-up interference,” then the later, more advanced areas of the brain may be delayed in development. This could then delay or prevent the top-down maturational processes that ultimately inhibit these reflexes (11–13). Babinski also noted that not only the response delay of the downward toes in the plantar reflex but also the asymmetry of this response had clinical significance. Asymmetry of the Babinski sign is significant and may relate to a functional maturational dysfunction of the corticospinal tract (14–16).
Several authors have emphasized that frontal lobe development eventually leads to top-down control and inhibition of primitive reflexes. If there is degeneration or damage to the frontal lobe or corticospinal tract later in life, these reflexes can return. They are considered to be frontal release signs (17–20).
The controversy surrounding RPRs is not whether they exist or not. Although not typically part of the current pediatric examination, primitive reflex testing was previously included as part of a routine pediatric neurology examination. They are, however, a well-accepted part of the evaluation of effective child development (3, 7, 21–23), whose normal temporal trajectory is reported in Figures 1, 2. The controversy surrounds the inhibition of these reflexes. In mainstream pediatrics, it is assumed that primitive reflexes are completely inhibited by the end of the infant's first year postpartum. However, many studies have indicated that in a certain percentage of the population, primitive reflexes are not inhibited in the first year of life and persist into middle childhood and even into adulthood (24, 25).
Figure 1. Development of postural reflexes. The diminishing of primitive reflexes and the growing importance of postural reactions indicate the development of requisite conditions for the development of the purposeful movement. The collective time course of primitive reflexes under normal circumstances is compared with the time course of the maintenance of static deep tendon reflexes and postural reactions. Reproduced with permission Pedroso FS. Reflexes. In: Haith MM, Benson JB, editors. Encyclopedia of Infant and Early Childhood Development. San Diego, CA: Academic Press (2008). p. 11–23.
It has also been documented that in children, adolescents, and adults with RPRs, a neurobehavioral disorder or “learning disability” coexists (4, 26, 27). Individuals with ADHD, (25) autism, (28) Tourette's, (29) dyslexia (30), or other neurobehavioral disorders, frequently demonstrate RPRs that are thought to be related to maturational delay in the nervous system (3, 31–34).
Movement allows us to interact with the environment in more sophisticated ways and can help improve our chances of survivability. Interacting with the environment purposefully and beneficially requires the development of sensory organs that supply the individual with information about our location, potential danger, and satisfaction, allowing one to negotiate the surroundings and develop a sensory-motor map of the world that we can use for prediction and goal-directed adaptive behavior (35, 36).
Organisms developed brains because they moved, and as they moved, they interacted with their environment in increasingly complex ways, leading to the development of a more complex brain (37, 38). Bipedalism is the most complex of movement strategies that have evolved in any organism, and it is, for the most part, unique to hominids. The relatively large brains of humans support the distinctive erect bodily position and cognitive abilities driven by bipedalism (39). Upright bipedalism permits less flexibility of the human pelvis's anatomy and size and structure in the human pelvis compared with quadrupeds (40). Relatively large-headed infants are born to mothers with relatively small birth canals. The infant's skull and brain cannot be fully developed at birth for the mother and neonate to survive the birth process unscathed (41, 42).
To stimulate the growth and development of the brain, an infant needs to move and interact with its environment (43–45). Movement can be impeded by a brain and nervous system that is not sufficiently mature at birth. What makes some volitional movement possible are the infantile primitive reflexes already intact at birth that allow for reflexogenic movement and interaction with the environment in fundamental ways that help increase the chances of survival. These reflexes appear prenatally and are thought to aid in the birth process. Most of these reflexes are present at birth and then become inhibited within the first few months, with the longest (the plantar reflex) remaining until the end of the first year postnatally. These reflexes allow for basic reflexogenic movement contributing to early motor milestones such as rolling over, creeping, crawling, grasping, sucking, and eventually crawling and walking (43, 44). Postural reflexes that allow for more sophisticated individualized movements are replaced with voluntary movement in most cases. Primitive reflexes allow for basic movements, which allow for simple interaction with the environment and form the basis of the early movement as well as in the stimulation of sensory organs and receptors. This increase in sensory feedback and stimulation is thought to result in the expression of genes related to protein synthesis and the building of functional connections (46). The stimulation of glial cell proliferation increases the size and connectivity of neurons (47). As neurons grow in size, density, and connectivity, they will eventually inhibit, through propriospinal projections, lower or more primitive areas of the brain. They will stimulate the growth and activation of higher, more sophisticated regions of the brainstem and neocortex. While primitive reflexes eventually become inhibited or integrated, they are never entirely eliminated (48). Ultimately, all reflexes seem to come under the control of the frontal lobe (17, 18, 49–51).
In individuals with frontal lobe damage, dysfunction, or degeneration, the reappearance of primitive reflexes known as frontal release signs is oftentimes manifested (17, 18, 34, 49–56).
Upper motor lesions also do not infrequently result in the reappearance of primitive reflexes such as the Babinski reflex or plantar reflex (57). This is thought to be associated with the loss of the descending inhibitory connections from the cortical spinal tract, which reflects the maturation and growth of the frontal lobe and the sensory-motor cortex.
It has also been noted (58) that the presence of RPRs is a common feature of children with Autism Spectrum Disorder (ASD) (28, 58–60). In most of these disorders, there is no visible damage, injury, lesion, or degeneration as a basis for hypothesizing that the RPRs reflect a maturational delay of brain areas that would typically inhibit these reflexes, especially those in feedback with the frontal lobes (61–63).
The absence or reduction of environmental influences that would generally promote growth and development, and neuroplasticity within higher brain regions, would typically lead to the inhibition of primitive reflexes and the expression of postural reflexes (3, 64) according to the timeline represented in Figure 1 and in Table 1. The persistence of these primitive reflexes can reflect a maturational lag. The RPRs, especially with asymmetric persistence, will reflect not only a maturational delay of the brain but may also indicate, depending on the timing, abnormal asymmetrical development of the hemispheres (64, 65). In children with cerebral palsy, an injury on one side of the brain can lead to asymmetric retention or lack of development of PRs (28, 66, 67).
According to our current understanding, the prevalence of RPRs is considered variable, and there is disagreement about the pathological significance of these reflexes in both aging and child development. However, evidence from large data sets indicates a significant relationship between RPRs, maturation, and cognitive function (3, 25), and the description of these reflexes is presented in Table 1.
The relationship between cognitive deficits and RPRs has been controversial. Some authors consider these reflexes predictive of diffuse cerebral dysfunction as these signs are significantly correlated with cognitive deficits in a wide age range of individuals (68–73). It is therefore important to differentiate “retained primitive reflexes” from “returned primitive reflex” (RtPR). Recently, RtPRs have been described in dementia and Parkinson's Disease (74–78). While primitive reflexes are considered adaptive responses that are present in the neonate and disappear or are inhibited as the brain matures, RtPRs can reappear in childhood, adolescence, and adulthood (78) and when they do so, they are reportedly invariably associated with cognitive effects (74, 78). Some authors consider that RtPRs (in particular the Babinski and grasp reflex) are indicative of diffuse cerebral dysfunction as there exists a significant correlation between these signs and cognitive dysfunction in a wide age range of individuals (69–73). Regarding RtPRs, some authors reported that in individuals with Alzheimer's disease, no relation existed between cerebral atrophy based on psychometric testing (e.g., Wechsler Memory Scale) or CT-scan and grasp, snout, or glabellar reflexes.
Retained primitive reflexes indicate cortico-subcortical neuronal network impairment or possibly neuronal developmental delay. Some authors have stated that RPRs are evidenced in neurotypical populations. The palomental reflex, for example, was found in 6–27% of individuals aged between 20 and 50 years, and 28–60% of those above 60 years (64), snouting in 13% of individuals between 40 and 57 years (64); 22–33% of those above 60 years of age (64), and the sucking reflex, which some authors associate with “frontal lobe disease” (79), and Tarawneh and Galvin (80) had noted that in neurotypical individuals between 73 and 93 years of age, the palmomental reflex was evidenced in six percent them.
The Babinski sign (i.e., plantar response) and grasp reflex are two reflexes that are clinically accepted as indicators of central nervous system disease or disorder. Some of the arguments may be accounted for by differences in opinion and interpretation of the reflexes, which can vary significantly from clinician to clinician (4, 26, 81–83).
Another study examined the relationship between cognitive functioning and RPRs in individuals with dementia and without in order to determine the most predictive elements of cognitive testing or the neurological examination for brain dysfunction (84). Using the Cognitive Abilities Screening Instrument-Short Form (CASI-S), Gellis (85) concluded that in those with dementia, individuals with the highest primitive reflex (PR) scores tended to be associated with the lowermost cognitive scores and, in particular, to SPECT scan configurations. Therefore, these researchers concluded that the existence of numerous PRs and cognitive scores could be effective in predicting diffuse cerebral dysfunction. In particular, the presence of the Babinski and grasp responses, or the combination of the snout, suck, paratonia, and palmomental reflexes, are effective indicators of diffuse brain dysfunction, in particular when RtPRs are evidenced and complemented by deficits in cognitive testing scores.
The presence of multiple primitive reflexes is an indicator of diffuse brain dysfunction in elderly populations. Their persistence and presence in children and adolescents may indicate diffuse cortical maturational delay and correlate with cognitive and executive developmental absence or delay. If developmental milestones are not appropriately achieved, we hypothesize that synchronicity, optimization, the efficiency of behavioral-environmental interaction, coordination of movement, and synchronization of the overlapping brain will all be affected (25, 27, 43, 44, 86).
One of the unique features of the human brain is its degree of lateralization or asymmetry. Humans have the most asymmetrical and lateralized brains of any species. This is thought to be another factor that leads to the significant differences in intelligence between humans and other species. A more lateralized brain allows for the development of a greater variety of centers that can individually process and control numerous functions, combining these individual centers into various networks leading to the unique cognitive abilities shared by humans. This lateralization develops with increasing age, brain, and nervous system development (87–93).
A small child does not have the same degree of lateral asymmetry as an adult. Laterality is a product of the maturity of the brain and especially of the neocortex and the frontal lobes (43, 89). The development of laterality and asymmetric control of functions increases the cognitive potential and requires greater coordination and synchronization of cortical networks (94, 95). For various functions to bind together, all associated brain regions that control complex functions and their networks must be activated simultaneously. This coordination is a byproduct of maturity (4, 21, 43).
As the brain grows and as neurons become interconnected, the speed and coordination of inter- and intra-hemispheric cortical networks increases, allowing for synchronization and integration of a significantly greater number of functions. The two hemispheres of the brain do not develop simultaneously; the right hemisphere is thought to develop more rapidly and earlier than the left, with the most significant development being prenatal and for the first 2–3 years of life (96, 97). Then the left hemisphere is significantly more greatly emphasized in development for the next 2–3 years of life. Once the differences and advantages are established during the first 6 years of life, this forms the basis of hemispheric specialization and lateralization that will increase throughout development (87, 90–93, 97).
Where neural connections (anatomic and functional) are not adequately developed in infancy and early childhood, asynchronicity, the inefficiency of behavioral-environmental interaction, coordination of movement, and synchronization of brain networks may be evidenced (40, 98–104).
A global immaturity of the function of cortical networks in childhood can be associated with a reduction in motor activity (3), spatial exploration (105), experience-dependent plasticity (106), RPRs and delayed postural reflexes (107, 108). A more specific dysfunctionality would be expected if there was asymmetric development of RPRs. If there existed unilateral RPRs and, in particular, unilateral delay of postural reflexes, we would expect an asymmetric maturity and growth of the brain since this would be associated with an asymmetry of tone, in turn altering sensory and muscle feedback, potentially impairing the main driving factors to brain development (109).
Futagi et al. examined the relationship between plantar grasp response asymmetry during infancy and neurological outcome. They, during a follow-up period of between 2.8 and 11.9 years, reviewed the neurologic consequences of 61 children having demonstrated, during infancy, asymmetric plantar grasp responses. All children demonstrated neurological signs or perinatal risk factors during infancy. Futagi and colleagues reported intellectual disabilities in three, borderline intelligence in nine individuals, cerebral palsy in 38, delayed motor development in six, and neurotypical function in five. The majority exhibited a relationship between the side of the retained plantar grasp response, the side of the motor function deficits, and the side of the abnormal CT results (6). These findings were supported in a systematic review by Hamer and Hadders-Algra (110).
The asymmetry that Futagi et al. (1995) observed in the plantar grasp response strongly suggested brain dysfunction (6, 111, 112). Their studies showed an association between the persistence of motor abnormalities related to the same body side and the asymmetric development of primitive reflexes (113). The plantar response is one of the reflexes most related to brain dysfunction, whether due to injury or functional developmental delays (108, 114). This type of asymmetrical development of the developing brain has been commonly noted in almost all neurobehavioral disorders, especially ASD (115, 116). Along with anatomical asymmetries, there have also been functional asymmetries noted with a characteristic “unevenness” of skills observable in all of these disorders, to varying degrees (25).
A significant feature of those with ASD is the “unevenness” of cognitive function (117). We have proposed (43) that the diverse aberrant behaviors noted in ASD and in other neurobehavioral disorders can be understood better by viewing ASD in the context of functional brain disconnectivity, of the kind that has been noted in minimally conscious states (118, 119) and even in sleep (120), or as reported in people with dyslexia (121, 122). Functional asymmetry within widespread cortical networks could decrease temporal coherence in certain functional networks and enhance temporal coherence in others (123). Recent research has suggested that increased functional ability or intelligence is related to augmented activity in specific networks (124). It is also possible that an increase in the complexity and integration of functional networks may be related to increased temporal coherence that may impart a selective advantage in particular regions of the brain (125, 126). This could explain how certain talents and abilities seem to be inherited and run in families (127).
