- 1Department of Luhe Institute of Neuroscience, Capital Medical University, Beijing, China
- 2Department of Neurology, Beijing Luhe Hospital, Capital Medical University, Beijing, China
- 3Department of Neurosurgery, Wayne State University School of Medicine, Detroit, MI, United States
- 4Lake Erie College of Osteopathic Medicine at Seton Hill, Greensburg, PA, United States
Thrombectomy or thrombolysis are the current standards of care for acute ischemic stroke (AIS), however, due to time constraints regarding operations and a multitude of contraindications, AIS remains one of the leading causes of death and chronic disability worldwide. In recent years, therapeutic hypothermia has been explored as an adjuvant therapy for AIS treatment and has shown potential to improve outcomes in patients with AIS. In particular, selective therapeutic hypothermia has shown to markedly reduce infarct volumes and have neuroprotective effects, while also minimizing many systemic side effects seen with systemic therapeutic hypothermia. Both preclinical and clinical trials have demonstrated that selective therapeutic hypothermia is a safe and feasible therapy for patients who have suffered an AIS. In this review, we summarize the current update on selective hypothermia through major studies that have been conducted in rodents, large animals, and clinical trials, and briefly discuss the prospects of selective hypothermic research. We hope this review helps facilitate the exploration of other possible adjuvant treatment modalities in the neuroprotection of ischemic stroke, whether upon symptom onset or after vascular recanalization.
Introduction
Fifteen million people suffer a stroke annually, making it the leading cause of acquired disability and second leading cause of death worldwide (1). Acute Ischemic Stroke (AIS) treatment and rehabilitation is an astronomical burden on healthcare systems despite its limited efficacy. AIS has a striking 30-day case fatality rate of 16–23% and leaves up to 50% of survivors chronically disabled (2–4). Vascular recanalization, including thrombolysis and thrombectomy, has been proven to be effective in ideal circumstances. However, because of the narrow therapeutic window for vascular recanalization, AIS morbidity and mortality rates remain high (5). Thus, there is an urgent need to explore alternative and adjuvant treatments (6, 7).
Therapeutic Hypothermia (TH) is a novel treatment for AIS that has been heavily studied and proven to be one of the most effective adjunctive treatments for AIS in Pre-clinical models (8–12). TH intentionally lowers the body temperature to reduce neurologic damage. TH serves as a neuroprotectant by attenuating numerous metabolic and molecular pathways involved in the progression of AIS such as suppressing free radical production, reducing production of inflammatory mediators, modifying ischemia-mediated calcium influx, and reducing blood brain barrier disruption (13). There is growing hope that TH could substantially reduce AIS morbidity and mortality.
TH may be done systemically, by whole body cooling, or selectively, by lowering brain temperature while maintaining core temperature. Systemic TH is done through external surface cooling (e.g., using air blankets, cold saline and alcohol washes, water mattresses, and ice packs), infusions of cooled saline into the veins, or special transvenous endovascular cooling devices. Studies on systemic TH have provided valuable insight on the safety and practicality of this treatment. However, systemic TH poses risks of serious side effects such as hypotension, cardiac arrhythmia, and pneumonia due to its whole-body cooling effects (14–20). Further, previous studies on systemic TH have demonstrated great variability in time-to-target temperature, some up to several hours. Since the neuroprotective window for AIS is usually only 4.5 h, achieving target temperature through systemic methods may not be a practical therapeutic method. However, more recent systemic TH studies showed that, compared with surface cooling techniques that may take over 4 h, intravascular cooling (via the inferior vena cava) combined with intravenous tissue plasminogen activator or a drug cocktail only takes about 1 h to reach target temperature (21, 22).
Selective TH can be achieved through external surface cooling of the head and neck intranasally via devices that pump coolant mists or cooled air into the nasal cavity, endovascular cold saline infusion via intraarterial catheters, or more directly into the ischemic region using intracranial catheters (23–25). Selective TH minimizes systemic side effects seen with systemic TH, such as systemic hypothermia and pneumonia, and reaches target temperatures faster than systemic methods using less fluid than required for systemic cooling (24). Selective TH has made major breakthroughs in rodent and large animal models and shows great clinical promise as an adjuvant treatment for AIS.
In recent years, researchers have made great progress in demonstrating the neuroprotective effects of selective TH in patients with AIS. From rodents, primates, to clinical studies, it has been shown that selective TH is a viable adjunctive technique both Pre- and Post-reperfusion. In this review, we introduce the latest progress of selective TH research, laying the foundation for its transition to clinical practice.
The Concept of Selective Hypothermia and Animal Research
Rodent Stroke Models
Rodent models were the preferred models for early selective TH studies after AIS. Many studies demonstrated that selective TH significantly reduced the volume of infarction in the experimental group compared to the control group with normal body temperature (Table 1). However, these studies varied in the timing of selective TH induction, as well as varying degrees of hypothermia and ischemic injury.
Intra-arterial cold saline infusion has been the core method used in the study of selective TH for preclinical AIS rodent models. IA-CSI was a novel method of selective TH evaluated by Ding et al. in 2002 in an effort to localize TH to minimize its systemic side effects (26). Ding et al. induced transient middle cerebral artery occlusion (tMCAO) using an intraluminal filament, and subsequently infused 7 ml of 23°C or 37°C isotonic saline into the ischemic region at a rate of 2 ml/min (Figure 1). Both 23°C and 37°C saline infusion were found to significantly reduce infarct volumes and improve functional neurologic preservation 48 h Post- reperfusion. A follow-up study conducted by Ding et al. in 2003 (27) demonstrated that Pre-reperfusion IA-CSI was associated with decreased expression of inflammatory markers such as TNF-alpha, ICAM-1, and IL-1beta. This was further supported by a study by Kurisu et al. in 2016 which found that Pre-reperfusion IA-CSI was associated with decreased activation of the inflammatory cascade and improved cerebral microcirculation (32). Kurisu et al. also found that IA-CSI has a protective effect on the blood brain barrier through inhibition of Post-reperfusion aquaporin 4 surge. Improved blood brain barrier preservation was also demonstrated in a 2004 finding by Ding et al. which found that selective TH improves blood brain barrier preservation through decreased matrix metalloproteinase overexpression and marked reductions in cerebral edema (32, 33). Additionally, studies have shown that Pre-reperfusion IA-CSI treatment for AIS widens the therapeutic window for reperfusion to 2–2.5 h, which has the potential to significantly improve morbidity and mortality for AIS patients (28).
