- 1Department of Neurology, Johns Hopkins University School of Medicine, Baltimore, MD, United States
- 2Neuroscience Laboratory, Faculty of Medicine and Biomedical Sciences, The University of Yaoundé I, Brain Research Africa Initiative (BRAIN), Yaoundé, Cameroon
- 3Department of Infectious Diseases, Institute of Biomedicine, Sahlgrenska Academy at University of Gothenburg, Gothenburg, Sweden
- 4Department of Infectious Diseases, Region Västra Götaland, Sahlgrenska University Hospital, Gothenburg, Sweden
- 5Department of Neurology, University of California San Francisco (UCSF), San Francisco, CA, United States
This short review provides an overview of the interactions of human immunodeficiency virus type 1 (HIV), immune and inflammatory reactions, and CNS injury over the course of infection. Systemic infection is the overall driver of disease and serves as the “platform” for eventual CNS injury, setting the level of immune dysfunction and providing both the HIV seeding and immune-inflammatory responses to the CNS. These systemic processes determine the timing of and vulnerability to HIV-related neuronal injury which occurs in a separate “compartment” with features that parallel their systemic counterparts but also evolve independently. Direct CNS HIV infection, along with opportunistic infections, can have profound neurological consequences for the infected individual. HIV-related CNS morbidities are of worldwide importance but are enhanced by the particular epidemiological, socioeconomic and environmental factors that heighten the impact of HIV infection in Africa.
Introduction
HIV is a retrovirus taxonomically grouped in the genus Lentivirus (1) that entered the human population through multiple zoonotic infections from simian immunodeficiency virus–infected nonhuman primates (2). Its double-stranded RNA genome is more complex than many other retroviruses, and in addition to structural genes it contains several regulatory and accessory genes that contribute to its detailed life cycle, protracted course and pathological consequences. While all viral proteins presumably play a role in the character of infection, some have been singled out as particularly important in determining the character of CNS infection and its consequences. These include, for example, the env (envelope) gene that determines T-cell or macrophage tropism (T- or M-tropism) that dominate in different phases of CNS infection (3); Likewise, the accessory genes, including tat, may contribute to neurotoxicity (4). HIV is also subdivided into four groups with several subtypes or clades (5). The importance of group and clade variations for neurological complications, particularly those related to direct CNS infection, remains incompletely defined (6). This review focuses on emerging concepts in the neurobiology of more “direct” CNS complications of HIV-1 infection, particularly HIV-associated dementia (HAD) and, by inference, also milder cognitive impairments.
Clinical Background
HIV Epidemiology and Impact in Africa
Since its onset, the HIV pandemic has disproportionately impacted the African continent. While the first case definitions for AIDS were developed in 1982 (7), by the end of 2001 there were 40 million people living with HIV (PLWH), of whom 28.5 million (71%) were located in sub-Saharan Africa, at that time without access to antiretroviral therapy (ART) (8). While ART first became available to resource-rich countries in the 1990's, it took another decade of grass-roots political advocacy before ART first became more widely available in Africa through the United Nations Global Fund and the US President's Emergency Plan for AIDS Relief (9). Over the subsequent two decades, there has been tremendous progress in scaling up HIV care and treatment, and in 2021, 27.5 million PLWH globally were taking ART.
However, there remain important gaps. The prevalence of HIV in Africa varies widely among countries, from a low of <0.1% in Algeria and Egypt to more than 19% in South Africa, Botswana, Lesotho, and Eswatini (10). There remain 10.2 million PLWH who are not on HIV treatment, and in 2020 there were 1.5 million new HIV infections and 680,000 deaths (11). In sub-Saharan Africa, women and children are particularly vulnerable; in sub-Saharan Africa, women aged 15–49 make up 52% of new infections though they only represent 24% of the population. Older children (age 5–14 years) make up two-thirds of those not on treatment, and only 40% of children living with HIV had suppressed viral loads, as compared to 67% of adults (11).
CNS Disease in Africa
CNS complications of HIV are important causes of morbidity and mortality in Africa, and indeed globally (12). Descriptive epidemiology of HIV-associated CNS disease in Africa is limited by the availability of neurologists and advanced diagnostics such as computed tomography (CT), magnetic resonance imaging (MRI), and cerebrospinal fluid (CSF) analysis (13). Thus, many studies and clinical management decisions rely on syndromic clinical diagnoses with limited diagnostic precision, depending on the local resources. However, CNS opportunistic infections (OIs) are clearly common causes of hospitalization and may cause approximately 20% of deaths (14, 15). For disorders such as HIV-associated dementia (HAD) and, by inference, also milder cognitive impairments, diagnostic precision is even more limited.