As optimized brain function implies more efficient neural processing than non-optimized, one might expect optimized execution of motor tasks to be related to greater degrees of activity. However, the converse appears to be the case in the cerebral cortex where increased task effectiveness has been reported that has included: figural, numeric, and spatial reasoning (128) and verbal ability (129) is associated with reduced energy consumption in various cortical regions. This phenomenon has additionally been examined electrophysiologically. When examining resting-state activity [event-related desynchronization (ERD)] during cognitive tasks, there is a reported decrease in background power (7.5–12.5 Hz) decreases which has been reported to be related to the activity recorded in those with higher scores on IQ tests (130, 131), or with significantly greater performance after practice, that in turn is related to a more effective cognitive processing strategy (132, 133). Yet, the issues should include not only the expenditure of energy but also the nature of the functional connectivities between brain regions (94). Smaller regions of activity have been consistently evidenced in brain areas in those with ASD. These diminished areas of activity appear to be developmentally delayed brain regions as opposed to being reflective of pathological processes or damage (94, 95).
Individuals with ASD and other neurobehavioral disorders have also evidenced a reduction of interregional brain connectivity (25, 27, 43, 44, 94, 95, 102, 134–139). The corpus callosum appears to be the brain area associated with the reduced cortical connectivity found in individuals with ASD (140). This implies that the most frequently evidenced functional disconnectivity observed in childhood involves hemispheric interaction. This is a notable reported characteristic difference between ASD and normally developing toddlers (141).
We think that reduced inter-hemisphere coherence is associated with a reduction in the several sensory, motor, and cognitive functions coordinated by the ipsilateral brain hemisphere, and the higher proficiencies are sometimes related to enhanced within-hemisphere coherence (43, 94, 95). We have also described diminished coherence and connectivity in longer inter-hemispheric connections with augmented coherence and connectivity with shorter intra-hemispheric connections (94, 95) that we have hypothesized to be associated with enhanced performance abilities such as those that have been observed in forms of savantism (142).
Retained primitive reflexes have been noted in several neurobehavioral disorders, including ADHD and ASD and are understood to be associated with or absent or delayed developmental milestones in these (25, 27, 28, 44, 143–148). RPRs have been reportedly associated with the presence of clumsiness (25, 27, 43, 149, 150) incoordination (149), awkward posture (151), gait (152–154), and other motor disturbances (25, 155, 156). Most neurobehavioral disorders seem to be associated with motor incoordination and cognitive dysfunction (25, 27, 43, 44, 157, 158).
Teitelbaum et al. (159) theorized that in infants with movement disturbances, reflexes may have “gone astray” and may be early markers of ASD. They observed that some infants demonstrated RPRs that continued far beyond long infancy in the children they examined, whereas other primitive reflexes first appeared in infancy significantly later than though ought. The asymmetric tonic neck reflex, they thought, might be retained in ASD. The verticalization of the head as a consequence of body tilt, was reportedly absent in a subgroup of “autistic-to-be” infants, according to Teitelbaum and colleagues. They suggested that these reflexes might serve as a marker for ASD, and pediatricians could use them to screen for neurological dysfunction (160). In their earlier work, Teitelbaum et al. (161) showed that infants with a tendency to ASD demonstrated a distinctive constellation of disturbances in patterns of movement as early as 4–6 months of age, measured by Teitelbaum and colleagues in conjunction with laser disc still-frame analysis. Eshkol and Wachman (162) had earlier reported similar findings.
The Galant and Moro reflexes are among the most critical postnatal primitive reflexes that diminish later in development. At the same time, there exists no definitive evidence that these reflexes play a role in ADHD. Konicova and Bob studied school-aged ADHD children between 8–11 years who demonstrated Galant and Moro RPRs compared to an age-matched control group (72, 163). They found that ADHD children demonstrated a significantly greater occurrence of Moro and Galant reflexes than did the control group, indicating that ADHD symptoms may compensate for an immature brain.
Callcott (164) reported that children's learning difficulties relate to reduced movement proficiency, including school-readiness. Calcott investigated the prevalence and severity of the Asymmetrical Tonic Neck Reflex (ATNR) and studied the proficiency of movement in preprimary-aged Western Australian indigenous children. She found that 65% of those tested demonstrated moderate to high ATNR levels that were significantly related to academic achievement (164).
The findings of the Millennium Cohort Study in the United Kingdom (165) supported a relationship between the delayed achievements of motor milestones at 9 months of age and significantly lower cognitive development at age five (165). The Australian Early Development Index reported that nearly a fourth of school-aged are “at risk” in their physical and cognitive development (166). Williams and Holley (167) offered support for these findings linking motor development and cognition by addressing the influence that infant motor experiences in infancy and early childhood may have on higher-level cognitive abilities required for academic achievement in school. As we have already noted, motor function and gesture development typically require the effective inhibition of mouth and hand-related primitive reflexes (167). ASD children not infrequently demonstrate difficulty in executing skilled movements and possess as well as exhibit a poor gesture repertoire (168).
Chinello et al. (144) examined the association between three RPRs, motor behavior, and parental autistic-like traits, in infants aged between 12 and 17 months of age. Independent of age, RPRs were associated with infants' deficient motor skills and were highly correlated with parental autistic-like characteristics.
Numerous authors have reported on an association between clumsiness and incoordination, particularly in gait and posture, and ASD, ADHD, and other neurobehavioral disorders of childhood (163, 169, 170). The kind of gait and motor dysfunction has been mainly thought to possess either basal ganglia or cerebellar origins (25, 27, 40, 43, 171). Developmental Coordination Disorder (DCD), or more simply put, motor incoordination or “clumsiness,” is also usually of the same type, primarily involving the muscles that control gait and posture or gross motor activity (172, 173).
Sometimes, we observe that fine motor coordination can also be affected (21, 174, 175). Several authors have noted both differences and similarities between ASD and DCD. DCD individuals demonstrated greater fine and gross motor coordination, theory of mind, and emotional perception than did the ASD individuals, but evidenced comparable difficulties with response inhibition. These authors observed that based on symptom severity, children with ASD who were measured to be “more able” did not diverge on any measured skills from DCD children, in contradistinction to children classified as “less able.” The authors wondered whether DCD and ASD vary more in the range of symptom severity than in a singular behavioral domain (174).
Similar comorbidities have been found in children with ADHD (72, 163, 176–180) and those with developmental dyslexia (4, 181–186).
Sumner et al. (187) also found numerous overlapping features in verbal expression, speech, gaze, and face-processing, expression, in ASD and DCD individuals. These findings suggest that children with DCD may also demonstrate difficulties in processing social information. However, when examined with measures of socialization, the DCD individuals scored at an intermediate level in two other socialization measures. The authors concluded that socialization in DCD may not be as manifest as in individuals with ASD (187).
The greatest similarity between ASD and DCD in Sumner and colleagues' review was a paucity of significant effects of cognitive intervention. Concerning treatment, no significant improvement effects were noted in both DCD and ASD groups of individuals (174) or in IQ (188). The ability to train and improve in various domains, especially cognitive, is similar for both conditions. Additionally, no significant disparities were found in a qualitative study examining transitions from primary to secondary school, possibly because the main variables of the study's interest concerned the children's motor behaviors. On the other hand, cognitive intervention has been reported to be effective in reducing symptoms of ADHD (189).
It might seem somewhat confusing initially to observe that fine motor skills seem to be disrupted at almost equal levels as a gross motor. The literature supports the notion that manual dexterity is less effective for high functioning ASD individuals, but only for the non-dominant hand. This suggests a lateralized difference (190, 191). This would show that although fine motor coordinative skill is decreased in those with ASD and fine motor skill is primarily decreased in the left hand, associated with right hemisphere function. This is consistent with a deficit in effective coherence between the right and left hemispheres. Perhaps a parallel situation exists in ADHD individuals and in individuals with other neurobehavioral disorders (192–196).
Variations in the manifestations of ASD and DCD may be associated with differences in the maturation of asymmetries as a consequence of different maturational rates of the left and right hemispheres (197–199). Asymmetric RPRs may also be an early marker of developmental brain immaturity. This aberrant configuration of hemispheric asymmetry may be related to underconnectivity and desynchronization, and eventually to functional disconnectivity between lower brain regions and the neocortex (197–200).
Autistic Spectrum Disorder is a neurobehavioral disorder identifiable by dysfunction of communication, behavioral flexibility, eye contact, and social interaction as well as deficits in language, and executive function (201–204). Although there is a consensus about the symptoms that comprise ASD, there exist controversies regarding the precise definitions of ASD and the boundaries between manifestations of related disorders. Researchers have increasingly recognized that motor ability can have a significant effect on other developmental functions, such as language and social cognition (28, 205–209).
Retained primitive reflexes can disturb the natural course of development and create difficulties in social and educational functions in children (21, 210) as well as impact psychomotor development (211). Mature responses in a child's psychomotor behavior can only occur if the central nervous system has reached the appropriate level of maturity (21, 210, 212). The process consists of the transition from brainstem reflex response represented in Figure 2 to cortically controlled responses (213).
It has been argued that independent of a child's age, RPRs are significantly related to an infant's ability to interact with objects (i.e., agency) (4, 21, 144, 156, 210) as well as with others (i.e., copying gestures) (22), meaning that high scores in the assessment of primitive reflexes, is associated with an increased likelihood of RPRs, which, in turn, are also associated with low scores in motor responsivity, independent of the age of the infant.
As previously indicated, children with ASD demonstrate impediments in the performance of skilled movements and gestures. Numerous investigators have noted that delays in the maturation of motor function during the early years of development foretell the primary dysfunctions characteristic of individuals with ASD (214–216).
This hypothesis has been examined in the infant siblings of ASD children, who purportedly have an increased probability of developing ASD. In longitudinal studies of 3- to 6-month-old infants' motor development of high-risk (HR) infants, over 70% of infants with motor delay later demonstrated communication impairment. Motor development is associated with a normally automatic progression in which infant maturation inhibits more primitive motor responses (217–219).
Assessing RPRs in autism is essential for at least multiple purposes. Firstly, RPRs may be an encouraging early sign of ASD that, along with the early signs of difficulty in eye contact, attentional deficits, as well as other elements, might assist in characterizing the developmental trajectory of the wider ASD phenotype during infancy. Consistent with this thinking, it has been stated that slight disparities in initial periods of the brain's development (e.g., the persistence of primitive reflexes) can produce an adverse progressive effect not just on motor skills that develop later but also on a range of other behaviors (i.e., communicative and social behaviors as well as in object exploration) (144, 220, 221). Secondly, the ability to identify motor abnormalities early in life might also be encouraging for the differential diagnosis of ASD. The proportion of children with ASD and concomitantly with developmental motor and coordination dysfunction varies widely. The variability in these deficits in ASD is likely a result of the heterogeneity of ASD. That heterogeneity, on the other hand, allows us a unique opportunity to classify subtypes of ASD (101, 194, 195).
Retained primitive reflexes have the ability to disturb the normal maturation processes decreasing the ability of the brain to effectively process sensory information. RPRs then that are still present (beyond the average age of 12 months postpartum) can impede the subsequent development and maturation as well as serve as a potential biomarker of neurological dysfunction (144).
In supporting the cognitive effects of primitive reflexes and cognitive function, some authors consider the palmomental reflex (PMR) as being related to dementia. The PMR is a polysynaptic reflex that can be evoked by nociceptive stimulation of the thenar eminence, resulting in an ipsilateral involuntary mentalis muscle contraction (19). The extant PMR is found in infants up to ~12 months of age and then wanes and disappears, largely due to the frontal lobe maturation (19, 222). Consequentially, its recurrence in aged individuals with the pathology of the frontal lobe is thought of as a “cortical release” or “frontal lobe” sign, with a presumption of a lack of frontal inhibition on subcortical motor networks (223–225).
Anatomic (AC) and functional connectivity (FC) studies of linking the PMR with dysfunction of interrelating loops connecting the thalamus and basal ganglia with the motor, premotor, and prefrontal cortices, are consistent with our hypothesis (95, 190, 226–229).
Neuroimaging studies of individuals with ASD have also detected brain areas with atypical lateralization of motor function, with the capacity to detect subtle neuroendocrine phenotypes. Most studies agree that ASD individuals demonstrate an amplified rightward asymmetry that incorporates cerebral cortex volume, corpus callosum, premotor cortex, the sensorimotor resting network, and the inferior parietal lobule (190, 227, 228, 230, 231).
Floris et al. (190), in studying intra-hemispheric connectivity in ASD, demonstrated that high-functioning ASD children aged between 8 and 12 years demonstrated strong rightward lateralization in their motor circuitry's connectivity which was found to be necessary for effective motor responsivity. Notably, motor connectivity's rightward lateralization relates to effective motor response (e.g., gait and balance, as well as any timed sequential or movements) (190, 231).
Machado et al. (94) reported that qEEG changes in coherence and spectral analysis could be associated with a visual-auditory sensory integration impairment, which in ASD children is lateralized to the right hemisphere (94, 232, 233). Hence, several authors have affirmed that RPRs reflect anatomic and functional connectivity abnormalities in brain networks (190, 227, 228, 230, 231).
Several authors have suggested that RPRs correlate with motor function independent of the age of the infant, and significantly more so among infants whose parents demonstrated subclinical autistic characteristics. Hence, the RPRs might modify the developmental trajectory of the infant's motor function, and as a result, their assessment could serve as an early marker of atypical development (144, 156).
There exists a consensus that besides the principal features of ASD, delays, and abnormalities in motor development are key components of ASD regardless of multiple etiology and subtypes of the condition (25, 27, 44, 144, 156).
The anomalous frontostriatal cortex in AD individuals with RtPRs variously affects the motor pathways that suppress primitive reflexes. This notion is coherent with findings that ASD impacts various cognitive functions in the same way that dementia is related to dysfunction of the frontal lobes and/or basal ganglia (i.e., frontotemporal dementia or Parkinson's disease dementia). These findings in dementia have suggested functional and anatomic connectivity impairment in ASD and other neurodevelopmental disorders (19, 224).