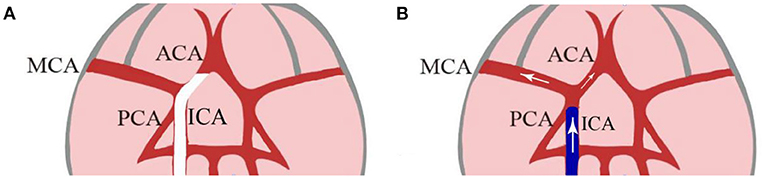
Figure 1. A hollow catheter lodges in the narrow proximal ACA and blocks the MCA at its origin (A). After 2 h of occlusion, saline was flushed into the junction of the MCA and ACA as the hollow filament is withdrawn 1 to 2 mm from the origin of MCA (B). [Modified from Ding et al. (26)]. ACA, anterior cerebral artery; MCA, middle cerebral artery; ECA, external carotid artery; ICA, internal carotid artery.
Several studies have also evaluated the effectiveness of selective TH through simultaneous IA-CSI and reperfusion therapy (25, 29, 30). These studies used slower, prolonged 20°C saline infusion rates at 0.6 ml/min for 10 min (25, 29, 30). Two of these studies achieved target temperatures of 33–34°C within 5 min, which was sustained up to an hour Post-reperfusion without vital sign abnormalities (25, 29). These studies reinforce the effectiveness of selective TH as adjuvant treatment with reperfusion therapy, also demonstrating significant reductions in infarction volume and improved neurologic preservation compared to systemic TH treatment or saline infusion at body temperature (37°C) (29, 30). One of these studies also found that improved neurologic preservation was maintained over 28 days postoperatively.
Post-reperfusion selective TH has also shown promise as a therapeutic intervention for AIS. In 2012, Ji et al. conducted a study introducing a novel, interrupted method for IA-CSI compared to traditional, continuous IA-CSI administration in order to counteract the effects of hemodilution (31). The interrupted IA-CSI technique consisted of tri-sectional infusions with 20 min no-infusion intervals between infusion sessions. Both methods of intervention demonstrated markedly reduced infarct volumes and cerebral edema, however, interrupted IA-CSI was found to drastically prolong hypothermia without evidence of hemodilution (31). In another study, Ji and colleagues assessed Post-reperfusion IA-CSI by comparing continuous IA-CSI with intra-carotid body temperature saline infusion at various latency periods (34). Their results found that in shorter periods, both methods significantly reduced infarct volume, but only IA-CSI was associated with decreased cerebral water content, reduced cell death mediator expression, and improved neurologic preservation (34). Further, Ji et al. found that during extended latency periods, only IA-CSI showed beneficial outcomes. This landmark study built upon the hypothesis that IA-CSI treatment may widen the therapeutic window for reperfusion in AIS patients, which has potential for tremendous reductions in AIS morbidity and mortality and set the precedent for future primate and human trials (34).
As an alternative to saline infusion alone, IA-CSI with alternative neuroprotective agents have also been studied. A study by Chen et al. evaluated the effects of local, cold low-dose albumin infusions as an alternative to saline infusion in AIS (35). Regional, low-dose 0°C human albumin was infused at a rate of 0.5 g/kg into rats with induced tMCAO was found to outperform local cold saline, local and systemic normothermic albumin; and local and systemic normothermic albumin in infarct volume reduction and neurologic function recovery (35). IA-CSI with magnesium sulfate has also been found to reduce infarct volume and enhance neuroprotection in AIS rats, further reflecting the synergistic effects of IA-SCI with other neuroprotective agents (35, 36).
The majority of studies on the utility of selective TH in AIS have used rodent models. This could partly be due to the ability of rodents to better tolerate deeper hypothermia compared to primates and ease of handling, therefore providing more convenient study conditions. However, the use of rodent AIS treatment models accompanies many limitations that limit its direct translation to the clinical setting, such as the differences in brain size (smaller brain size inherently results in faster cooling rates) and post-AIS molecular inflammatory cascades in rodents compared to humans (37). This, along with recommendations from the Stroke Therapy Academic Roundtable to study novel AIS treatment in large animals prior to human trials, has led to increased use of primate models in studying AIS therapeutic intervention in more recent years (37–39) (Table 2).
Large Animal Models
The first study of selective TH for AIS in large animals was conducted in 2007 by Furuse and colleagues using endovascular intra-arterial infusion into the right common carotid artery of canines with tMCAO (40). Ringer's lactate solution at 6.5°C was infused at a rate of 3 ml/kg/min for a duration of 30 min using an angiographic catheter through the right femoral artery (40). The cooling rate of the right cerebral hemisphere was 4°C/30 min, with no significant increase in cerebral extracellular lactate concentration, although hemoglobin and hematocrit decreased significantly during perfusion, they trended toward recovery in the Post-perfusion period (40). On the contrary, cardiac output significantly increased during perfusion and insignificantly decreased during the Post-perfusion period (40). All canines were successfully cooled rapidly and survived without neurological deficits (40). A more recent tMCAO canine study conducted in 2018 by King and colleagues reinforced the promise of selective TH through endovascular cooling using a novel insulated catheter. King et al. showed that selective TH can be achieved quickly and safely and result in significantly smaller infarct volumes than without selective TH (47). Selective TH through endovascular cooling was further validated in AIS canines in 2019 by Caroff et al. through infusion of cold saline at 4.5°C at a rate of 22 ml/min for 25 min using a novel insulated catheter to minimize heat transfer (41). The ipsilateral hemisphere was effectively cooled while minimizing infusion volume (41). This technique resulted in significantly smaller infarct volumes compared to the control group, making strides toward clinical translation by demonstrating these positive effects in large animals using realistic infusion rates and volumes (41).
