- 1Department of Neurology, Manchester Centre for Clinical Neurosciences, Salford Royal NHS Foundation Trust, Salford, United Kingdom
- 2Division of Neuroscience and Experimental Psychology, School of Biological Sciences, University of Manchester, Manchester, United Kingdom
- 3Department of Neuropsychology, Manchester Centre for Clinical Neurosciences, Salford Royal NHS Foundation Trust, Salford, United Kingdom
- 4Department of Neuroradiology, Manchester Centre for Clinical Neurosciences, Salford Royal NHS Foundation Trust, Salford, United Kingdom
This mini-review focuses on cognitive impairment in iNPH. This symptom is one of the characteristic triad of symptoms in a condition long considered to be the only treatable dementia. We present an update on recent developments in clinical, neuropsychological, neuroimaging and biomarker aspects. Significant advances in our understanding have been made, notably regarding biomarkers, but iNPH remains a difficult diagnosis. Stronger evidence for permanent surgical treatment is emerging but selection for treatment remains challenging, particularly with regards to cognitive presentations. Encouragingly, there has been increasing interest in iNPH, but more research is required to better define the underlying pathology and delineate it from overlapping conditions, in order to inform best practise for the clinician managing the cognitively impaired patient. In the meantime, we strongly encourage a multidisciplinary approach and a structured service pathway to maximise patient benefit.
Introduction
Idiopathic Normal Pressure Hydrocephalus (iNPH) is a clinical syndrome (1) derived by analogy from NPH but in the absence of a preceding insult (2). iNPH is characterised by the clinical trial of progressive and prominent decline in mobility, followed by less prominent but equally progressive cognitive impairment and bladder disturbance. When supported by ventriculomegaly on brain imaging, the diagnosis should be straightforward. In clinical practise, however, patients suspected of having iNPH frequently present significant cognitive impairment, often preceding or starting at the same time as the mobility disorder. This review focusses on such patients emphasising the clinical, imaging and neuropsychological differential diagnosis, highlighting gaps in our understanding, describing recent developments and making suggestions for future research.
Clinical
Cognitive impairment in iNPH is not universal but is frequently present. Insidious onset and more prevalent causes of cognitive impairment, such as vascular dementia (VaD) and Alzheimer's disease (AD), make early diagnosis of iNPH challenging. Significant cognitive impairment in the absence of an early, prominent and typical decline in mobility mandates consideration of other underlying causes given their impact on prognosis and therapeutic decision making.
The typical cognitive profile of iNPH is “subcortical” with impaired attention, reduced psychomotor speed and inefficient memory (3).
VaD results in a similar profile, making these two entities difficult to separate on clinical grounds (4). Cerebrovascular disease (CVD) is frequently present on routine MRI scanning but unless severe its significance is often uncertain. Furthermore, neuroimaging findings between iNPH and CVD overlap.
The commonest cognitive profile of AD, that of progressive amnesia (5), may not be detectable early on. Assessment beyond the Mini-Mental State Examination (MMSE) is required and may include cognitive batteries such as the CANTAB (6). Imaging may identify typical patterns of brain volume loss. CSF biomarkers and amyloid positron emission tomography (PET) may demonstrate AD neuropathological change (ADNC) but do not necessarily prove a causal link to the patient's cognitive impairment. Brain biopsies may be obtained at the time of shunt insertion but are therefore only available for those patients who have already been selected for surgery. Our understanding of the role of those biomarkers in the diagnosis of AD has evolved, recognising that they are increasingly prevalent with age and the apoE4 genotype, even in asymptomatic individuals (7). There is an association between CSF biomarkers and outcome (8, 9). While this is not categorical; it seems most robust for Amyloid-β (Aβ)-42 (10). To complicate matters, clinico-pathological relationships in vascular cognitive impairment (VCI) (11) and AD (12) are variable, co-pathology is common, and their interaction on the phenotype remains poorly understood (13).
Typical presentations of Lewy body disease (LBD) (14), progressive supranuclear palsy (PSP) (15) and corticobasal degeneration (CBD) (16) should not pose differential diagnostic difficulties. However, their combination of physical and cognitive decline may be phenotypically similar to iNPH. In their early stages, hallmark clinical features may not have emerged yet and structural brain imaging can be equivocal. Therefore iNPH has to be considered in the differential diagnosis, but co-pathology and mimicry should not be excluded. In this scenario biomarker findings of neurodegenerative disease are either evidence of pathology or co-pathology. If the index of suspicion for iNPH is high, such as after positive tap test, then shunting should be considered. In the shunt responsive iNPH patient neurodegenerative co-pathology seems to modify the clinical phenotype (17). Abnormal DaT imaging is proof of impaired dopaminergic function in the basal ganglia but is aetiologically non-specific and has also been described in iNPH (18, 19). CSF tau species in PSP and CBD have yielded conflicting results (20), CSF RT-QuIC of alpha-synuclein in LBD and tau in PSP and CBD (21) may be more promising.
Little has been published on frontotemporal dementia (FTD) and iNPH. FTD should not cause differential diagnostic problems as difficulties with mobility occur late in the course of the disease. If present early, and typical for iNPH, co-pathology should be suspected. A case report of a C9orf 72 positive patient, with co-occurrence of typical features of FTD and iNPH, described post-shunt improvements in gait and executive tests, while the behavioural disorder remained unaffected (22).
The differential diagnostic assessment of patients suspected of having iNPH, who have early and/or significant cognitive impairment, requires clinical expertise in cognitive and atypical movement disorders to delineate the presenting symptoms and signs. The assessment should conclude with a probabilistic diagnostic statement attributing the findings either to a single (atypical) morbidity or postulating co-morbidity. Neuroimaging, neuropsychology and CSF biomarkers all provide important diagnostic information, emphasising that the best approach to managing patients suspected of having iNPH with significant cognitive impairment is the protocol-driven multidisciplinary team assessment (23).
The existing evidence of cognitive outcomes after shunting has recently been systematically reviewed. Improvement was found in 61% of patients (24). The authors acknowledge several limitations, including a lack of uniform and standardised cognitive outcome measures, and rated the evidence as low to medium.
Probable iNPH patients who also have clinical features or biomarker evidence of CVD (25), AD (26), or LBD (17), if carefully selected for shunt surgery in a tertiary, multidisciplinary setting, have a good chance of improvement, in their gait disorder. They may also experience partial and temporary improvement of their cognitive impairment.
Long-term outcome studies of treated iNPH patients suggest that the numbers developing dementia are significantly greater than in the general population (27, 28). A longitudinal cohort study applying disease modelling to shunted iNPH patients found an overrepresentation of AD compared to the general population after a medium follow up of 5.3 years. Significant predictive factors were cortical biopsy, medial temporal atrophy on MRI and clinical symptoms (29).
Neither aetiology nor pathogenesis of iNPH are well understood (30). The potential role of a loss-of-function variant in CFAP43 (recently described in a Japanese kindred of familial iNPH and confirmed by a knocked out mouse model) in the aetiology of “sporadic” iNPH is currently uncertain (31). Post mortem studies do not go beyond case series and “definite” iNPH, pathological findings remain non-specific (32, 33). There is controversy over whether pathological findings of AD, CVD, LBD and PSP, represent co-morbidity (17), wrong diagnosis (34–36) or even subtype (26). Impaired glymphatic function has been found in AD and iNPH [for a review see (37)] which is probably mediated by aquaporin channels and represents the putative underlying pathophysiology of hydrocephalus and its compensation mechanisms [for a summary see (38)].
