- 1Department of Neurosurgery, West China Hospital, Sichuan University, Chengdu, China
- 2Medical Simulation Center, Chengdu First People's Hospital, Chengdu, China
- 3College of Electronic Engineering (College of Meteorological Observation), Chengdu University of Information Technology, Chengdu, China
- 4Department of Neurosurgery, The Second Affiliated Hospital of Chengdu Medical College, China National Nuclear Corporation 416 Hospital, Chengdu, China
Background: Photobiomodulation (PBM) using low-level light-emitting diodes (LEDs) can be rapidly applied to various neurological disorders safely and non-invasively.
Materials and Methods: Forty-eight rats were involved in this study. The traumatic brain injury (TBI) model of rat was set up by a controlled cortical impact (CCI) injury. An 8-channel cortex electrode EEG was fixed to two hemispheres, and gamma oscillations were extracted according to each electrode. A 40 hz blue LED stimulation was set at four points of the frontal and parietal regions for 60 s each, six times per day for 1 week. Modified Neurologic Severity Scores (mNSS) were used to evaluate the level of neurological function.
Results: In the right-side TBI model, the gamma oscillation decreased in electrodes Fp2, T4, C4, and O2; but significantly increased after 1 week of 40 hz Blue LED intervention. In the left-side TBI model, the gamma oscillation decreased in electrodes Fp1, T3, C3, and O1; and similarly increased after 1 week of 40 hz Blue LED intervention. Both left and right side TBI rats performed significantly better in mNSS after 40 hz Blue LED intervention.
Conclusion: TBI causes the decrease of gamma oscillations on the injured side of the brain of rats. The 40 hz Blue LED therapy could relieve the gamma oscillation changes caused by TBI and improve the prognosis of TBI.
Introduction
The invention of the electroencephalogram (EEG) facilitates the observation of brain electrical activity (1, 2); the most common frequency band in EEG is 8–12 Hz, known as α-rhythm, and there are faster oscillations where the frequency is between 30 and 80 Hz, named gamma oscillations (3). Gamma oscillations were first discovered by Adrian (4) who reported that the oscillations were induced by odorous substances. Since, studies have demonstrated that they could be induced by other stimuli and be presented in various structures in the human brain, with different functional connections, such as learning, sensory, consciousness, and motor functions (5–8).
More than 50 million people suffer TBIs each year worldwide, and it is a major cause of death and disability for children and adults in all countries, leading to a great amount of burden upon society (9). Deficiencies in TBI research need to be emphasized to figure out solutions to reduce societal costs of TBI. Although many works have investigated the relationship between changes in gamma oscillations and abnormality in the nervous system (10–12), the interplay between gamma oscillations and TBI has yet to be confirmed. In general, changes in brain electrical activity occur after TBI (13, 14). A study showed that subjects with TBI display disturbed gamma synchrony activity (15), but they did not propose the degree of changes. We aim to determine how gamma oscillations change in TBI models of different degrees of injury.
A small proportion of severe TBIs will remain in a coma or vegetative state (16), and many TBI patients are likely to exhibit cognitive function decline and motor dysfunction (17). Rehabilitation for patients with TBI is a complex process, the possible therapy includes right median nerve stimulation (16), but the therapeutic benefits need further verification. Bain PBM therapy is an innovative and effective treatment used in various neurological diseases such as TBI, Alzheimer's disease, and age-related cognitive decline (18, 19). This non-invasive therapy could produce treatment effects without causing adverse influence. Non-invasive 40 Hz light could stimulate elevation of gamma oscillations (16, 20). We further observe the interplay between gamma oscillations changes and try to observe prognosis in TBI models.
Understanding how gamma oscillations changes after TBI has implications for further intervention. Our purpose is to determine how gamma oscillations change following TBI and examine whether non-invasive 40 Hz light could be a possible intervention to improve the prognosis in TBI models.
Materials and Methods
Animals
A total of 48 two-month-old male Sprague-Dawley rats (200–225 g,) were obtained from the Laboratory Animal Center of Sichuan University, and free access was given to food and water throughout the study. All the rats scored 0 in mNSS before the experiment and there was no statistical difference. Animals were randomly divided into left hemisphere TBI and right hemisphere TBI and each was divided into four subgroups: control (rats received sham operation); TBI (rats received TBI induced by an impact device and immediate 8-channel cortex electrodes EEG detection after injury); TBI (7d) (8-channel cortex electrodes EEG was obtained seven days after TBI); TBI+40 Hz light (7d) (rats received 40 hz Blue LED intervention for consecutive seven days after TBI). Each subgroup consisted of six rats.