Retained primitive reflexes may be one of the earliest markers of abnormal or delayed cortical maturation and by extension, of ASD and other neurobehavioral disorders (231, 234). The rooting and sucking reflexes and many other primitive reflexes are present at birth (43). The inability of an infant to attach to its mother and breastfeed, often seen in children with developmental delays and delays or asymmetry of rolling over at 3–5 months of age, may be the early indicators of ASD (3, 77, 235). Therapists have recommended exercises that stimulate or reproduce primitive reflexes to remediate various neurobehavioral disorders (26, 149, 236).
Methods that have indicated some promise in the treatment of various neurodevelopmental disorders including ASD are ostensibly founded on the theory that attributes the difficulties to RPRs that affect the child's normal growth and development as well as academic and cognitive skills (25, 237–239).
Although limited, some studies indicate a neurodevelopmental basis for a range of difficulties associated with maturation and motor development that manifest in cognitive and social difficulties. The collective research in this area demonstrates that the existence of RPRs has implications for skills such as balance and coordination as well as learning and cognition. The work of Goddard Blythe (240–243) has concentrated on children between 7 and 9 years of age and supports the case for early interventions to improve and develop coordination and balance, especially when such neurological dysfunction may be contributing to cognitive and motor delays or effects. Brown's (244) intervention study appears to support this line of research as does Melillo et al. (25). She found that with children between 4 and 5 years of age, practicing particular movements facilitated their performance of the fine motor activity and academic performance by inhibiting RPRs. Similar findings were reported by McPhillips and Mulhern [(239), cited in (245), p. 69] who indicated the relation between children with reading problems and motor control and balance. Chambers and Sugden's (246) found ineffectiveness in motor skill performance was highly associated with academic performance. Activities of daily living were found to be successfully facilitated by intervention programs that supported fine and gross motor skills thus being significant factors in early childhood learning [(247), p. 50]. The evidence indicates that physical development is fundamental to the development of a child's cognitive abilities.
Programs, based on perceptual-motor interventions have suggested that relatively simtime have not ple training is capable of moderating the facilitation of learning and brain structure (248, 249). In short, the difficulty is that the majority of current programs of this sort at the present time have not undergone rigorous evaluation and scrutiny. These types of interventional strategies invariably employ specific motor activities and exercises. Some of the advocated tasks are adjusted to the individual's needs (250), while others may be generic (251–253). These types of interventions often integrate actions, such as throwing and catching, ostensibly improving vestibular function, fine and gross motor skills, and academic accomplishment.
While some programs have promoted exercises that imitate the actions of fetuses and infants, it has been noted that rehearsing the activities of the early stages of development can inhibit the perseverance of RPRs. This has oftentimes been used as a justification for programs that advocate exercises that simulate fetal and infant activity and infants (238, 239, 254). Claims have been made that movements following primitive reflex patterns will inhibit those reflexes and improve cognitive function and the ability to acquire academic skills (239).
Grzywniak (255) studies the effectiveness of exercises aimed at supporting the development of children with learning difficulties and RPRs. Their symptoms included visual-motor coordination and attentional deficits, hyperactivity, and reduced visual and auditory analysis and synthesis.
While not many studies have thoroughly examined the effects of reducing RPRs on clinical outcomes in developmental disabilities in general and in ASD in particular, of note are Pimentel (256) and Anderson (143, 257), who discussed the connections between RPRs and developmental delays. The comparative studies scrutinized groups with developmental delays and RPRs (144, 160, 164, 238, 258). McPhillips and Mulhern (239) performed a double-blind study of the cognitive effects of reducing the presence of RPRs. A regression analysis study was conducted by de Bildt et al. (259). Melillo (260) and Hyatt et al. (252) produced meta-analyses. While at least eight systematic review articles examined, RPRs alone, or meta-analyses of RPRs in development, none explored the evidence surrounding reflex-based interventions (108, 159, 241, 252, 260–263). Expert review studies over many years included those by Rider (264), Endler (265), Ottenbacher (262), Smith et al. (266), and Mailloux et al. (267). The studies analyzed RPRs of individuals with reflex delays, developmental disabilities, and difficulties with sensory integration skills, but none provided evidence for effectiveness in the treatment of developmental disabilities of any kind by RPR reduction.
Barret et al. (268) identified three best evidence reviews (144, 238, 242). Goddard Blythe (242) provided a summary of independent studies that examined academic performance associated with developmental exercise programs that demonstrated positive effects on academic performance. Jordan-Black (238) conducted a nonrandomized control study to establish evidence for the effectiveness of reflex-based interventions in improving academic performance, from which she indicated that her system reduces RPRs in particular the asymmetrical tonic neck reflex, and has a significant effect on reading and mathematics abilities of children who had undergone her intervention. Chinello et al. (144) found that the parents of infants demonstrating subclinical traits of ASD were more likely to demonstrate RPRs and were more susceptible to developing ASD later. The study did not measure the outcome of therapeutic intervention. Collectively, these papers demonstrate the best evidence and relevance to RPR reduction and its effect on cognitive function. reflex integration. Only Goddard Blythe (242) and Chinello et al. (144), examined the effects of RPRs in developmental disorders in general and to some extent in ASD. Jordan-Black's (238) study did not address children with ASD.
Although the mechanism of how these exercises can inhibit primitive reflexes and affect or improve neurobehavioral disorders has not been previously described to our knowledge. We speculate that utilizing these exercises can increase the sensory stimulation and feedback to the nervous system that stimulates synaptogenesis and neuroplasticity of more rostral and complex areas of the brain (269–271). We conjecture that this may be associated with inhibition through descending propriospinal connections that inhibit these reflexes that would, under normal circumstances, lead to more complex individualized volitional control of movement that will stimulate growth and cortical maturity. Ultimately, this can lead to the release of “bottom-up interference” that can delay the cortex's maturation and prevent appropriate top-down regulation that will ultimately inhibit primitive reflexes (25, 27, 44, 94).
The incidence of ASD has been increasing at epidemic levels, and we think that the driving force behind this increase is a combination of genetic and environmental factors, emphasizing environmental determinants. We think that epigenetic factors related to lifestyle changes over the past two decades, especially reduction of early motor activity and spatial exploration of children, have led, in part, to the significant rise in the incidence of ASD. We surmise that the reduction in the activation of activity and experience-dependent genes that stimulate synaptogenesis and neuronal plasticity of central neurons and glial cells can help increase the size and complexity of the brain during the first 3 years of life. We think that this is the basis of both the maturational cortical delay identified in almost all neurobehavioral disorders, including ASD, and their associated RPRs (272–276). After the first few months of life, the feedback created by primitive reflex-generated movement can lead ultimately to the inhibition of these reflexes and the activation of more complex subsequent postural reflexes (64, 211, 277) resulting in a more complex interaction with the environment, that in turn leads to greater sensory feedback thereby activating genes that allow for the creation of integration and coordination between various cortical networks [for a more detailed analysis cf. (44)]. As these cortical networks become more connected and integrated, they increase the speed of their interaction, and their synchronization improves, allowing more areas to be activated simultaneously (24, 39, 278–282).
Delayed cortical maturity and motor coordination may occur due to the abnormal persistence of primitive reflexes. In that case, the brain will not continue to grow and develop at a normal rate and sequence. As the brain's hemispheres develop at different rates and times (283, 284), with the abnormal, asymmetric persistence of primitive reflexes, a maturational dysfunction in between hemisphere coherence can be produced where one hemisphere may mature at an average rate and the other not (231, 285–288). This can be associated with significant synchronization and temporal coherence dysfunction, decreasing large cortical networks between the two hemispheres from binding temporally and spatially. This can result in a functional disconnection syndrome (88, 102, 135, 137, 279, 289, 290).
We can conclude based on our current understanding that if there is any delay in maturation of the pyramidal tracts, the brainstem, or the frontal lobe, it is reasonable to assume that there might be a delay in the disappearance of the Babinski sign and other primitive reflexes. Many neurologists and pediatricians assume that the Babinski sign and other primitive reflexes that are present at birth and sustained for the first year of life will automatically disappear after that initial period unless brain damage is present (25, 27, 40, 94, 95, 233, 291).
Therefore, primitive reflexes are the tools that can be employed early in neonatal and infant development to evaluate the integrity of the central nervous system. They are brainstem-mediated, automatic movement patterns present in full-term infants at birth. With the maturation of the central nervous system, these primitive reflexes become more challenging to evoke after the first year postpartum when the infant becomes capable of voluntary motor activity. RPRs are not infrequently present in children with ASD, ADHD, or cerebral palsy, and may be early indicators of brain-based deficits (3, 4, 22, 148, 292–295).
Retained primitive reflexes may occur in the absence of any injury. They could be used as a clinical sign of a maturational delay in cortical development that is thought by many to be highly associated with abnormal functional connectivity seen in many neurobehavioral disorders such as ASD. We have also demonstrated that the Babinski sign may reappear later in life is grossly intact in older individuals without any physical damage but is highly related to cognitive decline. This may be a functional degeneration or loss of frontal lobe function, and we can observe this with “frontal release signs.” Therefore, the presence of the Babinski's sign in a healthy aged population may be a “returned” reflex which may be an early clinical sign of frontal lobe dysfunction or degeneration, or it may represent that the Babinski reflex has been present in an individual throughout his or her lifetime. Even though they may be grossly healthy, these individuals may have struggled with neurobehavioral symptoms. This maturation process commences from the bottom of the brainstem and the primitive reflexes. They help promote bottom-up development, which then promotes growth and maturation of the brain and frontal lobes, which then, in top-down feedback, regulates the brainstem nuclei, coherent with Huglings Jackson, the Principles of Dissolution.
In conclusion, we hypothesize that RPRs in ASD are, in part, associated with maturational delays and imbalances and not necessarily a result of actual structural damage or pathology. They are, in part, a result of environmental influences and are therefore amenable to remediation. We think that the presence of RPRs and the developmental milestones that might be delayed or absent as a result may be the earliest markers of developmentally delayed children, in general, and those with ASDs in particular. Assessing RPRs in ASD then is essential for multiple reasons. Firstly, RPRs may be a possible biomarker for ASD that, jointly with early signs of attentional deficit, eye contact, and other factors, might aid in characterizing the developmental trajectory of the character of ASD in infancy. In line with this approach, it has been stated that slight disparities in motor behavior in early development (i.e., RPRs) might exert an adverse cascading effect on the subsequent development of motor skills and also in numerous other domains (i.e., communication and social behavior and /or object exploration). Secondly, the detection of early motor abnormalities could also be an encouraging avenue for the delineation of subtypes of ASD.
GL, RM, and CM: conceptualization and methodology. SK: software, visualization, validation, and investigation. GL, RM, CM, YM-F, MC-A, and TM: writing—original draft preparation. GL and EC: supervision. GL, RM, CM, YM-F, MC-A, TM, and EC: writing—reviewing and editing. All authors contributed to the article and approved the submitted version.
This work was funded by a grant from the National Institute for Brain and Rehabilitation Sciences to GL and EC.
The authors declare that the research was conducted in the absence of any commercial or financial relationships that could be construed as a potential conflict of interest.
All claims expressed in this article are solely those of the authors and do not necessarily represent those of their affiliated organizations, or those of the publisher, the editors and the reviewers. Any product that may be evaluated in this article, or claim that may be made by its manufacturer, is not guaranteed or endorsed by the publisher.
1. Buckley A. bservations concerning primitive reflexes as revealed in reactions in normal mental states. Brain. (1927) 50:573–600. doi: 10.1093/brain/50.3-4.573
2. Borsani E, Della Vedova AM, Rezzani R, Rodella LF, Cristini C. Correlation between human nervous system development and acquisition of fetal skills: an overview. Brain Dev. (2019) 41:225–33. doi: 10.1016/j.braindev.2018.10.009
4. Pecuch A, Gieysztor E, Wolanska E, Telenga M, Paprocka-Borowicz M. Primitive reflex activity in relation to motor skills in healthy preschool children. Brain Sci. (2021) 11:967. doi: 10.3390/brainsci11080967
5. Ding W, Liu R, Shan R. Effect of gangliosides combined with mouse NGF on the expression of serum HIF-1alpha, NSE, and sICAM-1 levels in neonates with HIE. Am J Transl Res. (2021) 13:10570–7.
6. Futagi Y, Toribe Y, Suzuki Y. The grasp reflex and moro reflex in infants: hierarchy of primitive reflex responses. Int J Pediatr. (2012) 2012:191562. doi: 10.1155/2012/191562
8. Saraga M, Resic B, Krnic D, Jelavic T, Krnic D, Sinovcic I, et al. A stereotypic “elbowing” movement, a possible new primitive reflex in newborns. Pediatr Neurol. (2007) 36:84–7. doi: 10.1016/j.pediatrneurol.2006.09.013
9. Schafer CB, Hoebeek FE. Convergence of primary sensory cortex and cerebellar nuclei pathways in the whisker system. Neuroscience. (2018) 368:229–39. doi: 10.1016/j.neuroscience.2017.07.036
10. Zheng Y, Horii A, Appleton I, Darlington CL, Smith PF. Damage to the vestibular inner ear causes long-term changes in neuronal nitric oxide synthase expression in the rat hippocampus. Neuroscience. (2001) 105:1–5. doi: 10.1016/S0306-4522(01)00217-2
11. Martin JG, Cox PH, Scholl CA, Riesenhuber M. A crash in visual processing: Interference between feedforward and feedback of successive targets limits detection and categorization. J Vis. (2019) 19:20. doi: 10.1167/19.12.20
12. Proulx MJ. Bottom-up guidance in visual search for conjunctions. J Exp Psychol Hum Percept Perform. (2007) 33:48–56. doi: 10.1037/0096-1523.33.1.48
13. Worhunsky PD, Stevens MC, Carroll KM, Rounsaville BJ, Calhoun VD, Pearlson GD, et al. Functional brain networks associated with cognitive control, cocaine dependence, and treatment outcome. Psychol Addict Behav. (2013) 27:477–88. doi: 10.1037/a0029092
14. Babinski J. Atrophie Musculaire D'origine Cérébrale, Avec Intégrité des Cornes Antérieures de la Moelle et Des Nerfs Moteurs, Vol. 8è Série, III. Paris: Comptes-rendus hebdomadaires des Séances et Mémoires de la Société de Biologie (1896).
