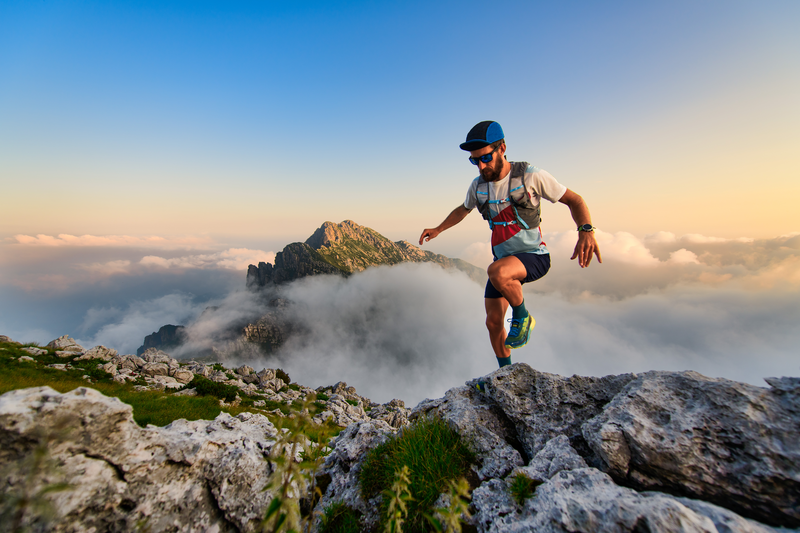
94% of researchers rate our articles as excellent or good
Learn more about the work of our research integrity team to safeguard the quality of each article we publish.
Find out more
ORIGINAL RESEARCH article
Front. Neurol. , 13 June 2022
Sec. Sleep Disorders
Volume 13 - 2022 | https://doi.org/10.3389/fneur.2022.882465
This article is part of the Research Topic Clinical Aspects of Obstructive Sleep Apnea and Cardiovascular Consequences View all 9 articles
Background: The titration pressure of continuous positive airway pressure (CPAP) is important in patients with obstructive sleep apnea (OSA). This study aimed to understand the difference between drug-induced sleep endoscopy (DISE)-guided CPAP titration and conventional sleep center (CSC) CPAP titration in patients with OSA.
Methods: In this randomized, controlled, and single-blind crossover trial, we compared the effects of 1-month CPAP treatment in patients with OSA with either DISE-guided CPAP titration or CSC CPAP titration. Twenty-four patients with OSA were recruited for the study. All patients underwent polysomnography, DISE-guided CPAP titration, and accommodation. Initially, patients were randomly assigned to receive either DISE-guided CPAP titration or CSC CPAP treatment for the first month. They were then switched to other treatments in the second month. The Epworth sleepiness scale (ESS) score was recorded at baseline, 1 and 2 months.
Results: The upper limit of the pressure of DISE-guided titration and CSC CPAP titration was not significantly different (13.9 ± 0.7 vs. 13.5 ± 0.5 cm H2O; P = 0.92). The residual apnea-hypopnea index and compliance were also not significantly different between the groups. ESS score significantly improved from baseline to 1 month after CPAP treatment in both groups. Both epiglottis (anterior-posterior collapse) and tongue base collapse were significantly associated with 95% CPAP pressure (P = 0.031 and 0.038, respectively). After multivariate regression analyses, the epiglottis (anterior-posterior collapse) was an independent factor for 95% CPAP pressure. The incidence rate of bradycardia was 58.3%, which is a safety concern for DISE. Despite the high incidence of bradycardia, all patients with bradycardia recovered with proper management.
Conclusion: Both modalities were comparable in terms of establishing the pressure settings required to treat patients. Further large-scale studies are required to confirm these results.
Trial registration: https://clinicaltrials.gov/, NCT03523013.
Obstructive sleep apnea (OSA) is a prevalent disorder with severe cardiovascular and neurocognitive consequences that can be reduced by effective treatment (1–4). Continuous positive airway pressure ventilation (CPAP), the first line of gold standard therapy, is used to treat OSA using a pneumatic splint and increase lung volume to keep the airway open, while the airway muscles relax during sleep (5, 6). When CPAP is applied to patients with OSA during sleep, all levels of airway obstruction are anticipated to be adequately opened. However, some patients with OSA cannot tolerate CPAP because of the pressure setting. Therefore, it is important to titrate adequate pressure for patients.