TBI Model
The traumatic brain injury (TBI) model of the rats was set up by a controlled cortical impact (CCI) injury (21). Rats were anesthetized with 5% isoflurane and then maintained with 2% isoflurane for the duration of the procedure. After the operative region was shaved, the animal was positioned in a stereotactic frame. Then, the skin above the skull was prepped with bated iodine solution, and the skull was exposed through a small skin incision made by a sterile scalpel blade. An area about 5 mm in diameter was exposed with the use of a dental drill, and the center of the area is 2.7 mm beside the midline and 3 mm in front of the lambda suture. The dura was kept intact during the operation. The exposed cortex was subjected to CCI. The position of the impactor was is 1.2 mm beside the midline and 1.5 mm in front of the lambda suture. CCI was produced carefully in the center above the craniotomy area, using an electromagnetic CCI device with a 3.0 mm diameter impactor rod-tip, hitting the cortex at a velocity of 4.0 m/s with an impact depth of 2.5 mm and impact time of 50 ms to produce injury within the left or right parietal cortex. After the CCI procedure, bone wax was applied to the exposed surface of the dura and skull surface. The skin incision was sutured, followed by the application of bated iodine solution over the sutured area. The same surgical procedures were done to the sham injury control rats except for the CCI procedures. Animals with TBI were kept warm during the experiments.
Gamma Data Acquisition
All animals were placed stably in a stereotaxic apparatus to acquire the gamma data using an 8-channel cortex electrodes EEG (Fp1, Fp2, T3, T4, C3, C4, O1, and O2). Fp1, T3, C3, and O1 are fixed to the frontal, temporal, parietal, and occipital lobes of the left hemisphere, whereas Fp2, T4, C4, and O2 are fixed to the frontal, temporal, parietal, and occipital lobe of the right hemisphere, respectively (Figure 1). We recorded the gamma oscillations power of control and TBI groups after CCI or the sham operation, respectively. Similarly, we recorded the gamma oscillations power 1 week after TBI.
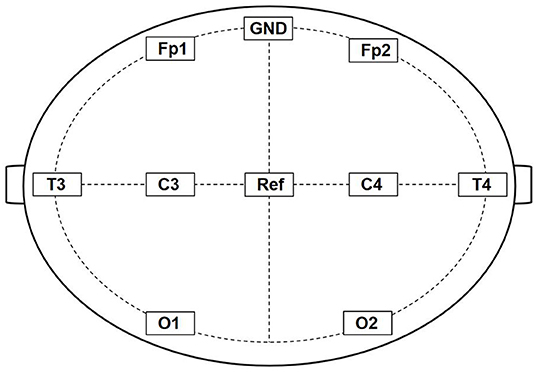
Figure 1. Schematic diagram of 8-channel cortex electrodes EEG. Fp1, T3, C3, and O1 are fixed to the frontal, temporal, parietal, and occipital lobes of the left hemisphere, whereas Fp2, T4, C4, and O2 are fixed to the frontal, temporal, parietal, and occipital lobe of the right hemisphere, respectively. GND was connected to an electrical ground and Ref is the Reference electrode.
40 Hz Blue Light-Emitting Diode Therapy Protocol
Rats were submitted to 40 hz Blue LED at a 473 nm wavelength, with 0.2 W LED power, 70-mW/cm2 power density, 10-J/cm2 energy density, 13-mm spot size, and 40 J/cm2 per treatment. At short wavelengths, absorption occurs predominantly by chromophores such as melanin and hemoglobin.
In order to avoid skin burning, the power density of 70 mW/cm2 was considered safe. The wavelength (~473 nm) is characterized by a relatively low energy density (40 J/cm2) for therapeutic uses. The application of LED irradiation will be in four points in the frontal and parietal regions for 60 s at each point (240 s total/session) six times per day during a week.
Modified Neurologic Severity Scores
The mNSS was used to evaluate the level of neurological function of TBI rats. The neurological severity score is a composite of motor, sensor, balance, and reflex which could reflect the level of neurological function of rats (22–24). The higher the score, the more severe the injury (Table 1).
Statistical Analysis
All the data was described as mean ± SEM. Statistical analysis was performed with SPSS for Windows, version 19.0. The differences among multiple groups were assessed using a one-way analysis of variance (ANOVA) with a significance level of p < 0.05. Post-hoc comparisons between groups were further detected using the least significant difference (LSD) method. Sphericity test used methods KMO and Bartlett's Test. All statistical analysis was done by a professional statistician.