17. Behere RV. Dorsolateral prefrontal lobe volume and neurological soft signs as predictors of clinical social and functional outcome in schizophrenia: a longitudinal study. Indian J Psychiatry. (2013) 55:111–6. doi: 10.4103/0019-5545.111445
18. Burns A, Jacoby R, Levy R. Neurological signs in Alzheimer's disease. Age Ageing. (1991) 20:45–51. doi: 10.1093/ageing/20.1.45
19. Gabelle A, Gutierrez LA, Dartigues JF, Ritchie K, Touchon J, Berr C. Palmomental reflex a relevant sign in early Alzheimer's disease diagnosis? J Alzheimers Dis. (2016) 49:1135–41. doi: 10.3233/JAD-150436
20. Girling DM, Berrios GE. Extrapyramidal signs, primitive reflexes and frontal lobe function in senile dementia of the Alzheimer type. Br J Psychiatry. (1990) 157:888–93. doi: 10.1192/bjp.157.6.888
21. Gieysztor EZ, Choinska AM, Paprocka-Borowicz M. Persistence of primitive reflexes and associated motor problems in healthy preschool children. Arch Med Sci. (2018) 14:167–73. doi: 10.5114/aoms.2016.60503
22. Matuszkiewicz M, Galkowski T. Developmental language disorder and uninhibited primitive reflexes in young children. J Speech Lang Hear Res. (2021) 64:935–48. doi: 10.1044/2020_JSLHR-19-00423
24. Allen MC, Capute AJ. Neonatal neurodevelopmental examination as a predictor of neuromotor outcome in premature infants. Pediatrics. (1989) 83:498–506. doi: 10.1542/peds.83.4.498
25. Melillo R, Leisman G, Mualem R, Ornai A, Carmeli E. Persistent childhood primitive reflex reduction effects on cognitive, sensorimotor, and academic performance in ADHD. Front Public Health. (2020) 8:431835. doi: 10.3389/fpubh.2020.431835
26. Chandradasa M, Rathnayake L. Retained primitive reflexes in children, clinical implications and targeted home-based interventions. Nurs Child Young People. (2020) 32:37–42. doi: 10.7748/ncyp.2019.e1132
27. Leisman G, Braun-Benjamin O, Melillo R. Cognitive-motor interactions of the basal ganglia in development. Front Syst Neurosci. (2014) 8:16. doi: 10.3389/fnsys.2014.00016
28. Nagai Y, Nomura K, Uemura O. Primitive reflexes in very low birth weight infants later diagnosed with autism spectrum disorder. Minerva Pediatr. (2020). doi: 10.23736/S0026-4946.20.05784-9. [Epub ahead of print].
29. Black KJ, Kim S, Schlaggar BL, Greene DJ. The new tics study: a novel approach to pathophysiology and cause of tic disorders. J Psychiatr Brain Sci. (2020) 5:e200012. doi: 10.20900/jpbs.20200012
30. Thiede A, Parkkonen L, Virtala P, Laasonen M, Makela JP, Kujala T. Neuromagnetic speech discrimination responses are associated with reading-related skills in dyslexic and typical readers. Heliyon. (2020) 6:e04619. doi: 10.1016/j.heliyon.2020.e04619
31. Kapogiannis BG, Chakhtoura N, Hazra R, Spong CY. Bridging knowledge gaps to understand how Zika virus exposure and infection affect child development. JAMA Pediatr. (2017) 171:478–85. doi: 10.1001/jamapediatrics.2017.0002
32. Morgan AM, Koch V, Lee V, Aldag J. Neonatal neurobehavioral examination. A new instrument for quantitative analysis of neonatal neurological status. Phys Ther. (1988) 68:1352–8. doi: 10.1093/ptj/68.9.1352
33. Morrison DC, Hinshaw SP, Carte ET. Signs of neurobehavioral dysfunction in a sample of learning disabled children: stability and concurrent validity. Percept Mot Skills. (1985) 61(3 Pt 1):863–72. doi: 10.2466/pms.1985.61.3.863
34. Tsai JD, Ho MC, Lee HY, Shen CY, Li JY, Weng JC. Disrupted white matter connectivity and organization of brain structural connectomes in tuberous sclerosis complex patients with neuropsychiatric disorders using diffusion tensor imaging. MAGMA. (2020) 34:189–200. doi: 10.1007/s10334-020-00870-4
35. Bakchine S, Lacomblez L, Palisson E, Laurent M, Derouesne C. Relationship between primitive reflexes, extra-pyramidal signs, reflective apraxia and severity of cognitive impairment in dementia of the Alzheimer type. Acta Neurol Scand. (1989) 79:38–46. doi: 10.1111/j.1600-0404.1989.tb03707.x
36. Bartlett D. Primitive reflexes and early motor development. J Dev Behav Pediatr. (1997) 18:151–7. doi: 10.1097/00004703-199706000-00002
37. Cleal M, Fontana BD, Ranson DC, McBride SD, Swinny JD, Redhead ES, et al. The Free-movement pattern Y-maze: a cross-species measure of working memory and executive function. Behav Res Methods. (2021) 53:536–57. doi: 10.3758/s13428-020-01452-x
38. Gollnitz G. [Development of motoricity as functional-neurologic diagnosis]. Psychiatr Neurol Med Psychol Beih. (1970) 13–4:49–61.
39. Warren JLA, De León MSP, Hopkins WD, Zollikofer CP. Evidence for independent brain and neurocranial reorganization during hominin evolution. Proc Natl Acad Sci USA. (2019) 116:22115–21. doi: 10.1073/pnas.1905071116
40. Israely S, Leisman G. Can neuromodulation techniques optimally exploit cerebello-thalamo-cortical circuit properties to enhance motor learning post-stroke? Rev Neurosci. (2019) 30:821–37. doi: 10.1515/revneuro-2019-0008
41. Falk D, Zollikofer CP, Morimoto N, Ponce de Leon MS. Metopic suture of Taung (Australopithecus africanus) and its implications for hominin brain evolution. Proc Natl Acad Sci USA. (2012) 109:8467–70. doi: 10.1073/pnas.1119752109
42. Ishikawa T, Furuyama M, Ishikawa M, Ogawa J, Wada Y. Growth and achievement of large- and small-headed children in a normal population. Brain Dev. (1988) 10:295–9. doi: 10.1016/S0387-7604(88)80059-7
43. Leisman G, Melillo R. Effects of motor sequence training on attentional performance in ADHD children. Int J Disabil Hum Dev. (2010) 9:275–82. doi: 10.1515/IJDHD.2010.043
44. Melillo R, Leisman G. Neurobehavioral Disorders of Childhood: An Evolutionary Perspective. New York, NY: Springer (2009). doi: 10.1007/978-1-4419-1231-2
45. Needlman R, Silverstein M. Pediatric interventions to support reading aloud: how good is the evidence? J Dev Behav Pediatr. (2004) 25:352–63. doi: 10.1097/00004703-200410000-00007
46. Kuiper MJ, Brandsma R, Lunsing RJ, Eggink H, Ter Horst HJ, Bos AF, et al. The neurological phenotype of developmental motor patterns during early childhood. Brain Behav. (2019) 9:e01153. doi: 10.1002/brb3.1153
47. Fields RD, Stevens-Graham B. New insights into neuron-glia communication. Science. (2002) 298:556–62. doi: 10.1126/science.298.5593.556
48. Mill RD, Winfield EC, Cole MW, Ray S. Structural MRI and functional connectivity features predict current clinical status and persistence behavior in prescription opioid users. NeuroImage. (2021) 30:102663. doi: 10.1016/j.nicl.2021.102663
49. Biswas P, Malhotra S, Malhotra A, Gupta N. Comparative study of neurological soft signs in schizophrenia with onset in childhood, adolescence and adulthood. Acta Psychiatr Scand. (2007) 115:295–303. doi: 10.1111/j.1600-0447.2006.00901.x
50. Black DN, Stip E, Bedard M, Kabay M, Paquette I, Bigras MJ. Leukotomy revisited: late cognitive and behavioral effects in chronic institutionalized schizophrenics. Schizophr Res. (2000) 43:57–64. doi: 10.1016/S0920-9964(99)00176-0
51. Cordato NJ, Halliday GM, Caine D, Morris JG. Comparison of motor, cognitive, and behavioral features in progressive supranuclear palsy and Parkinson's disease. Mov Disord. (2006) 21:632–8. doi: 10.1002/mds.20779
52. Chen B. A preliminary study of atypical cortical change ability of dynamic whole-brain functional connectivity in autism spectrum disorder. Int J Neurosci. (2020) 132:213–25. doi: 10.1080/00207454.2020.1806837
53. Frongia I, Rizzi S, Baga M, Ceteroni LM, Spagnoli C, Salerno GG, et al. Infantile-onset charcot-marie-tooth disease with pyramidal features and white matter abnormalities due to a de novo MORC2 gene variant: a case report and brief review of the literature. Front Neurol. (2021) 12:718808. doi: 10.3389/fneur.2021.718808
54. Funayama M, Takata T. Forced gazing: a stimulus-bound behavior. Cogn Behav Neurol. (2021) 34:140–9. doi: 10.1097/WNN.0000000000000259
55. Huang Y, Zhang B, Cao J, Yu S, Wilson G, Park J, et al. Potential locations for noninvasive brain stimulation in treating autism spectrum disorders-a functional connectivity study. Front Psychiatry. (2020) 11:388. doi: 10.3389/fpsyt.2020.00388
57. Purves D, Augustine GJ, Fitzpatrick D. Damage to Descending Motor Pathways: The Upper Motor Neuron Syndrome. Sunderland, MA: Sinauer Associates (2001).
58. Gabis LV, Attia OL, Roth-Hanania R, Foss-Feig J. Motor delay-An early and more common “red flag” in girls rather than boys with autism spectrum disorder. Res Dev Disabil. (2020) 104:103. doi: 10.1016/j.ridd.2020.103702
59. Fisher WW, Luczynski KC, Blowers AP, Vosters ME, Pisman MD, Craig AR, et al. A randomized clinical trial of a virtual-training program for teaching applied-behavior-analysis skills to parents of children with autism spectrum disorder. J Appl Behav Anal. (2020) 53:1856–75. doi: 10.1002/jaba.778
60. Lima JL, Axt G, Teixeira DS, Monteiro D, Cid L, Yamamoto T, et al. Exergames for children and adolescents with autism spectrum disorder: an overview. Clin Pract Epidemiol Ment Health. (2020) 16:1–6. doi: 10.2174/1745017902016010001
61. Bartolotti J, Sweeney JA, Mosconi MW. Functional brain abnormalities associated with comorbid anxiety in autism spectrum disorder. Dev Psychopathol. (2020) 32:1273–86. doi: 10.1017/S0954579420000772
62. Li L, He C, Jian T, Guo X, Xiao J, Li Y, et al. Attenuated link between the medial prefrontal cortex and the amygdala in children with autism spectrum disorder: Evidence from effective connectivity within the “social brain”. Prog Neuropsychopharmacol Biol Psychiatry. (2020) 110147. doi: 10.1016/j.pnpbp.2020.110147
63. Modabbernia A, Janiri D, Doucet GE, Reichenberg A, Frangou S. Multivariate patterns of brain-behavior-environment associations in the adolescent brain and cognitive development study. Biol Psychiatry. (2020) 87:S147. doi: 10.1016/j.biopsych.2020.02.391
64. Sigafoos J, Roche L, O'Reilly MF, Lancioni GE. Persistence of primitive reflexes in developmental disorders. Curr Develop Disord Rep. (2021) 8:98–105. doi: 10.1007/s40474-021-00232-2
65. Smyser CD, Snyder A, Neil JJ. Functional connectivity MRI in infants: exploration of the functional organization of the developing brain. Neuroimage. (2011) 56:1437–52. doi: 10.1016/j.neuroimage.2011.02.073
66. Carvalho A, Brites C, Mochida G, Ventura P, Fernandes A, Lage ML, et al. Clinical and neurodevelopmental features in children with cerebral palsy and probable congenital Zika. Brain Dev. (2019) 41:587–94. doi: 10.1016/j.braindev.2019.03.005
67. Garfinkle J, Li P, Boychuck Z, Bussieres A, Majnemer A. Early clinical features of cerebral palsy in children without perinatal risk factors: a scoping review. Pediatr Neurol. (2020) 102:56–61. doi: 10.1016/j.pediatrneurol.2019.07.006
68. Damasceno A, Delicio AM, Mazo DFC, Zullo JFD, Scherer P, Ng TY, et al. Primitive reflexes and cognitive function. Arq Neuropsiquiatr. (2005) 63:577–82. doi: 10.1590/S0004-282X2005000400004
69. Berne SA. The primitive reflexes: considerations in the infant. Optomet Vis Dev. (2006) 37:139–45.
70. Feldhacker D, Cosgrove R, Feiten B, Schmidt K, Stewart M. Relationship between retained primitive reflexes and scholastic performance. Am J Occup Ther. 75:7512505161–4. doi: 10.5014/ajot.2021.75S2-RP164
71. Konicarova J, Bob P. Retained primitive reflexes and ADHD in children. Act Nerv Super. (2012) 54:134–7. doi: 10.1007/BF03379591
72. Konicarova J, Bob P, Raboch J. Persisting primitive reflexes in medication-naive girls with attention-deficit and hyperactivity disorder. Neuropsychiatr Dis Treat. (2013) 9:1457–61. doi: 10.2147/NDT.S49343
73. Stewart M. The correlation between retained primitive reflexes and scholastic performance among early elementary students. J Occup Ther Schools Early Interven. (2021) 1–14. doi: 10.1080/19411243.2021.1959482
74. Camarda C, Camarda R, Pipia C, Azzarello D, Grassedonio E, Sottile G, et al. Isolated, subtle neurological abnormalities in mild cognitive impairment types. Can J Neurol Sci. (2020) 47:77–91. doi: 10.1017/cjn.2019.293
75. Dopper EG, Seelaar H, Chiu WZ, de Koning I, van Minkelen R, Baker MC, et al. Symmetrical corticobasal syndrome caused by a novel C.314dup progranulin mutation. J Mol Neurosci. (2011) 45:354–8. doi: 10.1007/s12031-011-9626-z
76. Franco JG, Trzepacz PT, Velasquez-Tirado JD, Ocampo MV, Serna PA, Giraldo AM, et al. Discriminant performance of dysexecutive and frontal release signs for delirium in patients with high dementia prevalence: implications for neural network impairment. J Acad Consult Liaison Psychiatry. (2021) 62:56–69. doi: 10.1016/j.psym.2020.04.002
77. Kobayashi S, Maki T, Kunimoto M. Clinical symptoms of bilateral anterior cerebral artery territory infarction. J Clin Neurosci. (2011) 18:218–22. doi: 10.1016/j.jocn.2010.05.013
79. Shoenberg M, Scott J. The Little Black Book of Neuropsychology: A Syndrome-Bases Approach. Nova Iorque: Springer (2011).