Experience from AD, may serve as a model for future research (see Table 1): cooperation between basic science and clinical researchers studying deeply phenotyped, multi-modality assessed and post mortem verified patients has led to increased understanding of aetiology and pathogenesis. These efforts have defined pathological hallmarks and resulted in the development of disease biomarkers. Amyloid PET and CSF amyloid and tau are now available clinically. They have revolutionised clinical treatment trials (39) and are used to screen for ADNC in patients suspected of having iNPH but their role needs to be further clarified. For iNPH, a better understanding of aetiology and pathogenesis, definition of pathological hallmarks and discovery of iNPH specific biomarkers would transform the field. Yet clinical treatment trials in Alzheimer's disease illustrate the challenges of RCT designs in a cognitive disorder. Using multiple modalities and complex sets of cognitive and social outcome measures, they remain in search of the best combination for providing high sensitivity and specificity to reliably demonstrate cognitive change over short time frames (40, 41).
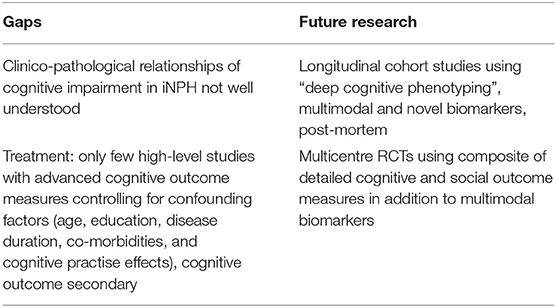
Table 1. The pathological basis of cognitive impairment in iNPH and the treatment response to shunting of cognitive impairment in iNPH requires further study.
Imaging
Since the delineation of iNPH as a clinical syndrome, imaging has been needed to demonstrate ventriculomegaly and help evaluate differential diagnoses, including alternative or co-existing causes for cognitive impairment (42). Here, we review the role of imaging in assessing the cognitive aspects of iNPH, focussing on the more recent developments in the evaluation of brain/CSF morphology, diffusion tensor imaging, resting state functional MRI, amyloid PET, and imaging targeting glymphatic clearance.
Morphology: DESH
In the context of iNPH a widely studied pattern of CSF space morphology is the combination of (i) hydrocephalus (Evans index ≥0.3), (ii) high-convexity/midline tightness and (iii) Sylvian fissure enlargement. This “disproportionately enlarged subarachnoid-space hydrocephalus” (DESH) (43, 44) is found in many but not all (45) cases of iNPH, and as a potential marker of response to shunt surgery (44, 46, 47), some centres have incorporated it into management pathways (30). The pathophysiological mechanisms underlying DESH are unclear but it is likely to be associated with disrupted CSF dynamics (48).
A number of recent studies have now investigated the presence of DESH in the wider population, where it is found in 1–7% and associated with poorer cognition (49–52). A study examining over a thousand participants with either no or only mild cognitive impairment found that DESH was a predictor of progressive cognitive decline independent of established features including age, cortical thickness, or APOE status (48). There is also evidence that in some cases DESH may be a marker of preclinical iNPH: a long term follow up study of asymptomatic individuals with DESH imaging features found that approximately 17% per year subsequently progressed to symptomatic iNPH (53).
Structural Connectivity: DTI
Diffusion tensor imaging (DTI) is an MRI technique which measures orientation-specific water diffusivity to interrogate brain micro-structure and characterise white matter tracts. White matter injury and dysfunction are proposed components of iNPH pathogenesis and DTI has demonstrated differences in white matter when compared to healthy controls, particularly the corticospinal tract (54) and corpus callosum (55). In several recent studies measures of cognitive impairment in iNPH have been correlated with abnormalities in specific neuroanatomical regions of interest: the forceps minor (56), frontal subcortical white matter (57), right cingulum-hippocampus (58), internal capsules and centrum semiovale (59); these findings are suggestive of the circuits involved in cognitive impairment although study samples are relatively small and patient populations are at risk of other causes of dementia such as AD and VaD. Interestingly, there is evidence that DTI white matter abnormalities can respond to shunt surgery (55, 60) and are potentially partially reversible. Most investigations in iNPH patients have relied on conventional DTI but a few have applied more advanced techniques to further probe tissue microstructure, including kurtosis DTI (57), q-space imaging (61) and neurite orientation dispersion and density imaging (60). There are known technical challenges with comparing DTI datasets between different scanners, however recent experience has confirmed that repeatability and cross-scanner comparability is possible across differing sites (62), allowing future multicentre longitudinal trials.
Functional Connectivity
Whilst DTI provides measures of structural brain connectivity, MRI techniques are also able to probe functional connectivity. Resting state functional MRI (rsfMRI) examines correlations in brain activity, identifying sets of brain regions that activate simultaneously in the absence of a specific cognitive task. One such set, known as the default mode network (DMN) (63), has been widely studied and changes in DMN connectivity have been associated with cognitive dysfunction across a range of different pathologies (64–66). Altered DMN connectivity has been found in iNPH patients where it is associated with executive dysfunction (67, 68) and poorer cognitive outcomes after shunt placement (68).
Moreover, further studies suggest that the dysfunction seen in iNPH may involve multiple networks in addition to the DMN (69, 70) and can partially normalise after a CSF tap test (70).
Glymphatic Imaging
There has been increasing interest in imaging targeting the “glymphatic system”: the glia-lymphatic structures which allow the interchange between cerebrospinal fluid and the interstitial space (71–73); this interchange is critical in maintaining interstitial homeostasis and glymphatic dysfunction has been implicated in a range of neurological diseases (74). Multiple in vivo MR imaging techniques have been explored (75), particularly those which directly follow the transport of gadolinium based contrast agents (GBCA) after intrathecal administration (76–79). A number of other pilot studies have used MR techniques which do not require an exogeneous tracer: intravoxel incoherent motion MRI (80), DTI (81), chemical exchange saturation transfer imaging (82) and visualisation of lymphatic channels (83).
When applied in iNPH patients, intrathecal GBCA studies have demonstrated differences in CSF redistribution of tracer compared to controls, with significantly more ventricular reflux (77–79)–a finding consistent with previous radionuclide cisternographic studies (84). Interestingly, there also appears to be delayed clearance of GBCA within brain parenchyma (77, 79), including the entorhinal cortex of the mesial temporal lobe (85). It must be noted, however, that the control populations for the above studies were significantly younger than the iNPH group, and increasing age is known to be associated reduced glymphatic function in animal studies (86). Moreover, in the control population the rate of clearance appears to vary widely (77). Further investigation will be required to confirm these findings. The challenges associated with these techniques are well known (75, 87) and, although none are currently suitable for clinical implementation, this is an area of active development.
Amyloid PET Imaging
Recent work has highlighted the potential of PET imaging for the in vivo assessment of amyloid deposition. Amyloid-β (Aβ) is the main component of the plaques found in AD, a frequent co-morbidity in iNPH patients that contributes to cognitive decline (42, 88, 89). Cortical biopsies in iNPH patients frequently detect Aβ, a finding which confers a tenfold increase in the risk of subsequent Alzheimer's disease (90). The PET radiopharmaceutical [11C] Pittsburgh Compound B and newer [18F]-labelled tracers (flutemetamol, florbetapir, florbetaben) now offer the ability to identify Aβ non-invasively (91–94). Multiple studies have demonstrated strong concordance between histopathology for Aβ and amyloid PET imaging in iNPH patients (91, 95–98), offering a new window on the assessment of this significant pathology.
Neuropsychology
Individuals with iNPH perform significantly worse than controls on various cognitive measures (6, 99–101). Poorer baseline cognitive status is associated with older age, longer disease duration, worse motor performance (100), and increased mortality after shunt surgery (28). However, variable cognitive patterns have been reported in the literature. Many studies demonstrate early executive dysfunction and psychomotor slowing (102), followed by more widespread cognitive decline at later stages (103). Yet others report early and diffuse cognitive changes, including visuospatial dysfunction and memory impairments (99).