Result
In the rats with moderate to severe TBI on the right side, the EEG pattern was shown in Figure 2. The gamma oscillation we recorded after TBI showed a significant decrease in cortex electrodes Fp2 (P = 0.002), T4 (P = 0.001), C4 (P= 0.009), and O2 (P = 0.001) (Figure 3A). After 1 week of 40 hz Blue LED intervention, the gamma oscillation of rats significantly increased in these cortex electrodes, Fp2 (P = 0.001), T4 (P < 0.001), C4 (P < 0.001), and O2 (P < 0.007), compared with the TBI (R,7d) group (Figure 3B, Table 2).
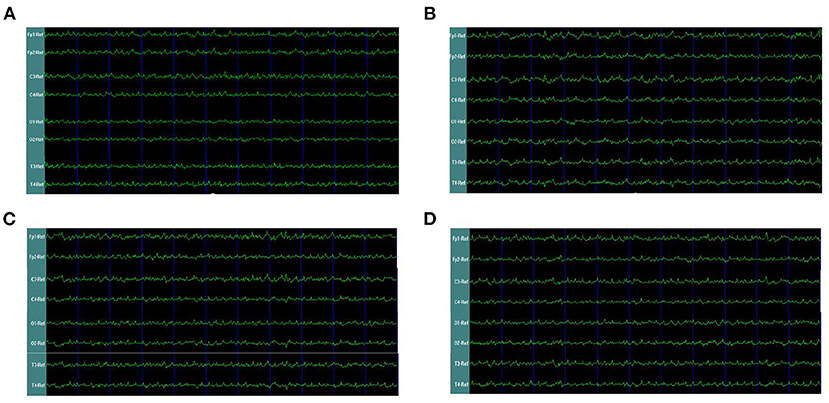
Figure 2. EEG waveforms of right-side TBI in rats. (A) EEG waveforms of control subgroup (B) EEG waveforms recorded immediately after TBI (C) EEG waveforms of 7 days after TBI without treatments (D) EEG waveforms of TBI after 7-days treatment of 40 hz Blue LED.
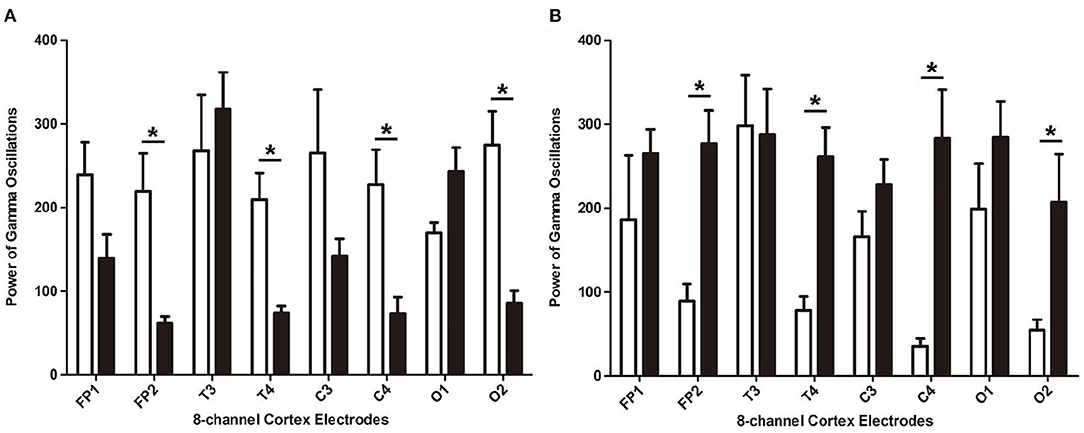
Figure 3. The power of gamma oscillations of right-side TBI group in different electrodes. (A) Power of gamma oscillations of rat before and after TBI. The white bar represents the control group (a), and the black bar represents TBI (R, 7d) group. (B) Power of gamma oscillations of TBI with and without treatment of 40 hz Blue LED. The white bar represents TBI (R, 7d), and the black bar represents TBI (R)+40 hz light (7d). P-values correspond to one-way ANOVA. Data are expressed as mean±SEM. *P < 0.05.
In our second phase of the research, the rat model of left side TBI was built. The EEG pattern is shown in Figure 4. We found that the gamma oscillation of rats with left TBI significantly decreased in cortex electrodes Fp1 (P < 0.001), T3 (P < 0.001), C3 (P < 0.001), and O1 (P = 0.002) (Figure 5A, Table 3). Similarly, after receiving 1 week of 40 hz Blue LED intervention, the gamma oscillation of rats significantly increased in these cortex electrodes Fp1 (P = 0.001), T3 (P < 0.001), C3 (P = 0.001), and O1 (P = 0.001), comparing with the TBI(L,7d) group (Figure 5B, Table 4) and this result was completely consistent with that of right side TBI rats.