80. Tarawneh R, Galvin JE. Neurologic signs in the elderly. In: Fillit HM, Rockwood K, Young JB, editors. Brocklehurst's Textbook of Geriatric Medicine and Gerontology. Elsevier Ltd. (2010). p. 101–5. doi: 10.1016/B978-1-4160-6231-8.10016-9
81. Khadilkar SV, Chheda AH. A systematic and quantitative evaluation of plantar stimulation: the effect of type, pattern, force of stimulation in eliciting an accurate plantar response. Clin Neurol Neurosurg. (2018) 168:83–90. doi: 10.1016/j.clineuro.2018.03.002
82. Diaz J, Abiola S, Kim N, Avaritt O, Flock D, Yu J, et al. Therapeutic hypothermia provides variable protection against behavioral deficits after neonatal hypoxia-ischemia: a potential role for brain-derived neurotrophic factor. Dev Neurosci. (2017) 39:257–72. doi: 10.1159/000454949
83. Grigg TM, Fox-Turnbull W, Culpan I. Retained primitive reflexes: perceptions of parents who have used Rhythmic Movement Training with their children. J Child Health Care. (2018) 22:406–18. doi: 10.1177/1367493518760736
84. Chrzan R, Gleń A, Bryll A, Urbanik A. computed tomography assessment of brain atrophy in centenarians. Int J Environ Res Public Health. (2019) 16:3659. doi: 10.3390/ijerph16193659
85. Gellis ZD, McClive-Reed KP, Brown E. Treatments for depression in older persons with dementia. Ann Longterm Care. (2009) 17:29–36.
86. Manto M. Cerebellar motor syndrome from children to the elderly. Handb Clin Neurol. (2028) 154:151–66. doi: 10.1016/B978-0-444-63956-1.00009-6
87. Bowyer SM, Zillgitt A, Greenwald M, Lajiness-O'Neill R. Language mapping with magnetoencephalography: an update on the current state of clinical research and practice with considerations for clinical practice guidelines. J Clin Neurophysiol. (2020) 37:554–63. doi: 10.1097/WNP.0000000000000489
88. Coburn KL, Williams DL. Development of neural structure and function in autism spectrum disorder: potential implications for learning language. Am J Speech Lang Pathol. (2020) 29:1–15. doi: 10.1044/2020_AJSLP-19-00209
89. Neudorf J, Kress S, Gould L, Gibb K, Mickleborough M, Borowsky R. Language lateralization differences between left and right temporal lobe epilepsy as measured by overt word reading fMRI activation and DTI structural connectivity. Epilepsy Behav. (2020) 112:107467. doi: 10.1016/j.yebeh.2020.107467
90. Shvarts V, Makela JP. Auditory mapping with MEG: an update on the current state of clinical research and practice with considerations for clinical practice guidelines. J Clin Neurophysiol. (2020) 37:574–84. doi: 10.1097/WNP.0000000000000518
91. Spiezio C, Sandri C, Joubert F, Muzungaile MM, Remy S, Mattarelli P, et al. The “right” side of sleeping: laterality in resting behaviour of Aldabra giant tortoises (Aldabrachelys gigantea). Anim Cogn. (2021) 25:195–203. doi: 10.1007/s10071-021-01542-z
92. Timocin G, Toprak A, Aralasmak A. Relationships of language lateralization with diffusion tensor imaging metrics of corpus callosum, tumor grade, and tumors distance to language-eloquent areas in glial neoplasms. J Comput Assist Tomogr. (2020) 44:956–68. doi: 10.1097/RCT.0000000000001103
93. Trebuchon A, Liegeois-Chauvel C, Gonzalez-Martinez JA, Alario FX. Contributions of electrophysiology for identifying cortical language systems in patients with epilepsy. Epilepsy Behav. (2020) 112:107407. doi: 10.1016/j.yebeh.2020.107407
94. Machado C, Estevez M, Leisman G, Melillo R, Rodriguez R, DeFina P, et al. QEEG spectral and coherence assessment of autistic children in three different experimental conditions. J Autism Dev Disord. (2015) 45:406–24. doi: 10.1007/s10803-013-1909-5
95. Machado C, Rodriguez R, Estevez M, Leisman G, Melillo R, Chinchilla M, et al. Anatomic and functional connectivity relationship in autistic children during three different experimental conditions. Brain Connect. (2015) 5:487–96. doi: 10.1089/brain.2014.0335
96. Galaburda AM, Rosen GD, Sherman GF. Individual variability in cortical organization: Its relationship to brain laterality and implications to function. Neuropsychologia. (1990) 28:529–46. doi: 10.1016/0028-3932(90)90032-J
97. Gilmore JH, Knickmeyer RC, Gao W. Imaging structural and functional brain development in early childhood. Nat Rev Neurosci. (2018) 19:123–37. doi: 10.1038/nrn.2018.1
98. Howell BR, Styner MA, Gao W, Yap PT, Wang L, Baluyot K, et al. The UNC/UMN Baby Connectome Project (BCP): an overview of the study design and protocol development. Neuroimage. (2019) 185:891–905. doi: 10.1016/j.neuroimage.2018.03.049
99. Machado CO, Griesi-Oliveira K, Rosenberg C, Kok F, Martins S, Passos-Bueno MR, et al. Collybistin binds and inhibits mTORC1 signaling: a potential novel mechanism contributing to intellectual disability and autism. Eur J Hum Genet. (2016) 24:59–65. doi: 10.1038/ejhg.2015.69
100. Zille P, Calhoun VD, Stephen JM, Wilson TW, Wang YP. Fused estimation of sparse connectivity patterns from rest fMRI-application to comparison of children and adult brains. IEEE Trans Med Imaging. (2018) 37:2165–75. doi: 10.1109/TMI.2017.2721640
101. Howells K, Sivaratnam C, Lindor E, He J, Hyde C, McGillivray J, et al. Can a community-based football program benefit motor ability in children with autism spectrum disorder? A pilot evaluation considering the role of social impairments. J Autism Dev Disord. (2021) 50:3714–27. doi: 10.1007/s10803-021-04933-w
102. Wang W, Han R, Zhang M, Wang Y, Wang T, Wang Y, et al. A network-based method for brain disease gene prediction by integrating brain connectome and molecular network. Brief Bioinform. (2021) 23:bbab459. doi: 10.1093/bib/bbab459
103. Lambert K, Eisch AJ, Galea LA, Kempermann G, Merzenich M. Optimizing brain performance: Identifying mechanisms of adaptive neurobiological plasticity. Neurosci Biobehav Rev. (2019) 105:60–71. doi: 10.1016/j.neubiorev.2019.06.033
104. Nebel MB, Eloyan A, Nettles CA, Sweeney KL, Ament K, Ward RE, et al. Intrinsic visual-motor synchrony correlates with social deficits in autism. Biol Psychiatry. (2016) 79:633–41. doi: 10.1016/j.biopsych.2015.08.029
105. Budisavljevic S, Castiello U, Begliomini C. Handedness and white matter networks. Neuroscientist. (2021) 27:88–103. doi: 10.1177/1073858420937657
106. Chang YN, Lambon Ralph MA. A unified neurocomputational bilateral model of spoken language production in healthy participants and recovery in poststroke aphasia. Proc Natl Acad Sci USA. (2020) 117:32779–90. doi: 10.1073/pnas.2010193117
107. Orekhova EV, Tsetlin MM, Butorina AV, Novikova SI, Gratchev VV, Sokolov PA, et al. Auditory cortex responses to clicks and sensory modulation difficulties in children with autism spectrum disorders (ASD). PLoS ONE. (2012) 7:e39906. doi: 10.1371/journal.pone.0039906
108. Zafeiriou DI. Primitive reflexes and postural reactions in the neurodevelopmental examination. Pediatr Neurol. (2004) 31:1–8. doi: 10.1016/j.pediatrneurol.2004.01.012
109. Longman D, Stock JT, Wells JCK. A trade-off between cognitive and physical performance, with relative preservation of brain function. Sci Rep. (2017) 7:13709. doi: 10.1038/s41598-017-14186-2
110. Hamer EG, Hadders-Algra M. Prognostic significance of neurological signs in high-risk infants - a systematic review. Dev Med Child Neurol. (2016) 58(Suppl. 4):53–60. doi: 10.1111/dmcn.13051
111. Futagi Y, Suzuki Y, Goto M. Clinical significance of plantar grasp response in infants. Pediatr Neurol. (1999) 20:111–5. doi: 10.1016/S0887-8994(98)00103-9
112. Futagi Y, Tagawa T, Otani K. Primitive reflex profiles in infants: differences based on categories of neurological abnormality. Brain Dev. (1992) 14:294–8. doi: 10.1016/S0387-7604(12)80146-X
113. Fiorentino RM. Normal and Abnormal Development: The Influence of Pri-Mitive Reflexes on Motor Development. Springfield IL: C Thomas Publisher (1980).