Traditionally, adequate pressure has been determined by manual titration in a sleep laboratory. However, laboratory-based manual titration of CPAP is time-consuming, expensive, and labor-intensive. Therefore, Auto-CPAP titration at home under new-generation ventilator machine programs is being used to solve this dilemma. A multicenter and randomized study showed that home-based auto-CPAP titration is not inferior to traditional laboratory-based manual CPAP titration in terms of acceptance, adherence, treatment time, and functional improvements (7). Although auto-CPAP titration is non-inferior to manual CPAP titration, the former still takes weeks to achieve adequate pressure even under the modern ventilator mode (8). Drug-induced sleep endoscopy (DISE)-guided CPAP titration may be a more efficient method to solve this challenge. DISE-guided CPAP takes less than an hour to determine the adequate CPAP pressure for patients with OSA. Additionally, Civelek et al. (9) showed that the results of DISE-guided and traditional CPAP titrations were not significantly different. Furthermore, DISE has been used to simulate upper airway obstruction during sleep in terms of the level and degree of obstruction (9–15). DISE also plays an important role in accessing surgical and non-surgical treatment alternatives (16–21). However, it is still uncertain whether patients will benefit from the DISE procedure before CPAP treatment, which explores the CPAP titration level, surgical plan, and alternative treatments.
No study has compared the results of DISE-guided and doctor-guided CPAP titrations. Therefore, we conducted a randomized prospective controlled crossover trial to examine the differences between DISE-guided and doctor-guided CPAP titrations. Additionally, the relationship between CPAP pressure and location and patterns of upper airway obstruction during DISE has seldom been studied (10, 14, 15). The second primary endpoint was the identification of the independent location and pattern of upper airway collapse associated with CPAP pressure. Additionally, we also described the complication rate of the DISE procedure for safety concerns.
This prospective, randomized, single-blind, controlled, and crossover study was performed at the sleep center of a tertiary hospital (Chang Gung Memorial Hospital) from March 2018 to December 2019. The local ethics committee of the Chang Gung Memorial Hospital approved the research protocol (NCT03523013), and each patient provided written informed consent.
Outpatients with moderate-to-severe OSA were enrolled in the thoracic medicine department of Chang Gung Memorial Hospital. Polysomnography was performed in all patients using standard techniques. Sleep stage and arousal were scored according to the American Academy of Sleep Medicine criteria (22). All participants were naïve to CPAP therapy at the start of the study. The inclusion criteria were apnea-hypopnea index (AHI) of >15 events/h and Epworth sleepiness scale (ESS) score > 10. The exclusion criteria were central or mixed type sleep apnea, age < 20 years, severe asthma, acute asthma attack, chronic obstructive pulmonary disease (COPD), bronchiectasis, congestive heart failure Fc IV, neuromuscular disease, chronic respiratory failure, American Society of Anesthesiologists class > 3, allergy to dexmedetomidine, and history of upper airway surgery, such as uvulopalatopharyngoplasty.
This study investigated the effect of CPAP treatment with either DISE-guided CPAP titration or conventional sleep center (CSC) CPAP titration in patients with moderate-to-severe OSA. The protocol used in this study is illustrated in Figure 1. Randomization was conducted immediately after patients agreed to participate in the study. All patients underwent DISE-guided CPAP titration approximately 2 weeks later. Patients in the CSC CPAP titration group underwent auto-CPAP titration first if not contraindicated, such as those with congestive heart failure Fc IV, COPD, or neuromuscular disease; if they could not tolerate auto-CPAP titration, an overnight CPAP titration in the sleep center was arranged. Doctors determined the upper limit of the pressure auto-CPAP based on their experiences, such as body mass index (BMI), craniofacial features, and comorbidities such as allergic rhinitis or rhinosinusitis. All patients tolerated CPAP treatment in this study. In contrast, the upper limit of the pressure of the DISE-guided CPAP titration was determined during DISE. According to randomization, patients were grouped into groups A and B. In group A, patients received auto-CPAP treatment (RESMED Air Sense 10 Autoset CPAP, Bella Vista, Australia) with DISE-guided CPAP titration pressure as the upper limit of pressure for the first month. The upper limit of pressure was then modified to the CSC CPAP titration pressure for the second month. In group B, patients received CSC CPAP titration pressure for the first month. The upper limit of pressure was then shifted to the DISE-guided CPAP titration pressure for the second month. Patients were blinded to the CPAP pressure setting. Each patient was fitted with a CPAP mask and provided with instructions on CPAP operation. All patients underwent cognitive behavior therapy to maximize compliance with the CPAP therapy. All patients returned to outpatient clinics every 2 weeks to check the pressure setting. ESS score was recorded at baseline 1 month post CPAP treatment, and 2 months post CPAP treatment.