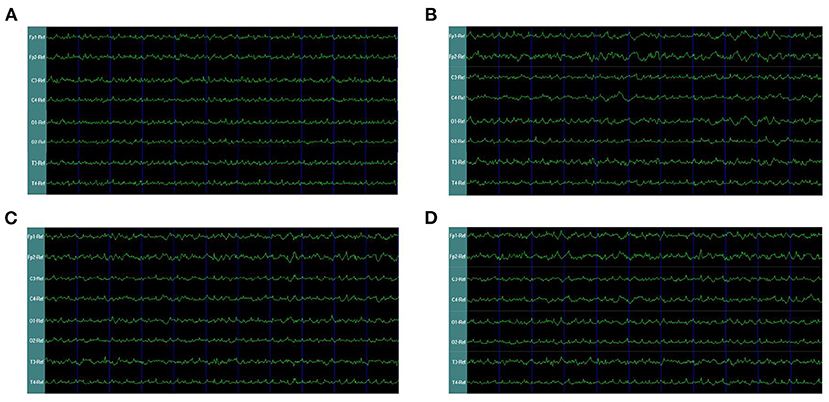
Figure 4. EEG waveforms of left-side TBI in rats. (A) EEG waveforms of control subgroup (B) EEG waveforms recorded immediately after TBI (C) EEG waveforms of 7 days after TBI without treatments (D) EEG waveforms of TBI after 7-days treatment of 40 hz Blue LED.
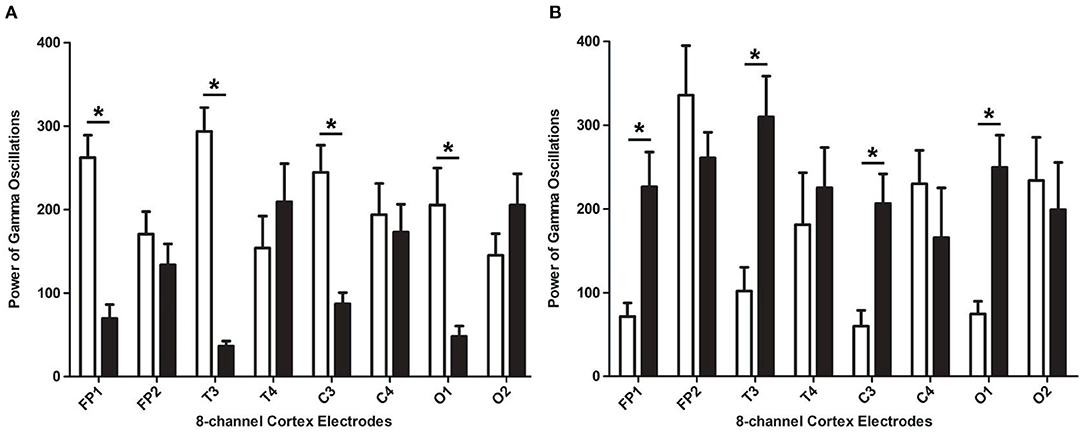
Figure 5. The absolute energy of gamma oscillations of left-side TBI group in different electrodes. (A) Absolute energy of gamma oscillations of rat before and after TBI. The white bar represents control group (b), and the black bar represents TBI (L, 7d). (B) Absolute energy of gamma oscillations of TBI with and without 7-days treatment of 40 hz Blue LED. The white bar represents TBI (L, 7d), and the black bar represents TBI (L)+40 hz light (7d). P-values correspond to one-way ANOVA. Data are expressed as mean ± SEM. *P < 0.05.
The rats that received 40 hz Blue LED invention performed significantly better in mNSS than the TBI rats 7 days after injury. This result appeared both in the right side TBI (P = 0.013) (Figure 6A) and left side TBI models (P = 0.004) (Figure 6B, Table 5).
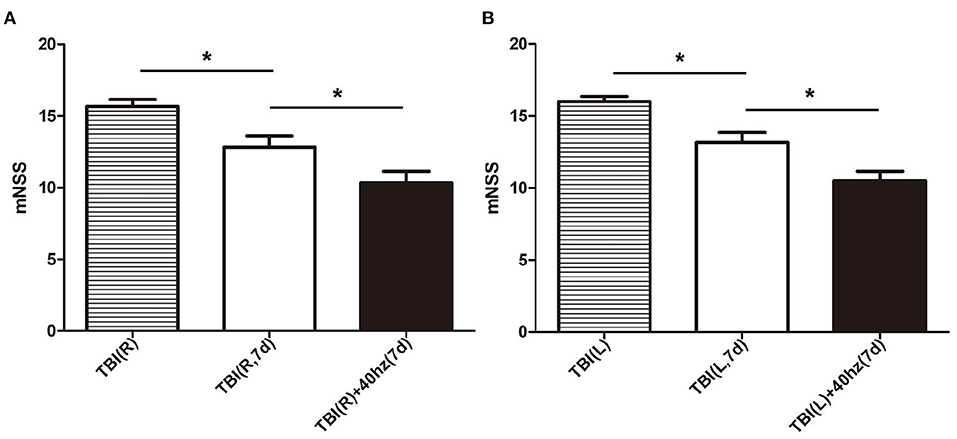
Figure 6. The mNSS in different subgroups. (A) The mNSS evaluated of right-side TBI group. The stripe, white and black bar represent for TBI (R), TBI (R, 7d) and TBI (R)+40 hz light (7d), respectively. (B) The mNSS evaluated of left-side TBI group. The stripe, white and black bar represent for TBI (L), TBI (L, 7d), and TBI(L)+40 hz light (7d), respectively. P-values correspond to one-way ANOVA. Data are expressed as mean ± SEM. *P < 0.05.
