- 1Department of Hematology and Oncology, International Cancer Center, Shenzhen Key Laboratory, Hematology Institution of Shenzhen University, Shenzhen University General Hospital, Shenzhen University Health Science Center, Shenzhen University, Shenzhen, China
- 2Department of Depressive Disorder, Shenzhen Kangning Hospital, Shenzhen Mental Health Center, Shenzhen, China
- 3Department of Radiology, Huazhong University of Science and Technology Union Shenzhen Hospital, Shenzhen, China
- 4Department of Radiology, Shenzhen Kangning Hospital, Shenzhen Mental Health Center, Shenzhen, China
Objective: Inter-hemispheric network dysconnectivity has been well-documented in patients with recurrent major depressive disorder (MDD). However, it has remained unclear how structural networks between bilateral hemispheres relate to inter-hemispheric functional dysconnectivity and depression severity in MDD. Our study attempted to investigate the alterations in corpus callosum macrostructural and microstructural as well as inter-hemispheric homotopic functional connectivity (FC) in patients with recurrent MDD and to determine how these alterations are related with depressive severity.
Materials and Methods: Resting-state functional MRI (fMRI), T1WI anatomical images and diffusion tensor MRI of the whole brain were performed in 140 MDD patients and 44 normal controls matched for age, sex, years of education. We analyzed the macrostructural and microstructural integrity as well as voxel-mirrored homotopic functional connectivity (VMHC) of corpus callosum (CC) and its five subregion. Two-sample t-test was used to investigate the differences between the two groups. Significant subregional metrics were correlated with depression severity by spearman's correlation analysis, respectively.
Results: Compared with control subjects, MDD patients had significantly attenuated inter-hemispheric homotopic FC in the bilateral medial prefrontal cortex, and impaired anterior CC microstructural integrity (each comparison had a corrected P < 0.05), whereas CC macrostructural measurements remained stable. In addition, disruption of anterior CC microstructural integrity correlated with a reduction in FC in the bilateral medial prefrontal cortex, which correlated with depression severity in MDD patients. Furthermore, disruption of anterior CC integrity exerted an indirect influence on depression severity in MDD patients through an impairment of inter-hemispheric homotopic FC.
Conclusion: These findings may help to advance our understanding of the neurobiological basis of depression by identifying region-specific interhemispheric dysconnectivity.
Introduction
Depression is a common mental disorder and a leading cause of disability, affecting more than 264 million people worldwide (1). Although it is highly prevalent, the precise neurobiological mechanisms underlying major depressive disorder (MDD) are still not fully elucidated (2). Convergent evidence from neuroimaging studies has suggested that patients with depression exhibit structural and functional abnormalities in cerebral regions or networks that participate in affective regulation (2–5).
Bihemispheric communication plays a vital role in integrating neural circuits and underlies coherent cognition, emotional regulation, and behavior (6, 7). Interhemispheric functional homotopy, reflecting bihemispheric communication, has been verified as one of the most salient and stable features of the brain's intrinsic functional infrastructure (8). Impairment of inter-hemispheric functional homotopy has been wildly documented in MDD patients via functional magnetic resonance imaging (fMRI) (7, 9–13). However, the results of such fMRI studies have been somewhat inconsistent. Interhemispheric functional dysconnectivity has been observed in the bilateral posterior default mode network (DMN) in first-episode medication-naïve MDD patients (9, 12), in the bilateral sub-genu anterior cingulate cortex in resilient MDD patients (14), and in the bilateral cuneus, putamen, superior temporal gyrus, insula, and precuneus in patients with current and recurrent MDD (11). Even in first-episode patients without treatment, some studies have implicated that interhemispheric dysconnectivity is mainly involved in the anterior subnetwork of the DMN (10, 15), while other studies have reported the posterior DMN (9, 12), insular cortex, and putamen (13) as being more vulnerable to MDD. These inconsistent and conflicting findings must be interpreted with caution, as most previous studies have only recruited relatively small sample sizes of MDD patients (9, 10, 13). Furthermore, the underlying structural mechanisms of altered inter-hemispheric functional connectivity (FC) in patients with MDD remain largely unknown (11).
The corpus callosum (CC) is the largest interhemispheric commissure and plays a cardinal role as the primary cortical projection system connecting the two brain hemispheres (16). CC degeneration, in terms of either volumetric reduction or diffusivity disruption, has been documented in MDD (12, 17, 18). However, functionally, the CC consists of different components that bridge different brain regions (16), The genu of the CC connects the orbitofrontal and frontal cortices, whereas the body and the splenium connects the temporal, parietal, and occipital regions (19). Therefore, it is important for further studies to determine which CC subfields are the most susceptible to depression, as well as the relationships among regional-specific CC impairment, inter-hemispheric homotopic functional dysconnectivity, and depression severity in MDD patients.
To address these questions, we combined high-resolution structural MRI, diffusion tensor MRI, resting-state functional MRI, and the Hamilton Depression Rating Scale (HDRS) to investigate disruption of inter-hemispheric homotopic FC, CC degeneration (and its subregions), and depression severity in a relatively large sample of recurrent MDD patients. We hypothesized that structural degeneration in the anterior CC (compared to that of the posterior CC) may be associated with bilateral prefrontal cortex dysconnectivity, which may underly depression severity in MDD.
Methods and Materials
Patients
We recruited 140 patients with recurrent MDD, all the patients were currently experiencing an episode of major depression, but had not receive standardized drug treatment under guidance of doctor. Patients were diagnosed according to the Structured Clinical Interview for the Diagnostic and Statistical Manual of Mental Disorders (DSM-IV) (SCID). Depression severity was measured using the 17-item HDRS. Inclusion criteria for individuals with MDD included the following: aged 16–70 years, right-hand dominant, and met the DSM-IV diagnostic criteria for MDD with a current depressive episode. Healthy controls were included if they met the following criteria: aged 16–70 years, right-hand dominant, and no personal or family history of major psychiatric illness.