Selective TH has also been studied using swine and ovine models. Cattaneo and colleagues assessed IA-CSI with a novel balloon cooling catheter system through the common carotid artery in tMCAO sheep. This study demonstrated that this novel method could rapidly induce hypothermia in the ipsilateral cerebral hemisphere and may benefit AIS patients in combination with mechanical thrombectomy (44). Using a swine model, Mattingly et al. studied selective TH in swine with tMCAO using a dual lumen balloon occlusion catheter simultaneously with reperfusion. Mattingly and colleagues induced tMCAO for 3 h using an aneurysm clip, and the outflow catheter was placed in the thoracic aorta to allow blood to exit, become chilled, and then reperfused through an inflow catheter in the common carotid artery (45). Target temperatures of <30°C were reached on average within 15 min and resulted in markedly reduced infarct volumes (45). In addition, intranasal cooling systems have also been widely used in models of hypothermia in swine and ovine stroke models. The nasal anatomical location contributes to heat exchange with the cervical blood and cerebrospinal cord fluid flowing into the brain. The nasal cooling was induced by either a cold water circulating device or coolant mist diffusion. For example, Abou-Chebl et al. assessed the utility of intranasal cooling using a coolant device that sprays perfluorocarbon-oxygen mist into the nasal cavity, where the coolant evaporates and absorbs heat from the tissue through direct conductive and indirect hematogenous mechanisms (48). Intranasal cooling has also been tested by Bakhsheshi et al., who used mechanical tubes to blow cooled air into the nasal cavities, which they theorized cooled the brain selectively due to the cavernous sinus's proximity to blood in the internal carotid artery and cerebrospinal fluid in the basal cistern (46). Both of these intranasal methods are advantageous because they achieve local brain cooling while being minimally invasive, portable, and user friendly without additional extensive training (46, 48). In addition, a new study using a closed-loop system has shown the effectiveness and safety in porcine models, which also prevented local and systemic adverse events (46, 48–51). Since the catheter is introduced only in the left nostril, the preferential decrease in brain temperature radiates on the left side. This effect may help determine the priority of cooling on one side in local neurological disorders such as stroke. The major limitation of swine and ovine experiments is the capability to perfectly mimic the physiology and anatomy of humans due to the presence of a larger nose-to-brain volume. Therefore, Non-human primate and human testing is crucial to ultimately prove cooling efficacy (Table 2).
Non-human Primate Models
Selective TH has also been studied in rhesus monkeys with induced tMCAO (42, 43, 52). In a 2016 study by Wang and colleagues, 0°C Ringer lactate solution was used to induce selective TH in rhesus monkeys with tMCAO (42). The volume of infusion was calculated in proportion to the infusion volume and body weight of rats used in Ding's earlier studies, and the total infusion volume was 100 ml (42). When IA-CSI was infused at 5 ml/min, 0–4°C, Ringer lactate solution reached mild cerebral hypothermia (<35°C) within 10 min; much faster than whole-body infusions using the same protocols (42). Importantly, no significant fluctuations in rectal temperature, hematocrit, cerebral blood flow velocity, or cerebrovascular reactivity were observed during or after reperfusion. In addition, no cerebral edema, new infarction, hemorrhage, or vasospasm were observed, which further validated the safety, feasibility, and efficacy of IA-CSI (42). A 2020 study by Wu et al. combined alteplase with the above-mentioned IA-CSI model for IA thrombolysis, found that those with full or partial reperfusion significantly reduced the volume of infarction, alleviated neurological dysfunction, and improved upper extremity motor dysfunction in both acute and chronic stages (43). However, no further neuroprotective was observed in monkeys without reperfusion (43).
Clinical Evidence for Selective Hypothermia and Potential Applications in Stroke
The progression to larger animals from rodent models and subsequent successful outcomes are encouraging evidence supporting clinical translation of selective TH in the treatment of AIS. However, several quandaries regarding physiologic differences between animals and humans remain that cannot be addressed using animal models (38). Given the promise IA-CSI has shown in the treatment for AIS in both rodent and large animal studies, clinical trials of selective TH have finally begun (Table 3).
Choi and colleagues (24) were the first to evaluate the safety and feasibility of selective TH in a study of 18 patients in 2010. Patients were equally divided into two groups. The first group was used to establish the safety of infusion: 12–17°C cold saline was injected into one side of the internal carotid artery at a rate of 33 ml/min for a duration of 10 min. The second group, using the same protocol, received infusions of lower temperature saline at 4–7°C, and although the bulb temperature decreased by an average of 0.84°C, the core temperature only dropped by an average of 0.15°C. The biggest drawback of this experiment is that it could not measure the temperature of the brain parenchyma directly as was done in animal studies. Since the jugular venous bulb drains blood from the entire head, the decrease in target parenchymal tissue was likely much greater than the measured 0.84°C (57, 58). However, in a subsequent 2013 study, the team used mathematical models to predict changes in brain parenchymal temperature more accurately. They utilized a previously established biophysical mathematical model, which estimated that a 10 min infusion time would decrease the temperature of ipsilateral anterior circulation territory by about 2°C. It takes less time to reach a temperature comparable to the systemic hypothermia temperature (59).
A study by Chen et al. in 2016 was the first pilot study of selective TH in AIS patients. Twenty six patients with large vessel occlusion eligible for mechanical thrombectomy were enrolled within 8 h of AIS onset (53). Each patient received IA-CSI with 4°C cold isotonic saline in conjunction with reperfusion treatment into the ischemic region. Although the study did not measure venous outflow temperatures, the combination of Pre-reperfusion and Post-reperfusion IA-CSI was estimated to decrease the temperature of ischemic cerebral tissue by at least 2°C during infusion of the 4°C isotonic saline and systemic temperatures were mildly reduced (53). Importantly, no obvious complications related to IA-CSI occurred (53). This landmark study demonstrated that IA-SCI treatment with cold saline is a safe and practical method to employ in patients with AIS (53).