Estimates of the prevalence of HIV-associated cognitive impairment have varied widely across the continent but are comparable to other world regions (16, 17) and have generally decreased as ART became more widely available (18–20). The prevalence of mild impairment was reported to be between 40 and 55% and moderate to severe impairment between 3 and 25% in two large multi-country cross-sectional and cohort studies using comprehensive neuropsychological test batteries in the African continent [the AIDS Clinical Trials Group 5199 (17, 21) and the African Cohort Study (22)], and in several larger studies from South Africa (23), Malawi (24), Tanzania (25) and Zimbabwe (26). Cognitive development is also impacted in pediatric HIV, where infants and young children with HIV do not perform as well as their HIV-exposed or HIV uninfected peers (27–30).
The variation in estimates of HIV-associated cognitive impairment in across Africa may be due in part to the use of tests with limited cultural validity, lack of well-matched norms and relying on screening tools with limited sensitivity and specificity when resources for neuropsychological testing are limited (31–33). In particular, the clinical relevance of mild impairment on neuropsychological tests in African populations is unclear (31) and test performance is impacted by literacy (22) and education level (23). HIV-uninfected individuals often perform poorly on tests (22), there is significant between country variation in normative data (21), and particularly among older individuals, there may be no group level differences observed between HIV-infected and -uninfected individuals (34, 35).
Pathophysiology: HIV Neuroimmune-Virus Interactions and Their Impact on the CNS
Among the viruses considered in this collection, HIV likely has the most complex and intimate interactions with the immune system and inflammatory responses, both outside (i.e., systemically) and within the CNS. In both systemic and CNS compartments these interactions change over the long course of chronic infection (36, 37). Figure 1 diagrams these interactions, dividing the systemic (left) from CNS (right) processes. The elements in these two compartments interact, and more particularly, systemic HIV disease serves as the foundation for the CNS complications in several aspects. It establishes the conditions of immunosuppression and immune activation that underlie CNS vulnerability (37–40), and, more directly, supplies the key elements of neuropathogenesis, including HIV invasion and major blood-derived cells involved in CNS immune-inflammatory reactions. However, while CNS virus-immune interactions partially echo those occurring systemically, there are important differences, with the CNS interactions being highly compartmentalized despite these systemic origins (36).
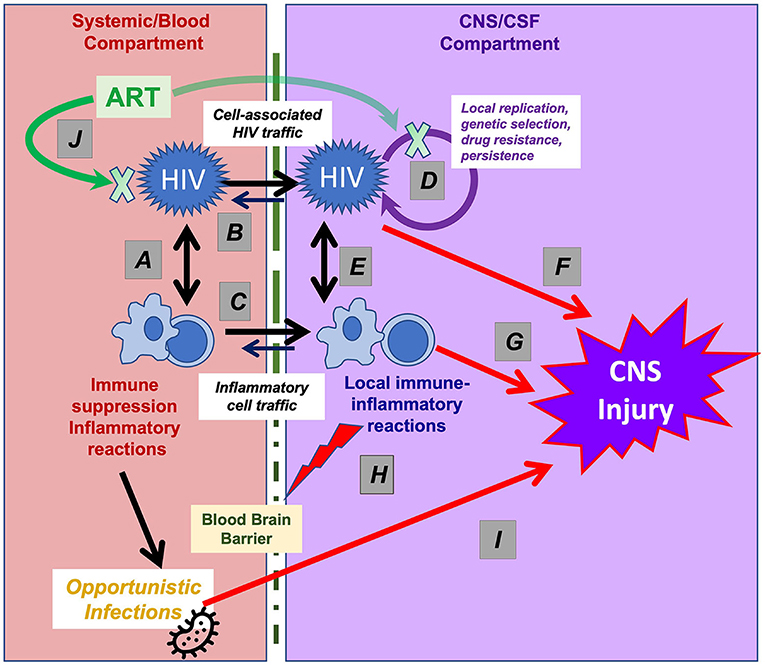
Figure 1. Interactions of HIV and immune-inflammatory responses in systemic and CNS infection. This simplified schematic outlines the systemic and CNS viral-immune interactions that determine the immunopathogenesis of CNS injury. Systemic interactions (shown in the left part of the figure) establish the foundation for CNS vulnerability that are partly echoed by interactions within the CNS (right part of the figure), though with important differences. (A) HIV targets CD4+ T lymphocytes (and to a lesser degree myeloid cells) in which viral replication both sustains viremia and establishes long-term viral persistence, leading to gradual T-cell loss and immunosuppression and to lifelong infection. Virus-induced T-cell activation, in turn, enhances viral replication and dissemination. (B) Systemic viremia is the source of CNS HIV infection, beginning early in infection, likely mainly via infected T cells that migrate through the