Exclusion criteria were as follows: a history of another DSM-IV Axis-I disorder (e.g., schizophrenia, schizoaffective disorder); any other physical disease affecting brain function (e.g., brain trauma, epilepsy, stroke); a history of substance dependence or abuse; or other contraindications for undergoing an MRI (e.g., serious medical illness, breastfeeding, pregnancy).
Written informed consent was acquired from each participant before study enrollment. The study protocols were approved by the Institutional Review Board of the Medical Ethics Committee of Shenzhen Kangning Hospital.
Clinical and Cognitive Outcome Measures
Symptom severity for each patient was assessed with the 17-item HDRS (20, 21). Neuropsychological assessments were conducted by research personnel, who were trained and supervised by a registered clinical neuropsychologist (Yingli Zhang).
MRI Acquisition
All MRI data were acquired on a 3.0-Tesla scanner (Discovery MR750 System; General Electric, Milwaukee, WI, USA) with an 8-channel head coil. Each participant was asked to lay in a supine position with his or her head snugly fixed by a belt and foam pads, and earplugs were used to reduce noise. Before MRI scanning was initiated, participants were required to keep their eyes closed, to not fall asleep, and remain motionless during the scanning period. First, a T1-weighted image (repetition time [TR] = 1,750 ms, echo time [TE] = 24 ms) and T2-FLAIR images (TR = 8,400 ms, TE = 120 ms, and inversion time [TI] = 2,100 ms) were captured to exclude subjects with abnormalities. Then, a resting-state fMRI scan with an echo-planar imaging (EPI) sequence, a high-resolution structural MRI scan with a fast-field echo (FFE) three-dimensional T1-weighted (3D-T1WI) sequence, and a diffusion tensor MRI scan with a single-shot spin-echo-planar sequence were sequentially conducted. Detailed imaging parameters were as follows: (1) resting-state fMRI: TR/TE = 2,000/30 ms, flip angle = 90°, thickness/gap = 3.5/0 mm, acquisition matrix = 64 × 64, field of view (FOV) = 224 × 224 mm2, 33 axial slices, and 240 time points (8 min); (2) 3D-T1WI: TR/TE = 6.65/2.93 ms, flip angle = 12°, acquisition matrix = 256 × 256, FOV = 256 × 256 mm2, and 192 sagittal slices with no inter-slice gap; (3) diffusion tensor MRI: 64 diffusion-weighted images (b = 1,000 s/mm2) and 10 non-diffusion-weighted images (b = 0 s/mm2) using a spin-echo echo-planar imaging sequence with the following parameters: number of excitations = 1, repetition time (TR) = 8,724 ms, echo time (TE) = 81.4 ms, acquisition matrix = 112 × 112, and voxel size = 2 mm × 2 mm × 2 mm.
Preprocessing of Structural Images and CC Segmentation
We preprocessed structural MRI data according to the FreeSurfer pipeline (version 6.0, http://surfer.nmr.mgh.harvard.edu), according to previous studies (22, 23). Manual editing was performed where appropriate, and scans with unresolvable reconstruction errors were excluded from further analysis. The CC was automatically identified and segmented into five segments according to our previously published protocol (23–25), with each section representing a fifth of the total area/volume that broadly corresponds to the following functional subdivisions (26): anterior (CC1), mid-anterior (CC2), central (CC3), mid-posterior (CC4), and posterior portions (CC5; Supplementary Figure 1). The total CC volume was calculated as the sum of the five segments.
Preprocessing of Diffusion Tensor MRI Data and Derivation of CC Subregional Microstructure
FreeSurfer's dt_recon program was used to preprocess the diffusion tensor MRI data. First, eddy-current compensation and motion-correction procedures were conducted on diffusion volumes using FLIRT (27). Non-brain tissues were then removed from the diffusion-weighted imaging using the Brain Extraction Tool (BET) from FSL (28). Next, general linear model (GLM) fitting and tensor construction were conducted to generate fractional anisotropy (FA) maps (29). Thereafter, low b-diffusion images were registered to the same-subject anatomical coordinates using a boundary-based registration procedure (30). Finally, the above-generated FA maps were mapped to talairach space (31). Then the average FA values of the CC subfields were extracted through mri_segstats. The mean head-motion parameters for translation and rotation were also computed for each subject and were included as a covariate in statistical analyses. Details can be found at http://surfer.nmr.mgh.harvard.edu/fswiki/dt_recon#dtrecon.
Preprocessing of Resting-State FMRI Data and Derivation of Interhemispheric FC
We preprocessed the resting-state fMRI data using Data Processing & Analysis of Brain Imaging (DPABI 1.2) (32), which is based on statistical parametric mapping (SPM8, http://www.fil.ion.ucl.ac.uk/spm), following our previous approaches (24, 25, 33). Details regarding our preprocessing of resting-state fMRI data can be found in the Supplementary Materials. After quality control, all participants met the criterion of minimum absolute translation and rotation not exceeding 1.5 mm or 1.5°. Moreover, the mean framewise displacement (FD) was computed by averaging the FD from every time point for each subject, and it was included as a covariate in statistical analyses (34).
We computed subject-level interhemispheric FC by using voxel-mirrored homotopic connectivity (VMHC) method, which calculated the functional connectivity between each voxel in one hemisphere and its mirrored counterpart in the other following previous research (35). Shortly speaking, for every subject, Pearson's correlation was used to obtain the homotopic FC between each voxel's residual time series and that of its mirrored inter-hemispheric counterpart. Thereafter, correlation values were Fisher's z-transformed to produce subject-specific VMHC z-score maps for further statistical analyses.