Our DISE protocol has been discussed in detail previously (13). In brief, patients were placed in the supine position. One nasal cavity was anesthetized using topical xylocaine jelly. The bispectral index (BIS) (version 3.11, Aspect Medical Systems, Inc., Newton, MA, USA), electrocardiogram, blood pressure, and oxygen saturation were monitored. Drug-induced sleep was achieved using an intravenous administration of dexmedetomidine at a rate of 0.5 mcg/ (kg. h), after an initial loading dose of 1 mcg/kg, infused over 10 min. The infusion rate of dexmedetomidine was adjusted up and down by 0.2 mcg/(kg.h) to maintain the BIS level between 65 and 75. Additionally, electroencephalography (EEG), electrooculogram, and electromyography were used to monitor the sleep stages of participants during DISE. Upper airway patterns of patients were first evaluated in an awake state before dexmedetomidine injection was administered. The tip of the endoscope was held in the velopharynx during the induction of sedation to avoid further irritation. DISE was performed under a stable N2 sleep stage, with a BIS level between 65 and 75. The video-recorded examination included sequential observations of the velum, oropharynx, tongue base, and epiglottis. The CPAP pressure was increased by at least 1 cm of H2O at intervals of more than 2 min to eliminate obstructive apnea or hypopnea. These were determined by the simultaneous observation of flow on the CPAP mode of the bi-level positive airway pressure ventilator (Philips Respironics Trilogy 100 Ventilator Murraysville, PA, USA) and the DISE monitor screen. Apnea was defined as cessation of flow (from ventilator screen) or complete obstruction of DISE for more than 10 s. Hypopnea was defined as a 50% reduction in flow for more than 10 s, 30% reduction followed by arousal, or >3% decrease in oxygen saturation. After an adequate CPAP pressure level was achieved, sequential observations of the velum, oropharynx, tongue base, and epiglottis were repeated using the video-recorded examination.
The VOTE classification system was used to characterize DISE findings. It focuses on specific upper airway structures that may contribute to obstruction (velum, oropharynx, tongue base, and epiglottis). At each anatomical level, the configuration of the obstruction was described as anteroposterior, lateral, or concentric. The degree of obstruction had a score for each structure: no obstruction (typically with no vibration of the involved structures); partial obstruction (typically with vibration, 25–75%); and complete obstruction (typically with total or near-total obstruction, > 75%) (11, 15). The consensus scores were determined by two investigators who were blinded to each other. They reassessed the disagreements and reached a new consensus.
Data are expressed as group percentages (categorical variables) or mean ± standard error (continuous variables). Categorical variables were compared using the chi-square or Fisher's exact test, where appropriate. The Wilcoxon matched signed-rank test was used for paired samples with non-normal distributions (all P < 0.05). The Pearson product correlation coefficient was used to examine correlations between variables and AHI or 95% CPAP pressure. Multivariate linear regression analysis was used to determine independent factors for AHI or 95% CPAP pressure. All analyses were performed using the Statistical Package for the Social Sciences software version 13.0 and Prism 5, and a value of P < 0.05 was considered to indicate statistical significance.
The baseline patient characteristics are presented in Table 1. Twenty-four patients with moderate-to-severe OSA were recruited for this study. The patients included 22 men and two women, with an average age of 47.5 ± 2.3 years, baseline AHI of 60.4 ± 5.8 events/h, and BMI of 29.4 ± 1 kg/m2. Physical features such as neck circumference, Friedman tongue position (FTP), and tonsil size are listed in Supplementary Table S1. Twenty-two patients (91.7%) had high tongue positions (FTP IV).
The VOTE grading during DISE for both sleep and CPAP application is presented in Table 2. During sleep, 100% of the velum collapsed, with most cases involving a complete concentric collapse (18/24, 75%). Additionally, the CPAP application led to the resolution of a complete collapse in 18/18 (100%) of patients. Moreover, 16/24 (66.7%) of patients had a complete lateral collapse of the oropharynx. Considering the anteroposterior collapse of the tongue base, 16/24 (66.7%) patients had a complete collapse, while 4/24 (16.7%) patients had a partial collapse. CPAP application also led to the resolution of the complete collapse in 16/16 (100%) of patients. However, 15/24 (62.5%) of patients showed a partial collapse of the tongue base. In contrast, only 7/24 (29.2%) of patients still showed a partial collapse of the velum. For the epiglottis, both partial and complete lateral collapse of the epiglottis was observed in 33.3% of cases, which were all resolved by CPAP application. In contrast, both partial and complete anteroposterior collapse of the epiglottis was observed in 45.8% of patients; however, 25% partial collapse remained even after CPAP application.
The mean CSC CPAP titration pressure was 13.5 ± 0.5, whereas the mean DISE-guided CPAP titration pressure was 13.9 ± 0.7. There were no statistically significant differences between the CSC CPAP and DISE-guided CPAP titration pressure values (Table 3). After 1 month of CPAP treatment, the 95% CPAP pressure recorded from the CPAP mechanism also showed no significant differences between the two groups. Additionally, the residual AHI and compliance were not significantly different between the two groups. ESS score significantly improved from 12.5 ± 1 to 7.5 ± 0.8 in the CSC CPAP pressure group (Table 3). Additionally, ESS score significantly improved from 11.2 ± 1 to 8.3 ± 0.8 in the DISE-guided CPAP titration pressure group. The improvement in ESS score was not significantly different between the two groups.
The 95% CPAP pressure in the epiglottis-anterioposterior (epiglottis-AP) group was significantly higher than that in the oropharynx-lateral (oropharynx-L) group (12.1± 0.5 vs. 10.5± 0.5; p = 0.04). The 95% CPAP pressure in the epiglottis-AP group was higher than that in the velum-anterioposterior (velum-AP) group (12.1 ± 0.5 vs. 9.6 ± 1.1; p = 0.08) (Supplement Figure S1). In the univariate analysis, both epiglottis-AP and tongue base significantly correlated with 95% CPAP pressure (Table 4); however, they did not correlate with velum-AP, velum-lateral (velum-L), velum-concentric (velum-C), oropharynx-L, and epiglottis-lateral. Although tongue base and epiglottis-AP were chosen for analysis in the multivariate stepwise linear regression, only epiglottis-AP was an independent factor for 95% CPAP pressure.