blood-brain barrier (BBB, depicted by vertical dotted line). (C) Cells important to the CNS inflammatory response also derive from blood sources; these include CD4+ and CD8+ T cells and macrophages that elaborate cytokines and other signaling and toxic molecules that contribute to the compartmentalized CNS inflammatory response within the CNS and are reflected in CSF. (D) HIV can replicate locally within these migrating CD4+ T cells and macrophages sustaining a genetically independent infection and perhaps establishing a longer-lived second viral reservoir within the CNS. (E) The interaction of the local HIV infection with “imported” inflammatory cells and native CNS cells (including astrocytes and microglia) establish an independent inflammatory milieu that evolves over the course of disease and is particularly heightened in HAD/HIVE. (F) Both HIV gene products and (G) host inflammatory reactions likely contribute to ‘indirect’ CNS injury. (H) inflammatory reactions can disrupt the blood-brain barrier, further exacerbating this injury. (I) CNS OIs may involve a similar pathway, first with loss of systemic immune surveillance allowing entry or activation of pathogens that then invade the CNS and cause neurological disease by direct injury or through a local inflammatory response. (J) ART reverses or mitigates all of these processes. By suppressing HIV replication, treatment fosters a variable degree of CD4+ T cells restoration and partial reversal of these pathological processes. Abrupt restoration of immunity may lead to robust local inflammation and the immune restoration inflammatory response (IRIS) with exacerbation of neurological symptoms and signs. The blood-brain barrier variably impedes CNS concentrations of certain drug components of ART, delaying or reducing local antiviral effects and, in rare cases, contributing to the development of neurosymptomatic CSF escape despite systemic viral suppression.
In both systemic and CNS compartments the interactions of HIV and immune reactions evolve in important ways over the protracted course of chronic untreated infection. While the CNS infection echoes its systemic counterpart, it also diverges in important details, including in virus populations and particular inflammatory profiles (36, 41). If unchecked by ART this chronic course may be complicated by a range of disorders afflicting the brain, including major OIs and direct neuropathic HIV CNS infection (42, 43). Because of space constraints, this review omits detailed discussion of CNS OIs as well as disorders of the spinal cord and peripheral nervous system (PNS) that may be impacted by similar disease processes (44).
Progressive Systemic HIV Infection: Prerequisite and Facilitator of Major AIDS-Associated CNS Diseases
A number of the features of systemic HIV infection are important for the development of CNS HIV infection and disease. Ultimately, these stem from the fact that CD4+ T lymphocytes and, to a lesser extent, macrophages and related myeloid cells, are the main cellular targets of HIV infection (45–50). This targeted infection leads to progressive immunosuppression and also to a state of enhanced immunoactivation, with both contributing to CNS disease consequences (51–54). HIV infection is chronic and persistent, but importantly mitigated by ART. It remains, however, a major challenge to therapeutic cure efforts (55, 56), and stopping ART almost inevitably leads to a return of viremia accompanied by CSF viral rebound (57, 58).
Complications of HIV vary with the stage of systemic disease progression, most easily assessed by the blood CD4+ T lymphocyte count (38, 59, 60). AIDS is defined by the development of major OIs (and, in parallel, including HIV-associated dementia, HAD) or by a CD4+ count falling below 200 per μl (61).