Statistical Analysis
Categorical variables were inspected using chi-squared tests, and continuous variables were compared using two-sample t-tests or non-parametric tests.
CC subfield volumes and FA were compared via univariate analysis in SPSS version 26.0 (IBM Inc., Armonk, NY). Analyses were two-tailed and evaluated for significance at the 0.05 alpha level (controlling for age, gender, education, TIV [for volume], and head motion [for FA]). Multiple-comparison corrections were performed using Bonferroni corrections.
Interhemispheric FC differences between groups were assessed by independent-sample t-tests in SPM v12 (Wellcome Trust Centre for Neuroimaging, UCL, London, UK), and nuisance covariates included age, gender, education, and head motion. The results were reported at a height threshold of P < 0.01 and a cluster threshold of P < 0.05, with a Gaussian random field (GRF) correction.
Results
Demographic Information
There were no group differences in terms of age, sex distribution, education, or head motion (Table 1). For MDD patients, the median disease duration for MDD was 3.35 years, and the median HDRS score was 22.
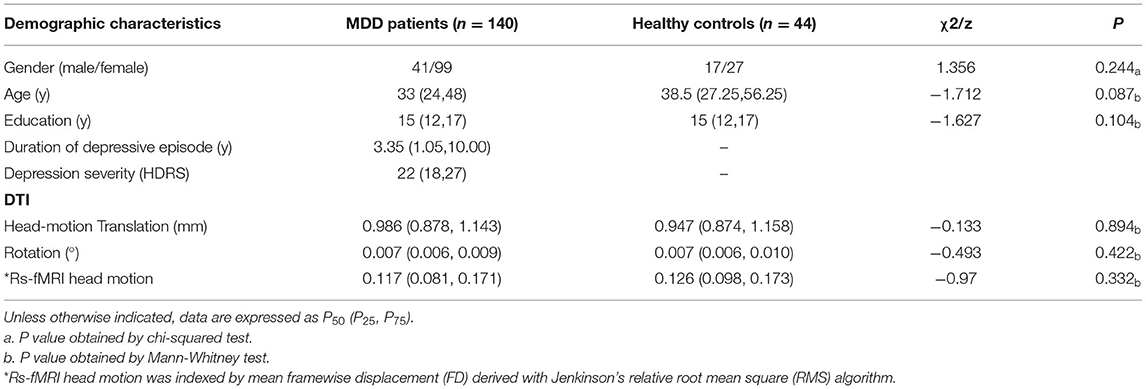
Table 1. Demographic and clinical characteristics of patients with recurrent major depressive disorder (MDD) and healthy controls.
Disruption of Anterior CC Microstructural but Not Macrostructural Integrity in MDD Patients
MDD patients had lower FA of the anterior CC, but a relatively stable volume, when compared with these parameters in healthy controls, suggesting that MDD patients exhibit microstructural integrity disruption in the anterior CC (Figure 1).
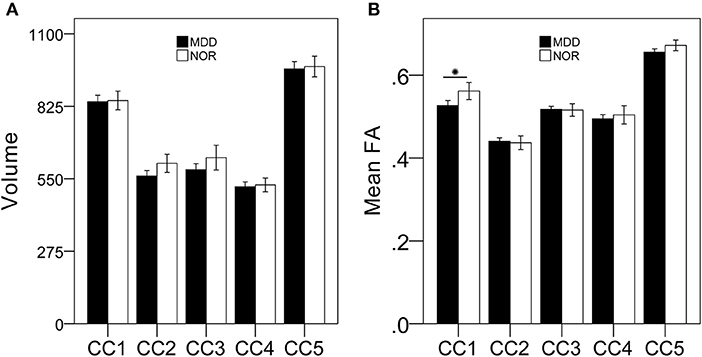
Figure 1. MDD patients exhibit damaged anterior CC microstructural but not macrostructural integrity compared with that of healthy controls. There were no significant differences in CC subregional volumes between MDD patients and healthy controls (A); MDD patients had lower FA of the anterior CC subregion compared to that of healthy controls (B). *p < 0.05, bonferroni corrected.
Disruption of Inter-hemispheric Homotopic FC of the Bilateral Medial Prefrontal Cortex in MDD Patients
Compared to that in healthy controls, MDD patients showed a significant decrease in inter-hemispheric FC in the bilateral medial prefrontal cortex (Figure 2, Table 2).
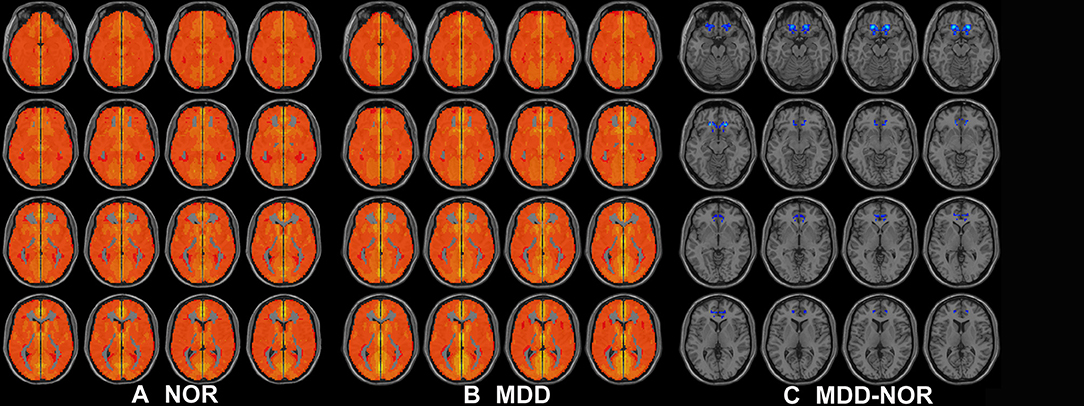
Figure 2. Axial MRI scans show interhemispheric FC within and between groups. Regions show significant interhemispheric FC in (A) healthy controls and (B) MDD patients (P < 0.05, AlphaSim corrected). (C) Homotopic regions show decreased (cool color) FC in the MDD group (P < 0.05, AlphaSim corrected).