Following the success of the 2016 pilot study, a larger prospective cohort study was launched in 2018 by Wu et al. to evaluate the safety and efficacy of IA-CSI in patients undergoing mechanical thrombectomy compared to mechanical thrombectomy without IA-CSI (54). Using the same pre- and Post-reperfusion infusion protocol, the Wu et al. study found that IA-CSI reduced infarct volumes by an average of 19.1 ml, based on Non-contrast CT 3–7 days Post-intervention. Further, this study demonstrated that IA-CSI promoted functional independence measured at 90 days Post-procedure compared to patients who received only thrombectomy therapy, although this difference was not statistically significant (54). However, patients who received both IA-CSI and reperfusion therapy had lower Alberta Stroke Program Early Computed Tomography Scores and poor collateral circulation at baseline, thus it is likely that the neurological function retention provided by IA-CSI may be stronger than shown in this study (54). Nonetheless, these results still provide valuable data for future follow-up clinical trials. Currently, two clinical trials are underway, being conducted by Tokairin et al. and Wu et al. to further evaluate the utility of IA-SCI combined with mechanical thrombectomy in AIS patients (60, 61).
Due to the Non-invasive and convenient nature of the nasal cooling technique for TH, this method may be a favorable possibility for clinical translation. Twenty stroke patients who received neurological monitoring and treatment in a neurocare unit underwent intravenous cold systemic cooling or intranasal cooling at a rate of 60 L/minute in a 1:1 random manner (55). While systemic cold infusions achieve brain cooling faster than intranasal infusions, their effects on systolic arterial pressure, mean arterial pressure, intracranial pressure, and cerebral perfusion pressure raise concerns about the safety of both cooling methods. A similar study in stroke patients in intensive care investigated the feasibility of cooling the brain within 1 h of nasal cooling with a coolant flow rate of 80 L/minute (48). However, the core temperature dropped by 0.6°C. Transient hypertension was the only adverse event in 1 patient, for whom intranasal cooling was stopped.
The administration of fluorocarbon-rich gasses (48, 55, 62) and oxygen (46, 49–51) do not allow precise temperature control, and safety concerns remain (63). So, the closed-loop system for circulating cold water was explored. A prospective, Non-randomized, interventional clinical trial involving five patients with severe traumatic brain injury (56). Interventions included inducing and maintaining selective brain cooling for 24 h by positioning the catheter in the nasopharynx and circulating cold in a closed loop arrangement within the catheter. Using counter-warming to keep the core temperature at ≥35°C. Studies have demonstrated that this method is safe and effective in humans. However, due to low recruitment rates, investigators chose to terminate the study early. Although the sample size is small, five patients are sufficient to determine the outcome of the Pre-specified outcome and have the potential to demonstrate the clinical and physiological benefits of the technique.
Other Novel Selective Hypothermia
More recently, a novel technique of brain cooling via the internal jugular vein (IJV) has been explored as a more convenient, faster, and economically savvy method of inducing selective TH. Duan et al. demonstrated the effectiveness of IJV administration in rats with tMCAO, by comparing IJV cooling with internal carotid artery cooling, both of which were infused with 6 ml of 0°C isotonic saline for 30 min. Findings demonstrated similar efficacy and neuroprotective benefits to those provided by intracarotid hypothermia (60).
The main advantage of using the IJV as a cold infusion channel is the ease of entry. The IJV is the most common site for central tube placement, a common bedside procedure. Thus when an acute stroke occurs, a tube for cold infusion could theoretically be placed with ease in the emergency room within minutes. In contrast, the carotid artery or direct access to the infarction site can only be accessed under surgical conditions. Additionally, theoretical models have shown that when the two carotid arteries are at sufficiently different temperatures, they tend to lose heat through IJV reverse cooling (64). Therefore, selective TH through IJV cold infusion may be cooled by the countercurrent of the carotid blood. Although research must be done to demonstrate the efficacy and safety of selective TH through IJV cold infusion, the use of the IJV for infusion could be instrumental in providing early AIS treatment to minimize brain damage and maximize functional preservation.
Hypothermia has also been found to enhance the efficacy of other neuroprotective agents (65). Combination therapies involving hypothermia and adjunctive drugs, including phenobarbital, topiramate, erythropoietin, xenon, magnesium sulfate solution, have been reported to be more effective than TH alone (36, 66–69). In a 2017 preclinical study, Wu and colleagues found that while IA-CSI or dihydrocapsaicin alone achieved acceptable cooling and neuroprotection, their combined administration further reduced brain temperature and infarct volume and improved ischemic neurological deficit. The combination of physical and pharmacological hypothermia positively affects energy metabolism, oxidative stress, apoptosis, blood-brain barrier integrity, and inflammatory responses (70, 71). In 2021, Byun et al. demonstrated that the combination of carnosine and hypothermia was neuroprotective in a neonatal rat model of hypoxic-ischemic brain injury (72). In the same year, a study conducted using neonatal rats with hypoxic brain injury used combined treatment with carnosine and hypothermia, which resulted in reduced brain damage (73). These studies provide the possibility to explore more diverse treatments for neurological diseases.
Perspective and Prospective
While research on selective TH has made major strides in the last two decades, there is still an urgent need for progress in clinical translation prior to clinical implementation as an adjuvant treatment for AIS. Studies completed to date have utilized a variety of target temperatures, infusion durations, induction times, and methods, and thus a standardized approach for selective TH has not yet been established. Further, while studies have demonstrated safety and efficacy of this treatment, this procedure has the potential for serious adverse effects resulting from deep hypothermia or excessive infusion. In order for selective TH to gain clinical approval, depth, duration, and treatment window, as well as type of infusion with or without other medicines must all be determined and optimized. Future studies should focus on optimizing these variables in clinical trials so that a standard operating procedure may be established.