Some studies report post-tap test improvements using either cognitive screens (104) or more comprehensive neuropsychological testing (101). Benefits to cognition have also been reported 3, 12 months (105, 106), and 1–3 years after shunt surgery (107). One meta-analysis (108) reported robust improvements in memory and executive function after shunt surgery. However, post-treatment cognitive outcomes can be variable, and their relationship to other iNPH symptoms remains unclear. Bugalho et al. (99) found no relationship between cognition and gait. Yasar et al. (109) found no improvement in cognitive status after shunt surgery, but an improvement in balance and gait; and Grasso et al. (107) found cognition was not maintained alongside gait improvements at 10 year follow up.
Cognitive screens, such as the MMSE (110), are commonly used in assessment, but may be inadequate for differentiating iNPH from other neurodegenerative disorders (3, 6). Furthermore, practice effects are an important consideration when quantifying true change in cognitive performance across serial assessments (111). Significant practice effects are seen in healthy participants (112) and in post-surgical patients (113) within the first 3 months of serial testing, but were not evident in a sample of iNPH patients over 4 consecutive days (114). It is likely that practice effects differ based on a variety of factors, including age, disease status, test selection, and test-retest interval (111). Interestingly, Duff (111) suggests that practise effects themselves may be important predictors of future cognitive status and treatment outcomes.
Even studies that control for practice effects show variable outcomes. Kambara et al. (115) showed that MMSE scores improved at 3 and 6 months post-surgery, but declined in association with age and poorer scores on an iNPH grading scale. In a large, well-designed study, Solana et al. (116) reported improvements in all cognitive domains 6 months after shunt surgery in group analysis, but only 50% of their participants showed significant improvements on individual analysis.
Another reason for variability in cognitive outcomes may be the presence of alternative or co-morbid neurodegenerative diseases. In one study with a median follow up of 4.8 years, 80% of a shunt responsive group demonstrated cognitive decline, and 46% met the criteria for dementia, with the most common diagnoses being AD and VaD (117). The best predictor of dementia was having memory problems as the first symptom (117). Detailed neuropsychological testing comparing iNPH and Parkinson's disease (PD), in their first year of symptom onset, found more frequent (65% iNPH vs. 25.5% PD) and diffuse cognitive deficits in iNPH (118). Laidet et al. (119) reported that iNPH “mimics” – including PD, atypical PD, VaD, and FTD – failed to demonstrate cognitive improvements after CSF tap test, and that verbal fluency scores distinguished iNPH from this mixed-diagnosis group. Similarly, Liouta et al. (120) used comprehensive neuropsychological tests to show that an iNPH group demonstrated post-tap test and post-shunting cognitive improvements (86 and 97%, respectively), while none of a group including VaD, atypical PD, and FTD showed improvements.
In studies examining neurodegenerative biomarkers, the results are also mixed. A higher incidence of AD biomarkers has been reported in iNPH compared with controls, and was associated with cognitive decline at 2 years (121). While individuals with pathological levels of biomarkers on CSF analysis may show cognitive improvements after tap test (26) and shunt surgery (122, 123), others show less improvements in cognition (122–124). Nerg (6) examined biopsy-acquired AD biomarkers alongside cognition, and found poorer verbal fluency and clock drawing in iNPH, and worse word list learning and picture naming in AD, but little relationship between AD biomarkers and cognitive results.
Specific neuropsychological markers can aid in distinguishing iNPH from other neurodegenerative disorders (6, 117, 125–127), but a discussion of their relative merits is beyond the scope of this review. Therefore, detailed cognitive analysis by a trained neuropsychologist is essential.
Summary of Neuropsychology
Cognitive impairments in iNPH typically involve executive dysfunction, but may be accompanied by more widespread deficits. Poorer cognition is associated with older age, longer disease duration, co-morbidity, variable outcomes after shunt surgery, and increased mortality.
Variable outcomes may be due to inadequate control for confounding factors, inadequate cognitive measures, or whole-group analyses which average-out individual variability (see Table 1). Where significant cognitive improvements are reported, effect sizes tend to be small, and hence their clinical relevance to individual patients remains uncertain.
Robust neuropsychological methods that control for practice effects in serial testing are needed (128). Detailed cognitive analysis by a trained neuropsychologist is a crucial part of a wider multidisciplinary consensus diagnosis.
Discussion
We have presented evidence to inform patient management for practitioners confronted with cognitively impaired patients in whom a suspicion of iNPH has been raised. Recent developments have helped to improve differential diagnosis and patient selection for treatment. Neuropsychological differential diagnosis, advanced imaging, and CSF biomarkers are powerful tools starting to enter mainstream clinical use. We encourage active management of these patients through the optimal use of these tools within a structured clinical service. Hence the complex needs of patients with iNPH are best met within a multidisciplinary team. The nosology requires further clarification in prospective cohort studies in cooperation with basic science. An iNPH specific biomarker would revolutionise the field. However, agreement needs to be reached on standardised assessment methods and outcome measures of gait and cognition, where advanced neuropsychological batteries may serve to stratify clinical populations by cognitive features in future RCTs.
Author Contributions
All authors listed have made a substantial, direct, and intellectual contribution to the work and approved it for publication.
Conflict of Interest
The authors declare that the research was conducted in the absence of any commercial or financial relationships that could be construed as a potential conflict of interest.
Publisher's Note
All claims expressed in this article are solely those of the authors and do not necessarily represent those of their affiliated organizations, or those of the publisher, the editors and the reviewers. Any product that may be evaluated in this article, or claim that may be made by its manufacturer, is not guaranteed or endorsed by the publisher.
Acknowledgments
We are grateful to the Northern Care Alliance for enabling the NPH pathway at our institution. We would also like to thank Calvin Heal from Research and Innovation at the Northern Care Alliance, for his statistical expertise.
