- 1Department of Radiology, The Second Affiliated Hospital and Yuying Children's Hospital of Wenzhou Medical University, Wenzhou, China
- 2Wenzhou Key Laboratory of Basic Science and Translational Research of Radiation Oncology, Wenzhou, China
Inflammation-based scores have been increasingly used for prognosis prediction in neurological diseases. This study aimed to investigate the predictive value of inflammation-based scores combined with radiological characteristics in children with moderate or severe traumatic brain injury (MS-TBI). A total of 104 pediatric patients with MS-TBI were retrospectively enrolled and randomly divided into training and validation cohorts at a 7:3 ratio. Univariate and multivariate logistic regression analyses were performed to identify independent predictors of prognosis in pediatric patients with MS-TBI. A prognostic nomogram was constructed, and its predictive performance was validated in both the training and validation cohorts. Sex, admission platelet-to-lymphocyte ratio, and basal cistern status from initial CT findings were identified as independent prognostic predictors for children with MS-TBI in multivariate logistic analysis. Based on these findings, a nomogram was then developed and its concordance index values were 0.918 [95% confidence interval (CI): 0.837–0.999] in the training cohort and 0.86 (95% CI: 0.70–1.00) in the validation cohort, which significantly outperformed those of the Rotterdam, Marshall, and Helsinki CT scores. The proposed nomogram, based on routine complete blood count and initial CT scan findings, can contribute to individualized prognosis prediction and clinical decision-making in children with MS-TBI.
Introduction
Traumatic brain injury (TBI) is a major social and clinical issue because it is one of the leading causes of mortality or permanent disability in children and adolescents worldwide (1). Pediatric TBI has exhibited various unique characteristics compared with adult TBI, which may be explained by age-related anatomical and physiological differences (2). While the clinical management of TBI has improved significantly in recent years, children with moderate or severe TBI (MS-TBI) continue to have poor clinical outcomes (2).
Predicting outcomes is critical for satisfactory pediatric TBI care. Undeniably, individual prognosis prediction contributes to improved clinical decision-making and helps minimize secondary brain injury (SBI). Computerized tomography (CT) is routinely performed to evaluate structural lesions in pediatric TBI because of its availability and speed. Indeed, it has been studied for its predictive value for mortality and functional outcomes in both individual injury characteristics and composite grading systems, including the Rotterdam CT score (3), Marshall CT classification (4), and Helsinki CT scoring system (5). However, few studies have comprehensively examined and compared the predictive values of admission CT imaging characteristics in pediatric TBI.
Hemodynamic alterations and systemic inflammation are always present in patients who sustain TBI (6). Additionally, complete blood counts are frequently performed as non-invasive laboratory procedures in clinical practice. Peripheral blood counts, including neutrophil, lymphocyte, and platelet counts, have previously been used to assess central nervous system and peripheral inflammation following injury (7). Recently, several inflammation-based prognostic scores, including the neutrophil-to-lymphocyte ratio (NLR) (8), platelet-to-lymphocyte ratio (PLR) (9), lymphocyte-to-monocyte ratio (LMR) (10) and systemic immune-inflammation index (SII) (11) have been demonstrated to accurately predict outcomes in patients with neurological diseases such as stroke, subarachnoid hemorrhage (SAH), and Alzheimer's disease (12–14). Indeed, these are low-cost and reproducible diagnostics that can be quickly calculated from a blood sample collected under simple laboratory conditions and can identify the mortality risk associated with TBI. However, no study has evaluated the predictive value of these scores in pediatric TBI.
Therefore, the purpose of this study was to retrospectively evaluate and compare the prognostic values of initial CT scan findings and blood test-based inflammation scores in a cohort of 104 children with TBI. Moreover, we established an accessible and easy-to-use nomogram to predict early mortality in pediatric TBI based on intake examinations.