Discussion
Gamma oscillations play a pivotal role in multiple cognitive functions. They enable coordinated activity and communication of local assemblies, while abnormalities in gamma oscillations exist in different neurological and psychiatric diseases (25, 26). Increasing evidence shows that guiding or driving gamma oscillations are being investigated as a potential treatment paradigm for memory deficits due to Alzheimer's disease and other memory impairments (27, 28), but it has not been explored in TBI. Hannah et al. have reported that 40 Hz gamma oscillations may induce an overall neuroprotective response that recruits both neurons and microglia (11). In our study, we are trying to find out how TBI effects the gamma oscillations in rats, and if 40 hz Blue LED could be a non-invasive adjuvant therapy for TBI.
In our study, the cortex electrodes Fp1, T3, C3, and O1 were fixed to the frontal, temporal, parietal, and occipital lobe of the left hemisphere, and the cortex electrodes Fp2, T4, C4, and O2 were fixed to the frontal, temporal, parietal, and occipital lobe of the right hemisphere, respectively. In the first phase, we found that the rats with right side TBI showed a decrease in cortex electrodes Fp2, T4, C4, and O2, which corresponds to the injured side. This result coincides with several previous studies which have demonstrated that lateral fluid percussion TBI in rats resulted in decreased theta power (29, 30). After building the TBI model, the rats received 40 hz Blue LEDs for 60 s at each point during a week. Surprisingly, the result showed TBI+40 hz light (R-7d) subgroup had a significant increase in those cortex electrodes but remained at the same level in the Fp1, T3, C3, and O1 cortex electrodes. In other words, the 40 hz Blue LED intervention partly reverses the decline in gamma oscillations caused by TBI.
In order to verify the effectiveness and feasibility of 40 hz Blue LED therapy, we established the left brain injury rat model in the second stage. We built rat TBI model of the left side,'with all other conditions being completely the same. As expected theoretically, after the TBI in the left side, we recorded a decrease in cortex electrodes Fp1, C3, T3, and O1. This indicates that the gamma oscillation decreases only in the affected side of the TBI, but have no influence on the lobe of the healthy side. After the 40 hz Blue LED intervention for a week, the gamma oscillations in cortex electrodes Fp1, C3, T3, and O1 were increased significantly, compared to the TBI(L, 7d) group. The results of these two phases strongly suggest that 40 hz Blue LED therapy could effectively reverse the gamma oscillations decrease in the injured cerebral cortex.
Many studies have reported that continuous low-frequency stimulation could significantly improve spatial learning in injured animals (31, 32). The effects of PBM therapy have also received increasing attention on multiple diseases, especially neurological disorders such as Alzheimer's and Parkinson's (26, 33). However, relatively few studies focus on the PBM therapy for TBI. In our study, the rats received 40 hz Blue LED therapy performed significantly better in mNSS than the TBI rats 7 days after injury; we could infer that 40 hz Blue LED therapy could improve the prognosis of different types of TBI, and possibly by increasing the gamma oscillations to repair the neurological function. These results are is analogous to some research groups that focused on the PBM therapy in TBI. Oron et al., for example, examined the effects of low-level laser therapy for TBI mice. A closed-head injury was induced by a weight-drop device. They found that TBI mice treated with laser showed better outcomes in neurobehavioral function (34). Khuman et al. demonstrated that low-level laser light therapy could improve cognitive function in CCI mice (35). The underlying mechanism could be explained in two phases. In the first phase, optogenetics induce or enhance the gamma oscillations. It has been demonstrated that by stimulating the optogenetic activator channelrhodopsin-2 (ChR2), expressed either in excitatory neurons of CA3 or CA1 or in stellate cells and interneurons in the mEC, local gamma oscillations can be induced in each of these areas (36–38). In the second phase, induced gamma oscillations in the rat highlight the intersection both in memory and locomotion mediating well-timed interactions within and across neuronal networks (39, 40). Conclusively, our research indicates that 40 hz Blue LED intervention may be an effective and novel neuromodulatory therapy for the treatment of persistent cognitive deficits following TBI.