CNS OIs develop when there is loss of systemic immune surveillance that allows certain organisms to escape a latent or quiescent presence in the body (e.g., JC virus or Toxoplassma gondii) or to evade defenses that would otherwise prevent systemic dissemination (e.g., Cryptococcus neoformans); this is followed by subsequent failure of these same defenses to eliminate these pathogens within the CNS. The spectrum of common CNS OIs is relatively circumscribed and involves organisms of relatively low pathogenicity that are otherwise readily contained or prevented by T-cell/macrophage defenses in the normal host. In Africa where M. tuberculosis is common in the community, HIV infection also enhances susceptibility even if this organism isn't readily classified as strictly “opportunistic” and is more common even at CD4 counts above those defining AIDS (15). However, the common CNS OIs generally occur at <200 CD4+ cells/μl (43). We emphasize this well-known susceptibility here because this also defines the susceptibility to HIV encephalitis (HIVE) and HAD which usually develops below this T-cell threshold (62), indicating that a similar level of immunosuppression is a prerequisite. In a sense HIVE might also be viewed as a CNS OI in which the same virus “creates the opportunity” through chronic systemic infection of CD4+ T cells before it can then “opportunistically” cause encephalitis. However, as discussed below, this does not apply to overall susceptibility to CNS HIV infection per se, but to “invasive” neuropathic encephalitic infection. In fact, low-grade HIV-1 meningeal infection is a common feature of systemic HIV infection that develops early in its course (37). The CNS is exposed to HIV very early in systemic infection, though it is often silent or accompanied by headache, fatigue or other unspecific symptoms. More rarely, acute encephalitis may develop during primary infection, likely involving an immunological pathogenesis (63). Over the course of chronic infection, milder neurocognitive impairment may develop and relate to low-grade forms of the viral and immunological processes that underlie HAD/HIVE, though these connections remain to be more precisely defined.
Systemic Origin of the Elements of CNS Infection
In addition to providing the background foundation and necessary level of immunosuppression for OIs and HIVE, systemic infection more directly underlies HIV CNS disease by providing both the invading virus and principal inflammatory cells that react to infection and contribute to immunopathology.
Most probably, HIV seeding of the CNS occurs via trafficking infected CD4+ T cells rather than by more direct virion penetration of the blood-brain barrier (64, 65). Infected cells entering the CNS can clonally expand and release (clonal) virus; this can then lead to further infection of susceptible cells, amplifying infection and establishing local replication (66). During later stage infection monocytes may also enter the CNS (65–70). This later CNS infection may be more compartmentalized with more notable evolution of virus populations independent of those examined in blood. Uninfected CD4+ T cells and monocytes may also enter the CNS contributing to amplified infection. This can also lead to local CNS HIV persistence after treatment, though, this has been less clearly defined, including the types of cells and anatomic locations, state of viral expression and mechanisms of replication control.
Dynamics of CNS Infection With Disease Progression: Transition From Meningitis to Encephalitis
A central feature of CNS HIV infection is its changing character with systemic disease progression. This includes shifts in the relation of CSF and blood viral populations (71–73), changes in the accompanying inflammatory profiles (36) and eventual shift in the main anatomic site of productive infection from the leptomeninges to the brain in some individuals. In the earlier phase of infection when blood CD4+ T cell levels are above 200 per μl, the leptomeninges are the most conspicuous location of chronic CNS HIV-1 infection so that a clinically silent aseptic meningitis is frequent. This infection is largely “equilibrated” with CSF HIV RNA concentrations maintained at levels near 10 percent of those in blood (37, 74, 75), and CSF and blood populations are genetically similar (76), presumably because of continuous and fresh virus traffic from blood to CSF. When CD4 cells fall below 50/μl, the ratio of CSF to blood virus deceases to near 1% blood HIV RNA levels as CSF pleocytosis also diminishes, consistent with a relation between CSF WBCs and viral load (77–80). The extent of penetration of infection into the brain parenchyma at this stage is uncertain, but if present it is largely clinically silent. Whether this early type of infection and inflammation is responsible for milder cognitive impairment is still not definitively established, though often presumed.
These relationships change in those who develop HIVE that presents clinically as subacute HAD (36). This condition usually develops after blood CD4+ cells fall below 200/μl and represents an extension of infection from meninges into the brain parenchyma. White matter abnormalities are usually prominent on MRI but gray matter also is frequently affected, particularly the basal ganglia (81–83). While inflammation in those without HIVE largely involves lymphocyte-related cytokines, as CD4+ T cell counts fall, macrophage-related inflammation increases. In those with overt HIVE there is augmentation of both lymphocytic and macrophage biomarkers (36). CNS viral populations in these individuals are more compartmentalized in relation to those in blood, and exhibit macrophage tropism (76, 84). While astrocytes can be infected by HIV, this is usually considered to be non-productive with limited gene expression; hence, their role in persistence and neuropathogenesis is still uncertain (85, 86). Importantly, neurons are not infected, and thus damage to neurons is largely or exclusively by “indirect” mechanisms, meaning that they are injured from without by signals and toxins released by neighboring cells rather than from direct effects of viral genes and their products expressed within these cells (87). Likely the external toxic signals are elaborated mainly from inflammatory cells, perhaps predominantly from macrophages and other myeloid cells. Late in infection HIVE also commonly disrupts the blood-brain barrier, further contributing to neuronal injury and dysfunction (36, 88, 89).