Materials and Methods
Patients
We retrospectively screened 104 pediatric patients with MS-TBI admitted to our hospital, the 2nd Affiliated Hospital of the Wenzhou Medical University Emergency Department, between January 2015 and January 2020. This study was approved by The Research Ethics Committee of the 2nd Affiliated Hospital of Wenzhou Medical University and complied with the standards of the Declaration of Helsinki. Informed consent was obtained from each patient for using their data for research. The inclusion criteria of our study were as follows: (I) history of TBI <24 h, (II) age <15 years, (III) initial CT scan conducted within 24 h of injury, (IV) presence of TBI at hospital admission (Glasgow Coma Scale [GCS]: 3–12). The exclusion criteria were as follows: (I) history of TBI or chronic psychiatric conditions, (II) lack of clinical information and imaging, and (III) presence of multiple trauma. All pediatric patients were assessed and treated according to standard procedures. Early mortality was defined as death at hospital discharge (15, 16). Patients were randomly divided into a training cohort (N = 73) and validation cohort (N = 31) in a 7:3 ratio. The training cohort was used to screen variables and construct the model. The validation cohort was used to validate the results obtained from the training cohort. The flowchart of patient enrollment and scheme for analysis is shown in Figure 1.
Clinical and Radiological Variables
Clinical variables were retrospectively retrieved from the electronic medical records, including patient characteristics (age, sex, and GCS score), blood test results (neutrophil, lymphocyte, monocyte, red blood cell, white blood cell and platelet counts, hemoglobin, hematocrit (HCT), mean corpuscular volume (MCV), red cell distribution width–coefficient of variation (RDW-CV), and radiological variables (hemorrhagic mass volume, intraventricular hemorrhage (IVH), SAH, midline shift, subdural hematoma (SDH), epidural hematoma (EDH), intracerebral hematoma, hemorrhagic brain contusion, and basal cistern status). All blood samples were collected before treatment at admission. All initial CT data obtained within 24 h of injury were evaluated independently by two neuroradiologists. A consensus was used to settle disagreements. CT scans were performed using a GE Light-Speed VCT 64 slice scanner (GE Healthcare, Milwaukee, WI, USA). The CT scan parameters were a tube voltage of 120 kV, automatic tube current modulation, and a layer thickness of 5 mm.
Rotterdam scores are based on the following CT presentations: whether the basal cistern was categorized as normal, compressed, or absent; whether the midline shift was <5 mm or <5 mm; whether EDH was absent or present; and whether SAH/IVH was absent or present. Marshall CT scores use the three CT findings (midline shift, basal cisterns status, and lesion volume) and the type of hemorrhagic mass management (whether it was surgically evacuated or not). Helsinki CT scores were classified according to the level of TBI severity, as evaluated by the authors (Supplementary Table 1). In addition, the inflammation-based prognostic scores including the SII, NLR, PLR, and LMR were calculated and are described in Supplementary Table 2. The optimal threshold value for each score and blood test parameters was determined by performing an analysis of the receiver operating characteristic (ROC) for early mortality status. The value with the maximum Youden index was chosen as the optimal cut-off value.
Statistics
Data are presented as median with interquartile range (IQR) for continuous variables and as counts with percentages for categorical variables. Univariate and multivariate logistic regression analyses were used to identify independent risk factors for early mortality in the training cohort. All variables in the univariate analysis (P < 0.05) were included in the multivariate analysis, and a backward stepwise selection was performed. The nomogram for predicting early mortality was developed based on variables that were considered statistically significant in the multivariate analysis. The ROC analysis and concordance index (C-index) value were applied to measure the discrimination performance of the nomogram in comparison with current CT scoring systems in both the training and validation cohorts.
All statistical analyses were performed using Stata version 15.1 (StataCorp, College Station, TX, USA) and R version 4.1.1. Differences were considered statistically significant when the two-tailed P-value was < 0.05.
Results
Patient Characteristics
The entire cohort consisted of 104 patients (60 male and 44 female) with MS-TBI. The median age of the patients was 6 (IQR: 3.5–8) years. The median GCS score was 7 (IQR: 5–9). The primary reason for trauma was fall from height (75 patients, 72.1%), followed by motor vehicle/motor cycle crashes (27 patients, 26.0%), and violence (2 patients, 1.9%).
Among these pediatric patients, the basal cistern was normal in 18 (17.3%) patients, compressed in 49 (47.1%) patients, and absent in 37 (35.6%) patients. EDH, SDH, and intracerebral hematoma were observed in 71 (68.3%), 49 (47.1%), and 71 (68.3%) patients, respectively. SAH and IVH were present in 98 (94.2%) and 17 (16.3%) patients, respectively. Moreover, the midline shift was > 5 mm in 24 patients (23.1%). Regarding the CT scoring systems, the median values of the Rotterdam, Marshall, and Helsinki CT scores were 4 (IQR: 3–5), 5 (IQR: 2–5), and 3 (IQR: 1–6), respectively. At the time of discharge from the hospital, 86 children survived, while 18 died, resulting in a 17.3% mortality rate. Other clinicopathological characteristics, including blood test variables, are summarized in Table 1.