Bilateral Medial Prefrontal Dysconnectivity Correlates With Disruption of Anterior CC Microstructural Integrity and Depression Severity in MDD Patients
Interhemispheric homotopic FC of the bilateral medial prefrontal cortex correlated with anterior CC microstructural integrity (r = 0.201; P = 0.014) (Figure 3) and depression severity (r = −0.304; P < 0.001) in MDD patients (Figure 4).
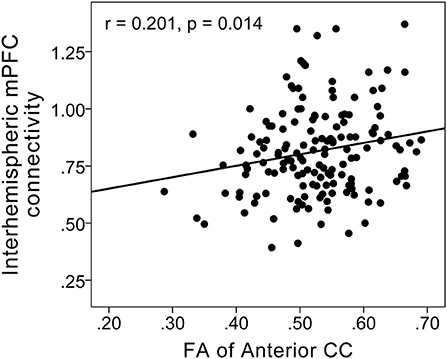
Figure 3. Scatterplots showing a positive correlation between FC in the bilateral medial prefrontal cortex and fractional anisotropy of the anterior CC in MDD patients. Interhemispheric homotopic FC of the bilateral medial prefrontal cortex correlated with anterior CC microstructural integrity (r = 0.201; P = 0.014).
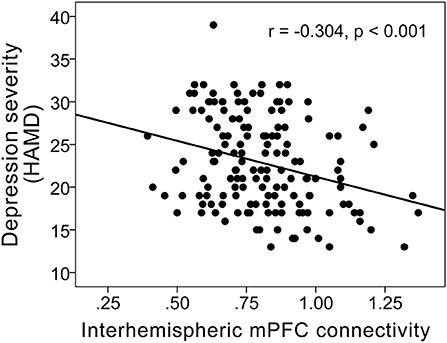
Figure 4. Scatterplots showing a negative correlation between FC of the bilateral medial prefrontal cortex and depression severity (r = −0.304; P < 0.001) in MDD patients.
Disruption of the Anterior CC Has an Indirect Influence on Depression Severity in MDD Patients
Anterior CC disruption had an indirect impact (through exerting effects on FC of the bilateral medial prefrontal cortex) on depression severity in MDD (Figure 5).
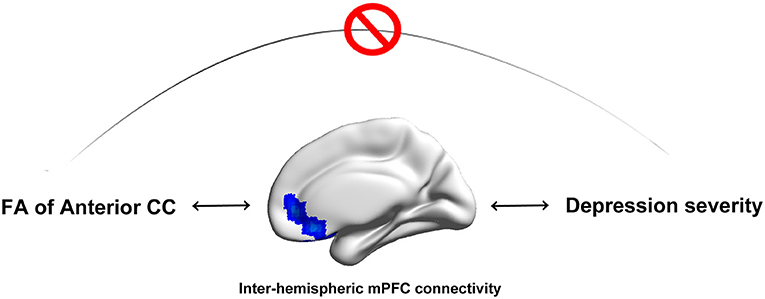
Figure 5. Anterior CC microstructural integrity has an indirect influence on depression severity in MDD patients. Disruption of anterior CC microstructural integrity has an indirect impact (through exerting effects on FC of the bilateral medial prefrontal cortex) on depression severity in MDD patients.
Discussion
To the best of our knowledge, our present study represents the first report of reduced interhemispheric homotopic FC and structural connectivity (evaluated by CC and its subregional macrostructure and microstructure) in MDD patients, as elucidated via multimodal MRI. A primary finding of the present study was significantly decreased VMHC in the bilateral medial prefrontal cortex, which correlated with disruption of anterior CC (genu) microstructural but not macrostructural integrity in MDD patients. Further analysis revealed that severe depressive symptoms (higher HDRS scores) correlated with decreased interhemispheric FC of the bilateral medial prefrontal cortex in MDD patients. Moreover, aberrant anterior CC integrity exerted an indirect effect on depression severity in MDD patients, which was associated with reduced FC in the bilateral medial prefrontal cortex. Taken together, these findings may help to advance our understanding of the neurobiological basis of depression by identifying region-specific inter-hemispheric dysconnectivity in MDD patients.