Impact of ART on CNS Infection and Disease
ART has had a profound effect on preserving CNS integrity, both in preventing HAD/HIVE development and in mitigating this CNS disease after it manifests (90, 91). This effect may be in part through preservation or restoration of immunity but mainly by more directly suppressing both systemic viremia and HIV replication within the CNS. As a result, HAD incidence is now markedly reduced and confined largely to those not receiving ART.
For individuals who present with HAD, having fallen through defects in the treatment network, ART can arrest and often reverse the severity of its impact, depending on the time frame of HAD development and treatment initiation. Diagnosis should be made quickly, and treatment begun rapidly. This is a setting in which both the antiviral potency and CNS penetration of the components ART regimens are likely important (92, 93). In some of treated individuals the degree of short- and long-term recovery can be remarkable.
CSF Escape
This term refers to situations in which the impact of ART on CNS HIV infection is relatively reduced compared to that on systemic infection, leading to CSF HIV RNA levels exceeding those of plasma (94–98). Three distinct types of CSF escape have been defined: asymptomatic, neurosymptomatic and secondary. The most important of these is neurosymptomatic CSF escape in which ART-treated individuals present with new or progressive neurological deficits (96–100). Most often, in addition to symptoms and signs of CNS injury and dysfunction, there is CSF pleocytosis, elevated CSF neurofilament light chain protein (NfL) concentration, and neuroimaging abnormalities consistent with active CNS HIV infection. Neurosymptomatic escape overlaps with pathologically-defined CD8 encephalitis (101–103). In most cases a background of reduced treatment adherence and drug resistance, at times in combination with insufficient CNS penetration of component antiviral drugs, can be identified (96). This provides further support for the need for targeted treatment of CNS, at least in some settings. Inflammation and immunopathology may be an important mechanistic component in this setting in which CD4+ T cell counts are higher than in HAD/HIVE because of the disproportionate systemic efficacy of ART that fosters CD4+ cell recovery and suppresses systemic viremia. CNS HIV isolates often exhibit drug resistance, though not always. The main avenue of treatment is changing the ART regimen to a potent antiviral drug combination that includes component drugs to which the CSF/CNS virus is susceptible and also achieve therapeutic brain concentrations.
The other two forms of CSF escape are of less clinical importance. Asymptomatic escape is an incidental finding mainly in CSF cohort studies. It is characterized by detectable CSF HIV RNA in the presence of plasma viral suppression; CSF HIV RNA levels are usually low with little or no pleocytosis. By definition these individuals lack new neurological symptoms or signs (104, 105). Secondary escape entails a disproportional increase in CSF HIV RNA in association with another CNS inflammatory process (usually another CNS infection) that provokes local HIV replication through recruitment of activated lymphocytes. Treatment of the provoking infection leads to reduction of the CSF HIV RNA elevation (79).
CNS Persistence and Cure
Despite the effectiveness of ART in suppressing systemic and CSF HIV infection, it does not cure HIV. When ART is stopped, viremia and CNS replication re-emerge (106). Because of this intractable persistence of HIV, efforts are now underway to effect a systemic cure using a variety of strategies (55). There is precedent with bone marrow transplant using an HIV-resistant donor. In one well-studied case, not only was there no evidence of viral persistence systemically but also no trace of virus in CSF (107). More broadly it remains an open issue as to whether the CNS serves as an independent viral reservoir that might require CNS-targeted cure strategies.
Conclusion
CNS HIV infection is a component of the “ecology” of HIV, an offshoot of systemic viremia that can lead to important morbidity and mortality. Fortunately, ART has a major impact on CNS infection and its effects, from Pre-Exposure Prophylaxis (PrEP) preventing initial infection, to early treatment of infection that likely reduces the CNS reservoir (91), to treatment of established HAD/HIVE. Thus, while additional interventions, including vaccines and cure efforts are welcome, widespread use of preventative and therapeutic ART continue to have a major impact on neurological disease in HIV and AIDS.
Author Contributions
All authors listed have made a substantial, direct, and contribution to the work and approved it for publication.
Conflict of Interest
MG has received research grants from Abbott, Baxter, Bristol-Myers Squibb, Gilead Sciences, GlaxoSmithKline, Merck, Pfizer, Roche and Tibotec, and he has received honoraria as a speaker and/or scientific advisor from Abbott/Abbvie, Amgen, Biogen, Bioinvent, Boehringer-Ingelheim, Bristol-Myers Squibb, Gilead Sciences, GlaxoSmithKline, Janssen-Cilag, MSD, Novocure, Novo Nordic, Pfizer, Roche and Tibotec. A-CM is a paid employee of Denali Therapeutics.