Risk Factors for Early Mortality in Children With MS-TBI
The optimal cut-off values for blood test parameters were determined by ROC analysis and are presented in Table 2. In the training cohort, our univariate analysis revealed that basal cistern (OR = 6.84, 95% confidence interval [CI]: 1.83–25.59, P = 0.004), sex (OR = 6.15, 95% CI: 1.50–25.23, P = 0.012), MCV (OR = 0.15, 95% CI: 0.04–0.62, P = 0.009), HCT (OR = 0.16, 95% CI: 0.04–0.62, P = 0.008), RDW-CV (OR = 3.69, 95% CI: 1.02–13.37, P = 0.047), NLR (OR = 3.62, 95% CI: 1.01–12.99, P = 0.048), PLR (OR = 9.17, 95% CI: 2.24–37.58, P = 0.002) and LMR (OR = 0.18, 95% CI: 0.04–0.90, P = 0.036) were associated with early mortality in children with MS-TBI. Furthermore, multivariate analysis demonstrated that basal cistern (OR = 22.12, 95% CI: 2.43–201.58, P = 0.006), sex (OR = 8.38, 95% CI: 1.30–54.18, P = 0.026), and PLR (OR = 63.22, 95% CI: 4.50–888.79, P = 0.002) were independent risk factors for early mortality in this population (Table 3).
Development and Validation of the Nomogram
Based on the independent risk factors identified by the multivariate logistic regression analysis in the training cohort, we constructed a nomogram to predict the risk of early mortality in pediatric patients with MS-TBI. Each variable was assigned a score based on its β coefficient (Figure 2). The nomogram had C-index values of 0.918 in the training cohort and 0.86 in the validation cohort (Table 4). The area under the curve (AUC) values demonstrated the excellent predictive ability of our current nomogram [training cohort: AUC = 0.92 (95% CI: 0.84–1.00); validation cohort: AUC = 0.86 (95% CI: 0.70–1.00)], which significantly outperformed the currently used Rotterdam, Marshall, and Helsinki CT scores (Table 4 and Figure 3).
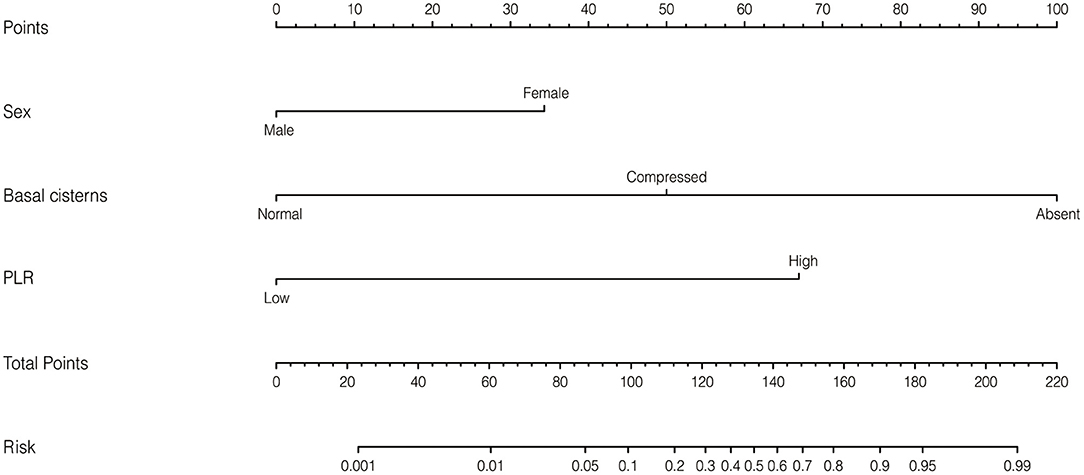
Figure 2. Nomogram to predict early mortality in pediatric patients with moderate or severe traumatic brain injury (MS-TBI).