Selectively Anterior CC Microstructural but Not Macrostructural Damage in MDD Patients
It is noteworthy that MDD patients had selective impairment of anterior CC microstructural integrity, but not of any other CC subfields, relative to matched healthy controls. This finding is not surprising given that the anterior CC bridges bilateral prefrontal and orbitofrontal regions (36), which are central to current theories of mood regulation in humans (37, 38). Disruption of anterior CC microstructural integrity, implying decreased structural connectivity between prefrontal and orbitofrontal regions, may constitute a common pathway underlying mood dysregulation in MDD (38). Selective anterior CC damage is a well-replicated finding in MDD patients from previous studies (17, 18, 38, 39). Using a region-of-interest method, Dillon et al. found that MDD patients had reduced FA in the genu of the CC, in addition to lower FA in the anterior limb of the internal capsule, the cingulum bundle near the ACC, and the uncinate fasciculus. Moreover, they demonstrated an inverse relationship between depressive severity and FA in the genu of the CC (r = −0.44, P < 0.01) (39). Xu et al. also found that FA values were significantly lower in the anterior genu of the CC in the medication-free MDD group than in the control group (P = 0.009, corrected), while no significant differences were found in any other CC subregions (17). In a meta-analysis of medication-free patients with MDD, Jiang et al. found that only medication-naive patients with MDD displayed FA reductions in the genu of the CC and in right anterior thalamic projections (18). Similarly, another meta-analysis also showed that patients with major depression and bipolar disorder were characterized by abnormalities in white matter tracts of the genu of the CC that connect the two hemispheres of the prefrontal cortex implicated in mood regulation (36). Taken together, these findings suggest that disruption of anterior CC microstructural integrity, implying structural dysconnectivity between prefrontal and orbitofrontal regions, may be an underlying neurobiological mechanism of mood dysregulation in MDD.
Unexpectedly, in our present study, volumetric measurements of the CC and its subfields did not differ between MDD patients and matched healthy controls. In contrast to the consistent results from studies of CC microstructural integrity, studies of CC volumetric measurements have yielded inconsistent results in MDD patients (12). Ran et al. revealed that patients with MDD had smaller volumes in the genu of the CC than in controls, as determined by FreeSurfer segmentation (12). In addition, Emsell et al. did not find any differences in CC subregional thickness or surface area (by dividing the mid-sagittal CC into seven subregions); thus, they demonstrated that callosal structure was largely preserved in late-life depression (40). Ozalay et al. also demonstrated that there were no differences in the areas or lengths of the total CC or any CC subfields (by dividing the CC into five parts) between MDD patients and healthy controls. Our present findings are in agreement with most previous studies (41). These findings suggest that CC microstructural alterations are more sensitive to changes in tissues than are volumetric measurements, and may predate macrostructural changes in MDD. Therefore, a reduction in anterior CC microstructural integrity (but not macrostructural integrity) may serve as an in vivo biomarker of MDD.
Interhemispheric Functional Dysconnectivity in the Medial Prefrontal Cortices of MDD Patients
As expected, we found that MDD patients exhibited reductions in inter-hemispheric homotopic FC in the medial prefrontal cortex, which extends to the anterior cingulate cortex and orbitofrontal cortex, the cardinal regions responsible for emotional regulation (37). This finding is consistent with previous pathology-based evidence of prefrontal abnormalities in MDD patients (42, 43) and multimodal imaging models (2, 11, 44, 45). The medial prefrontal cortex is a key part of the cortico-striato-thalamo-cortical loop, with multiple connections to the limbic system (including the amygdala and hippocampus), basal ganglia, and thalamus. Given this extensive network, the medial prefrontal cortex and ACC have been postulated to be functional in the monitoring and regulation of emotional states; thus, dysconnectivity of the bilateral medial prefrontal cortex may be implicated in mood dysregulation in MDD patients.
Furthermore, we found that FC in the bilateral medial prefrontal cortex was positively correlated with FA of the anterior CC, and was inversely correlated with depression severity (HDRS scores), indicating that anterior CC microstructural disruption may represent the structural basis of dysconnectivity in the bilateral prefrontal cortex, and that reduced FC in the bilateral prefrontal cortex may represent depression severity in MDD patients. Furthermore, we found that anterior CC microstructural deficits exerted an indirect effect on depression severity in MDD patients, which was associated with reduced FC in the bilateral medial prefrontal cortex, suggesting that white matter microstructural disruptions the anterior CC itself may not directly exert effects on depression, but that impaired anterior CC white matter integrity may lead to an imbalance in frontal cortical networks, which in turn may confer depression.
Despite the strengths of our present study—including its relatively large sample size and integrated use of multimodal MRI methods—there were also some limitations. First, although current DTI analysis revealed anterior CC subfield's microstructural integrity was impaired in MDD patients, the present results should be interpreted with caution, given the contamination of neuroinflammation as well as crossing fiber should be considered. Thus, free water corrected-DTI analysis as well as advanced diffusion imaging, such as diffusion spectrum imaging (46) and high-angular resolution diffusion imaging (47), should be conducted to validate these preliminary findings in future. Second, as a cross-sectional study, we can only observe the interaction effects among impairment of anterior CC integrity, dysfunctional of inter-hemispheric homotopic FC of the bilateral medial prefrontal cortex and depression severity in MDD patients. However, we cannot absolutely assert the causation among them. Longitudinal are needed in future. Third, the included MDD patients were clinically heterogeneous in terms of illness duration, age of onset, number of episodes, and prior medication history. These factors may have weakened our sensitivity in revealing MDD-related alterations in the CC and its subregional volumes. Forth, although we integrated multiple imaging modes (structural, diffusion, and resting-state BOLD) in the present study, we only focused on inter-hemispheric structural and functional aberrations in MDD patients. In future, other quantitative indices (e.g., whole-brain network-related connectivity analysis; voxel/vertex-based morphological analysis) should be investigated in future studies to further elucidate the pathogenesis of MDD. Fifth, VMHC methods may have inherent limitations due to the bilateral hemispheres not being entirely symmetric. However, spatial smoothing was applied to improve the spatial correspondence between homotopic areas and to reduce the impact of the asymmetric nature of the brain, which can also minimize the potential confounder of inter-subject anatomical variability. Last, the CC was equally sub-divided with the FreeSurfer method, which might not accurately reflect the true fiber compositions in each subregion.