The incidence of bradycardia (<60 beats per min) was relatively high (14/24 patients, 58.3%) (Table 5). In contrast, the incidences of hypotension and hypoxemia were relatively low. All patients who presented with bradycardia, hypoxemia, or hypotension have recovered with proper management. None of the patients had intubation or even mortality.
Table 5. Proportions of bradycardia, hypoxemia, and hypotension during drug-induced sleep endoscopy (DISE).
The present study demonstrated that treating patients with moderate-to-severe OSA with CPAP significantly reduced subjective daytime sleepiness in both groups. There were no significant differences in CPAP pressure, residual AHI, compliance, and ESS between the two groups. Notably, epiglottis (anterior-posterior collapse) was an independent factor for 95% CPAP pressure. Hemodynamic and respiratory parameters were stable during DISE. Although some bradycardia events were noted, no cases of clinically significant bradycardia were observed in the present study. This is the first prospective, randomized controlled, single-blind, and crossover study to compare the effects of DISE-guided and CSC CPAP pressure determination on subjective daytime sleepiness in patients with moderate-to-severe OSA.
To the best of our knowledge, the current study was the first to demonstrate that there are no differences between DISE-guided CPAP and CSC CPAP in terms of titration pressure, subjective daytime sleepiness, residual AHI, and compliance. Initially, we assumed that DISE-guided CPAP titration was more efficient to titrate CPAP pressure than CSC CPAP pressure. The first step in CSC CPAP titration is auto-CPAP titration, if patients do not have any contraindications. The titration from auto-CPAP requires weeks to achieve adequate pressure even under the modern ventilator mode (8). The population of enrolled patients with moderate-to-severe OSA may be the main reason for the negative results. Sleepiness and high severity of OSA are associated with better compliance with CPAP treatment in patients (23, 24). Additionally, those with central and mixed type sleep apnea were also excluded, which may result in better compliance with auto-CPAP.
The DISE-guided CPAP titration may also offer an evaluation of the upper airway while patients are struggling with CPAP. It is important to evaluate whether the downstream flows from CPAP further push the epiglottis down into the laryngeal inlet (25–28). Previous studies have reported a wide variation in the prevalence of epiglottis collapse in patients with OSA. The prevalence ranges from 29% to 73,5% (29–31). This study revealed a 79.2% prevalence of epiglottis collapse in patients with moderate-to-severe OSA, mainly because the definition of epiglottis collapse in the current study includes both tongue-related and isolated epiglottic collapse. Additionally, 91% of patients in the present study showed tongue-related posterior displacement of the epiglottis. Similar to our results, Lin et al. (30) reported that epiglottic collapse in 94.9% of patients was tongue related. While floppy epiglottis is the etiology of isolated epiglottic collapse (32), some studies have revealed that CPAP pressure may further push the epiglottis down into the laryngeal inlet (25–27). DISE-guided CPAP titration may also be used to observe whether the epiglottis is pushed into the laryngeal inlet. However, the CPAP pressure did not further push the epiglottis down into the laryngeal inlet during DISE-guided CPAP titration in the other 9% of patients with the isolated epiglottic collapse in this study. This is another reason for the negative results of this study.
Regarding the site of collapse at atmospheric pressure for predicting CPAP pressure, 95% CPAP pressure in the epiglottis-AP group was significantly higher than that in the oropharynx-L group (12.1 ± 0.5 vs. 10.5 ± 0.5; p = 0.04). Additionally, only epiglottis-AP was an independent factor for 95% CPAP pressure in the multivariate stepwise linear regression, which is compatible with the findings of a previous study that found that CPAP pressure had the greatest impact on the lateral collapse of the oropharynx, and then, the velopharynx. The tongue base and epiglottis collapse were more resistant to CPAP pressure (10). However, another study revealed that velum-C and oropharynx-L were associated with CPAP titration pressure (15). This was possible because the patient populations were different. Most patients in the current study had severe OSA with a high AHI (60 events/h). Furthermore, the sample size of this study was relatively small. Further studies are warranted to confirm these results. Twenty-two patients (91.7%) had a high tongue position (FTP IV) in the current study, as well as many complete concentric collapses. A recent study identified a positive correlation between higher tongue position and complete concentric collapse, which may elucidate the findings of this study (33).