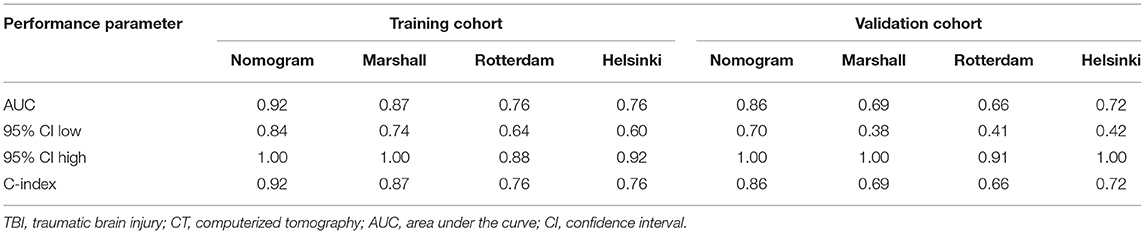
Table 4. Performance of the nomogram in predicting early mortality in pediatric patients with TBI comparing with current CT scoring systems.
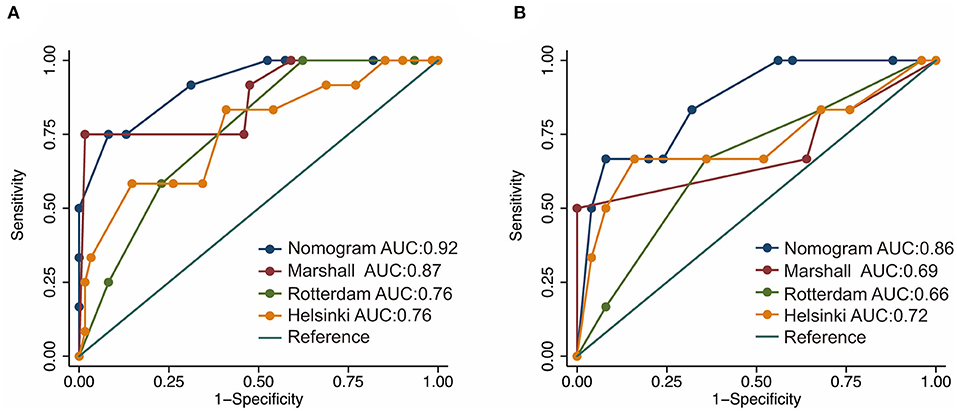
Figure 3. Receiver operating characteristic (ROC) curves of the nomogram and current CT scoring systems in the training (A) and validation cohorts (B).
Optimal Cut-Off Value of the Nomogram
To facilitate the use of the newly developed nomogram, we determined the optimal threshold value of the nomogram to be 135 points based on the ROC analysis of the entire cohort. Patients were divided into low-risk (score ≤ 135 points) and high-risk (score >135 points) groups. In the training cohort, the early mortalities were 5.1% in the low-risk group and 64.3% in the high-risk group. In the validation cohort, the early mortalities were 9.1% in the low-risk group and 44.4% in the high-risk group. Therefore, the current nomogram could serve as an easy-to-use model with good performance for risk discrimination in pediatric patients with MS-TBI.
Discussion
TBI is an injury to the cranium and its intracranial components produced by an external mechanical force, which changes the brain's structure and function (17). Primary and SBI is a pathological process following TBI (18). Primary brain injury occurs when the brain tissue is subjected to mechanical force, resulting in axonal, vascular, and glial cell damage. SBI occurs due to the initiation of inflammatory cascades by the release of different inflammatory chemicals and neurotransmitters from the injured neuronal and glial cells in the brain (19).
Currently, the GCS is the most commonly used technique for assessing the severity of TBI. On the other hand, GCS is a collection of clinical descriptive data that does not include structural information about cerebral abnormalities. Additionally, the GCS had a low prognostic value in the early stages post-injury, and neurological examination in children is challenging. More importantly, a history of neurological illnesses and the presence of an EDH during the intermediate waking state may affect the accuracy of GCS (20). As a result, finding efficient biomarkers for the prognosis of pediatric TBI from admission examinations is critical. The predictive value of inflammation scores in pediatric TBI cases with a combination of intake CT findings is still unclear and has not been extensively explored. To bridge this gap, the present study, for the first time, compared the predictive values of various inflammation prognostic scores and CT scan parameters in a large cohort of 104 children with MS-TBI.