114. Zafeiriou DI. Plantar grasp reflex in high-risk infants during the first year of life. Pediatr Neurol. (2000) 22:75–6. doi: 10.1016/S0887-8994(99)00110-1
115. Bi X, Zhao J, Xu Q, Sun Q, Wang Z. Abnormal functional connectivity of resting state network detection based on linear ICA analysis in autism spectrum disorder. Front Physiol. (2018) 9:475. doi: 10.3389/fphys.2018.00475
116. Wei Y, Scholtens LH, Turk E, van den Heuvel MP. Multiscale examination of cytoarchitectonic similarity and human brain connectivity. Netw Neurosci. (2018) 3:124–37. doi: 10.1162/netn_a_00057
117. Russo N, Flanagan T, Iarocci G, Berringer D, Zelazo PD, Burack JA. Deconstructing executive deficits among persons with autism: implications for cognitive neuroscience. Brain Cogn. (2007) 65:77–86. doi: 10.1016/j.bandc.2006.04.007
118. Demertzi A, Soddu A, Laureys S. Consciousness supporting networks. Curr Opin Neurobiol. (2013) 23:239–44. doi: 10.1016/j.conb.2012.12.003
119. Fernández-Espejo D, Soddu A, Cruse D, Palacios EM, Junque C, Vanhaudenhuyse A, et al. A role for the default mode network in the bases of disorders of consciousness. Ann Neurol. (2012) 72:335–43. doi: 10.1002/ana.23635
120. Cirelli C, Tononi G. Is Sleep Essential? PLoS Biol. (2008) 6:e216. doi: 10.1371/journal.pbio.0060216
121. Leisman G, Ashkenazi M. Aetiological factors in dyslexia: IV. Cerebral hemispheres are functionally equivalent. Int J Neurosci. (1980) 11:157–64. doi: 10.3109/00207458009147581
122. Leisman G, Zenhausern R. Integratory systems deficits in developmental dyslexia. Neuropsychol Cogn. (1982) 2:479–507. doi: 10.1007/978-94-009-7654-2_44
123. Bastos AM, Vezoli J, Bosman CA, Schoffelen JM, Oostenveld R, Dowdall JR, et al. Visual areas exert feedforward and feedback influences through distinct frequency channels. Neuron. (2015) 85:390–401. doi: 10.1016/j.neuron.2014.12.018
124. Hilger K, Ekman M, Fiebach CJ, Basten U. Intelligence is associated with the modular structure of intrinsic brain networks. Sci Rep. (2017) 7:16088. doi: 10.1038/s41598-017-15795-7
125. de Pasquale F, Della Penna S, Snyder AZ, Marzetti L, Pizzella V, Romani GL, et al. A cortical core for dynamic integration of functional networks in the resting human brain. Neuron. (2012) 74:753–64. doi: 10.1016/j.neuron.2012.03.031
126. Peters DH, Adam T, Alonge O, Agyepong IA, Tran N. Implementation research: what it is and how to do it. BMJ. (2013) 347:731–6. doi: 10.1136/bmj.f6753
127. Schultz DH, Cole MW. Higher intelligence is associated with less task-related brain network reconfiguration. JNeurosci. (2016) 36:8551–61. doi: 10.1523/JNEUROSCI.0358-16.2016
128. Young CJ, Levine SC, Mix KS. The connection between spatial and mathematical ability across development. Front Psychol. (2018) 9:755. doi: 10.3389/fpsyg.2018.00755
129. Downey D, Brigadoi S, Trevithick L, Elliott R, Elwell C, McAllister-Williams RH, et al. Frontal haemodynamic responses in depression and the effect of electroconvulsive therapy. J Psychopharmacol. (2019) 33:1003–14. doi: 10.1177/0269881119858313
130. Jung RE, Gasparovic C, Chavez RS, Caprihan A, Barrow R, Yeo RA. Imaging intelligence with proton magnetic resonance spectroscopy. Intelligence. (2009) 37:192–8. doi: 10.1016/j.intell.2008.10.009
131. Zheng S, LeWinn K, Ceja T, Hanna-Attisha M, O'Connell L, Bishop S. Adaptive behavior as an alternative outcome to intelligence quotient in studies of children at risk: a study of preschool-aged children in Flint, MI, USA. Front Psychol. (2021) 12:3254. doi: 10.3389/fpsyg.2021.692330
132. Ito T, Kulkarni KR, Schultz DH, Mill RD, Chen RH, Solomyak LI, et al. Cognitive task information is transferred between brain regions via resting-state network topology. Nat Commun. (2017) 8:1027. doi: 10.1038/s41467-017-01000-w
133. Spronk M, Keane BP, Ito T, Kulkarni K, Ji JL, Anticevic A, et al. A whole-brain and cross-diagnostic perspective on functional brain network dysfunction. Cereb Cortex. (2020) 31:547–61. doi: 10.1093/cercor/bhaa242
134. Cifre I, Miller Flores MT, Penalba L, Ochab JK, Chialvo DR. Revisiting nonlinear functional brain co-activations: directed, dynamic, and delayed. Front Neurosci. (2021) 15:700171. doi: 10.3389/fnins.2021.700171
135. Li J, Wang F, Pan J, Wen Z. Identification of autism spectrum disorder with functional graph discriminative network. Front Neurosci. (2021) 15:729937. doi: 10.3389/fnins.2021.729937
136. Liu M, Li B, Hu D. Autism spectrum disorder studies using fMRI data and machine learning: a review. Front Neurosci. (2021) 15:697870. doi: 10.3389/fnins.2021.697870
137. Milovanovic M, Grujicic R. Electroencephalography in assessment of autism spectrum disorders: a review. Front Psychiatry. (2021) 12:686021. doi: 10.3389/fpsyt.2021.686021
138. Rakhimberdina Z, Liu X, Murata AT. Population graph-based multi-model ensemble method for diagnosing autism spectrum disorder. Sensors. (2020) 20:21. doi: 10.3390/s20216001
139. Wang N, Yao D, Ma L, Liu M. Multi-site clustering and nested feature extraction for identifying autism spectrum disorder with resting-state fMRI. Med Image Anal. (2021) 75:102279. doi: 10.1016/j.media.2021.102279
140. Roland JL, Snyder AZ, Hacker CD, Mitra A, Shimony JS, Limbrick DD, et al. On the role of the corpus callosum in interhemispheric functional connectivity in humans. Proc Natl Acad Sci USA. (2017) 114:13278–83. doi: 10.1073/pnas.1707050114
141. Nordahl CW, Iosif A-M, Young GS, Perry LM, Dougherty R, Lee A, et al. Sex differences in the corpus callosum in preschool-aged children with autism spectrum disorder. Mol Autism. (2015) 6:26–26. doi: 10.1186/s13229-015-0005-4
142. Treffert DA. The savant syndrome: an extraordinary condition. A synopsis: past, present, future. Philos Trans R Soc Lond Ser B Biol Sci. (2009) 364:1351–7. doi: 10.1098/rstb.2008.0326
143. Anderson GM. The potential role for emergence in autism. Autism Res. (2008) 1:18–30. doi: 10.1002/aur.2
144. Chinello A, Di Gangi V, Valenza E. Persistent primary reflexes affect motor acts:potential implications for autism spectrum disorder. Res Dev Disabil. (2018) 83:287–95. doi: 10.1016/j.ridd.2016.07.010
145. Khan NZ, Muslima H, Begum D, Shilpi AB, Akhter S, Bilkis K, et al. Validation of rapid neurodevelopmental assessment instrument for under-two-year-old children in Bangladesh. Pediatrics. (2010) 125:e755–62. doi: 10.1542/peds.2008-3471
146. Koshiba M, Karino G, Mimura K, Nakamura S, Yui K, Kunikata T, et al. Psycho-cognitive intervention for ASD from cross-species behavioral analyses of infants, chicks and common marmosets. CNS Neurol Disord Drug Targets. (2016) 15:578–86. doi: 10.2174/1871527315666160413121613
147. Monks DT, Palanisamy A. Oxytocin: at birth and beyond. A systematic review of the long-term effects of peripartum oxytocin. Anaesthesia. (2021) 76:1526–37. doi: 10.1111/anae.15553
148. Shaw T, Soto-Garcia M. Chiropractic management of toe-walking in an eight-year-old male diagnosed with autism spectrum disorder utilizing a functional approach: a case study. J Bodyw Mov Ther. (2021) 26:538–41. doi: 10.1016/j.jbmt.2021.03.014
149. Grigg I, Ivashko-Pachima Y, Hait TA, Korenková V, Touloumi O, Lagoudaki R, et al. Tauopathy in the young autistic brain: novel biomarker and therapeutic target. Transl Psychiatry. (2020) 10:228. doi: 10.1038/s41398-020-00904-4
150. Kogan MD, Vladutiu CJ, Schieve LA, Ghandour RM, Blumberg SJ, Zablotsky B, et al. The prevalence of parent-reported autism spectrum disorder among US children. Pediatrics. (2018) 142:e20174161. doi: 10.1542/peds.2017-4161
151. Dawson G, Campbell K, Hashemi J, Lippmann SJ, Smith V, Carpenter K, et al. Atypical postural control can be detected via computer vision analysis in toddlers with autism spectrum disorder. Sci Rep. (2018) 8:17008. doi: 10.1038/s41598-018-35215-8
152. Fournier KA, Hass CJ, Naik SK, Lodha N, Cauraugh JH. Motor coordination in autism spectrum disorders: a synthesis and meta-analysis. J Autism Dev Disord. (2010) 40:1227–40. doi: 10.1007/s10803-010-0981-3
153. Gieysztor E, Pecuch A, Kowal M, Borowicz W, Paprocka-Borowicz M. Pelvic symmetry is influenced by asymmetrical tonic neck reflex during young children's gait. Int J Environ Res Public Health. (2020) 17:4759. doi: 10.3390/ijerph17134759
154. Gieysztor E, Kowal M, Paprocka-Borowicz M. Primitive reflex factors influence walking gait in young children: an observational study. Int J Environ Res Public Health. (2022) 19:4070. doi: 10.3390/ijerph19074070
155. Mualem R, Leisman G, Zbedat Y, Ganem S, Mualem O, Amaria M, et al. The effect of movement on cognitive performance. Front Publ Health. (2018) 6:100. doi: 10.3389/fpubh.2018.00100
156. Setoh P, Marschik PB, Einspieler C, Esposito G. Autism spectrum disorder and early motor abnormalities: connected or coincidental companions? Res Dev Disabil. (2017) 60:13–5. doi: 10.1016/j.ridd.2016.11.001
157. Higashionna T, Iwanaga R, Tokunaga A, Nakai A, Tanaka K, Nakane H, et al. Relationship between motor coordination, cognitive abilities, and academic achievement in japanese children with neurodevelopmental disorders. Hong Kong J Occup Ther. (2017) 30:49–55. doi: 10.1016/j.hkjot.2017.10.002
158. Kentrou V, de Veld DMJ, Mataw KJK, Begeer S. Delayed autism spectrum disorder recognition in children and adolescents previously diagnosed with attention-deficit/hyperactivity disorder. Autism. (2018) 23:1065–72. doi: 10.1177/1362361318785171
159. Teitelbaum P, Teitelbaum OB, Fryman J, Maurer R. Reflexes gone astray in autism in infancy. J Dev Learn Disord. (2002) 6:15–22.
160. Teitelbaum O, Benton T, Shah PK, Prince A, Kelly JL, Teitelbaum P. Eshkol-Wachman movement notation in diagnosis: the early detection of Asperger's syndrome. Proc Natl Acad Sci USA. (2004) 101:11909–14. doi: 10.1073/pnas.0403919101
161. Teitelbaum P, Teitelbaum O, Nye J, Fryman J, Maurer RG. Movement analysis in infancy may be useful for early diagnosis of autism. Proc Natl Acad Sci USA. (1998) 95:13982–7. doi: 10.1073/pnas.95.23.13982
163. Konicarova J, Bob P. Asymmetric tonic neck reflex and symptoms of attention deficit and hyperactivity disorder in children. Int J Neurosci. (2013) 123:766–9. doi: 10.3109/00207454.2013.801471
164. Callcott DL. Retained primary reflexes in pre-primary-aged Indigenous children: the effect on movement ability and school readiness. Austr J Early Childhood. (2012) 37:132–40. doi: 10.1177/183693911203700218
166. Brinkman SA, Gregory TA, Goldfeld S, Lynch JW, Hardy M. Data resource profile: the Australian early development index (AEDI). Int J Epidemiol. (2014) 43:1089–96. doi: 10.1093/ije/dyu085
167. Williams J, Holley P. Linking motor development in infancy and early childhood to later school learning. Austr J Child Fam Health Nurs. (2013) 10:15–21.
168. de Marchena A, Eigsti IM. Conversational gestures in autism spectrum disorders: asynchrony but not decreased frequency. Autism Res. (2010) 3:311–22. doi: 10.1002/aur.159
169. Manicolo O, Grob A, Hagmann-von Arx P. Gait in children with attention-deficit hyperactivity disorder in a dual-task paradigm. Front Psychol. (2017) 8:34. doi: 10.3389/fpsyg.2017.00034
170. Neely KA, Mohanty S, Schmitt LM, Wang Z, Sweeney JA, Mosconi MW. Motor memory deficits contribute to motor impairments in autism spectrum disorder. J Autism Dev Disord. (2019) 49:2675–84. doi: 10.1007/s10803-016-2806-5
171. Donnellan AM, Hill DA, Leary MR. Rethinking autism: implications of sensory and movement differences for understanding and support. Front Integr Neurosci. (2013) 6:124. doi: 10.3389/fnint.2012.00124
172. Biotteau M, Albaret JM, Chaix Y. Developmental coordination disorder. Handb Clin Neurol. (2020) 174:3–20. doi: 10.1016/B978-0-444-64148-9.00001-6
173. Wilmut K, Du W, Barnett AL. Gait patterns in children with developmental coordination disorder. Exper Brain Res. (2016) 234:1747–55. doi: 10.1007/s00221-016-4592-x
174. Caçola P, Miller HL, Ossom Williamson P. Behavioral comparisons in autism spectrum disorder and developmental coordination disorder: a systematic literature review. Res Autism Spectr Disord. (2017) 38:6–18. doi: 10.1016/j.rasd.2017.03.004
175. Muskens JB, Velders FP, Staal WG. Medical comorbidities in children and adolescents with autism spectrum disorders and attention deficit hyperactivity disorders: a systematic review. Eur Child Adol Psychiat. (2017) 26:1093–103. doi: 10.1007/s00787-017-1020-0
176. Alm PA, Risberg J. Stuttering in adults: the acoustic startle response, temperamental traits, and biological factors. J Commun Disord. (2007) 40:1–41. doi: 10.1016/j.jcomdis.2006.04.001
177. Chen J, Niemeier M. Altered perceptual pseudoneglect in ADHD: evidence for a functional disconnection from early visual activation. Neuropsychologia. (2017) 99:12–23. doi: 10.1016/j.neuropsychologia.2017.02.022
178. Johnson P, Ahamat B, McConnachie A, Puckering C, Marwick H, Furnivall D, et al. Motor activity at age one year does not predict ADHD at seven years. Int J Methods Psychiatr Res. (2014) 23:9–18. doi: 10.1002/mpr.1436
179. Kim DJ, Min BK. Rich-club in the brain's macrostructure: Insights from graph theoretical analysis. Comput Struct Biotechnol J. (2020) 18:1761–73. doi: 10.1016/j.csbj.2020.06.039
180. King JA, Colla M, Brass M, Heuser I, von Cramon D. Inefficient cognitive control in adult ADHD: evidence from trial-by-trial Stroop test and cued task switching performance. Behav Brain Funct. (2007) 3:42. doi: 10.1186/1744-9081-3-42
181. Dawes P, Bishop DV. Maturation of visual and auditory temporal processing in school-aged children. J Speech Lang Hear Res. (2008) 51:1002–15. doi: 10.1044/1092-4388(2008/073)