References
2. Adams RD, Fisher CM, Hakim S, Ojemann RG, Sweet WH. Symptomatic Occult Hydrocephalus with “Normal” cerebrospinal-fluid PressureA treatable syndrome. N Engl J Med. (1965) 273:117–26. doi: 10.1056/NEJM196507152730301
3. Picascia M, Zangaglia R, Bernini S, Minafra B, Sinforiani E, Pacchetti C, et al. Review of cognitive impairment and differential diagnosis in idiopathic normal pressure hydrocephalus. Funct Neurol. (2015) 30:217–28. doi: 10.11138/FNeur/2015.30.4.217
4. Hellstrom P, Edsbagge M, Archer T, Tisell M, Tullberg M, Wikkelso C. The Neuropsychology of patients with clinically diagnosed idiopathic normal pressure hydrocephalus. Neurosurgery. (2007) 61:1219–26. doi: 10.1227/01.neu.0000306100.83882.81
5. Dubois B, Feldman HH, Jacova C, Hampel H, Molinuevo JL, Blennow K, et al. Advancing research diagnostic criteria for alzheimer's disease: The Iwg-2 criteria. Lancet Neurol. (2014) 13:614–29. doi: 10.1016/S1474-4422(14)70090-0
6. Nerg O, Junkkari A, Hallikainen I, Rauramaa T, Luikku A, Hiltunen M, et al. The cerad neuropsychological battery in patients with idiopathic normal pressure hydrocephalus compared with normal population and patients with mild Alzheimer's disease. J Alzheimer's Disease: JAD. (2021) 1117–30. doi: 10.3233/JAD-201363
7. Jansen WJ, Janssen O, Tijms BM, Vos SJB, Ossenkoppele R, Visser PJ, et al. Prevalence estimates of amyloid abnormality across the alzheimer disease clinical spectrum. JAMA Neurol. (2022) 79:228–43. doi: 10.1001/jamaneurol.2021.5216
8. Braun M, Bjurnemark C, Seo W, Freyhult E, Nyholm D, Niemela V, et al. Higher levels of neurofilament light chain and total tau in csf are associated with negative outcome after shunt surgery in patients with normal pressure hydrocephalus. Fluids Barriers CNS. (2022) 19:15. doi: 10.1186/s12987-022-00306-2
9. Lukkarinen H, Jeppsson A, Wikkelso C, Blennow K, Zetterberg H, Constantinescu R, et al. Cerebrospinal fluid biomarkers that reflect clinical symptoms in idiopathic normal pressure hydrocephalus patients. Fluids Barriers CNS. (2022) 19:11. doi: 10.1186/s12987-022-00309-z
10. Lukkarinen H, Tesseur I, Pemberton D, Van Der Ark P, Timmers M, Slemmon R, et al. Time trends of cerebrospinal fluid biomarkers of neurodegeneration in idiopathic normal pressure hydrocephalus. Journal of Alzheimer's disease: JAD. (2021) 80:1629–42. doi: 10.3233/JAD-201361
11. Skrobot OA, Attems J, Esiri M, Hortobagyi T, Ironside JW, Kalaria RN, et al. Vascular cognitive impairment neuropathology guidelines (Vcing): the contribution of cerebrovascular pathology to cognitive impairment. Brain. (2016) 139:2957–69. doi: 10.1093/brain/aww214
12. Hyman BT, Phelps CH, Beach TG, Bigio EH, Cairns NJ, Carrillo MC, et al. National institute on aging-Alzheimer's association guidelines for the neuropathologic assessment of Alzheimer's disease. Alzheimers Dement. (2012) 8:1–13. doi: 10.1016/j.jalz.2011.10.007
13. Robinson AC, Roncaroli F, Chew-Graham S, Davidson YS, Minshull J, Horan MA, et al. The contribution of vascular pathology toward cognitive impairment in older individuals with intermediate braak stage tau pathology. J Alzheimers Dis. (2020) 77:1005–15. doi: 10.3233/JAD-200339
14. McKeith IG, Boeve BF, Dickson DW, Halliday G, Taylor JP, Weintraub D, et al. Diagnosis and management of dementia with lewy bodies: fourth consensus report of the Dlb consortium. Neurology. (2017) 89:88–100. doi: 10.1212/WNL.0000000000004058
15. Hoglinger GU, Respondek G, Stamelou M, Kurz C, Josephs KA, Lang AE, et al. Clinical diagnosis of progressive supranuclear palsy: the movement disorder society criteria. Mov Disord. (2017) 32:853–64. doi: 10.1002/mds.26987
16. Armstrong MJ, Litvan I, Lang AE, Bak TH, Bhatia KP, Borroni B, et al. Criteria for the diagnosis of corticobasal degeneration. Neurology. (2013) 80:496–503. doi: 10.1212/WNL.0b013e31827f0fd1
17. Sakurai A, Tsunemi T, Ishiguro Y, Okuzumi A, Hatano T, Hattori N. Comorbid alpha synucleinopathies in idiopathic normal pressure hydrocephalus. J Neurol. (2022) 269:2022–9. doi: 10.1007/s00415-021-10778-1
18. Lee JY, Park SB, Lee M, Ju H, Im K, Kwon KY. Detailed visual assessment of striatal dopaminergic depletion in patients with idiopathic normal pressure hydrocephalus: unremarkable or not? BMC Neurol. (2020) 20:277. doi: 10.1186/s12883-020-01861-7
19. Todisco M, Zangaglia R, Minafra B, Pisano P, Trifirò G, Bossert I, et al. Clinical outcome and striatal dopaminergic function after shunt surgery in patients with idiopathic normal pressure hydrocephalus. Neurology. (2021) 96:e2861–e73. doi: 10.1212/WNL.0000000000012064
20. Oeckl P, Steinacker P, Feneberg E, Otto M. Neurochemical biomarkers in the diagnosis of frontotemporal lobar degeneration: an update. J Neurochem. (2016) 138:184–92. doi: 10.1111/jnc.13669
21. Standke HG, Kraus A. Seed amplification and Rt-Quic assays to investigate protein seed structures and strains. Cell Tissue Res. (2022). doi: 10.1007/s00441-022-03595-z. [Epub ahead of print].
22. Korhonen VE, Solje E, Suhonen NM, Rauramaa T, Vanninen R, Remes AM, et al. Frontotemporal dementia as a comorbidity to idiopathic normal pressure hydrocephalus (Inph): a short review of literature and an unusual case. Fluids Barriers CNS. (2017) 14:10. doi: 10.1186/s12987-017-0060-7
23. Junkkari A, Luikku AJ, Danner N, Jyrkkänen HK, Rauramaa T, Korhonen VE, et al. The kuopio idiopathic normal pressure hydrocephalus protocol: initial outcome of 175 patients. Fluids and Barriers of the CNS. (2019) 16. doi: 10.1186/s12987-019-0142-9
24. Giordan E, Palandri G, Lanzino G, Murad MH, Elder BD. Outcomes and complications of different surgical treatments for idiopathic normal pressure hydrocephalus: a systematic review and meta-analysis. J Neurosurg. (2018) 131:1–13. doi: 10.3171/2018.5.JNS1875
25. Tullberg M, Jensen C, Ekholm S, Wikkelso C. Normal pressure hydrocephalus: vascular white matter changes on mr images must not exclude patients from shunt surgery. AJNR Am J Neuroradiol. (2001) 22:1665–73.