In conclusion, we investigated interhemispheric homotopic FC and structural connectivity in patients with MDD via multimodal MRI. An impairment in interhemispheric FC correlated with clinical depression severity in MDD patients. Furthermore, disruption of anterior CC integrity exerted an indirect effect on depression severity in MDD patients. Our findings provide new insights into the neural mechanisms of MDD and reinforce an integrative view of the functional and structural organization of the interhemispheric brain in MDD patients. These findings may help to advance our understanding of the neurobiological basis of depression by identifying region-specific interhemispheric dysconnectivity in MDD patients.
Data Availability Statement
The original contributions presented in the study are included in the article/Supplementary Material, further inquiries can be directed to the corresponding author/s.
Ethics Statement
The studies involving human participants were reviewed and approved by the Institutional Review Board of the Medical Ethics Committee of Shenzhen Kangning Hospital. Written informed consent to participate in this study was provided by the participants' legal guardian/next of kin. Written informed consent was obtained from the individual(s) for the publication of any potentially identifiable images or data included in this article.
Author Contributions
QY and HG conception and design. GZ, ZY, CS, ZZ, and PB collection and assembly of the data. QY development of the methodology. All authors: data analysis and interpretation, manuscript writing, and final approval of the manuscript.
Funding
This work was supported by the grants from the Natural Scientific Foundation of China (grant numbers: 81560283 and 81201084), the Guangdong Basic and Applied Basic Research Foundation (2020A1515011332), the Shenzhen Sanming Project (No. SZSM201512038), and the Shenzhen Fund for Guangdong Provincial High-level Clinical Key Specialties (No. SZGSP013), Shenzhen Key Medical Discipline Construction Fund (No. SZXK041), Shenzhen Science and Technology Foundation (JCYJ20190808163601776, JCYJ20200109113810154), Shenzhen Key Laboratory Foundation (ZDSYS20200811143757022), and Sanming Project of Medicine in Shenzhen (SZSM202111004). The funding source of the study had no role in the study design, data collection, data analysis, data interpretation, writing of this report, or decision to submit for publication. The corresponding author had full access to all data in the study and had final responsibility for the decision to submit for publication.
Conflict of Interest
The authors declare that the research was conducted in the absence of any commercial or financial relationships that could be construed as a potential conflict of interest.
Publisher's Note
All claims expressed in this article are solely those of the authors and do not necessarily represent those of their affiliated organizations, or those of the publisher, the editors and the reviewers. Any product that may be evaluated in this article, or claim that may be made by its manufacturer, is not guaranteed or endorsed by the publisher.
Acknowledgments
We thank LetPub (www.letpub.com) for its linguistic assistance during the preparation of this manuscript.
Supplementary Material
The Supplementary Material for this article can be found online at: https://www.frontiersin.org/articles/10.3389/fneur.2022.852330/full#supplementary-material
References
1. Liao W, Li J, Duan X, Cui Q, Chen H, Chen H. Static and dynamic connectomics differentiate between depressed patients with and without suicidal ideation. Hum Brain Mapp. (2018) 39:4105–18. doi: 10.1002/hbm.24235
2. Yan CG, Chen X, Li L, Castellanos FX, Bai TJ, Bo QJ, et al. Reduced default mode network functional connectivity in patients with recurrent major depressive disorder. Proc Natl Acad Sci USA. (2019) 116:9078–83. doi: 10.1073/pnas.1900390116
3. Sheline YI, Barch DM, Price JL, Rundle MM, Vaishnavi SN, Snyder AZ, et al. The default mode network and self-referential processes in depression. Proc Natl Acad Sci USA. (2009) 106:1942–7. doi: 10.1073/pnas.0812686106
4. Ho TC, Gutman B, Pozzi E, Grabe HJ, Hosten N, Wittfeld K, et al. Subcortical shape alterations in major depressive disorder: findings from the ENIGMA major depressive disorder working group. Hum Brain Mapp. (2020) 43:341–51. doi: 10.1101/534370
5. Schmaal L, Veltman DJ, van Erp TG, Samann PG, Frodl T, Jahanshad N, et al. Subcortical brain alterations in major depressive disorder: findings from the ENIGMA Major Depressive Disorder working group. Mol Psychiatry. (2016) 21:806–12. doi: 10.1038/mp.2015.69
6. Gee DG, Biswal BB, Kelly C, Stark DE, Margulies DS, Shehzad Z, et al. Low frequency fluctuations reveal integrated and segregated processing among the cerebral hemispheres. Neuroimage. (2011) 54:517–27. doi: 10.1016/j.neuroimage.2010.05.073
7. Jin X, Liang X, Gong G. Functional integration between the two brain hemispheres: evidence from the homotopic functional connectivity under resting state. Front Neurosci. (2020) 14:932. doi: 10.3389/fnins.2020.00932