The neuroprotective benefits of selective TH have been well demonstrated in preclinical models and its safety and feasibility have been well established in clinical models of AIS. It is worth mentioning that the technical skills and equipment required for selective TH treatment are no different than those to perform a thrombectomy, and there are no obvious financial barriers to employing selective TH in conjunction with reperfusion treatment. Therefore, selective TH shows immense promise as an adjuvant technique to improve acute and long-term outcomes in patients who have suffered AIS.
Author Contributions
XW, AW, SK, and NC wrote and edited the manuscript. XG and YD were also involved in drafting the manuscript and revising it critically for important intellectual content. All authors contributed to the article and approved the submitted version.
Funding
This study was supported partially by the National Natural Science Foundation of China (82072549, 81871838, 82001277, and 82101436), the Youth Scientific Research Incubation Program of Beijing Luhe Hospital, Capital Medical University (LHYY2021-JC04), the Beijing Tongzhou District Financial Fund, and the Science and Technology Plan of Beijing Tongzhou District (KJ2022CX033).
Conflict of Interest
The authors declare that the research was conducted in the absence of any commercial or financial relationships that could be construed as a potential conflict of interest.
Publisher's Note
All claims expressed in this article are solely those of the authors and do not necessarily represent those of their affiliated organizations, or those of the publisher, the editors and the reviewers. Any product that may be evaluated in this article, or claim that may be made by its manufacturer, is not guaranteed or endorsed by the publisher.
References
1. Katan M, Luft A. Global Burden of Stroke. Semin Neurol. (2018) 38:208–11. doi: 10.1055/s-0038-1649503
2. Grysiewicz RA, Thomas K, Pandey DK. Epidemiology of ischemic and hemorrhagic stroke: incidence, prevalence, mortality, and risk factors. Neurol Clin. (2008) 26:871–95, vii. doi: 10.1016/j.ncl.2008.07.003
3. Feigin VL, Lawes CM, Bennett DA, Anderson CS. Stroke epidemiology: a review of population-based studies of incidence, prevalence, and case-fatality in the late 20th century. Lancet Neurol. (2003) 2:43–53. doi: 10.1016/S1474-4422(03)00266-7
4. Lopez AD, Mathers CD, Ezzati M, Jamison DT, Murray CJ. Global and regional burden of disease and risk factors, 2001: systematic analysis of population health data. Lancet. (2006) 367:1747–57. doi: 10.1016/S0140-6736(06)68770-9
5. Leng T, Xiong ZG. Treatment for ischemic stroke: from thrombolysis to thrombectomy and remaining challenges. Brain Circ. (2019) 5:8–11. doi: 10.4103/bc.bc_36_18
6. Zhao W, Wu C, Dornbos D, 3rd, Li S, Song H, et al. Multiphase Adjuvant Neuroprotection: A Novel Paradigm for Improving Acute Ischemic Stroke Outcomes. Brain Circ. (2020) 6:11–8. doi: 10.4103/bc.bc_58_19
7. Hollist M, Morgan L, Cabatbat R, Au K, Kirmani MF, Kirmani BF. Acute stroke management: overview and recent updates. Aging Dis. (2021) 12:1000–9. doi: 10.14336/AD.2021.0311
8. van der Worp HB, Sena ES, Donnan GA, Howells DW, Macleod MR. Hypothermia in animal models of acute ischaemic stroke: a systematic review and meta-analysis. Brain. (2007) 130(Pt 12):3063–74. doi: 10.1093/brain/awm083
9. Dumitrascu OM, Lamb J, Lyden PD. Still cooling after all these years: meta-analysis of pre-clinical trials of therapeutic hypothermia for acute ischemic stroke. J Cereb Blood Flow Metab. (2016) 36:1157–64. doi: 10.1177/0271678X16645112
10. Campbell BC, Mitchell PJ, Kleinig TJ, Dewey HM, Churilov L, Yassi N, et al. Endovascular therapy for ischemic stroke with perfusion-imaging selection. N Engl J Med. (2015) 372:1009–18. doi: 10.1056/NEJMoa1414792
11. Hill MD, Goyal M, Menon BK, Nogueira RG, McTaggart RA, Demchuk AM, et al. Efficacy and safety of nerinetide for the treatment of acute ischaemic stroke (Escape-Na1): a multicentre, double-blind, randomised controlled trial. Lancet. (2020) 395:878–87. doi: 10.1016/S0140-6736(20)30258-0
12. Lee H, Ding Y. Temporal limits of therapeutic hypothermia onset in clinical trials for acute ischemic stroke: how early is early enough? Brain Circ. (2020) 6:139–44. doi: 10.4103/bc.bc_31_20
13. Zhong W, Yuan Y, Gu X, Kim SI, Chin R, Loye M, et al. Neuropsychological deficits chronically developed after focal ischemic stroke and beneficial effects of pharmacological hypothermia in the mouse. Aging Dis. (2020) 11:1–16. doi: 10.14336/AD.2019.0507
14. Kurisu K, Yenari MA. Therapeutic hypothermia for ischemic stroke; pathophysiology and future promise. Neuropharmacology. (2018) 134(Pt B):302–9. doi: 10.1016/j.neuropharm.2017.08.025
15. Georgiadis D, Schwarz S, Kollmar R, Schwab S. Endovascular cooling for moderate hypothermia in patients with acute stroke: first results of a novel approach. Stroke. (2001) 32:2550–3. doi: 10.1161/hs1101.097382
16. Hoedemaekers CW, Ezzahti M, Gerritsen A, van der Hoeven JG. Comparison of cooling methods to induce and maintain normo- and hypothermia in intensive care unit patients: a prospective intervention study. Crit Care. (2007) 11:R91. doi: 10.1186/cc6104