26. Müller-Schmitz K, Krasavina-Loka N, Yardimci T, Lipka T, Kolman AGJ, Robbers S, et al. Normal pressure hydrocephalus associated with Alzheimer's disease. Ann Neurol. (2020) 88:703–11. doi: 10.1002/ana.25847
27. Jaraj D, Wikkelsø C, Rabiei K, Marlow T, Jensen C, Östling S, et al. Mortality and risk of dementia in normal-pressure hydrocephalus: a population study. Alzheimers Dement. (2017) 13:850–7. doi: 10.1016/j.jalz.2017.01.013
28. Andren K, Wikkelso C, Sundstrom N, Israelsson H, Agerskov S, Laurell K, et al. Survival in treated idiopathic normal pressure hydrocephalus. J Neurol. (2020) 267:640–8. doi: 10.1007/s00415-019-09598-1
29. Luikku AJ, Hall A, Nerg O, Koivisto AM, Hiltunen M, Helisalmi S, et al. Predicting development of alzheimer's disease in patients with shunted idiopathic normal pressure hydrocephalus. J Alzheimers Dis. (2019) 71:1233–43. doi: 10.3233/JAD-190334
30. Nakajima M, Yamada S, Miyajima M, Ishii K, Kuriyama N, Kazui H, et al. Guidelines for management of idiopathic normal pressure hydrocephalus (Third Edition): endorsed by the Japanese Society of normal pressure hydrocephalus. Neurol Med Chir (Tokyo). (2021) 61:63–97. doi: 10.2176/nmc.st.2020-0292
31. Morimoto Y, Yoshida S, Kinoshita A, Satoh C, Mishima H, Yamaguchi N, et al. Nonsense mutation in Cfap43 causes normal-pressure hydrocephalus with ciliary abnormalities. Neurology. (2019) 92:e2364–e74. doi: 10.1212/WNL.0000000000007505
32. McCarty AM, Jones DT, Dickson DW, Graff-Radford NR. Disproportionately enlarged subarachnoid-space hydrocephalus (Desh) in normal pressure hydrocephalus misinterpreted as atrophy: autopsy and radiological evidence. Neurocase. (2019) 25:151–5. doi: 10.1080/13554794.2019.1617319
33. Espay AJ, Narayan RK, Duker AP. Barrett ET, de Courten-Myers G. Lower-body Parkinsonism: reconsidering the threshold for external lumbar drainage. Nat Clin Pract Neurol. (2008) 4:50–5. doi: 10.1038/ncpneuro0688
34. Magdalinou NK, Ling H, Smith JD, Schott JM, Watkins LD, Lees AJ. Normal pressure hydrocephalus or progressive supranuclear palsy? A clinicopathological case series. J Neurol. (2013) 260:1009–13. doi: 10.1007/s00415-012-6745-6
35. Starr BW, Hagen MC, Espay AJ. Hydrocephalic Parkinsonism: lessons from normal pressure hydrocephalus mimics. J Clin Mov Disord. (2014) 1:2. doi: 10.1186/2054-7072-1-2
36. Espay AJ, Da Prat GA, Dwivedi AK, Rodriguez-Porcel F, Vaughan JE, Rosso M, et al. Deconstructing normal pressure hydrocephalus: ventriculomegaly as early sign of neurodegeneration. Ann Neurol. (2017) 82:503–13. doi: 10.1002/ana.25046
37. Reeves BC, Karimy JK, Kundishora AJ, Mestre H, Cerci HM, Matouk C, et al. Glymphatic system impairment in alzheimer's disease and idiopathic normal pressure hydrocephalus. Trends Mol Med. (2020) 26:285–95. doi: 10.1016/j.molmed.2019.11.008
38. de Laurentis C, Cristaldi P, Arighi A, Cavandoli C, Trezza A, Sganzerla EP, et al. Role of aquaporins in hydrocephalus: what do we know and where do we stand? A systematic review. J Neurol. (2021) 268:4078–94. doi: 10.1007/s00415-020-10122-z
39. Cummings J. The role of biomarkers in Alzheimer's disease drug development. Adv Exp Med Biol. (2019) 1118:29–61. doi: 10.1007/978-3-030-05542-4_2
40. Howlett J, Hill SM, Ritchie CW, Tom BDM. Disease modelling of cognitive outcomes and biomarkers in the european prevention of Alzheimer's dementia longitudinal cohort. Front Big Data. (2021) 4:676168. doi: 10.3389/fdata.2021.676168
41. Koychev I, Lawson J, Chessell T, Mackay C, Gunn R, Sahakian B, et al. Deep and frequent phenotyping study protocol: an observational study in prodromal Alzheimer's disease. BMJ Open. (2019) 9:e024498. doi: 10.1136/bmjopen-2018-024498
42. Malm J, Graff-Radford NR, Ishikawa M, Kristensen B, Leinonen V, Mori E, et al. Influence of comorbidities in idiopathic normal pressure hydrocephalus - research and clinical care. a report of the ishcsf task force on comorbidities in inph fluids. Barriers CNS. (2013) 10:22. doi: 10.1186/2045-8118-10-22
43. Kitagaki H, Mori E, Ishii K, Yamaji S, Hirono N, Imamura T. Csf spaces in idiopathic normal pressure hydrocephalus: morphology and volumetry. AJNR Am J Neuroradiol. (1998) 19:1277–84.
44. Hashimoto M, Ishikawa M, Mori E, Kuwana N. Study of Inph on neurological improvement. Diagnosis of idiopathic normal pressure hydrocephalus is supported by mri-based scheme: a prospective cohort study. Cerebrospinal Fluid Res. (2010) 7:18. doi: 10.1186/1743-8454-7-18
45. Agerskov S, Wallin M, Hellström P, Ziegelitz D, Wikkelsö C, Tullberg M. Absence of disproportionately enlarged subarachnoid space hydrocephalus, a sharp callosal angle, or other morphologic mri markers should not be used to exclude patients with idiopathic normal pressure hydrocephalus from shunt surgery. Am J Neuroradiol. (2019) 40:74–9. doi: 10.3174/ajnr.A5910
46. Narita W, Nishio Y, Baba T, Iizuka O, Ishihara T, Matsuda M, et al. High-convexity tightness predicts the shunt response in idiopathic normal pressure hydrocephalus. Am J Neuroradiol. (2016) 37:1831–7. doi: 10.3174/ajnr.A4838
47. Ahmed AK, Luciano M, Moghekar A, Shin J, Aygun N, Sair HI, et al. Does the presence or absence of desh predict outcomes in adult hydrocephalus? Am J Neuroradiol. (2018) 39:2022–6. doi: 10.3174/ajnr.A5820
48. Cogswell PM, Graff-Radford J, Wurtz LI, Graff-Radford NR, Johnson DR, Hunt CH, et al. Csf dynamics disorders: association of brain mri and nuclear medicine cisternogram findings. Neuroimage Clin. (2020) 28:102481. doi: 10.1016/j.nicl.2020.102481
49. Akiba C, Gyanwali B, Villaraza S, Nakajima M, Miyajima M, Cheng CY, et al. The prevalence and clinical associations of disproportionately enlarged subarachnoid space hydrocephalus (Desh), an imaging feature of idiopathic normal pressure hydrocephalus in community and memory clinic based singaporean cohorts. J Neurol Sci. (2020) 408:116510. doi: 10.1016/j.jns.2019.116510
50. Nishikawa T, Akiguchi I, Satoh M, Hara A, Hirano M, Hosokawa A, et al. The association of disproportionately enlarged subarachnoid space hydrocephalus with cognitive deficit in a general population: the ohasama study. Sci Rep. (2021) 11:17061. doi: 10.1038/s41598-021-95961-0
51. Graff-Radford J, Gunter JL, Jones DT, Przybelski SA, Schwarz CG, Huston J. 3rd, et al. Cerebrospinal fluid dynamics disorders: relationship to alzheimer biomarkers and cognition. Neurology. (2019) 93:e2237–e46. doi: 10.1212/WNL.0000000000008616
52. Engel DC, Pirpamer L, Hofer E, Schmidt R, Brendle C. Incidental findings of typical inph imaging signs in asymptomatic subjects with subclinical cognitive decline. Fluids Barriers CNS. (2021) 18:37. doi: 10.1186/s12987-021-00268-x