In this study, ROC analysis was applied to determine the optimal cut-off values of various blood test parameters (Table 2). In the training cohort, the univariate and multivariate logistic regression analyses demonstrated that the basal cistern, sex, and PLR could independently predict early death in pediatric patients with MS-TBI (Table 3). To further facilitate clinical practice, we constructed a nomogram for prognosis prediction (Figure 2). The C-index and AUCs demonstrated that the nomogram significantly outperformed current CT scoring systems, including the Rotterdam CT, Marshall CT, and Helsinki CT scores in both the training and validation cohorts (Table 4). All these data demonstrated that the current nomogram could serve as a preferable model for pediatric patients with MS-TBI.
The mechanism underlying the excellent predictive value of the newly developed nomogram may be explained as follows. PLR was initially introduced to represent systemic inflammation and to estimate the prognosis of patients with cancer and autoimmune diseases (21, 22). Recent research has demonstrated that PLR is a simple parameter that can be used to predict clinical outcomes in patients with stroke, cerebral hemorrhage, and SAH (13, 23–26). In response to physiological stress, such as traumatic injury, the body produces more cortisol, whose elevated levels can result in lymphopenia (27); the higher the physiological stress, the higher the cortisol level, which results in a decrease in the body's lymphocyte count. Contrastingly, an increased lymphocyte count indicates a more targeted immune response and a more persistent inflammatory pathway (28). Moreover, lymphocytes represent a more tightly controlled inflammatory route, and apoptosis induced by lymphocytes is less harmful to neighboring cells than other cell death models caused by uncontrolled inflammation (29). Thus, a decreased lymphocyte count post-TBI may reflect the damaging effect of the inflammatory response, resulting in an exacerbated SBI post-TBI. On the other hand, along with their well-known hemostatic functions, platelets play an active role in inflammation regulation. While platelets bind to coagulation factors, they also include a large variety of inflammatory factors, such as TNF-α, interleukins, and serotonin, all of which are involved in tissue injury and repair (7). Therefore, it is reasonable to conclude that a high PLR may be associated with an increased risk of death in pediatric patients with MS-TBI.
Additionally, previous research has demonstrated that obliterated cisterns are associated with increased intracranial pressure and poor outcomes (30, 31), suggesting that the status of the cisterns can be used as an early non-invasive method for identifying patients at high risk of death or severe disability, despite the initial neurological examination indicating otherwise. Further, some studies that focused on adults explored sex differences in outcomes following moderate/severe TBIs, mainly showing an absence of differences or better outcomes in women compared to men. However, following mild TBI, most studies indicate worse psychological and global functioning outcomes in women. Biological differences, particularly sex steroids, represent another pathway that can mediate these sex differences in outcomes. Animal models suggest that the hypothalamic-pituitary-adrenal axis, which modulates the stress response, and microglia, which influence cerebral inflammation, have sex-specific responses. Indeed, these preclinical models have shown that female rodents present a stronger inflammatory response than males after TBI (32–37).
Nevertheless, this study has several limitations that are worth mentioning. First, as a single-center retrospective study, potential selection bias was inevitable, although we had performed strict inclusion criteria. Second, the cut-off values for inflammation-based indices used in this study were determined by ROC analysis based on outcome data, and further validation studies determining optimal threshold values for these inflammation indices are needed. Third, peripheral platelet and lymphocyte counts may be affected by other pathological conditions, including metabolic syndrome and renal dysfunction. Hence, the clinical significance of PLR should be cautiously explained with full consideration of any concurrent diseases.
Conclusions
We identified sex, admission PLR, and basal cistern status from initial CT findings as independent prognostic predictors in pediatric patients with MS-TBI, which may contribute to individualized prognosis prediction and better clinical decision making. However, a larger data set from multiple centers is still needed to confirm the findings of this research and promote its clinical implementation.
Data Availability Statement
The raw data supporting the conclusions of this article will be made available by the authors, without undue reservation.
Ethics Statement
The studies involving human participants were reviewed and approved by the Research Ethics Committee of The Second Affiliated Hospital of Wenzhou Medical University. Written informed consent to participate in this study was provided by the participants' legal guardian/next of kin.
Author Contributions
PZh, NH, ZY, and XY contributed to the conception of the study. LLi, JT, YX, YW, PZo, and KS performed the data collection. PZh, NH, and GB performed the data analyses and wrote the manuscript. JT, LLin, LLi, ZY, and XY helped perform the analysis with constructive discussions. All authors contributed to the article and approved the submitted version.
Funding
This work was supported by grants from the Natural Science Foundation of Zhejiang Province (No. LY19H180003) and the National Natural Science Foundation of China (No. 82071902).