17. Hong JM, Lee JS, Song HJ, Jeong HS, Choi HA, Lee K. Therapeutic hypothermia after recanalization in patients with acute ischemic stroke. Stroke. (2014) 45:134–40. doi: 10.1161/STROKEAHA.113.003143
18. de Georgia MA, Krieger DW, Abou-Chebl A, Devlin TG, Jauss M, Davis SM, et al. Cooling for acute ischemic brain damage (cool aid): a feasibility trial of endovascular cooling. Neurology. (2004) 63:312–7. doi: 10.1212/01.WNL.0000129840.66938.75
19. Bi M, Ma Q, Zhang S, Li J, Zhang Y, Lin L, et al. Local mild hypothermia with thrombolysis for acute ischemic stroke within a 6-H window. Clin Neurol Neurosurg. (2011) 113:768–73. doi: 10.1016/j.clineuro.2011.08.010
20. Els T, Oehm E, Voigt S, Klisch J, Hetzel A, Kassubek J. Safety and therapeutical benefit of hemicraniectomy combined with mild hypothermia in comparison with hemicraniectomy alone in patients with malignant ischemic stroke. Cerebrovasc Dis. (2006) 21:79–85. doi: 10.1159/000090007
21. Nielsen N, Wetterslev J, Cronberg T, Erlinge D, Gasche Y, Hassager C, et al. Targeted temperature management at 33 degrees C vs. 36 degrees C after cardiac arrest. N Engl J Med. (2013) 369:2197–206. doi: 10.1056/NEJMoa1310519
22. Hemmen TM, Raman R, Guluma KZ, Meyer BC, Gomes JA, Cruz-Flores S, et al. Intravenous thrombolysis plus hypothermia for acute treatment of ischemic stroke (Ictus-L): final results. Stroke. (2010) 41:2265–70. doi: 10.1161/STROKEAHA.110.592295
23. Kuczynski AM, Marzoughi S, Al Sultan AS, Colbourne F, Menon BK, van Es A, et al. Therapeutic hypothermia in acute ischemic stroke-a systematic review and meta-analysis. Curr Neurol Neurosci Rep. (2020) 20:13. doi: 10.1007/s11910-020-01029-3
24. Choi JH, Marshall RS, Neimark MA, Konstas AA, Lin E, Chiang YT, et al. Selective brain cooling with endovascular intracarotid infusion of cold saline: a pilot feasibility study. AJNR Am J Neuroradiol. (2010) 31:928–34. doi: 10.3174/ajnr.A1961
25. Luan XD, Li J, McAllister JP, Diaz FG, Clark JC, Fessler RD, et al. Regional brain cooling induced by vascular saline infusion into ischemic territory reduces brain inflammation in stroke. Acta Neuropathol. (2004) 107:227–34. doi: 10.1007/s00401-003-0802-2
26. Ding YC, Li J, Rafols JA, Phillis JW, Diaz FG. Prereperfusion saline infusion into ischemic territory reduces inflammatory injury after transient middle cerebral artery occlusion in rats. Stroke. (2002) 33:2492–8. doi: 10.1161/01.STR.0000028237.15541.CC
27. Ding Y, Young CN, Li J, Luan X, McAllister JP, 2nd, et al. Reduced inflammatory mediator expression by pre-reperfusion infusion into ischemic territory in rats: a real-time polymerase chain reaction analysis. Neurosci Lett. (2003) 353:173–6. doi: 10.1016/j.neulet.2003.09.055
28. Zhao WH, Ji XM, Ling F, Ding YC, Xing CH, Wu H, et al. Local mild hypothermia induced by intra-arterial cold saline infusion prolongs the time window of onset of reperfusion injury after transient focal ischemia in rats. Neurol Res. (2009) 31:43–51. doi: 10.1179/174313208X327982
29. Ding Y, Li J, Luan X, Lai Q, McAllister JP 2nd, et al. Local saline infusion into ischemic territory induces regional brain cooling and neuroprotection in rats with transient middle cerebral artery occlusion. Neurosurgery. (2004) 54:956–64. doi: 10.1227/01.NEU.0000114513.96704.29
30. Li J, Luan X, Lai Q, Clark JC, McAllister JP 2nd, et al. Long-term neuroprotection induced by regional brain cooling with saline infusion into ischemic territory in rats: a behavioral analysis. Neurol Res. (2004) 26:677–83. doi: 10.1179/016164104225015903
31. Ji YB, Wu YM, Ji Z, Song W, Xu SY, Wang Y, et al. Interrupted intracarotid artery cold saline infusion as an alternative method for neuroprotection after ischemic stroke. Neurosurg Focus. (2012) 33:E10. doi: 10.3171/2012.5.FOCUS1215
32. Kurisu K, Abumiya T, Nakamura H, Shimbo D, Shichinohe H, Nakayama N, et al. Transarterial regional brain hypothermia inhibits acute aquaporin-4 surge and sequential microvascular events in ischemia/reperfusion injury. Neurosurgery. (2016) 79:125–34. doi: 10.1227/NEU.0000000000001088
33. Ding YH, Li J, Rafols JA, Ding Y. Reduced brain edema and matrix metalloproteinase (Mmp) expression by pre-reperfusion infusion into ischemic territory in rat. Neurosci Lett. (2004) 372:35–9. doi: 10.1016/j.neulet.2004.09.010
34. Ji Y, Hu Y, Wu Y, Ji Z, Song W, Wang S, et al. Therapeutic time window of hypothermia is broader than cerebral artery flushing in carotid saline infusion after transient focal ischemic stroke in rats. Neurol Res. (2012) 34:657–63. doi: 10.1179/1743132812Y.0000000061
35. Chen J, Fredrickson V, Ding Y, Cheng H, Wang N, Ling F, et al. Enhanced neuroprotection by local intra-arterial infusion of human albumin solution and local hypothermia. Stroke. (2013) 44:260–2. doi: 10.1161/STROKEAHA.112.675462
36. Song W, Wu YM, Ji Z, Ji YB, Wang SN, Pan SY. Intra-carotid cold magnesium sulfate infusion induces selective cerebral hypothermia and neuroprotection in rats with transient middle cerebral artery occlusion. Neurol Sci. (2013) 34:479–86. doi: 10.1007/s10072-012-1064-3