The remaining authors declare that the research was conducted in the absence of any commercial or financial relationships that could be construed as a potential conflict of interest.
Publisher's Note
All claims expressed in this article are solely those of the authors and do not necessarily represent those of their affiliated organizations, or those of the publisher, the editors and the reviewers. Any product that may be evaluated in this article, or claim that may be made by its manufacturer, is not guaranteed or endorsed by the publisher.
References
1. Vogt VM. Retroviral virions and genomes. In: Coffin JM, Hughes SH, Varmus HE. editors. Retroviruses. Harbor, NY:Cold Spring (1997).
2. Korber B, Muldoon M, Theiler J, Gao F, Gupta R, Lapedes A, et al. Timing the ancestor of the HIV-1 pandemic strains. Science. (2000) 288:1789–96. doi: 10.1126/science.288.5472.1789
3. Arrildt KT, Joseph SB, Swanstrom R. The HIV-1 env protein: a coat of many colors. Curr HIV/AIDS Rep. (2012) 9:52–63. doi: 10.1007/s11904-011-0107-3
4. Nath A, Steiner J. Synaptodendritic injury with HIV-Tat protein: what is the therapeutic target? Exp Neurol. (2014) 251:112–4. doi: 10.1016/j.expneurol.2013.11.004
5. Robertson DL, Anderson JP, Bradac JA, Carr JK, Foley B, Funkhouser RK, et al. HIV-1 nomenclature proposal. Science. (2000) 288:55–6. doi: 10.1126/science.288.5463.55d
6. Tyor W, Fritz-French C, Nath A. Effect of HIV clade differences on the onset and severity of HIV-associated neurocognitive disorders. J Neurovirol. (2013) 19:515–22. doi: 10.1007/s13365-013-0206-6
7. Quinn TC. Forty years of AIDS: a retrospective and the way forward. J Clin Invest. (2021) 131:e154196: doi: 10.1172/JCI154196
8. JUNPO (UNAIDS) HA. Report on the Global HIV/AIDS Epidemic. Fighting AIDS A New Global Resolve (2002).
9. Fauci AS, Eisinger RW. PEPFAR - 15 Years and Counting the Lives Saved. N Engl J Med. (2018) 378:314–316. doi: 10.1056/NEJMp1714773
10. National AID Sinfo. HIV Prevalence- Adults (15-49) Global data on HIV Epidemiology and Response. National AID Sinfo (2020).
11. Joint Joint United Nations Programme on HIV/AIDS (UNAIDS) Global AIDS Update 2021. Confronting Inequalities: Lessons for Pandemic Responses from 40 Years of AIDS, Geneva, Switzerland. UNAIDS (2021).
12. Hogan C, Wilkins E. Neurological complications in HIV. Clin Med. (2011) 11:571–5. doi: 10.7861/clinmedicine.11-6-571
13. World Health Organization. Atlas: Country Resources for Neurological Disorders. 2nd ed. Geneva: World Health Organization (2017).
14. Howlett WP. Neurological disorders in HIV in Africa: a review. Afr Health Sci. (2019) 19:1953-77. doi: 10.4314/ahs.v19i2.19
15. Ghislain MR, Mushebenge GA, Magula N. Cause of hospitalization and death in the antiretroviral era in Sub-Saharan Africa published 2008-2018: a systematic review. Medicine. (2021) 100:e27342. doi: 10.1097/MD.0000000000027342
16. Wang Y, Liu M, Lu Q, Farrell M, Lappin JM, Shi J, et al. Global prevalence and burden of HIV-associated neurocognitive disorder: A meta-analysis. Neurology. (2020) 95:e2610–21. doi: 10.1212/WNL.0000000000010752
17. Robertson K, Jiang H, Kumwenda J, Supparatpinyo K, Evans S, Campbell TB, et al. Improved neuropsychological and neurological functioning across three antiretroviral regimens in diverse resource-limited settings: AIDS Clinical Trials Group study a5199, the International Neurological Study. Clin Infect Dis. (2012) 55:868–76. doi: 10.1093/cid/cis507
18. Habib AG, Yakasai AM, Owolabi LF, Ibrahim A, Habib ZG, Gudaji M, et al. Neurocognitive impairment in HIV-1-infected adults in Sub-Saharan Africa: a systematic review and meta-analysis. Int J Infect Dis. (2013) 17:e820–31. doi: 10.1016/j.ijid.2013.06.011