53. Kimihira L, Iseki C, Takahashi Y, Sato H, Kato H, Kazui H, et al. A multi-center, prospective study on the progression rate of asymptomatic ventriculomegaly with features of idiopathic normal pressure hydrocephalus on magnetic resonance imaging to idiopathic normal pressure hydrocephalus. J Neurol Sci. (2020) 419:117166. doi: 10.1016/j.jns.2020.117166
54. Hattori T, Yuasa T, Aoki S, Sato R, Sawaura H, Mori T, et al. Altered microstructure in corticospinal tract in idiopathic normal pressure hydrocephalus: comparison with alzheimer disease and parkinson disease with dementia. Am J Neuroradiol. (2011) 32:1681–7. doi: 10.3174/ajnr.A2570
55. Keong NC, Pena A, Price SJ, Czosnyka M, Czosnyka Z, DeVito EE, et al. Diffusion tensor imaging profiles reveal specific neural tract distortion in normal pressure hydrocephalus. PLoS ONE. (2017) 12:e0181624. doi: 10.1371/journal.pone.0181624
56. Grazzini I, Redi F, Sammartano K, Cuneo GL. Diffusion tensor imaging in idiopathic normal pressure hydrocephalus: clinical and csf flowmetry correlations. Neuroradiol J. (2020) 33:66–74. doi: 10.1177/1971400919890098
57. Kamiya K, Kamagata K, Miyajima M, Nakajima M, Hori M, Tsuruta K, et al. Diffusional kurtosis imaging in idiopathic normal pressure hydrocephalus: correlation with severity of cognitive impairment. Magn Reson Med Sci. (2016) 15:316–23. doi: 10.2463/mrms.mp.2015-0093
58. Kang K, Yoon U, Choi W, Lee HW. Diffusion tensor imaging of idiopathic normal-pressure hydrocephalus and the cerebrospinal fluid tap test. J Neurol Sci. (2016) 364:90–6. doi: 10.1016/j.jns.2016.02.067
59. Huang W, Fang X, Li S, Mao R, Ye C, Liu W, et al. Preliminary exploration of the sequence of nerve fiber bundles involvement for idiopathic normal pressure hydrocephalus: a correlation analysis using diffusion tensor imaging. Front Neurosci. (2021) 15:794046. doi: 10.3389/fnins.2021.794046
60. Kamiya K, Hori M, Irie R, Miyajima M, Nakajima M, Kamagata K, et al. Diffusion imaging of reversible and irreversible microstructural changes within the corticospinal tract in idiopathic normal pressure hydrocephalus. Neuroimage Clin. (2017) 14:663–71. doi: 10.1016/j.nicl.2017.03.003
61. Hori M, Kamiya K, Nakanishi A, Fukunaga I, Miyajima M, Nakajima M, et al. Prospective estimation of mean axon diameter and extra-axonal space of the posterior limb of the internal capsule in patients with idiopathic normal pressure hydrocephalus before and after a lumboperitoneal shunt by using q-space diffusion MRI. Eur Radiol. (2016) 26:2992–8. doi: 10.1007/s00330-015-4162-9
62. Zhou X, Sakaie KE, Debbins JP, Narayanan S, Fox RJ, Lowe MJ. Scan-rescan repeatability and cross-scanner comparability of dti metrics in healthy subjects in the sprint-ms multicenter trial. Magn Reson Imaging. (2018) 53:105–11. doi: 10.1016/j.mri.2018.07.011
63. Damoiseaux JS, Rombouts SARB, Barkhof F, Scheltens P, Stam CJ, Smith SM, et al. Consistent resting-state networks across healthy subjects. Proc Natl Acad Sci U S A. (2006) 103:13848–53. doi: 10.1073/pnas.0601417103
64. Bonnelle V, Leech R, Kinnunen KM, Ham TE, Beckmann CF, De Boissezon X, et al. Default mode network connectivity predicts sustained attention deficits after traumatic brain injury. J Neurosci. (2011) 31:13442–51. doi: 10.1523/JNEUROSCI.1163-11.2011
65. Greicius MD, Srivastava G, Reiss AL, Menon V. Default-mode network activity distinguishes alzheimer's disease from healthy aging: evidence from functional MRI. Proc Natl Acad Sci U S A. (2004) 101:4637–42. doi: 10.1073/pnas.0308627101
66. Hawellek DJ, Hipp JF, Lewis CM, Corbetta M, Engel AK. Increased functional connectivity indicates the severity of cognitive impairment in multiple sclerosis. Proc Natl Acad Sci U S A. (2011) 108:19066–71. doi: 10.1073/pnas.1110024108
67. Khoo HM, Kishima H, Tani N, Oshino S, Maruo T, Hosomi K, et al. Default mode network connectivity in patients with idiopathic normal pressure hydrocephalus. J Neurosurg. (2016) 124:350–8. doi: 10.3171/2015.1.JNS141633
68. Kanno S, Ogawa KI, Kikuchi H, Toyoshima M, Abe N, Sato K, et al. Reduced default mode network connectivity relative to white matter integrity is associated with poor cognitive outcomes in patients with idiopathic normal pressure hydrocephalus. BMC Neurol. (2021) 21:353. doi: 10.1186/s12883-021-02389-0
69. Ogata Y, Ozaki A, Ota M, Oka Y, Nishida N, Tabu H, et al. Interhemispheric resting-state functional connectivity predicts severity of idiopathic normal pressure hydrocephalus. Front Neurosci. (2017) 11:470. doi: 10.3389/fnins.2017.00470
70. Griffa A, Bommarito G, Assal F, Herrmann FR, Van De Ville D, Allali G. Dynamic functional networks in idiopathic normal pressure hydrocephalus: alterations and reversibility by Csf tap test. Hum Brain Mapp. (2021) 42:1485–502. doi: 10.1002/hbm.25308
71. Mestre H, Mori Y, Nedergaard M. The brain's glymphatic system: current controversies. Trends Neurosci. (2020) 43:458–66. doi: 10.1016/j.tins.2020.04.003
72. Iliff JJ, Wang M, Liao Y, Plogg BA, Peng W, Gundersen GA, et al. A paravascular pathway facilitates Csf flow through the brain parenchyma and the clearance of interstitial solutes, including amyloid beta. Sci Transl Med. (2012) 4:147ra11. doi: 10.1126/scitranslmed.3003748
73. Klostranec JM, Vucevic D, Bhatia KD, Kortman HGJ, Krings T, Murphy KP, et al. Current concepts in intracranial interstitial fluid transport and the glymphatic system: part i-anatomy and physiology. Radiology. (2021) 301:502–14. doi: 10.1148/radiol.2021202043
74. Plog BA, Nedergaard M. The glymphatic system in central nervous system health and disease: past, present, and future. Annu Rev Pathol. (2018) 13:379–94. doi: 10.1146/annurev-pathol-051217-111018
75. Klostranec JM, Vucevic D, Bhatia KD, Kortman HGJ, Krings T, Murphy KP, et al. Current concepts in intracranial interstitial fluid transport and the glymphatic system: part II-imaging techniques and clinical applications. Radiology. (2021) 301:516–32. doi: 10.1148/radiol.2021204088
76. Watts R, Steinklein JM, Waldman L, Zhou X, Filippi CG. Measuring glymphatic flow in man using quantitative contrast-enhanced MRI. Am J Neuroradiol. (2019) 40:648–51. doi: 10.3174/ajnr.A5931
77. Ringstad G, Valnes LM, Dale AM, Pripp AH, Vatnehol SS, Emblem KE, et al. Brain-wide glymphatic enhancement and clearance in humans assessed with MRI. JCI Insight. (2018) 3:e121537. doi: 10.1172/jci.insight.121537
78. Eide PK, Pripp AH, Ringstad G. Magnetic resonance imaging biomarkers of cerebrospinal fluid tracer dynamics in idiopathic normal pressure hydrocephalus. Brain Commun. (2020) 2:fcaa187. doi: 10.1093/braincomms/fcaa187
79. Geir R, Sirirud VSA, Kristian EP. Glymphatic MRI in idiopathic normal pressure hydrocephalus. Brain. (2017) 140:2691–705. doi: 10.1093/brain/awx191