Conflict of Interest
The authors declare thatthe research was conducted in the absence of any commercial or financial relationships that could be construed as a potential conflict of interest.
Publisher's Note
All claims expressed in this article are solely those of the authors and do not necessarily represent those of their affiliated organizations, or those of the publisher, the editors and the reviewers. Any product that may be evaluated in this article, or claim that may be made by its manufacturer, is not guaranteed or endorsed by the publisher.
Supplementary Material
The Supplementary Material for this article can be found online at: https://www.frontiersin.org/articles/10.3389/fneur.2022.865084/full#supplementary-material
Abbreviations
MS-TBI, moderate or severe traumatic brain injury; PLR, platelet to lymphocyte ratio; C-index, concordance index; CI, confidence interval; CT, computerized tomography; SBI, secondary brain damage progression; CNS, central nervous system; NLR, neutrophil to lymphocyte ratio; LMR, lymphocyte to monocyte ratio; SII, systemic immune-inflammation index; GCS, Glasgow Coma Scale; Hb, hemoglobin; HCT, Hematocrit; MCV, mean corpuscular volume; RDW-CV, red cell distribution width–coefficient of variation; IVH, intraventricular hemorrhage; SAH, subarachnoid hemorrhage; SDH, subdural hematoma; EDH, epidural hematoma; ROC, receiver operating characteristic; IQR, interquartile range; OR, odds ratio; AUC, area under the curve; HPA, hypothalamic–pituitary–adrenal axis.
References
1. Rubiano AM, Carney N, Chesnut R, Puyana JC. Global neurotrauma research challenges and opportunities. Nature. (2015) 527:S193–7. doi: 10.1038/nature16035
2. Araki T, Yokota H, Morita A. Pediatric traumatic brain injury: characteristic features, diagnosis, and management. Neurol Med Chir. (2017) 57:82–93. doi: 10.2176/nmc.ra.2016-0191
3. Maas AI, Hukkelhoven CW, Marshall LF, Steyerberg EW. Prediction of outcome in traumatic brain injury with computed tomographic characteristics: a comparison between the computed tomographic classification and combinations of computed tomographic predictors. Neurosurgery. (2005) 57:1173–82. doi: 10.1227/01.NEU.0000186013.63046.6B
4. Marshall LF, Marshall SB, Klauber MR, van Berkum Clark M, Eisenberg HM, Jane JA, et al. A new classification of head injury based on computerized tomography. J Neurosurg. (1991) 75:S14–20. doi: 10.3171/sup.1991.75.1s.0s14
5. Raj R, Siironen J, Skrifvars MB, Hernesniemi J, Kivisaari R. Predicting outcome in traumatic brain injury: development of a novel computerized tomography classification system (Helsinki computerized tomography score). Neurosurgery. (2014) 75:632–47. doi: 10.1227/NEU.0000000000000533
6. Zhang J, Zhang F, Dong J-F. Coagulopathy induced by traumatic brain injury: systemic manifestation of a localized injury. Blood J Am Soc Hematol. (2018) 131:2001–6. doi: 10.1182/blood-2017-11-784108
7. Ge X, Zhu L, Li W, Sun J, Chen F, Li Y, et al. Red cell distribution width to platelet count ratio: a promising routinely available indicator of mortality for acute traumatic brain injury. J Neurotrauma. (2021) 38:2487. doi: 10.21203/rs.3.rs-80607/v1
8. Mano Y, Shirabe K, Yamashita Y, Harimoto N, Tsujita E, Takeishi K, et al. Preoperative neutrophil-to-lymphocyte ratio is a predictor of survival after hepatectomy for hepatocellular carcinoma a retrospective analysis. Ann Surg. (2013) 258:301–5. doi: 10.1097/SLA.0b013e318297ad6b
9. Peng W, Li C, Zhu WJ, Wen TF, Yan LN Li B, et al. Prognostic value of the platelet to lymphocyte ratio change in liver cancer. J Surg Res. (2015) 194:464–70. doi: 10.1016/j.jss.2014.12.021