One strength of this study is the controlled sedation level according to both BIS (BIS 65–75) and EEG (N2 sleep stage). Another strength of this study is the use of dexmedetomidine, which has less influence on upper airway muscles (34) and better hemodynamic and respiratory stability during DISE. Although dose-related bradycardia due to dexmedetomidine has been reported (35), no clinically significant bradycardia was observed in this study. Controlling sedative depth using an objective tool was critical in this study. Different BIS levels are associated with different degrees of upper airway obstruction (36). Our previous study also evaluated upper airway obstruction at different sedative depths (BIS 50–60 for deep sedation vs. BIS 65–75 for light sedation) (13). We found that greater sedative depth increased upper airway obstruction. Most importantly, dexmedetomidine had less effect on the upper airway muscles and did not result in significant respiratory depression, making it suitable for DISE and bronchoscopy (34, 37–41). However, dexmedetomidine still had less effect, which may be revealed by the difference in titration pressure between auto- and DISE-guided CPAP titration. According to four randomized control trials (42–45) and three clinical series (46), the titration pressure between auto- and manual CPAP titration is generally 1–2 cm of H2O. Therefore, the delta titration pressure (3.8 cm of H2O) between auto- and DISE-guided CPAP titration is still larger than 1–2 cm of H2O. This may be due to the sedative agents used during the DISE. We selected dexmedetomidine rather than propofol because dexmedetomidine causes less dynamic collapse than propofol (47–50). However, the anteroposterior diameter of the retroglossal airway during high-dose dexmedetomidine infusion significantly decreased compared to that during low-dose dexmedetomidine infusion (51), which indicates that dexmedetomidine might still increase upper airway collapsibility and then increase the DISE-guided CPAP titration level.
This study was limited by its small and selected sample size, and larger studies are warranted. In addition, the study included more male patients than female patients. Therefore, the results of the present study should be interpreted with caution. Additionally, the study population mainly presented with severe OSA (AHI 60 events/h). Therefore, the results of this study may not apply to patients with mild OSA. Furthermore, this study assessed the effect of CPAP over a brief period only (1 month). Thus, further research using a larger number of individuals with a longer evaluation period is warranted.
In conclusion, the two modalities are comparable in terms of establishing the pressure settings required to treat patients. Further large-scale studies are required to confirm these results.
The datasets presented in this article are not readily available because of patient confidentiality and participant privacy. Requests to access the datasets should be directed to TYW (d2FuZzU1MzFAZ21haWwuY29t).
The studies involving human participants were reviewed and approved by the local Ethics Committee of Chang Gung Memorial Hospital, research protocol NCT03523013. The patients/participants provided their written informed consent to participate in this study.
TYW and YLL designed this study. TYW, YCH, and YLL enrolled the patients and organized the database. TYW, YCH, and TYL were responsible for data analysis. TYW, YLN, and TYL performed statistical analyses. TYW contributed to the data interpretation and manuscript drafting. YLL contributed to the critical review and interpretation of data. YLL supervised the project, participated in its coordination, and helped to draft the manuscript. All authors have read and approved the final manuscript.
This study was supported by a research grant from Chang Gung Memorial Hospital, Taiwan (CMRPG3H0381) and the Ministry of Science and Technology (MOST−108-2320-B-182-027). The funders had no role in the design of this study; the collection, analysis, and interpretation of data, or the writing of this manuscript.
The authors declare that the research was conducted in the absence of any commercial or financial relationships that could be construed as a potential conflict of interest.
All claims expressed in this article are solely those of the authors and do not necessarily represent those of their affiliated organizations, or those of the publisher, the editors and the reviewers. Any product that may be evaluated in this article, or claim that may be made by its manufacturer, is not guaranteed or endorsed by the publisher.
The Supplementary Material for this article can be found online at: https://www.frontiersin.org/articles/10.3389/fneur.2022.882465/full#supplementary-material
OSA, Obstructive sleep apnea; CSC, Conventional sleep center; CPAP, Continuous positive airway pressure ventilation; DISE, Drug-induced sleep endoscopy; ESS, Epworth sleepiness scale; AHI, Apnea-hypopnea index; COPD, chronic obstructive pulmonary disease; BIS, bispectral index; EEG, Electroencephalography; BMI, body mass index; velum-AP, Velum-anterioposterior; velum-C, Velum-concentric; oropharynx-L, Oropharynx-lateral; epiglottis-AP, Epiglottis-anterioposterior.