80. Wong SM, Backes WH, Drenthen GS, Zhang CE, Voorter PHM, Staals J, et al. Spectral diffusion analysis of intravoxel incoherent motion mri in cerebral small vessel disease. J Magn Reson Imaging. (2020) 51:1170–80. doi: 10.1002/jmri.27149
81. Harrison IF, Siow B, Akilo AB, Evans PG, Ismail O, Ohene Y, et al. Non-invasive imaging of csf-mediated brain clearance pathways via assessment of perivascular fluid movement with diffusion tensor MRI. eLife. (2018) 7. doi: 10.7554/eLife.34028
82. Chen Y, Dai Z, Fan R, Mikulis DJ, Qiu J, Shen Z, et al. Glymphatic system visualized by chemical-exchange-saturation-transfer magnetic resonance imaging. ACS Chem Neurosci. (2020) 11:1978–84. doi: 10.1021/acschemneuro.0c00222
83. Ringstad G, Eide PK. Cerebrospinal fluid tracer efflux to parasagittal dura in humans. Nat Commun. (2020) 11:354. doi: 10.1038/s41467-019-14195-x
84. Vanneste J, Augustijn P, Davies GA, Dirven C, Tan WF. Normal-pressure hydrocephalus. Is cisternography still useful in selecting patients for a shunt? Arch Neurol. (1992) 49:366–70. doi: 10.1001/archneur.1992.00530280046021
85. Eide PK, Ringstad G. Delayed clearance of cerebrospinal fluid tracer from entorhinal cortex in idiopathic normal pressure hydrocephalus: a glymphatic magnetic resonance imaging study. J Cereb Blood Flow Metab. (2019) 39:1355–68. doi: 10.1177/0271678X18760974
86. Kress BT, Iliff JJ, Xia M, Wang M, Wei HS, Zeppenfeld D, et al. Impairment of paravascular clearance pathways in the aging brain. Ann Neurol. (2014) 76:845–61. doi: 10.1002/ana.24271
87. Naganawa S, Taoka T. The glymphatic system: a review of the challenges in visualizing its structure and function with mr imaging. Magn Reson Med Sci. (2022) 21:182–94. doi: 10.2463/mrms.rev.2020-0122
88. Golomb J, Wisoff J, Miller DC, Boksay I, Kluger A, Weiner H, et al. Alzheimer's disease comorbidity in normal pressure hydrocephalus: prevalence and shunt response. J Neurol Neurosurg Psychiatry. (2000) 68:778–81. doi: 10.1136/jnnp.68.6.778
89. Savolainen S, Paljarvi L, Vapalahti M. Prevalence of Alzheimer's disease in patients investigated for presumed normal pressure hydrocephalus: a clinical and neuropathological study. Acta Neurochir (Wien). (1999) 141:849–53. doi: 10.1007/s007010050386
90. Leinonen V, Koivisto AM, Savolainen S, Rummukainen J, Tamminen JN, Tillgren T, et al. Amyloid and Tau proteins in cortical brain biopsy and Alzheimer's disease. Ann Neurol. (2010) 68:446–53. doi: 10.1002/ana.22100
91. Rinne JO, Wong DF, Wolk DA, Leinonen V, Arnold SE, Buckley C, et al. [(18)F]Flutemetamol pet imaging and cortical biopsy histopathology for fibrillar amyloid beta detection in living subjects with normal pressure hydrocephalus: pooled analysis of four studies. Acta Neuropathol. (2012) 124:833–45. doi: 10.1007/s00401-012-1051-z
92. Clark CM, Pontecorvo MJ, Beach TG, Bedell BJ, Coleman RE, Doraiswamy PM, et al. Cerebral pet with florbetapir compared with neuropathology at autopsy for detection of neuritic amyloid-beta plaques: a prospective cohort study. Lancet Neurol. (2012) 11:669–78. doi: 10.1016/S1474-4422(12)70142-4
93. Sabri O, Sabbagh MN, Seibyl J, Barthel H, Akatsu H, Ouchi Y, et al. Florbetaben pet imaging to detect amyloid beta plaques in alzheimer's disease: phase 3 study. Alzheimers Dement. (2015) 11:964–74. doi: 10.1016/j.jalz.2015.02.004
94. Curtis C, Gamez JE, Singh U, Sadowsky CH, Villena T, Sabbagh MN, et al. Phase 3 trial of flutemetamol labeled with radioactive fluorine 18 imaging and neuritic plaque density. JAMA Neurol. (2015) 72:287–94. doi: 10.1001/jamaneurol.2014.4144
95. Leinonen V, Alafuzoff I, Aalto S, Suotunen T, Savolainen S, Nagren K, et al. Assessment of beta-amyloid in a frontal cortical brain biopsy specimen and by positron emission tomography with carbon 11-labeled pittsburgh compound B. Arch Neurol. (2008) 65:1304–9. doi: 10.1001/archneur.65.10.noc80013
96. Wolk DA, Grachev ID, Buckley C, Kazi H, Grady MS, Trojanowski JQ, et al. Association between in vivo fluorine 18-labeled flutemetamol amyloid positron emission tomography imaging and in vivo cerebral cortical histopathology. Arch Neurol. (2011) 68:1398–403. doi: 10.1001/archneurol.2011.153
97. Wong DF, Moghekar AR, Rigamonti D, Brasic JR, Rousset O, Willis W, et al. An in vivo evaluation of cerebral cortical amyloid with [18f]flutemetamol using positron emission tomography compared with parietal biopsy samples in living normal pressure hydrocephalus patients. Mol Imaging Biol. (2013) 15:230–7. doi: 10.1007/s11307-012-0583-x
98. Rinne JO, Suotunen T, Rummukainen J, Herukka SK, Nerg O, Koivisto AM, et al. [11c]Pib pet is associated with the brain biopsy amyloid-beta load in subjects examined for normal pressure hydrocephalus. J Alzheimers Dis. (2019) 67:1343–51. doi: 10.3233/JAD-180645
99. Bugalho P, Alves L, Miguel R, Ribeiro O. Profile of cognitive dysfunction and relation with gait disturbance in normal pressure hydrocephalus. Clin Neurol Neurosurg. (2014) 118:83–8. doi: 10.1016/j.clineuro.2014.01.006
100. Picascia M, Minafra B, Zangaglia R, Gracardi L, Pozzi NG, Sinforiani E, et al. Spectrum of cognitive disorders in idiopathic normal pressure hydrocephalus. Funct Neurol. (2016) 31:143–7. doi: 10.11138/FNeur/2016.31.3.143
101. da Rocha SFB, Kowacs PA, de Souza RKM, Pedro MKF, Ramina R, Teive HAG. Serial tap test of patients with idiopathic normal pressure hydrocephalus: impact on cognitive function and its meaning. Fluids Barriers CNS. (2021) 18:22. doi: 10.1186/s12987-021-00254-3
102. Tarnaris A, Toma AK, Pullen E, Chapman MD, Petzold A, Cipolotti L, et al. Cognitive, biochemical, and imaging profile of patients suffering from idiopathic normal pressure hydrocephalus. Alzheimers Dement. (2011) 7:501–8. doi: 10.1016/j.jalz.2011.01.003
103. Iddon JL, Pickard JD, Cross JJ, Griffiths PD, Czosnyka M, Sahakian BJ. Specific patterns of cognitive impairment in patients with idiopathic normal pressure hydrocephalus and alzheimer's disease: a pilot study. J Neurol Neurosurg Psychiatry. (1999) 67:723–32. doi: 10.1136/jnnp.67.6.723
104. Gallagher RM, Marquez J, Osmotherly P. Cognitive and upper limb symptom changes from a tap test in idiopathic normal pressure hydrocephalus. Clin Neurol Neurosurg. (2018) 174:92–6. doi: 10.1016/j.clineuro.2018.09.015
105. Murakami Y, Matsumoto Y, Hoshi K, Ito H, Fuwa TJ, Yamaguchi Y, et al. Rapid increase of 'brain-type' transferrin in cerebrospinal fluid after shunt surgery for idiopathic normal pressure hydrocephalus: a prognosis marker for cognitive recovery. J Biochem. (2018) 164:205–13. doi: 10.1093/jb/mvy043
106. Nakajima M, Miyajima M, Ogino I, Akiba C, Sugano H, Hara T, et al. Cerebrospinal fluid biomarkers for prognosis of long-term cognitive treatment outcomes in patients with idiopathic normal pressure hydrocephalus. J Neurol Sci. (2015) 357:88–95. doi: 10.1016/j.jns.2015.07.001