8. Stark DE, Margulies DS, Shehzad ZE, Reiss P, Kelly AM, Uddin LQ, et al. Regional variation in interhemispheric coordination of intrinsic hemodynamic fluctuations. J Neurosci. (2008) 28:13754–64. doi: 10.1523/JNEUROSCI.4544-08.2008
9. Guo W, Cui X, Liu F, Chen J, Xie G, Wu R, et al. Decreased interhemispheric coordination in the posterior default-mode network and visual regions as trait alterations in first-episode, drug-naive major depressive disorder. Brain Imaging Behav. (2018) 12:1251–58. doi: 10.1007/s11682-017-9794-8
10. Guo W, Liu F, Dai Y, Jiang M, Zhang J, Yu L, et al. Decreased interhemispheric resting-state functional connectivity in first-episode, drug-naive major depressive disorder. Prog Neuropsychopharmacol Biol Psychiatry. (2013) 41:24–9. doi: 10.1016/j.pnpbp.2012.11.003
11. Hermesdorf M, Sundermann B, Feder S, Schwindt W, Minnerup J, Arolt V, et al. Major depressive disorder: Findings of reduced homotopic connectivity and investigation of underlying structural mechanisms. Hum Brain Mapp. (2016) 37:1209–17. doi: 10.1002/hbm.23097
12. Ran S, Zuo Z, Li C, Yin X, Qu W, Tang Q, et al. Atrophic corpus callosum associated with altered functional asymmetry in major depressive disorder. Neuropsychiatr Dis Treat. (2020) 16:1473–82. doi: 10.2147/NDT.S245078
13. Yang H, Wang C, Ji G, Feng Z, Duan J, Chen F, et al. Aberrant interhemispheric functional connectivity in first-episode, drug-naive major depressive disorder. Brain Imaging Behav. (2019) 13:1302–10. doi: 10.1007/s11682-018-9917-x
14. Workman CI, Lythe KE, McKie S, Moll J, Gethin JA, Deakin JF, et al. A novel resting-state functional magnetic resonance imaging signature of resilience to recurrent depression. Psychol Med. (2017) 47:597–607. doi: 10.1017/S0033291716002567
15. Lai CH, Wu YT. Decreased inter-hemispheric connectivity in anterior sub-network of default mode network and cerebellum: significant findings in major depressive disorder. Int J Neuropsychopharmacol. (2014) 17:1935–42. doi: 10.1017/S1461145714000947
16. de Lacoste MC, Kirkpatrick JB, Ross ED. Topography of the human corpus callosum. J Neuropathol Exp Neurol. (1985) 44:578–91. doi: 10.1097/00005072-198511000-00004
17. Xu K, Jiang W, Ren L, Ouyang X, Jiang Y, Wu F, et al. Impaired interhemispheric connectivity in medication-naive patients with major depressive disorder. J Psychiatry Neurosci. (2013) 38:43–8. doi: 10.1503/jpn.110132
18. Jiang J, Zhao YJ, Hu XY, Du MY, Chen ZQ, Wu M, et al. Microstructural brain abnormalities in medication-free patients with major depressive disorder: a systematic review and meta-analysis of diffusion tensor imaging. J Psychiatry Neurosci. (2017) 42:150–63. doi: 10.1503/jpn.150341
19. Chao YP, Cho KH, Yeh CH, Chou KH, Chen JH, Lin CP. Probabilistic topography of human corpus callosum using cytoarchitectural parcellation and high angular resolution diffusion imaging tractography. Hum Brain Mapp. (2009) 30:3172–87. doi: 10.1002/hbm.20739
20. Williams JB. A structured interview guide for the Hamilton Depression Rating Scale. Arch Gen Psychiatry. (1988) 45:742–7. doi: 10.1001/archpsyc.1988.01800320058007
21. Moberg PJ, Lazarus LW, Mesholam RI, Bilker W, Chuy IL, Neyman I, et al. Comparison of the standard and structured interview guide for the Hamilton Depression Rating Scale in depressed geriatric inpatients. Am J Geriatr Psychiatry. (2001) 9:35–40. doi: 10.1097/00019442-200102000-00006
23. Qiu YW, Jiang GH, Ma XF, Su HH, Lv XF, Zhuo FZ. Aberrant interhemispheric functional and structural connectivity in heroin-dependent individuals. Addict Biol. (2017) 22:1057–67. doi: 10.1111/adb.12387
24. Qiu YW, Lv XF, Jiang GH, Su HH, Ma XF, Tian JZ, et al. Larger corpus callosum and reduced orbitofrontal cortex homotopic connectivity in codeine cough syrup-dependent male adolescents and young adults. Eur Radiol. (2017) 27:1161–8. doi: 10.1007/s00330-016-4465-5
25. Ye M, Guo Z, Li Z, Lin X, Li J, Jiang G, et al. Aberrant inter-hemispheric coordination characterizes the progression of minimal hepatic encephalopathy in patients with HBV-related cirrhosis. Neuroimage Clin. (2020) 25:102175. doi: 10.1016/j.nicl.2020.102175
26. Collinson SL, Gan SC, Woon PS, Kuswanto C, Sum MY, Yang GL, et al. Corpus callosum morphology in first-episode and chronic schizophrenia: combined magnetic resonance and diffusion tensor imaging study of Chinese Singaporean patients. Br J Psychiatry. (2014) 204:55–60. doi: 10.1192/bjp.bp.113.127886
27. Jenkinson M, Bannister P, Brady M, Smith S. Improved optimization for the robust and accurate linear registration and motion correction of brain images. Neuroimage. (2002) 17:825–41. doi: 10.1006/nimg.2002.1132
28. Smith SM. Fast robust automated brain extraction. Hum Brain Mapp. (2002) 17:143–55. doi: 10.1002/hbm.10062
29. Basser PJ, Pierpaoli C. Microstructural and physiological features of tissues elucidated by quantitative-diffusion-tensor MRI. J Magn Reson B. (1996) 111:209–19. doi: 10.1006/jmrb.1996.0086
30. Salat DH, Greve DN, Pacheco JL, Quinn BT, Helmer KG, Buckner RL, et al. Regional white matter volume differences in nondemented aging and Alzheimer's disease. Neuroimage. (2009) 44:1247–58. doi: 10.1016/j.neuroimage.2008.10.030