37. Kwiecien TD, Sy C, Ding Y. Rodent models of ischemic stroke lack translational relevance … are baboon models the answer? Neurol Res. (2014) 36:417–22. doi: 10.1179/1743132814Y.0000000358
38. Stroke Therapy Academic Industry R. Recommendations for standards regarding preclinical neuroprotective and restorative drug development. Stroke. (1999) 30:2752–8. doi: 10.1161/01.STR.30.12.2752
39. Fisher M, Feuerstein G, Howells DW, Hurn PD, Kent TA, Savitz SI, et al. Update of the stroke therapy academic industry roundtable preclinical recommendations. Stroke. (2009) 40:2244–50. doi: 10.1161/STROKEAHA.108.541128
40. Furuse M, Preul MC, Kinoshita Y, Nishihara K, Isono N, Kuroiwa T. Rapid induction of brain hypothermia by endovascular intra-arterial perfusion. Neurol Res. (2007) 29:53–7. doi: 10.1179/174313206X152537
41. Caroff J, King RM, Mitchell JE, Marosfoi M, Licwinko JR, Gray-Edwards HL, et al. Focal cooling of brain parenchyma in a transient large vessel occlusion model: proof-of-concept. J Neurointerv Surg. (2020) 12:209–13. doi: 10.1136/neurintsurg-2019-015179
42. Wang B, Wu D, Dornbos Iii D, Shi J, Ma Y, Zhang M, et al. Local cerebral hypothermia induced by selective infusion of cold lactated ringer's: a feasibility study in rhesus monkeys. Neurol Res. (2016) 38:545–52. doi: 10.1080/01616412.2016.1187827
43. Wu D, Chen J, Hussain M, Wu L, Shi J, Wu C, et al. Selective intra-arterial brain cooling improves long-term outcomes in a non-human primate model of embolic stroke: efficacy depending on reperfusion status. J Cereb Blood Flow Metab. (2020) 40:1415–26. doi: 10.1177/0271678X20903697
44. Cattaneo G, Schumacher M, Maurer C, Wolfertz J, Jost T, Buchert M, et al. Endovascular cooling catheter for selective brain hypothermia: an animal feasibility study of cooling performance. AJNR Am J Neuroradiol. (2016) 37:885–91. doi: 10.3174/ajnr.A4625
45. Mattingly TK, Denning LM, Siroen KL, Lehrbass B, Lopez-Ojeda P, Stitt L, et al. Catheter based selective hypothermia reduces stroke volume during focal cerebral ischemia in swine. J Neurointerv Surg. (2016) 8:418–22. doi: 10.1136/neurintsurg-2014-011562
46. Fazel Bakhsheshi M, Wang Y, Keenliside L, Lee TY. A new approach to selective brain cooling by a ranque-hilsch vortex tube. Intensive Care Med Exp. (2016) 4:32. doi: 10.1186/s40635-016-0102-5
47. King R MJ, Brooks O, et al. Focal cooling through a novel insulated catheter allows fast and safe therapeutic hypothermia in a canine ischemic stroke model. J Neurointerv Surg. (2018) 10(Suppl 2):A117 LP-A118. doi: 10.1136/neurintsurg-2018-SNIS.216
48. Abou-Chebl A, Sung G, Barbut D, Torbey M. Local brain temperature reduction through intranasal cooling with the rhinochill device: preliminary safety data in brain-injured patients. Stroke. (2011) 42:2164–9. doi: 10.1161/STROKEAHA.110.613000
49. Chava R, Zviman M, Raghavan MS, Halperin H, Maqbool F, Geocadin R, et al. Rapid induction of therapeutic hypothermia using transnasal high flow dry air. Ther Hypothermia Temp Manag. (2017) 7:50–6. doi: 10.1089/ther.2016.0016
50. Sedlacik J, Kjorstad A, Nagy Z, Buhk JH, Behem CR, Trepte CJ, et al. Feasibility study of a novel high-flow cold air cooling protocol of the porcine brain using MRI temperature mapping. Ther Hypothermia Temp Manag. (2018) 8:45–52. doi: 10.1089/ther.2017.0031
51. Westermaier T, Nickl R, Koehler S, Fricke P, Stetter C, Rueckriegel SM, et al. Selective brain cooling after traumatic brain injury: effects of three different cooling methods-case report. J Neurol Surg A Cent Eur Neurosurg. (2017) 78:397–402. doi: 10.1055/s-0036-1596057
52. Chen Y, Quddusi A, Harrison KA, Ryan PE, Cook DJ. Selection of preclinical models to evaluate intranasal brain cooling for acute ischemic stroke. Brain Circ. (2019) 5:160–8. doi: 10.4103/bc.bc_20_19
53. Chen J, Liu L, Zhang H, Geng X, Jiao L, Li G, et al. Endovascular hypothermia in acute ischemic stroke: pilot study of selective intra-arterial cold saline infusion. Stroke. (2016) 47:1933–5. doi: 10.1161/STROKEAHA.116.012727
54. Wu C, Zhao W, An H, Wu L, Chen J, Hussain M, et al. Safety, feasibility, and potential efficacy of intraarterial selective cooling infusion for stroke patients treated with mechanical thrombectomy. J Cereb Blood Flow Metab. (2018) 38:2251–60. doi: 10.1177/0271678X18790139
55. Poli S, Purrucker J, Priglinger M, Ebner M, Sykora M, Diedler J, et al. Rapid induction of cooling in stroke patients (Icool1): a randomised pilot study comparing cold infusions with nasopharyngeal cooling. Crit Care. (2014) 18:582. doi: 10.1186/s13054-014-0582-1
56. Ferreira RES, de Paiva BLC, de Freitas FGR, Machado FR, Silva GS, Raposo RM, et al. Efficacy and safety of a nasopharyngeal catheter for selective brain cooling in patients with traumatic brain injury: a prospective, non-randomized pilot study. Neurocrit Care. (2021) 34:581–92. doi: 10.1007/s12028-020-01052-9
57. Martin S. Therapeutic hypothermia and acute brain injury. Anaesth Intensive Care [Preprint]. (2016) doi: 10.1016/j.mpaic.2016.09.014 (accessed April 21, 2022).