107. Grasso G, Torregrossa F, Leone L, Frisella A, Landi A. Long-term efficacy of shunt therapy in idiopathic normal pressure hydrocephalus. World Neurosurg. (2019) 129:e458–e63. doi: 10.1016/j.wneu.2019.05.183
108. Peterson KA, Savulich G, Jackson D, Killikelly C, Pickard JD, Sahakian BJ. The effect of shunt surgery on neuropsychological performance in normal pressure hydrocephalus: a systematic review and meta-analysis. J Neurol. (2016) 263:1669–77. doi: 10.1007/s00415-016-8097-0
109. Yasar S, Jusue-Torres I, Lu J, Robison J, Patel MA, Crain B, et al. Alzheimer's disease pathology and shunt surgery outcome in normal pressure hydrocephalus. PLoS ONE. (2017) 12:e0182288. doi: 10.1371/journal.pone.0182288
110. Folstein MF, Folstein SE, McHugh PR. “Mini-mental state”. A practical method for grading the cognitive state of patients for the clinician. J Psychiatr Res. (1975) 12:189–98. doi: 10.1016/0022-3956(75)90026-6
111. Duff K. Evidence-based indicators of neuropsychological change in the individual patient: relevant concepts and methods. Arch Clin Neuropsychol. (2012) 27:248–61. doi: 10.1093/arclin/acr120
112. Bartels C, Wegrzyn M, Wiedl A, Ackermann V, Ehrenreich H. Practice effects in healthy adults: a longitudinal study on frequent repetitive cognitive testing. BMC Neurosci. (2010) 11:118. doi: 10.1186/1471-2202-11-118
113. Racine AM, Gou Y, Fong TG, Marcantonio ER, Schmitt EM, Travison TG, et al. Correction for retest effects across repeated measures of cognitive functioning: a longitudinal cohort study of postoperative delirium. BMC Med Res Methodol. (2018) 18:69. doi: 10.1186/s12874-018-0530-x
114. Solana E, Poca MA, Sahuquillo J, Benejam B, Junque C, Dronavalli M. Cognitive and motor improvement after retesting in normal-pressure hydrocephalus: a real change or merely a learning effect? J Neurosurg. (2010) 112:399–409. doi: 10.3171/2009.4.JNS081664
115. Kambara A, Kajimoto Y, Yagi R, Ikeda N, Furuse M, Nonoguchi N, et al. Long-term prognosis of cognitive function in patients with idiopathic normal pressure hydrocephalus after shunt surgery. Front Aging Neurosci. (2020) 12:617150. doi: 10.3389/fnagi.2020.617150
116. Solana E, Sahuquillo J, Junqué C, Quintana M, Poca MA. Cognitive disturbances and neuropsychological changes after surgical treatment in a cohort of 185 patients with idiopathic normal pressure hydrocephalus. Arch Clin Neuropsychol. (2012) 27:304–17. doi: 10.1093/arclin/acs002
117. Koivisto A. M., Alafuzoff I., Savolainen S., Sutela A., Rummukainen J., Kurki M., et al. Poor cognitive outcome in shunt-responsive idiopathic normal pressure hydrocephalus. Neurosurgery. (2013) 72:1–8. doi: 10.1227/NEU.0b013e31827414b3
118. Picascia M, Pozzi NG, Todisco M, Minafra B, Sinforiani E, Zangaglia R, et al. Cognitive disorders in normal pressure hydrocephalus with initial parkinsonism in comparison with de novo Parkinson's disease. Eur J Neurol. (2019) 26:74–9. doi: 10.1111/ene.13766
119. Laidet M, Herrmann FR, Momjian S, Assal F, Allali G. Improvement in executive subfunctions following cerebrospinal fluid tap test identifies idiopathic normal pressure hydrocephalus from its mimics. Eur J Neurol. (2015) 22:1533–9. doi: 10.1111/ene.12779
120. Liouta E, Gatzonis S, Kalamatianos T, Kalyvas A, Koutsarnakis C, Liakos F, et al. Finger tapping and verbal fluency post-tap test improvement in inph: its value in differential diagnosis and shunt-treatment outcomes prognosis. Acta Neurochir. (2017) 159:2301–7. doi: 10.1007/s00701-017-3301-2
121. Akiba C, Nakajima M, Miyajima M, Ogino I, Motoi Y, Kawamura K, et al. Change of Amyloid-? 1-42 toxic conformer ratio after cerebrospinal fluid diversion predicts long-term cognitive outcome in patients with idiopathic normal pressure hydrocephalus. J Alzheimer's Dis: JAD. (2018) 63:989–1002. doi: 10.3233/JAD-180059
122. Lim TS, Choi JY, Park SA, Youn YC, Lee HY, Kim BG, et al. Evaluation of coexistence of Alzheimer's disease in idiopathic normal pressure hydrocephalus using elisa analyses for Csf biomarkers. BMC Neurol. (2014) 14:66. doi: 10.1186/1471-2377-14-66
123. Kazui H, Kanemoto H, Yoshiyama K, Kishima H, Suzuki Y, Sato S, et al. Association between high biomarker probability of alzheimer's disease and improvement of clinical outcomes after shunt surgery in patients with idiopathic normal pressure hydrocephalus. J Neurol Sci. (2016) 369:236–41. doi: 10.1016/j.jns.2016.08.040
124. Patel S, Lee EB, Xie SX, Law A, Jackson EM, Arnold SE, et al. Phosphorylated Tau/amyloid beta 1-42 ratio in ventricular cerebrospinal fluid reflects outcome in idiopathic normal pressure hydrocephalus. Fluids Barriers CNS. (2012) 9:7. doi: 10.1186/2045-8118-9-7
125. Kamohara C, Nakajima M, Kawamura K, Akiba C, Ogino I, Xu H, et al. Neuropsychological tests are useful for predicting comorbidities of idiopathic normal pressure hydrocephalus. Acta Neurol Scand. (2020) 142:623–31. doi: 10.1111/ane.13306
126. Ogino A, Kazui H, Miyoshi N, Hashimoto M, Ohkawa S, Tokunaga H, et al. Cognitive impairment in patients with idiopathic normal pressure hydrocephalus. Dement Geriatr Cogn Disord. (2006) 21:113–9. doi: 10.1159/000090510
127. Kaya D, Erken N, Ontan MS, Altun ZS, Isik AT. The applause sign in elderly patients with idiopathic normal pressure hydrocephalus. Appl Neuropsychol Adult. (2020). doi: 10.1080/23279095.2020.1818563. [Epub ahead of print].
Keywords: Idiopathic Normal Pressure Hydrocephalus, dementia, Alzheimer's disease, Lewy body disease, progressive supranuclear palsy, corticobasal degeneration, vascular dementia, cognitive
Citation: Langheinrich T, Chen C and Thomas O (2022) Update on the Cognitive Presentations of iNPH for Clinicians. Front. Neurol. 13:894617. doi: 10.3389/fneur.2022.894617
Received: 11 March 2022; Accepted: 19 May 2022;
Published: 20 July 2022.
Edited by:
Madoka Nakajima, Juntendo University, JapanReviewed by:
Diego Iacono, Neuroscience - Uniformed Services University of the Health Sciences (USU), United StatesCopyright © 2022 Langheinrich, Chen and Thomas. This is an open-access article distributed under the terms of the Creative Commons Attribution License (CC BY). The use, distribution or reproduction in other forums is permitted, provided the original author(s) and the copyright owner(s) are credited and that the original publication in this journal is cited, in accordance with accepted academic practice. No use, distribution or reproduction is permitted which does not comply with these terms.
*Correspondence: Tobias Langheinrich, dG9iaWFzLmxhbmdoZWlucmljaEBuY2EubmhzLnVr
†These authors have contributed equally to this work and share first authorship