182. Gavaret M, Guedj E, Koessler L, Trebuchon-Da Fonseca A, Aubert S, Mundler O, et al. Reading epilepsy from the dominant temporo-occipital region. J Neurol Neurosurg Psychiatry. (2010) 81:710–5. doi: 10.1136/jnnp.2009.175935
183. Lallier M, Donnadieu S, Valdois S. Visual attentional blink in dyslexic children: Parameterizing the deficit. Vision Res. (2010) 50:1855–61. doi: 10.1016/j.visres.2010.06.006
184. Legrand A, Bui-Quoc E, Dore-Mazars K, Lemoine C, Gerard CL, Bucci MP. Effect of a dual task on postural control in dyslexic children. PLoS ONE. (2012) 7:e35301. doi: 10.1371/journal.pone.0035301
185. Maillard L, Vignal JP, Raffo E, Vespignani H. Bitemporal form of partial reading epilepsy: further evidence for an idiopathic localization-related syndrome. Epilepsia. (2010) 51:165–9. doi: 10.1111/j.1528-1167.2009.02247.x
186. Stevenson RA, Wallace MT. Multisensory temporal integration: task and stimulus dependencies. Exp Brain Res. (2013) 227:249–61. doi: 10.1007/s00221-013-3507-3
187. Sumner E, Leonard HC, Hill EL. Overlapping phenotypes in autism spectrum disorder and developmental coordination disorder: a cross-syndrome comparison of motor and social skills. J Autism Dev Disord. (2016) 46:2609–20. doi: 10.1007/s10803-016-2794-5
188. Charman T, Pickles A, Simonoff E, Chandler S, Loucas T, Baird G. IQ in children with autism spectrum disorders: data from the Special Needs and Autism Project (SNAP). Psychol Med. (2011) 41:619–27. doi: 10.1017/S0033291710000991
189. Gharebaghy S, Rassafiani M, Cameron D. Effect of cognitive intervention on children with ADHD. Phys Occup Ther Pediatr. (2015) 35:13–23. doi: 10.3109/01942638.2014.957428
190. Floris DL, Barber AD, Nebel MB, Martinelli M, Lai M-C, Crocetti D, et al. Atypical lateralization of motor circuit functional connectivity in children with autism is associated with motor deficits. Mol Autism. (2016) 7:35–35. doi: 10.1186/s13229-016-0096-6
191. Lidstone DE, Miah FZ, Poston B, Beasley JF, Dufek JS. Manual dexterity in children with autism spectrum disorder: a cross-syndrome approach. Res Autism Spect Disord. (2020) 73:101546. doi: 10.1016/j.rasd.2020.101546
192. Craig F, Crippa A, Ruggiero M, Rizzato V, Russo L, Fanizza I, et al. Characterization of Autism Spectrum Disorder (ASD) subtypes based on the relationship between motor skills and social communication abilities. Hum Mov Sci. (2021) 77:102802. doi: 10.1016/j.humov.2021.102802
193. Ohara R, Kanejima Y, Kitamura M, Izawa KP. Association between social skills and motor skills in individuals with autism spectrum disorder: a systematic review. Eur J Invest Health Psychol Educ. (2019) 10:276–96. doi: 10.3390/ejihpe10010022
194. Ramos-Sanchez CP, Kortekaas D, Van Biesen D, Vancampfort D, Van Damme T. The relationship between motor skills and intelligence in children with autism spectrum disorder. J Autism Dev Disord. (2021) 52:1189–99. doi: 10.1007/s10803-021-05022-8
195. Sheedy AJ, Brent J, Dally K, Ray K, Lane AE. Handwriting readiness among digital native kindergarten students. Phys Occup Ther Pediatr. (2021) 41:655–69. doi: 10.1080/01942638.2021.1912247
196. Thomas S, Barnett LM, Papadopoulos N, Lander N, McGillivray J, Rinehart N. How do physical activity and sedentary behaviour affect motor competence in children with autism spectrum disorder compared to typically developing children: a pilot study. J Autism Dev Disord. (2021) 1–13. doi: 10.1007/s10803-021-05205-3
197. Labache L, Mazoyer B, Joliot M, Crivello F, Hesling I, Tzourio-Mazoyer N. Typical and atypical language brain organization based on intrinsic connectivity and multitask functional asymmetries. Elife. (2020) 9:e58722. doi: 10.7554/eLife.58722
198. Tzourio-Mazoyer N. Intra- and Inter-hemispheric connectivity supporting hemispheric specialization. In: Kennedy H, Van Essen DC, Christen Y, editors. Micro-, Meso- and Macro-Connectomics of the Brain. Cham (2016). p. 129–46. doi: 10.1007/978-3-319-27777-6_9
199. Tzourio-Mazoyer N, Zago L, Cochet H, Crivello F. Development of handedness, anatomical and functional brain lateralization. Handb Clin Neurol. (2020) 173:99–105. doi: 10.1016/B978-0-444-64150-2.00011-3
200. Yoon SH, Choi J, Lee WJ, Do JT. Genetic and epigenetic etiology underlying autism spectrum disorder. J Clin Med. (2020) 9:966. doi: 10.3390/jcm9040966
201. Bhaumik S, Tyrer F, Barrett M, Tin N, McGrother CW, Kiani R. The relationship between carers' report of autistic traits and clinical diagnoses of autism spectrum disorders in adults with intellectual disability. Res Dev Disabil. (2010) 31:705–12. doi: 10.1016/j.ridd.2010.01.012
202. Pham A, Kasenic A, Hayden L, Inwards-Breland DJ, Sumerwell C, Twible H, et al. A case series on disordered eating among transgender youth with autism spectrum disorder. J Adolesc Health. (2021) 68:1215–9. doi: 10.1016/j.jadohealth.2020.12.143
203. Shen Y, Li Y, Shi L, Liu M, Wu R, Xia K, et al. Autism spectrum disorder and severe social impairment associated with elevated plasma interleukin-8. Pediatr Res. (2021) 89:591–7. doi: 10.1038/s41390-020-0910-x
204. Tuchman RF. Deconstructing autism spectrum disorders: clinical perspective. Rev Neurol. (2013) 56(Suppl. 1):S3–12.
205. Freitag CM. [Autism Spectrum Disorder in DSM-5 - concept, validity, and reliability, impact on clinical care and future research]. Z Kinder Jugendpsychiatr Psychother. (2014) 42:185–92. doi: 10.1024/1422-4917/a000288
207. Joon P, Kumar A, Parle M. What is autism? Pharmacol Rep. (2021) 73:1255–64. doi: 10.1007/s43440-021-00244-0
208. Kim YS, Fombonne E, Koh YJ, Kim SJ, Cheon KA, Leventhal BL. A comparison of DSM-IV pervasive developmental disorder and DSM-5 autism spectrum disorder prevalence in an epidemiologic sample. J Am Acad Child Adolesc Psychiatry. (2014) 53:500–8. doi: 10.1016/j.jaac.2013.12.021
209. Smith IC, Reichow B, Volkmar FR. The effects of DSM-5 criteria on number of individuals diagnosed with autism spectrum disorder: a systematic review. J Autism Dev Disord. (2015) 45:2541–52. doi: 10.1007/s10803-015-2423-8
210. Gieysztor EZ, Sadowska L, Choinska AM, Paprocka-Borowicz M. Trunk rotation due to persistence of primitive reflexes in early school-age children. Adv Clin Exp Med. (2018) 27:363–6. doi: 10.17219/acem/67458
212. Marinov E, Svetkova S, Staneva K. Primitive reflexes of children with developmental coordination disorder. Yale Rev Educ Sci. (2015) 1:616–22.
213. Levman J, MacDonald P, Lim AR, Forgeron C, Takahashi E. A pediatric structural MRI analysis of healthy brain development from newborns to young adults. Hum Brain Mapp. (2017) 38:5931–42. doi: 10.1002/hbm.23799
214. Barbeau EB, Meilleur AAS, Zeffiro TA, Mottron L. Comparing motor skills in autism spectrum individuals with and without speech delay. Autism Res. (2015) 8:682–93. doi: 10.1002/aur.1483
215. Harris SR. Early motor delays as diagnostic clues in autism spectrum disorder. Eur J Pediatr. (2017) 176:1259–62. doi: 10.1007/s00431-017-2951-7
216. Horn AR, Swingler GH, Myer L, Linley LL, Raban MS, Joolay Y, et al. Early clinical signs in neonates with hypoxic ischemic encephalopathy predict an abnormal amplitude-integrated electroencephalogram at age 6 hours. BMC Pediatr. (2013) 13:52. doi: 10.1186/1471-2431-13-52
217. Bussu G, Jones EJ, Charman T, Johnson MH, Buitelaar JK. Prediction of autism at 3 years from behavioural and developmental measures in high-risk infants: a longitudinal cross-domain classifier analysis. J Autism Dev Disord. (2018) 48:2418–33. doi: 10.1007/s10803-018-3509-x
218. Pijl MKJ, Bussu G, Charman T, Johnson MH, Jones EJ, Pasco G. Temperament as an early risk marker for Autism Spectrum Disorders? A longitudinal study of high-risk and low-risk infants. J Autism Develop Disord. (2019) 49:1825–36. doi: 10.1007/s10803-018-3855-8
219. Sacrey LA-O, Zwaigenbaum L, Bryson S, Brian J, Smith IM. The reach-to-grasp movement in infants later diagnosed with autism spectrum disorder: a high-risk sibling cohort study. J Neurodev Disord. (2018) 10:1–11. doi: 10.1186/s11689-018-9259-4
220. Acquarone S. Signs of Autism in Infants: Recognition and Early Intervention Milton Park: Routledge (2018).
221. Shultz S, Klin A, Jones W. Neonatal transitions in social behavior and their implications for autism. Trends Cog Sci. (2018) 22:452–69. doi: 10.1016/j.tics.2018.02.012
222. Okamoto R, Saito Y, Inoue T, Maegaki Y, Nagaishi J, Ohno K. Forced mouth opening reaction: a primitive reflex released from cortical inhibition. Brain Dev. (2006) 28:272–4. doi: 10.1016/j.braindev.2005.09.001
223. Camarda C, Torelli P, Pipia C, Azzarello D, Battaglini I, Sottile G, et al. Nociceptive primitive reflexes in neurologically and cognitively healthy aging subjects. Can J Neurol Sci. (2019) 46:199–208. doi: 10.1017/cjn.2018.388
224. Gramespacher H, Richter N, Edwin Thanarajah S, Jacobs HIL, Dillen KNH, et al. Aberrant frontostriatal connectivity in Alzheimer's disease with positive palmomental reflex. Eur J Neurol. (2020) 27:2405–14. doi: 10.1111/ene.14443
225. Plutino A, Baldinelli S, Fiori C, Ranaldi V, Silvestrini M, Luzzi S. Inter-rater reliability of primitive signs in dementia. Clin Neurol Neurosurg. (2019) 187:105555. doi: 10.1016/j.clineuro.2019.105555
226. Machado C, Rodriguez R, Caiballo M, Korein J, Sanchez-Catasus C, Perez J, et al. Brain anatomy, cerebral blood flow, and connectivity in the transition from PVS to MCS. Rev Neurosci. (2009) 20:177–80. doi: 10.1515/REVNEURO.2009.20.3-4.177
227. D'Mello AM, Stoodley CJ. Cerebro-cerebellar circuits in autism spectrum disorder. Front Neurosci. (2015) 9:408. doi: 10.3389/fnins.2015.00408
228. Carper RA, Solders S, Treiber JM, Fishman I, Müller R-A. Corticospinal tract anatomy and functional connectivity of primary motor cortex in autism. J Am Acad Child Adolesc Psychiatry. (2015) 54:859–67. doi: 10.1016/j.jaac.2015.07.007
229. Floris DL, Howells H. A typical structural and functional motor networks in autism. Prog Brain Res. (2018) 238:207–48. doi: 10.1016/bs.pbr.2018.06.010
230. Anteraper SA, Guell X, Hollinshead MO, D'Mello A, Whitfield-Gabrieli S, Biederman J, et al. Functional alterations associated with structural abnormalities in adults with high-functioning autism spectrum disorder. Brain Connect. (2020) 10:368–76. doi: 10.1089/brain.2020.0746
231. Floris DL, Wolfers T, Zabihi M, Holz NE, Zwiers MP, Charman T, et al. Atypical brain asymmetry in autism-a candidate for clinically meaningful stratification. Biol Psychiatry. (2021) 6:802–12. doi: 10.1101/2020.03.24.000349
232. Baduel S, Guillon Q, Afzali MH, Foudon N, Kruck J, Roge B. The french version of the modified-checklist for autism in toddlers (M-CHAT): a validation study on a french sample of 24 month-old children. J Autism Dev Disord. (2017) 47:297–304. doi: 10.1007/s10803-016-2950-y
233. Machado C, Estevez M, Rodriguez R, Leisman G. Letter re: The autism “epidemic”: ethical, legal, and social issues in a developmental spectrum disorder. Neurology. (2017) 89:1310. doi: 10.1212/WNL.0000000000004405
234. Hallahan B, Daly EM, McAlonan G, Loth E, Toal F, O'Brien F, et al. Brain morphometry volume in autistic spectrum disorder: a magnetic resonance imaging study of adults. Psychol Med. (2009) 39:337–46. doi: 10.1017/S0033291708003383
235. Baranek GT, Watson LR, Boyd BA, Poe MD, David FJ, McGuire L. Hyporesponsiveness to social and nonsocial sensory stimuli in children with autism, children with developmental delays, and typically developing children. Dev Psychopathol. (2013) 25:307–20. doi: 10.1017/S0954579412001071
236. Lin CK, Kuo BC, Wu HM. Response to Niklasson's comment on Lin, et al. (2012): “the relation between postural movement and bilateral motor integration.” Percept Mot Skills. (2014) 119:650–4. doi: 10.2466/15.10.PMS.119c24z9
237. Goddard Blythe S. Early learning in the balance: priming the first ABC. Supp Learn. (2000) 15:154–8. doi: 10.1111/1467-9604.00168
238. Jordan-Black JA. The effects of the Primary Movement programme on the academic performance of children attending ordinary primary school. J Res Spec Educ Needs. (2005) 5:101–11. doi: 10.1111/j.1471-3802.2005.00049.x
239. McPhillips M, Hepper PG, Mulhern G. Effects of replicating primary-reflex movements on specific reading difficulties in children: a randomised, double-blind, controlled trial. Lancet. (2000) 355:537–41. doi: 10.1016/S0140-6736(99)02179-0
240. Goddard Blythe S, Hyland D. Screening for neurological dysfunction in the specific learning difficulty child. Br J Occup Ther. (1998) 61:459–64. doi: 10.1177/030802269806101008
241. Goddard Blythe S. Releasing educational potential through movement: a summary of individual studies carried out using the INPP test battery and developmental exercise programme for use in schools with children with special needs. Child Care Pract. (2005) 11:415–32. doi: 10.1080/13575270500340234
242. Goddard Blythe S. Attention, Balance and Coordination: The ABC of Learning Success. Chichester, UK: John Wiley and Sons (2017). doi: 10.1002/9781119164746
243. Goddard Blythe S, Duncombe R, Preedy P, Gorely T. Neuromotor readiness for school: the primitive reflex status of young children at the start and end of their first year at school in the United Kingdom. Education. (2021) 3–13:1–14. doi: 10.1080/03004279.2021.1895276
244. Brown C. Improving fine motor skills in young children: an intervention study. Educ Psychol Pract. (2010) 26:269–78. doi: 10.1080/02667363.2010.495213
245. Payler J, Davis G, Jarvis P, Georgeson J, Wood E, Lloyd E. BERA-TACTYC early childhood education and care review 2004-2015. In: British Educational Research Association Conference 2016. Leeds: University of Leeds (2017).