Conclusion
TBI causes a decrease of gamma oscillations in the injured brain side of rats. It appears 40 hz Blue LED therapy could relieve the gamma oscillation change caused by TBI and improve the prognosis of TBI which have the potential to become an effective and novel neuromodulatory therapy for the treatment of persistent cognitive deficits following TBI.
Data Availability Statement
The raw data supporting the conclusions of this article will be made available by the authors, without undue reservation.
Ethics Statement
The animal study was reviewed and approved by College of Basic Medicine and Forensic Medicine, Sichuan University.
Author Contributions
XY: study concept and design, animal experiments, analysis, and interpretation of data. XL: design and interpretation data. BD, YY, and TS: animal experiments. JY and HY: statistical analysis. AG and JG: study supervision. All authors contributed to the article and approved the submitted version.
Funding
This study was funded by the 1.3.5 project for disciplines of excellence-Clinical Research Incubation Project, West China Hospital, Sichuan University (21HXFH013), the National Natural Science Foundation of China (31371180), and the Key Technology Research and Development Program of Science and Technology of Sichuan Province (2015SZ0193).
Conflict of Interest
The authors declare that the research was conducted in the absence of any commercial or financial relationships that could be construed as a potential conflict ofinterest.
Publisher's Note
All claims expressed in this article are solely those of the authors and do not necessarily represent those of their affiliated organizations, or those of the publisher, the editors and the reviewers. Any product that may be evaluated in this article, or claim that may be made by its manufacturer, is not guaranteed or endorsed by the publisher.
Acknowledgments
We would like to thank West China Hospital of Sichuan University for its assistance in our experiments.
References
1. Berger H. On the electroencephalogram of man. Electroencephalogr Clin Neurophysiol. (1969) Suppl. 28:37.
2. Müller-Putz GR. Electroencephalography. Handb Clin Neurol. (2020) 168:249–62. doi: 10.1016/B978-0-444-63934-9.00018-4
3. Chatrian GE, Bickford RG, Uihlein A. Depth electrographic study of a fast rhythm evoked from the human calcarine region by steady illumination. Electroencephalogr Clin Neurophysiol. (1960) 12:167–76. doi: 10.1016/0013-4694(60)90070-5
4. Adrian ED. Olfactory reactions in the brain of the hedgehog. J Physiol. (1942) 100:459–73. doi: 10.1113/jphysiol.1942.sp003955
5. Wiesman AI, Murman DL, May PE, Schantell M, Wolfson SL, Johnson CM, et al. Visuospatial alpha and gamma oscillations scale with the severity of cognitive dysfunction in patients on the Alzheimer's disease spectrum. Alzheimer's Res Ther. (2021) 13:139. doi: 10.1186/s13195-021-00881-w
6. Li KT, Liang J, Zhou C. Gamma oscillations facilitate effective learning in excitatory-inhibitory balanced neural circuits. Neural Plast. (2021) 2021:6668175. doi: 10.1155/2021/6668175
7. Han C, Wang T, Wu Y, Li Y, Yang Y, Li L, et al. The generation and modulation of distinct gamma oscillations with local, horizontal, and feedback connections in the primary visual cortex: a model study on large-scale networks. Neural Plast. (2021) 2021:8874516. doi: 10.1155/2021/8874516
8. Leicht G, Björklund J, Vauth S, Mußmann M, Haaf M, Steinmann S, et al. Gamma-band synchronisation in a frontotemporal auditory information processing network. Neuroimage. (2021) 239:118307. doi: 10.1016/j.neuroimage.2021.118307
9. Capizzi A, Woo J, Verduzco-Gutierrez M. Traumatic brain injury: an overview of epidemiology, pathophysiology, and medical management. Med Clin North Am. (2020) 104:213–38. doi: 10.1016/j.mcna.2019.11.001
10. Adaikkan C, Middleton SJ, Marco A, Pao PC, Mathys H, Kim DN, et al. Gamma entrainment binds higher-order brain regions and offers neuroprotection. Neuron. (2019) 102:929–43.e8. doi: 10.1016/j.neuron.2019.04.011