19. Michael HU, Naidoo S, Mensah KB, Ramlall S, Oosthuizen F. The impact of antiretroviral therapy on neurocognitive outcomes among people living with HIV in Low- and Middle-Income Countries (LMICs): a systematic review. AIDS Behav. (2021) 25:492–523. doi: 10.1007/s10461-020-03008-8
20. Vecchio A, Robertson K, Saylor D, Nakigozi G, Nakasujja N, Kisakye A, et al. Neurocognitive effects of antiretroviral initiation among people living with hiv in rural Uganda. J Acquir Immune Defic Syndr. (2020) 84:534–42. doi: 10.1097/QAI.0000000000002385
21. Robertson K, Jiang H, Evans SR, Marra CM, Berzins B, Hakim J, et al. International neurocognitive normative study: neurocognitive comparison data in diverse resource-limited settings: AIDS Clinical Trials Group A5271. J Neurovirol. (2016) 22:472–8. doi: 10.1007/s13365-015-0415-2
22. Milanini B, Allen I, Paul R, Bahemana E, Kiweewa F, Nambuya A, et al. Frequency and predictors of HIV-related cognitive impairment in East Africa: The Africa Cohort Study (AFRICOS). J Acquir Immune Defic Syndr. (2020) 83:157–64. doi: 10.1097/QAI.0000000000002242
23. Joska JA, Westgarth-Taylor J, Myer L, Hoare J, Thomas KG, Combrinck M, et al. Characterization of HIV-Associated Neurocognitive Disorders among individuals starting antiretroviral therapy in South Africa. AIDS Behav. (2011) 15:1197–203. doi: 10.1007/s10461-010-9744-6
24. Kelly CM, van Oosterhout JJ, Ngwalo C, Stewart RC, Benjamin L, Robertson KR, et al. HIV associated neurocognitive disorders (HAND) in Malawian adults and effect on adherence to combination anti-retroviral therapy: a cross sectional study. PLoS ONE. (2014) 9:e98962. doi: 10.1371/journal.pone.0098962
25. Sanmartí M, Meyer AC, Jaen A, Robertson K, Tan N, Mapesi H, et al. HIV-associated neurocognitive impairment in stable people living with HIV on ART in rural Tanzania. HIV Med. (2021) 22:102–12. doi: 10.1111/hiv.12979
26. Nyamayaro P, Gouse H, Hakim J, Robbins RN, Chibanda D. Neurocognitive impairment in treatment-experienced adults living with HIV attending primary care clinics in Zimbabwe. BMC Infect Dis. (2020) 20:e383. doi: 10.1186/s12879-020-05090-8
27. Sherr L, Hensels IS, Tomlinson M, Skeen S, Macedo A. Cognitive and physical development in HIV-positive children in South Africa and Malawi: a community-based follow-up comparison study. Child Care Health Dev. (2018) 44:89–98. doi: 10.1111/cch.12533
28. le Roux SM, Donald KA, Brittain K, Phillips TK, Zerbe A, Nguyen KK, et al. Neurodevelopment of breastfed HIV-exposed uninfected and HIV-unexposed children in South Africa. AIDS. (2018) 32:1781–91. doi: 10.1097/QAD.0000000000001872
29. Gruver RS, Mall S, Kvalsvig JD, Knox JR, Mellins CA, Desmond C, et al. Cognitive and language development at age 4-6 years in children HIV-exposed but uninfected compared to those HIV-unexposed and to children living with HIV. New Dir Child Adolesc Dev. (2020) 2020:39–54. doi: 10.1002/cad.20351
30. Struyf T, Dube Q, Cromwell EA, Sheahan AD, Heyderman RS, Van Rie A. The effect of HIV infection and exposure on cognitive development in the first two years of life in Malawi. Eur J Paediatr Neurol. (2020) 25:157–64. doi: 10.1016/j.ejpn.2019.11.004
31. Nightingale S, Dreyer AJ, Saylor D, Gisslén M, Winston A, Joska JA. Moving on from hand: why we need new criteria for cognitive impairment in persons living with human immunodeficiency virus and a proposed way forward. Clin Infect Dis. (2021) 73:1113–8. doi: 10.1093/cid/ciab366
32. Mwangala PN, Newton CR, Abas M, Abubakar A. Screening tools for HIV-associated neurocognitive disorders among adults living with HIV in sub-Saharan Africa: a scoping review. AAS Open Res. (2018) 1:28. doi: 10.12688/aasopenres.12921.1