1. Nieto FJ, Young TB, Lind BK, Shahar E, Samet JM, Redline S, et al. Association of sleep-disordered breathing, sleep apnea, and hypertension in a large community-based study. sleep heart health study. JAMA. (2000) 283:1829–36. doi: 10.1001/jama.283.14.1829
2. Hung J, Whitford EG, Parsons RW, Hillman DR. Association of sleep apnoea with myocardial infarction in men. Lancet. (1990) 336:261–4. doi: 10.1016/0140-6736(90)91799-G
3. Wessendorf TE, Thilmann AF, Wang YM, Schreiber A, Konietzko N, Teschler H. Fibrinogen levels and obstructive sleep apnea in ischemic stroke. Am J Respir Crit Care Med. (2000) 162:2039–42. doi: 10.1164/ajrccm.162.6.2001048
4. Redline S, Strauss ME, Adams N, Winters M, Roebuck T, Spry K, et al. Neuropsychological function in mild sleep-disordered breathing. Sleep. (1997) 20:160–7. doi: 10.1093/sleep/20.2.160
5. Kribbs NB, Pack AI, Kline LR, Smith PL, Schwartz AR, Schubert NM, et al. Objective measurement of patterns of nasal CPAP use by patients with obstructive sleep apnea. Am Rev Respir Dis. (1993) 147:887–95. doi: 10.1164/ajrccm/147.4.887
6. Heinzer RC, Stanchina ML, Malhotra A, Jordan AS, Patel SR, Lo YL, et al. Effect of increased lung volume on sleep disordered breathing in patients with sleep apnoea. Thorax. (2006) 61:435–9. doi: 10.1136/thx.2005.052084
7. Rosen CL, Auckley D, Benca R, Foldvary-Schaefer N, Iber C, Kapur V, et al. A multisite randomized trial of portable sleep studies and positive airway pressure autotitration versus laboratory-based polysomnography for the diagnosis and treatment of obstructive sleep apnea: the HomePAP study. Sleep. (2012) 35:757–67. doi: 10.5665/sleep.1870
8. Johnson KG, Johnson DC. Treatment of sleep-disordered breathing with positive airway pressure devices: technology update. Med Devices. (2015) 8:425–37. doi: 10.2147/MDER.S70062
9. Civelek S, Emre IE, Dizdar D, Cuhadaroglu C, Eksioglu BK, Eraslan AK, et al. Comparison of conventional continuous positive airway pressure to continuous positive airway pressure titration performed with sleep endoscopy. Laryngoscope. (2012) 122:691–5. doi: 10.1002/lary.22494
10. Torre C, Liu SY, Kushida CA, Nekhendzy V, Huon LK, Capasso R. Impact of continuous positive airway pressure in patients with obstructive sleep apnea during drug-induced sleep endoscopy. Clin Otolaryngol. (2017) 42:1218–23. doi: 10.1111/coa.12851
11. Kezirian EJ, Hohenhorst W, de Vries N. Drug-induced sleep endoscopy: the VOTE classification. Eur Arch Otorhinolaryngol. (2011) 268:1233–6. doi: 10.1007/s00405-011-1633-8
12. E DEC, Fiorita A, Rizzotto G, Mennuni GF, Meucci D, Giuliani M, et al. The role of drug-induced sleep endoscopy in the diagnosis and management of obstructive sleep apnoea syndrome: our personal experience. Acta Otorhinolaryngol Ital. (2013) 33:405–13.
13. Lo YL, Ni YL, Wang TY, Lin TY, Li HY, White DP, et al. Bispectral index in evaluating effects of sedation depth on drug-induced sleep endoscopy. J Clin Sleep Med. (2015) 11:1011–20. doi: 10.5664/jcsm.5016
14. Jung SH, Koo SK, Choi JW, Moon JS, Lee SH. Upper airway structural changes induced by CPAP in OSAS patients: a study using drug-induced sleep endoscopy. Eur Arch Otorhinolaryngol. (2017) 274:247–52. doi: 10.1007/s00405-016-4233-9
15. Lan MC, Hsu YB, Lan MY, Huang YC, Kao MC, Huang TT, et al. The predictive value of drug-induced sleep endoscopy for CPAP titration in OSA patients. Sleep Breath. (2018) 22:949–54. doi: 10.1007/s11325-017-1600-8
16. Croft CB, Pringle M. Sleep nasendoscopy: a technique of assessment in snoring and obstructive sleep apnoea. Clin Otolaryngol Allied Sci. (1991) 16:504–9. doi: 10.1111/j.1365-2273.1991.tb01050.x
17. Hewitt RJ, Dasgupta A, Singh A, Dutta C, Kotecha BT. Is sleep nasendoscopy a valuable adjunct to clinical examination in the evaluation of upper airway obstruction? Eur Arch Otorhinolaryngol. (2009) 266:691–7. doi: 10.1007/s00405-008-0831-5
18. Kotecha BT, Hannan SA, Khalil HM, Georgalas C, Bailey P. Sleep nasendoscopy: a 10-year retrospective audit study. Eur Arch Otorhinolaryngol. (2007) 264:1361–7. doi: 10.1007/s00405-007-0366-1
19. Kastoer C, Op de., Beeck S, Dom M, Neirinckx T, Verbraecken J, Braem MJ, et al. Drug-induced sleep endoscopy upper airway collapse patterns and maxillomandibular advancement. Laryngoscope. (2019) 130:E268–74. doi: 10.1002/lary.28022
20. Wilcox LJ, Bergeron M, Reghunathan S, Ishman SL. An updated review of pediatric drug-induced sleep endoscopy. Laryngoscope Investig Otolaryngol. (2017) 2:423–31. doi: 10.1002/lio2.118