10. Lin ZX, Ruan DY Li Y, Wu DH, Ma XK, Chen J, et al. Lymphocyte-to-monocyte ratio predicts survival of patients with hepatocellular carcinoma after curative resection. World J Gastroenterol. (2015) 21:10898–906. doi: 10.3748/wjg.v21.i38.10898
11. Hu B, Yang XR, Xu Y, Sun YF, Sun C, Guo W, et al. Systemic immune-inflammation index predicts prognosis of patients after curative resection for hepatocellular carcinoma. Clin Cancer Res. (2014) 20:6212–22. doi: 10.1158/1078-0432.CCR-14-0442
12. Huang G, Chen H, Wang Q, Hong X, Hu P, Xiao M, et al. High platelet-to-lymphocyte ratio are associated with post-stroke depression. J Affect Disord. (2019) 246:105–11. doi: 10.1016/j.jad.2018.12.012
13. Tao C, Wang J, Hu X, Ma J, Li H, You C. Clinical value of neutrophil to lymphocyte and platelet to lymphocyte ratio after aneurysmal subarachnoid hemorrhage. Neurocrit Care. (2017) 26:393–401. doi: 10.1007/s12028-016-0332-0
14. Dong X, Nao J, Shi J, Zheng D. Predictive value of routine peripheral blood biomarkers in Alzheimer's disease. Front Aging Neurosci. (2019) 11:332. doi: 10.3389/fnagi.2019.00332
15. Mata-Mbemba D, Mugikura S, Nakagawa A, Murata T, Ishii K, Li L, et al. Early CT findings to predict early death in patients with traumatic brain injury: Marshall and Rotterdam CT scoring systems compared in the major academic tertiary care hospital in northeastern Japan. Acad Radiol. (2014) 21:605–11. doi: 10.1016/j.acra.2014.01.017
16. Pappacena S, Bailey M, Cabrini L, Landoni G, Udy A, Pilcher DV, et al. Early dysglycemia and mortality in traumatic brain injury and subarachnoid hemorrhage. Minerva Anestesiol. (2019) 85:830–9. doi: 10.23736/S0375-9393.19.13307-X
17. Williams O, Tallantyre EC, Robertson N. Traumatic brain injury: pathophysiology, clinical outcome and treatment. J Neurol. (2015) 262:1394–6. doi: 10.1007/s00415-015-7741-4
18. Werner JK, Stevens RD. Traumatic brain injury: recent advances in plasticity and regeneration. Curr Opin Neurol. (2015) 28:565–73. doi: 10.1097/WCO.0000000000000265
19. Liu Y-W, Li S, Dai S-S. Neutrophils in traumatic brain injury (TBI): friend or foe? J Neuroinflammation. (2018) 15:1–18. doi: 10.1186/s12974-018-1173-x
20. Murray GD, Brennan PM, Teasdale GM. Simplifying the use of prognostic information in traumatic brain injury. Part 2: graphical presentation of probabilities. J Neurosurg. (2018) 128:1621–34. doi: 10.3171/2017.12.JNS172782
21. El Dib R, Suzumura EA, Akl EA, Gomaa H, Agarwal A, Chang Y, et al. Electronic nicotine delivery systems and/or electronic non-nicotine delivery systems for tobacco smoking cessation or reduction: a systematic review and meta-analysis. BMJ Open. (2017) 7:e012680. doi: 10.1136/bmjopen-2016-012680
22. Cho SY, Jeon YL, Kim W, Kim W-S, Lee HJ, Lee W-I, et al. Mean platelet volume and mean platelet volume/platelet count ratio in infective endocarditis. Platelets. (2014) 25:559–61. doi: 10.3109/09537104.2013.857394
23. Zhang W, Shen Y. Platelet-to-lymphocyte ratio as a new predictive index of neurological outcomes in patients with acute intracranial hemorrhage: a retrospective study. Med Sci Monit Int Med J Exp Clin Res. (2018) 24:4413. doi: 10.12659/MSM.910845
24. Balta S, Demirkol S, Kucuk U. The platelet lymphocyte ratio may be useful inflammatory indicator in clinical practice. Hemodial Int. (2013) 17:668–9. doi: 10.1111/hdi.12058
25. Xu JH, He XW Li Q, Liu JR, Zhuang MT, Huang FF, et al. Higher platelet-to-lymphocyte ratio is associated with worse outcomes after intravenous thrombolysis in acute ischaemic stroke. Front Neurol. (2019) 10:1192. doi: 10.3389/fneur.2019.01192
26. Wen H, Chen Y. The predictive value of platelet to lymphocyte ratio and D-dimer to fibrinogen ratio combined with WELLS score on lower extremity deep vein thrombosis in young patients with cerebral hemorrhage. Neurol Sci. (2021) 42:3715–21. doi: 10.1007/s10072-020-05007-y