31. Bai YM, Chou KH, Lin CP, Chen IY, Li CT, Yang KC, et al. White matter abnormalities in schizophrenia patients with tardive dyskinesia: a diffusion tensor image study. Schizophr Res. (2009) 109:167–81. doi: 10.1016/j.schres.2009.02.003
32. Chao-Gan Y, Yu-Feng Z. DPARSF: a MATLAB toolbox for “Pipeline” data analysis of resting-state fMRI. Front Syst Neurosci. (2010) 4:13. doi: 10.3389/fnsys.2010.00013
33. Qiu Y, Liu S, Hilal S, Loke YM, Ikram MK, Xu X, et al. Inter-hemispheric functional dysconnectivity mediates the association of corpus callosum degeneration with memory impairment in AD amnestic MCI. Sci Rep. (2016) 6:32573. doi: 10.1038/srep32573
34. Yan CG, Craddock RC, He Y, Milham MP. Addressing head motion dependencies for small-world topologies in functional connectomics. Front Hum Neurosci. (2013) 7:910. doi: 10.3389/fnhum.2013.00910
35. Zuo XN, Kelly C, Di Martino A, Mennes M, Margulies DS, Bangaru S, et al. Growing together and growing apart: regional and sex differences in the lifespan developmental trajectories of functional homotopy. J Neurosci. (2010) 30:15034–43. doi: 10.1523/JNEUROSCI.2612-10.2010
36. Liao Y, Huang X, Wu Q, Yang C, Kuang W, Du M, et al. Is depression a disconnection syndrome? Meta-analysis of diffusion tensor imaging studies in patients with MDD. J Psychiatry Neurosci. (2013) 38:49–56. doi: 10.1503/jpn.110180
37. Davidson RJ, Jackson DC, Kalin NH. Emotion, plasticity, context, and regulation: perspectives from affective neuroscience. Psychol Bull. (2000) 126:890–909. doi: 10.1037/0033-2909.126.6.890
38. Wise T, Radua J, Nortje G, Cleare AJ, Young AH, Arnone D. Voxel-based meta-analytical evidence of structural disconnectivity in major depression and bipolar disorder. Biol Psychiatry. (2016) 79:293–302. doi: 10.1016/j.biopsych.2015.03.004
39. Dillon DG, Gonenc A, Belleau E, Pizzagalli DA. Depression is associated with dimensional and categorical effects on white matter pathways. Depress Anxiety. (2018) 35:440–7. doi: 10.1002/da.22734
40. Emsell L, Adamson C, De Winter FL, Billiet T, Christiaens D, Bouckaert F, et al. Corpus callosum macro and microstructure in late-life depression. J Affect Disord. (2017) 222:63–70. doi: 10.1016/j.jad.2017.06.063
41. Ozalay O, Calli C, Kitis O, Cagdas Eker M O., Donat Eker, Ozan E, et al. The relationship between the anterior corpus callosum size and prefrontal cortex volume in drug-free depressed patients. J Affect Disord. (2013) 146:281–5. doi: 10.1016/j.jad.2012.06.040
42. Rajkowska G, Miguel-Hidalgo JJ, Wei J, Dilley G, Pittman SD, Meltzer HY, et al. Morphometric evidence for neuronal and glial prefrontal cell pathology in major depression. Biol Psychiatry. (1999) 45:1085–98. doi: 10.1016/S0006-3223(99)00041-4
43. Ongur D, Drevets WC, Price JL. Glial reduction in the subgenual prefrontal cortex in mood disorders. Proc Natl Acad Sci USA. (1998) 95:13290–5. doi: 10.1073/pnas.95.22.13290
44. Drevets WC, Ongur D, Price JL. Neuroimaging abnormalities in the subgenual prefrontal cortex: implications for the pathophysiology of familial mood disorders. Mol Psychiatry. (1998) 3:220–6, 190–1. doi: 10.1038/sj.mp.4000370
45. Drevets WC, Price JL, Simpson JR Jr., Todd RD, Reich, Vannier M, et al. Subgenual prefrontal cortex abnormalities in mood disorders. Nature. (1997) 386:824–7. doi: 10.1038/386824a0
46. Schmahmann JD, Pandya DN, Wang R, Dai G, D'Arceuil HE, de Crespigny AJ, et al. Association fibre pathways of the brain: parallel observations from diffusion spectrum imaging and autoradiography. Brain. (2007) 130(Pt. 3):630–53. doi: 10.1093/brain/awl359
Keywords: depression, multimodal MRI, interhemispheric connectivity MDD, major depressive disorder, functional connectivity, voxel-mirrored homotopic connectivity, corpus callosum
Citation: Zheng G, Yingli Z, Shengli C, Zhifeng Z, Bo P, Gangqiang H and Yingwei Q (2022) Aberrant Inter-hemispheric Connectivity in Patients With Recurrent Major Depressive Disorder: A Multimodal MRI Study. Front. Neurol. 13:852330. doi: 10.3389/fneur.2022.852330
Received: 11 January 2022; Accepted: 11 March 2022;
Published: 08 April 2022.
Edited by:
Andrea Quattrone, University Magna Graecia, ItalyReviewed by:
Lena Kaethe Linda Oestreich, The University of Queensland, AustraliaGiuseppe Baselli, Politecnico di Milano, Italy
Copyright © 2022 Zheng, Yingli, Shengli, Zhifeng, Bo, Gangqiang and Yingwei. This is an open-access article distributed under the terms of the Creative Commons Attribution License (CC BY). The use, distribution or reproduction in other forums is permitted, provided the original author(s) and the copyright owner(s) are credited and that the original publication in this journal is cited, in accordance with accepted academic practice. No use, distribution or reproduction is permitted which does not comply with these terms.
*Correspondence: Hou Gangqiang, bmloYW9oZ3EmI3gwMDA0MDsxNjMuY29t
Qiu Yingwei, cWl1eXcxMjAxJiN4MDAwNDA7Z21haWwuY29t
†These authors have contributed equally to this work