11. Park K, Lee J, Jang HJ, Richards BA, Kohl MM, Kwag J. Optogenetic activation of parvalbumin and somatostatin interneurons selectively restores theta-nested gamma oscillations and oscillation-induced spike timing-dependent long-term potentiation impaired by amyloid β oligomers. BMC Biol. (2020) 18:7. doi: 10.1186/s12915-019-0732-7
12. Casanova MF, Shaban M, Ghazal M, El-Baz AS, Casanova EL, Sokhadze EM. Ringing decay of gamma oscillations and transcranial magnetic stimulation therapy in autism spectrum disorder. Appl Psychophysiol Biofeedback. (2021) 46:161–73. doi: 10.1007/s10484-021-09509-z
13. Hanley D, Prichep LS, Badjatia N, Bazarian J, Chiacchierini R, Curley KC, et al. A brain electrical activity electroencephalographic-based biomarker of functional impairment in traumatic brain injury: a multi-site validation trial. J Neurotrauma. (2018) 35:41–7. doi: 10.1089/neu.2017.5004
14. Hanley D, Prichep LS, Bazarian J, Huff JS, Naunheim R, Garrett J, et al. Emergency department triage of traumatic head injury using a brain electrical activity biomarker: a multisite prospective observational validation trial. Acad Emerg Med. (2017) 24:617–27. doi: 10.1111/acem.13175
15. Slewa-Younan S, Green AM, Baguley IJ, Felmingham KL, Haig AR. Gordon E. Is ‘gamma' (40 Hz) synchronous activity disturbed in patients with traumatic brain injury? Clin Neurophysiol. (2002) 113:1640–6. doi: 10.1016/S1388-2457(02)00239-0
16. Lei J, Wang L, Gao G, Cooper E, Jiang J. Right median nerve electrical stimulation for acute traumatic coma patients. J Neurotrauma. (2015) 32:1584–9. doi: 10.1089/neu.2014.3768
17. Ponsford J, Harrison-Felix C, Ketchum JM, Spitz G, Miller AC, Corrigan JD. Outcomes 1 and 2 years after moderate to severe traumatic brain injury: an international comparative study. Arch Phys Med Rehabil. (2021) 102:371–7. doi: 10.1016/j.apmr.2020.09.387
18. Salehpour F, Mahmoudi J, Kamari F, Sadigh-Eteghad S, Rasta SH, Hamblin MR. Brain photobiomodulation therapy: a narrative review. Mol Neurobiol. (2018) 55:6601–36. doi: 10.1007/s12035-017-0852-4
19. Santos J, Zaninotto A, Zângaro RA, Carneiro A, Neville IS, de Andrade AF, et al. Effects of transcranial LED therapy on the cognitive rehabilitation for diffuse axonal injury due to severe acute traumatic brain injury: study protocol for a randomized controlled trial. Trials. (2018) 19:249. doi: 10.1186/s13063-018-2632-5
20. Van der Tweel LH, Spekreijse H. Signal transport and rectification in the human evoked-response system. Ann N Y Acad Sci. (1969) 156:678–95. doi: 10.1111/j.1749-6632.1969.tb14007.x
21. Brody DL, Mac Donald C, Kessens CC, Yuede C, Parsadanian M, Spinner M, et al. Electromagnetic controlled cortical impact device for precise, graded experimental traumatic brain injury. J Neurotrauma. (2007) 24:657–73. doi: 10.1089/neu.2006.0011
22. Ruan J, Yao Y. Behavioral tests in rodent models of stroke. Brain Hemorrhages. (2020) 1:171–84. doi: 10.1016/j.hest.2020.09.001
23. Markgraf CG, Green EJ, Hurwitz BE, Morikawa E, Dietrich WD, McCabe PM, et al. Sensorimotor and cognitive consequences of middle cerebral artery occlusion in rats. Brain Res. (1992) 575:238–46. doi: 10.1016/0006-8993(92)90085-N
24. Borlongan CV, Randall TS, Cahill DW, Sanberg PR. Asymmetrical motor behavior in rats with unilateral striatal excitotoxic lesions as revealed by the elevated body swing test. Brain Res. (1995) 676:231–4. doi: 10.1016/0006-8993(95)00150-O
25. Koshiyama D, Miyakoshi M, Tanaka-Koshiyama K, Sprock J, Light GA. High-power gamma related delta phase alteration in schizophrenia patients at rest. Psychiatry Clin Neurosci. (2022) 76:179–86. doi: 10.1111/pcn.13331
26. Takasu K, Niidome K, Hasegawa M, Ogawa K. Histone deacetylase inhibitor improves the dysfunction of hippocampal gamma oscillations and fast spiking interneurons in Alzheimer's disease model mice. Front Mol Neurosci. (2021) 14:782206. doi: 10.3389/fnmol.2021.782206
27. Traikapi A, Konstantinou N. Gamma oscillations in Alzheimer's disease and their potential therapeutic role. Front Syst Neurosci. (2021) 15:782399. doi: 10.3389/fnsys.2021.782399