27. Thomson SP, McMahon LJ, Nugent CA. Endogenous cortisol: a regulator of the number of lymphocytes in peripheral blood. Clin lmmunol lmmunopathol. (1980) 17:506–14. doi: 10.1016/0090-1229(80)90146-4
28. Zouridakis EG, Garcia-Moll X, Kaski JC. Usefulness of the blood lymphocyte count in predicting recurrent instability and death in patients with unstable angina pectoris. Am J Cardiol. (2000) 86:449–51. doi: 10.1016/S0002-9149(00)00963-2
29. Basem A, Neeraj S, Meredith A. Mcginn Joseph T. Value of platelet/lymphocyte ratio as a predictor of all-cause mortality after non-ST-elevation myocardial infarction. J Thromb Thrombolysis. (2012) 34:326–34. doi: 10.1007/s11239-012-0718-6
30. Colquhoun I, Burrows E. The prognostic significance of the third ventricle and basal cisterns in severe closed head injury. Clin Radiol. (1989) 40:13–6. doi: 10.1016/S0009-9260(89)80006-6
31. Teasdale E, Cardoso E, Galbraith S, Teasdale GCT. scan in severe diffuse head injury: physiological and clinical correlations. J Neurol Neurosurg Psychiatry. (1984) 47:600–3. doi: 10.1136/jnnp.47.6.600
32. Gupte RP, Brooks WM, Vukas RR, Pierce JD, Harris JL. Sex differences in traumatic brain injury: what we know and what we should know. J Neurotrauma. (2019) 36:3063–91. doi: 10.1089/neu.2018.6171
33. Slewa-Younan S, Baguley IJ, Heriseanu R, Cameron ID, Pitsiavas V, Mudaliar Y, et al. Do men and women differ in their course following traumatic brain injury? A preliminary prospective investigation of early outcome. Brain Injury. (2008) 22:183–91. doi: 10.1080/02699050801888808
34. Cancelliere C, Donovan J, Cassidy JD. Is sex an indicator of prognosis after mild traumatic brain injury: a systematic analysis of the findings of the World Health Organization Collaborating Centre Task Force on Mild Traumatic Brain Injury and the International Collaboration on Mild Traumatic Brain Injury Prognosis. Arch Phys Med Rehabil. (2016) 97:S5–S18. doi: 10.1016/j.apmr.2014.11.028
35. Bazarian JJ, Blyth B, Mookerjee S, He H, McDermott MP. Sex differences in outcome after mild traumatic brain injury. J Neurotrauma. (2010) 27:527–39. doi: 10.1089/neu.2009.1068
36. Yue JK, Levin HS, Suen CG, Morrissey MR, Runyon SJ, Winkler EA, et al. Age and sex-mediated differences in six-month outcomes after mild traumatic brain injury in young adults: a TRACK-TBI study. Neurol Res. (2019) 41:609–23. doi: 10.1080/01616412.2019.1602312
Keywords: early mortality, moderate or severe traumatic brain injury, pediatrics, nomogram, radiology, inflammation
Citation: Zhu P, Hussein NM, Tang J, Lin L, Wang Y, Li L, Shu K, Zou P, Xia Y, Bai G, Yan Z and Ye X (2022) Prediction of Early Mortality Among Children With Moderate or Severe Traumatic Brain Injury Based on a Nomogram Integrating Radiological and Inflammation-Based Biomarkers. Front. Neurol. 13:865084. doi: 10.3389/fneur.2022.865084
Received: 29 January 2022; Accepted: 02 May 2022;
Published: 20 May 2022.
Edited by:
Takeo Mukai, The University of Tokyo, JapanCopyright © 2022 Zhu, Hussein, Tang, Lin, Wang, Li, Shu, Zou, Xia, Bai, Yan and Ye. This is an open-access article distributed under the terms of the Creative Commons Attribution License (CC BY). The use, distribution or reproduction in other forums is permitted, provided the original author(s) and the copyright owner(s) are credited and that the original publication in this journal is cited, in accordance with accepted academic practice. No use, distribution or reproduction is permitted which does not comply with these terms.
*Correspondence: Xinjian Ye, wzfskyexinjian@163.com; Zhihan Yan, yanzhihanwz@163.com
†These authors have contributed equally to this work