58. Rumana CS, Gopinath SP, Uzura M, Valadka AB, Robertson CS. Brain temperature exceeds systemic temperature in head-injured patients. Crit Care Med. (1998) 26:562–7. doi: 10.1097/00003246-199803000-00032
59. Neimark MA, Konstas AA, Lee L, Laine AF, Pile-Spellman J, Choi J. Brain temperature changes during selective cooling with endovascular intracarotid cold saline infusion: simulation using human data fitted with an integrated mathematical model. J Neurointerv Surg. (2013) 5:165–71. doi: 10.1136/neurintsurg-2011-010150
60. Duan Y, Wu D, Huber M, Shi J, An H, Wei W, et al. New endovascular approach for hypothermia with intrajugular cooling and neuroprotective effect in ischemic stroke. Stroke. (2020) 51:628–36. doi: 10.1161/STROKEAHA.119.026523
61. Tokairin K, Osanai T, Abumiya T, Kazumata K, Ono K, Houkin K. Regional transarterial hypothermic infusion in combination with endovascular thrombectomy in acute ischaemic stroke with cerebral main arterial occlusion: protocol to investigate safety of the clinical trial. BMJ Open. (2017) 7:e016502. doi: 10.1136/bmjopen-2017-016502
62. Boller M, Lampe JW, Katz JM, Barbut D, Becker LB. Feasibility of intra-arrest hypothermia induction: a novel nasopharyngeal approach achieves preferential brain cooling. Resuscitation. (2010) 81:1025–30. doi: 10.1016/j.resuscitation.2010.04.005
63. Busch HJ, Eichwede F, Fodisch M, Taccone FS, Wobker G, Schwab T, et al. Safety and feasibility of nasopharyngeal evaporative cooling in the emergency department setting in survivors of cardiac arrest. Resuscitation. (2010) 81:943–9. doi: 10.1016/j.resuscitation.2010.04.027
64. Zhu XL, Xu WX, Ding WG, Sheng HF, Liu J, Hu Y, et al. Analysis of correlative factors of spinal cord posterior displacement after single door laminoplasty. Zhongguo Gu Shang. (2018) 31:1114–8. doi: 10.3969/j.issn.1003-0034.2018.12.008
65. Zhang Z, Zhang L, Ding Y, Han Z, Ji X. Effects of therapeutic hypothermia combined with other neuroprotective strategies on ischemic stroke: review of evidence. Aging Dis. (2018) 9:507–22. doi: 10.14336/AD.2017.0628
66. Barks JD, Liu YQ, Shangguan Y, Silverstein FS. Phenobarbital augments hypothermic neuroprotection. Pediatr Res. (2010) 67:532–7. doi: 10.1203/PDR.0b013e3181d4ff4d
67. Liu Y, Barks JD, Xu G, Silverstein FS. Topiramate extends the therapeutic window for hypothermia-mediated neuroprotection after stroke in neonatal rats. Stroke. (2004) 35:1460–5. doi: 10.1161/01.STR.0000128029.50221.fa
68. Fan X, van Bel F, van der Kooij MA, Heijnen CJ, Groenendaal F. Hypothermia and erythropoietin for neuroprotection after neonatal brain damage. Pediatr Res. (2013) 73:18–23. doi: 10.1038/pr.2012.139
69. Martin JL, Ma D, Hossain M, Xu J, Sanders RD, Franks NP, et al. Asynchronous administration of xenon and hypothermia significantly reduces brain infarction in the neonatal rat. Br J Anaesth. (2007) 98:236–40. doi: 10.1093/bja/ael340
70. Wu D, Shi J, Elmadhoun O, Duan Y, An H, Zhang J, et al. Dihydrocapsaicin (Dhc) enhances the hypothermia-induced neuroprotection following ischemic stroke via Pi3k/Akt regulation in rat. Brain Res. (2017) 1671:18–25. doi: 10.1016/j.brainres.2017.06.029
71. Wu D, Zhi X, Duan Y, Zhang M, An H, Wei W, et al. Inflammatory cytokines are involved in dihydrocapsaicin (Dhc) and regional cooling infusion (Rci)-induced neuroprotection in ischemic rat. Brain Res. (2019) 1710:173–80. doi: 10.1016/j.brainres.2018.12.033
72. Byun JC, Lee SR, Kim CS. Effects of carnosine and hypothermia combination therapy on hypoxic-ischemic brain injury in neonatal rats. Clin Exp Pediatr. (2021) 64:422–8. doi: 10.3345/cep.2020.01837
Keywords: acute ischemic stroke (AIS), selective hypothermia, rodents, primates, clinical translation
Citation: Wang X, Wehbe A, Kaura S, Chaudhry N, Geng X and Ding Y (2022) Updates on Selective Brain Hypothermia: Studies From Bench Work to Clinical Trials. Front. Neurol. 13:899547. doi: 10.3389/fneur.2022.899547
Received: 18 March 2022; Accepted: 13 April 2022;
Published: 06 May 2022.
Edited by:
Jae H. Choi, Neurovascular Center, P.C., United StatesReviewed by:
Jeanne Teitelbaum, McGill University, CanadaAnatol Manaenko, Innsbruck Medical University, Austria
Copyright © 2022 Wang, Wehbe, Kaura, Chaudhry, Geng and Ding. This is an open-access article distributed under the terms of the Creative Commons Attribution License (CC BY). The use, distribution or reproduction in other forums is permitted, provided the original author(s) and the copyright owner(s) are credited and that the original publication in this journal is cited, in accordance with accepted academic practice. No use, distribution or reproduction is permitted which does not comply with these terms.
*Correspondence: Xiaokun Geng, xgeng@ccmu.edu.cn; Yuchuan Ding, yding@med.wayne.edu