21. Hsu YS, Jacobowitz O. Does sleep endoscopy staging pattern correlate with outcome of advanced palatopharyngoplasty for moderate to severe obstructive sleep apnea? J Clin Sleep Med. (2017) 13:1137–44. doi: 10.5664/jcsm.6756
22. Berry RB, Brooks R, Gamaldo C, Harding SM, Lloyd RM, Quan SF, et al. AASM scoring manual updates for 2017 (Version 2.4). J Clin Sleep Med. (2017) 13:665–6. doi: 10.5664/jcsm.6576
23. McArdle N, Devereux G, Heidarnejad H, Engleman HM, Mackay TW, Douglas NJ. Long-term use of CPAP therapy for sleep apnea/hypopnea syndrome. Am J Respir Crit Care Med. (1999) 159:1108–14. doi: 10.1164/ajrccm.159.4.9807111
24. Sin DD, Mayers I, Man GC, Pawluk L. Long-term compliance rates to continuous positive airway pressure in obstructive sleep apnea: a population-based study. Chest. (2002) 121:430–5. doi: 10.1378/chest.121.2.430
25. Chetty KG, Kadifa F, Berry RB, Mahutte CK. Acquired laryngomalacia as a cause of obstructive sleep apnea. Chest. (1994) 106:1898–9. doi: 10.1378/chest.106.6.1898
26. Andersen AP, Alving J, Lildholdt T, Wulff CH. Obstructive sleep apnea initiated by a lax epiglottis. a contraindication for continuous positive airway pressure. Chest. (1987) 91:621–3. doi: 10.1378/chest.91.4.621
27. Verse T, Pirsig W. Age-related changes in the epiglottis causing failure of nasal continuous positive airway pressure therapy. J Laryngol Otol. (1999) 113:1022–5. doi: 10.1017/S0022215100145888
28. Li HY, Lee LA, Tsai MS, Chen NH, Chuang LP, Fang TJ, et al. How to manage continuous positive airway pressure (CPAP) failure -hybrid surgery and integrated treatment. Auris Nasus Larynx. (2020). doi: 10.1016/j.anl.2020.03.007
29. Koutsourelakis I, Safiruddin F, Ravesloot M, Zakynthinos S, de Vries N. Surgery for obstructive sleep apnea: sleep endoscopy determinants of outcome. Laryngoscope. (2012) 122:2587–91. doi: 10.1002/lary.23462
30. Lin HS, Rowley JA, Badr MS, Folbe AJ, Yoo GH, Victor L, et al. Transoral robotic surgery for treatment of obstructive sleep apnea-hypopnea syndrome. Laryngoscope. (2013) 123:1811–6. doi: 10.1002/lary.23913
31. Kezirian EJ, White DP, Malhotra A, Ma W, McCulloch CE, Goldberg AN. Interrater reliability of drug-induced sleep endoscopy. Arch Otolaryngol Head Neck Surg. (2010) 136:393–7. doi: 10.1001/archoto.2010.26
32. Azarbarzin A, Marques M, Sands SA, Op de Beeck S, Genta PR, Taranto-Montemurro L, et al. Predicting epiglottic collapse in patients with obstructive sleep apnoea. Eur Respir J. (2017) 50:1700345. doi: 10.1183/13993003.00345-2017
33. Zhao C, Viana A, Ma Y, Capasso R. High tongue position is a risk factor for upper airway concentric collapse in obstructive sleep apnea: observation through sleep endoscopy. Nat Sci Sleep. (2020) 12:767–74. doi: 10.2147/NSS.S273129
34. Chang ET, Certal V, Song SA, Zaghi S, Carrasco-Llatas M, Torre C, et al. Dexmedetomidine versus propofol during drug-induced sleep endoscopy and sedation: a systematic review. Sleep Breath. (2017) 21:727–35. doi: 10.1007/s11325-017-1465-x
35. Shehabi Y, Ruettimann U, Adamson H, Innes R, Ickeringill M. Dexmedetomidine infusion for more than 24 hours in critically ill patients: sedative and cardiovascular effects. Intensive Care Med. (2004) 30:2188–96. doi: 10.1007/s00134-004-2417-z
36. Hong SD, Dhong HJ, Kim HY, Sohn JH, Jung YG, Chung SK, et al. Change of obstruction level during drug-induced sleep endoscopy according to sedation depth in obstructive sleep apnea. Laryngoscope. (2013) 123:2896–9. doi: 10.1002/lary.24045
37. Ehsan Z, Mahmoud M, Shott SR, Amin RS, Ishman SL. The effects of anesthesia and opioids on the upper airway: a systematic review. Laryngoscope. (2016) 126:270–84. doi: 10.1002/lary.25399
38. Venn RM, Hell J, Grounds RM. Respiratory effects of dexmedetomidine in the surgical patient requiring intensive care. Crit Care. (2000) 4:302–8. doi: 10.1186/cc712
39. Ryu JH, Lee SW, Lee JH, Lee EH, Do SH, Kim CS. Randomized double-blind study of remifentanil and dexmedetomidine for flexible bronchoscopy. Br J Anaesth. (2012) 108:503–11. doi: 10.1093/bja/aer400
40. Shteamer JW, Dedhia RC. Sedative choice in drug-induced sleep endoscopy: a neuropharmacology-based review. Laryngoscope. (2017) 127:273–9. doi: 10.1002/lary.26132
41. Kuyrukluyildiz U, Binici O, Onk D, Ayhan Celik S, Torun MT, Unver E, et al. Comparison of dexmedetomidine and propofol used for drug-induced sleep endoscopy in patients with obstructive sleep apnea syndrome. Int J Clin Exp Med. (2015) 8:5691–8.