28. Ma R, Xia X, Zhang W, Lu Z, Wu Q, Cui J, et al. High gamma and beta temporal interference stimulation in the human motor cortex improves motor functions. Front Neurosci. (2022) 15:800436. doi: 10.3389/fnins.2021.800436
29. Fedor M, Berman RF, Muizelaar JP, Lyeth BG. Hippocampal θ dysfunction after lateral fluid percussion injury. J Neurotrauma. (2010) 27:1605–15. doi: 10.1089/neu.2010.1370
30. Lee DJ, Gurkoff GG, Izadi A, Berman RF, Ekstrom AD, Muizelaar JP, et al. Medial septal nucleus theta frequency deep brain stimulation improves spatial working memory after traumatic brain injury. J Neurotrauma. (2013) 30:131–9. doi: 10.1089/neu.2012.2646
31. Lee DJ, Gurkoff GG, Izadi A, Seidl SE, Echeverri A, Melnik M, et al. Septohippocampal neuromodulation improves cognition after traumatic brain injury. J Neurotrauma. (2015) 32:1822–32. doi: 10.1089/neu.2014.3744
32. Esmaeilpour K, Sheibani V, Shabani M, Mirnajafi-Zadeh J. Effect of low frequency electrical stimulation on seizure-induced short- and long-term impairments in learning and memory in rats. Physiol Behav. (2017) 168:112–21. doi: 10.1016/j.physbeh.2016.11.001
33. Bicknell B, Liebert A, McLachlan CS, Kiat H. Microbiome changes in humans with Parkinson's disease after photobiomodulation therapy: a retrospective study. J Pers Med. (2022) 12:49. doi: 10.3390/jpm12010049
34. Oron A, Oron U, Streeter J, de Taboada L, Alexandrovich A, Trembovler V, et al. Low-level laser therapy applied transcranially to mice following traumatic brain injury significantly reduces long-term neurological deficits. J Neurotrauma. (2007) 24:651–6. doi: 10.1089/neu.2006.0198
35. Khuman J, Zhang J, Park J, Carroll JD, Donahue C, Whalen MJ. Low-level laser light therapy improves cognitive deficits and inhibits microglial activation after controlled cortical impact in mice. J Neurotrauma. (2012) 29:408–17. doi: 10.1089/neu.2010.1745
36. Pastoll H, Solanka L, van Rossum MC, Nolan MF. Feedback inhibition enables θ-nested γ oscillations and grid firing fields. Neuron. (2013) 77:141–54. doi: 10.1016/j.neuron.2012.11.032
37. Butler JL, Mendonça PR, Robinson HP, Paulsen O. Intrinsic cornu ammonis area 1 theta-nested gamma oscillations induced by optogenetic theta frequency stimulation. J Neurosci. (2016) 36:4155–69. doi: 10.1523/JNEUROSCI.3150-15.2016
38. Betterton RT, Broad LM, Tsaneva-Atanasova K, Mellor JR. Acetylcholine modulates gamma frequency oscillations in the hippocampus by activation of muscarinic M1 receptors. Eur J Neurosci. (2017) 45:1570–85. doi: 10.1111/ejn.13582
39. Dhaynaut M, Sprugnoli G, Cappon D, Macone J, Sanchez JS, Normandin M, et al. Impact of 40 Hz transcranial alternating current stimulation on cerebral tau burden in patients with Alzheimer's disease: a case series. J Alzheimer's Dis. (2021) 85:1667–76. doi: 10.3233/JAD-215072
Keywords: traumatic brain injury, gamma oscillations, LED, rat, electroencephalogram
Citation: Yang X, Li X, Yuan Y, Sun T, Yang J, Deng B, Yu H, Gao A and Guan J (2022) 40 Hz Blue LED Relieves the Gamma Oscillations Changes Caused by Traumatic Brain Injury in Rat. Front. Neurol. 13:882991. doi: 10.3389/fneur.2022.882991
Received: 24 February 2022; Accepted: 22 April 2022;
Published: 21 June 2022.
Edited by:
Guoyi Gao, Shanghai General Hospital, ChinaReviewed by:
Huaqiu Zhang, Huazhong University of Science and Technology, ChinaSoomaayeh Heysieattalab, University of Tabriz, Iran
Copyright © 2022 Yang, Li, Yuan, Sun, Yang, Deng, Yu, Gao and Guan. This is an open-access article distributed under the terms of the Creative Commons Attribution License (CC BY). The use, distribution or reproduction in other forums is permitted, provided the original author(s) and the copyright owner(s) are credited and that the original publication in this journal is cited, in accordance with accepted academic practice. No use, distribution or reproduction is permitted which does not comply with these terms.
*Correspondence: Junwen Guan, JunwenGuan@163.com