246. Chambers ME, Sugden DA. Intervention for young children displaying coordination disorders. J Early Childhood Res. (2016) 14:115–131. doi: 10.1177/1476718X14548780
247. Pascal C, Bertram T, Peckham K. DfE Review of Evidence on EYFS Early Learning Goals, Teaching Content and Pedagogy in Reception Year. London: DfE (2018). doi: 10.12968/nuwa.2018.9.34
248. Leisman G, Mualem R, Mughrabi SK. The neurological development of the child with the educational enrichment in mind. Psicol Educ. (2015) 21:79–96. doi: 10.1016/j.pse.2015.08.006
249. Slater JL, Tate MC. Timing deficits in ADHD: insights from the neuroscience of musical rhythm. Front Comput Neurosci. (2018) 12:51. doi: 10.3389/fncom.2018.00051
250. Reynolds D, Nicolson RI, Hambly H. Evaluation of an exercise-based treatment for children with reading difficulties. Dyslexia. (2003) 9:48–71. doi: 10.1002/dys.235
251. Niklasson M, Norlander T, Niklasson I, Rasmussen P. Catching-up: children with developmental coordination disorder compared to healthy children before and after sensorimotor therapy. PLoS ONE. (2017) 12:e0186126. doi: 10.1371/journal.pone.0186126
252. Hyatt KJ, Stephenson J, Carter M. A review of three controversial educational practices: perceptual motor programs, sensory integration, and tinted lenses. Educ Treat Child. (2009) 32:313–42. doi: 10.1353/etc.0.0054
253. Moreau D, Waldie KE. Developmental learning disorders: from generic interventions to individualized remediation. Front Psychol. (2016) 6:2053. doi: 10.3389/fpsyg.2015.02053
254. Faramarzi S, Rad SA, Abedi A. Effect of sensory integration training on executive functions of children with attention deficit hyperactivity disorder. Neuropsychiatry Neuropsychol. (2016) 11:1–5.
255. Grzywniak C. Integration exercise programme for children with learning difficulties who have preserved vestigial primitive reflexes. Acta Neuropsychol. (2017) 15:241–256. doi: 10.5604/01.3001.0010.5491
256. Pimentel ED. The disappearing reflex: a reevaluation of its role in normal and abnormal development. Phys Occup Ther Pediatr. (1996) 16:19–42. doi: 10.1080/J006v16n04_02
257. Anderson GM. Conceptualizing autism: the role for emergence. J Am Acad Child Adolesc Psychiatry. (2009) 48:688–91. doi: 10.1097/CHI.0b013e3181a5e3d5
258. Stanton ME, Peloso E, Brown KL, Rodier P. Discrimination learning and reversal of the conditioned eyeblink reflex in a rodent model of autism. Behav Brain Res. (2007) 176:133–40. doi: 10.1016/j.bbr.2006.10.022
259. de Bildt A, Mulder EJ, Van Lang NDJ, de With SAJ, Minderaa RB, Stahl SS, et al. The visual rooting reflex in individuals with autism spectrum disorders and cooccurring intellectual disability. Autism Res. (2012) 5:67–72. doi: 10.1002/aur.225
260. Melillo R. Chapter VI: Persistent primitive reflexes and childhood neurobehavioral disorders. In: Leisman G, Merrick J, editors. Neuroplasticity in Learning and Rehabilitation. New York, NY: Nova Biomedical Books; Hauppauge (2016). p. 65–99.
261. Futagi Y, Suzuki Y. Neural mechanism and clinical significance of the plantar grasp reflex in infants. Pediatr Neurol. (2010) 43:81–6. doi: 10.1016/j.pediatrneurol.2010.04.002
262. Ottenbacher K. Sensory integration therapy: affect or effect. Am J Occup Ther. (1982) 36:571–8. doi: 10.5014/ajot.36.9.571
263. Zwaigenbaum L, Bryson S, Garon N. Early identification of autism spectrum disorders. Behav Brain Res. (2013) 251:133–46. doi: 10.1016/j.bbr.2013.04.004
264. Rider BA. Relationship of postural reflexes to learning disabilities. Am J Occup Ther. (1972) 26:239–43.
265. Endler P. Postural and reflex integration in schizophrenic patients. Am J Occup Ther.. (1978) 32:456–9.
266. Smith J, Clark G, Schlabech T. Systematic review of interventions used in occupational therapy to promote motor performance for children ages birth-5 years. Am J Occup Ther. (2013) 67:413–24. doi: 10.5014/ajot.2013.005959
267. Mailloux Z, Leão M, Becerra TA, Mori AB, Soechting E, Roley SS, et al. Modification of the postrotary nystagmus test for evaluating young children. Am J Occup Ther. (2014) 68:514–21. doi: 10.5014/ajot.2014.011031
268. Barrett H, Benson A, Boucher A, Burch SG, Cash AJ, Creger A, et al. Reflex based interventions for children with autism and developmental disabilities: an evidence-based practice project (2016). St. Catherine University. Available online at: https://sophia.stkate.edu/ma_osot/11
269. So C, Pierluissi E. Attitudes and expectations regarding exercise in the hospital of hospitalized older adults: a qualitative study. J Amer Geriatr Soc. (2012) 60:713–8. doi: 10.1111/j.1532-5415.2012.03900.x
270. Thompson L, Penaloza RA, Stormfields K, Kooistra R, Valencia-Moscoso G, Muslima H, et al. Validation and adaptation of rapid neurodevelopmental assessment instrument for infants in Guatemala. Child Care Health Dev. (2015) 41:1131–9. doi: 10.1111/cch.12279
271. Thompson A, Murphy D, Dell'Acqua F, Ecker C, McAlonan G, Howells H, et al. Impaired communication between the motor and somatosensory homunculus is associated with poor manual dexterity in autism spectrum disorder. Biol Psychiatry. (2017) 81:211–9. doi: 10.1016/j.biopsych.2016.06.020
272. Rice C. Prevalence of autism spectrum disorders: autism and developmental disabilities monitoring network, United States, 2006. In: Morbidity and Mortality Weekly Report. Surveillance Summaries. Vol. 58. Centers for Disease Control and Prevention (2009).
273. Zilbovicius M, Garreau B, Samson Y, Remy P, Barthelemy C, Syrota A, et al. Delayed maturation of the frontal cortex in childhood autism. Am J Psychiatry. (1995) 152:248–52. doi: 10.1176/ajp.152.2.248
274. Farmer CA, Chilakamarri P, Thurm AE, Swedo SE, Holmes GL, Buckley AW. Spindle activity in young children with autism, developmental delay, or typical development. Neurology. (2018) 91:e112–22. doi: 10.1212/WNL.0000000000005759
275. Ha S, Lee H, Choi Y, Kang H, Jeon SJ, Ryu JH, et al. Maturational delay and asymmetric information flow of brain connectivity in SHR model of ADHD revealed by topological analysis of metabolic networks. Sci Rep. (2020) 10:1–13. doi: 10.1038/s41598-020-59921-4
276. Jarczok TA, Fritsch M, Kröger A, Schneider AL, Althen H, Siniatchkin M, et al. Maturation of interhemispheric signal propagation in autism spectrum disorder and typically developing controls: a TMS-EEG study. J Neural Transm. (2016) 123:925–35. doi: 10.1007/s00702-016-1550-5
277. Bilbilaj S. Measuring primitive reflexes in children with learning disorders. Eur J Multidiscip Stud. (2017) 2:285–98. doi: 10.26417/ejms.v5i1.p285-298
278. Ronicko JF, Thomas J, Thangavel P, Koneru V, Langs G, Dauwels J. Diagnostic classification of autism using resting-state fMRI data improves with full correlation functional brain connectivity compared to partial correlation. J Neurosci Methods. (2020) 345:108884. doi: 10.1016/j.jneumeth.2020.108884
279. Lepping RJ, McKinney WS, Magnon GC, Keedy SK, Wang Z, Coombes SA, et al. Visuomotor brain network activation and functional connectivity among individuals with autism spectrum disorder. Hum Brain Mapp. (2021) 43:844–859. doi: 10.1101/2021.03.31.21254423
280. Lorenzini L, van Wingen G, Cerliani L. Atypically high influence of subcortical activity on primary sensory regions in autism. Neuroimage Clin. (2021) 32:102839. doi: 10.1016/j.nicl.2021.102839
281. Tan U. A wrist-walker exhibiting no “Uner Tan Syndrome”: a theory for possible mechanisms of human devolution toward the atavistic walking patterns. Int J Neurosci. (2007) 117:147–56. doi: 10.1080/00207450600936866
282. Zhang Z, Gibson JR, Huber KM. Experience-dependent weakening of callosal synaptic connections in the absence of postsynaptic FMRP. Elife. (2021) 10:71555. doi: 10.7554/eLife.71555
283. Rossi AM, Fernandes VM, Desplan C. Timing temporal transitions during brain development. Curr Opin Neurobiol. (2017) 42:84–92. doi: 10.1016/j.conb.2016.11.010
284. Hambrick EP, Brawner TW, Perry BD. Timing of early-life stress and the development of brain-related capacities. Front Behav Neurosci. (2019) 183. doi: 10.3389/fnbeh.2019.00183
285. Silk TJ, Vilgis V, Adamson C, Chen J, Smit L, Vance A, et al. Abnormal asymmetry in frontostriatal white matter in children with attention deficit hyperactivity disorder. Brain Imaging Behav. (2016) 10:1080–9. doi: 10.1007/s11682-015-9470-9
286. He N, Palaniyappan L, Linli Z, Guo S. Abnormal hemispheric asymmetry of both brain function and structure in attention deficit/hyperactivity disorder: a meta-analysis of individual participant data. Brain Imaging Behav. (2022) 16:54–68. doi: 10.1007/s11682-021-00476-x
287. Wei L, Zhong S, Nie S, Gong G. Aberrant development of the asymmetry between hemispheric brain white matter networks in autism spectrum disorder. Eur Neuropsychopharmacol. (2018) 28:48–62. doi: 10.1016/j.euroneuro.2017.11.018
288. Postema MC, Van Rooij D, Anagnostou E, Arango C, Auzias G, Behrmann M, et al. Altered structural brain asymmetry in autism spectrum disorder in a study of 54 datasets. Nat Commun. (2019) 10:1–12. doi: 10.1038/s41467-019-13005-8
289. Cheung PPP, Lau BWM. Neurobiology of sensory processing in autism spectrum disorder. Prog Mol Biol Transl Sci. (2020) 173:161–81. doi: 10.1016/bs.pmbts.2020.04.020
290. Yuk V, Urbain C, Anagnostou E, Taylor MJ. Frontoparietal network connectivity during an n-back task in adults with autism spectrum disorder. Front Psychiatry. (2020) 11:551808. doi: 10.3389/fpsyt.2020.551808
291. Leisman G, Machado C, Machado Y, Chinchilla-Acosta M. Effects of low-level laser therapy in autism spectrum disorder. Adv Exp Med Biol. (2018) 1116:111–30. doi: 10.1007/5584_2018_234
293. Leisman G, Macahdo C, Melillo R, Mualem R. Intentionality and “free-will” from a neurodevelopmental perspective. Front Integr Neurosci. (2012) 6:36. doi: 10.3389/fnint.2012.00036
294. Mokobane M, Pillay BJ, Meyer A. Fine motor deficits and attention deficit hyperactivity disorder in primary school children. S Afr J Psychiatr. (2019) 25:1232. doi: 10.4102/sajpsychiatry.v25i0.1232
Keywords: top-down processing, bottom-up processing, neuronal synchrony, maturational delay, autism spectrum disorders, retained primitive reflexes
Citation: Melillo R, Leisman G, Machado C, Machado-Ferrer Y, Chinchilla-Acosta M, Kamgang S, Melillo T and Carmeli E (2022) Retained Primitive Reflexes and Potential for Intervention in Autistic Spectrum Disorders. Front. Neurol. 13:922322. doi: 10.3389/fneur.2022.922322
Received: 17 April 2022; Accepted: 06 June 2022;
Published: 07 July 2022.
Edited by:
Hong Ni, Children's Hospital of Soochow University, ChinaReviewed by:
Ewa Zofia Gieysztor, Wroclaw Medical University, PolandCopyright © 2022 Melillo, Leisman, Machado, Machado-Ferrer, Chinchilla-Acosta, Kamgang, Melillo and Carmeli. This is an open-access article distributed under the terms of the Creative Commons Attribution License (CC BY). The use, distribution or reproduction in other forums is permitted, provided the original author(s) and the copyright owner(s) are credited and that the original publication in this journal is cited, in accordance with accepted academic practice. No use, distribution or reproduction is permitted which does not comply with these terms.
*Correspondence: Gerry Leisman, Zy5sZWlzbWFuQGFsdW1uaS5tYW5jaGVzdGVyLmFjLnVr
Disclaimer: All claims expressed in this article are solely those of the authors and do not necessarily represent those of their affiliated organizations, or those of the publisher, the editors and the reviewers. Any product that may be evaluated in this article or claim that may be made by its manufacturer is not guaranteed or endorsed by the publisher.
Research integrity at Frontiers
Learn more about the work of our research integrity team to safeguard the quality of each article we publish.