42. Lloberes P, Ballester E, Montserrat JM, Botifoll E, Ramirez A, Reolid A, et al. Comparison of manual and automatic CPAP titration in patients with sleep apnea/hypopnea syndrome. Am J Respir Crit Care Med. (1996) 154:1755–8. doi: 10.1164/ajrccm.154.6.8970366
43. Stradling JR, Barbour C, Pitson DJ, Davies RJ. Automatic nasal continuous positive airway pressure titration in the laboratory: patient outcomes. Thorax. (1997) 52:72–5. doi: 10.1136/thx.52.1.72
44. Teschler H, Berthon-Jones M, Thompson AB, Henkel A, Henry J, Konietzko N. Automated continuous positive airway pressure titration for obstructive sleep apnea syndrome. Am J Respir Crit Care Med. (1996) 154:734–40. doi: 10.1164/ajrccm.154.3.8810613
45. Teschler H, Farhat AA, Exner V, Konietzko N, Berthon-Jones M. AutoSet nasal CPAP titration: constancy of pressure, compliance and effectiveness at 8 month follow-up. Eur Respir J. (1997) 10:2073–8. doi: 10.1183/09031936.97.10092073
46. Berkani M, Lofaso F, Chouaid C, Pia d'Ortho M, Theret D, Grillier-Lanoir V, et al. CPAP titration by an auto-CPAP device based on snoring detection: a clinical trial and economic considerations. Eur Respir J. (1998) 12:759–63. doi: 10.1183/09031936.98.12040759
47. Mahmoud M, Jung D, Salisbury S, McAuliffe J, Gunter J, Patio M, et al. Effect of increasing depth of dexmedetomidine and propofol anesthesia on upper airway morphology in children and adolescents with obstructive sleep apnea. J Clin Anesth. (2013) 25:529–41. doi: 10.1016/j.jclinane.2013.04.011
48. Mahmoud M, Gunter J, Donnelly LF, Wang Y, Nick TG, Sadhasivam S, et al. comparison of dexmedetomidine with propofol for magnetic resonance imaging sleep studies in children. Anesth Analg. (2009) 109:745–53. doi: 10.1213/ane.0b013e3181adc506
49. Cortinez LI, Hsu YW, Sum-Ping ST, Young C, Keifer JC, Macleod D, et al. Dexmedetomidine pharmacodynamics: Part II: Crossover comparison of the analgesic effect of dexmedetomidine and remifentanil in healthy volunteers. Anesthesiology. (2004) 101:1077–83. doi: 10.1097/00000542-200411000-00006
50. Hsu YW, Cortinez LI, Robertson KM, Keifer JC, Sum-Ping ST, Moretti EW, et al. Dexmedetomidine pharmacodynamics: part I: crossover comparison of the respiratory effects of dexmedetomidine and remifentanil in healthy volunteers. Anesthesiology. (2004) 101:1066–76. doi: 10.1097/00000542-200411000-00005
Keywords: CPAP, OSA, outcome, CPAP pressure, drug-induced sleep endoscopy (DISE)
Citation: Wang T-Y, Huang Y-C, Lin T-Y, Ni Y-L and Lo Y-L (2022) Outcome of CPAP Titration for Moderate-to-Severe OSA Under Drug-Induced Sleep Endoscopy: A Randomized Controlled Crossover Trial. Front. Neurol. 13:882465. doi: 10.3389/fneur.2022.882465
Received: 23 February 2022; Accepted: 02 May 2022;
Published: 13 June 2022.
Edited by:
Shazia M. Jamil, Scripps Clinic, United StatesReviewed by:
Jeremy Orr, University of California, San Diego, United StatesCopyright © 2022 Wang, Huang, Lin, Ni and Lo. This is an open-access article distributed under the terms of the Creative Commons Attribution License (CC BY). The use, distribution or reproduction in other forums is permitted, provided the original author(s) and the copyright owner(s) are credited and that the original publication in this journal is cited, in accordance with accepted academic practice. No use, distribution or reproduction is permitted which does not comply with these terms.
*Correspondence: Yu-Lun Lo, bG95dWx1bkBob3RtYWlsLmNvbQ==
Disclaimer: All claims expressed in this article are solely those of the authors and do not necessarily represent those of their affiliated organizations, or those of the publisher, the editors and the reviewers. Any product that may be evaluated in this article or claim that may be made by its manufacturer is not guaranteed or endorsed by the publisher.
Research integrity at Frontiers
Learn more about the work of our research integrity team to safeguard the quality of each article we publish.