33. Haddow LJ, Floyd S, Copas A, Gilson RJ. A systematic review of the screening accuracy of the HIV Dementia Scale and International HIV Dementia Scale. PLoS ONE. (2013) 8:e61826. doi: 10.1371/journal.pone.0061826
34. Asiimwe SB, Farrell M, Kobayashi LC, Manne-Goehler J, Kahn K, Tollman SM, et al. Cognitive differences associated with HIV serostatus and antiretroviral therapy use in a population-based sample of older adults in South Africa. Sci Rep. (2020) 10:16625. doi: 10.1038/s41598-020-73689-7
35. Bernard C, Font H, Diallo Z, Ahonon R, Tine JM, Abouo FN, et al. de rekeneire, effects of age, level of education and hiv status on cognitive performance in west african older adults: The West Africa Iedea Cohort Collaboration. AIDS Behav. (2021) 25:3316–26. doi: 10.1007/s10461-021-03309-6
36. Gisslen M, Keating SM, Spudich S, Arechiga V, Stephenson S, Zetterberg H, et al. Compartmentalization of cerebrospinal fluid inflammation across the spectrum of untreated HIV-1 infection, central nervous system injury and viral suppression. PLoS ONE. (2021) 16:e0250987. doi: 10.1371/journal.pone.0250987
37. Ulfhammer G, Eden A, Antinori A, Brew BJ, Calcagno A, Cinque P, et al. Cerebrospinal fluid viral load across the spectrum of untreated HIV-1 infection: a cross-sectional multi-center study. Clin Infect Dis. ciab943. (2021). doi: 10.1093/cid/ciab943. [Epub ahead of print].
38. Fitri FI, Rambe AS, Fitri A. Correlation between Lymphocyte CD4 Count, treatment duration, opportunistic infection and cognitive function in human immunodeficiency virus-acquired immunodeficiency syndrome (HIV-AIDS) Patients. Open Access Maced J Med Sci. (2018) 6:643–7. doi: 10.3889/oamjms.2018.152
39. Ellis RJ, Badiee J, Vaida F, Letendre S, Heaton RK, Clifford D, et al. CD4 nadir is a predictor of HIV neurocognitive impairment in the era of combination antiretroviral therapy. AIDS. (2011) 25:1747–51. doi: 10.1097/QAD.0b013e32834a40cd
40. Gisslen M, Fuchs D, Svennerholm B, Hagberg L. Cerebrospinal fluid viral load, intrathecal immunoactivation, and cerebrospinal fluid monocytic cell count in HIV-1 infection. J Acquir Immune Defic Syndr. (1999) 21:271–6. doi: 10.1097/00126334-199908010-00003
41. Adewumi OM, Dukhovlinova E, Shehu NY, Zhou S, Council OD, Akanbi MO, et al. HIV-1 central nervous system compartmentalization and cytokine interplay in non-subtype B HIV-1 infections in Nigeria and Malawi. AIDS Res Hum Retroviruses. (2020) 36:490–500. doi: 10.1089/aid.2019.0245
42. Le LT, Spudich SS. HIV-associated neurologic disorders and central nervous system opportunistic infections in HIV. Semin Neurol. (2016) 36:373–81. doi: 10.1055/s-0036-1585454
43. Pruitt AA. Central nervous system infections in immunocompromised patients. Curr Neurol Neurosci Rep. (2021) 21:37. doi: 10.1007/s11910-021-01119-w
44. Mochan A, Anderson D, Modi G. CIDP in a HIV endemic population: A prospective case series from Johannesburg, South Africa. J Neurol Sci. (2016) 363:39–42. doi: 10.1016/j.jns.2015.11.013
45. Joseph SB, Arrildt KT, Sturdevant CB, Swanstrom R. HIV-1 target cells in the CNS. J Neurovirol. (2015) 21:276–89. doi: 10.1007/s13365-014-0287-x
46. Aiamkitsumrit B, Sullivan NT, Nonnemacher MR, Pirrone V, Wigdahl B. Human immunodeficiency virus type 1 cellular entry and exit in the T Lymphocytic and monocytic compartments: mechanisms and target opportunities during viral disease. Adv Virus Res. (2015) 93:257–311. doi: 10.1016/bs.aivir.2015.04.001
47. Cenker JJ, Stultz RD, McDonald D. Brain microglial cells are highly susceptible to HIV-1 infection and spread. AIDS Res Hum Retroviruses. (2017) 33:1155–65. doi: 10.1089/aid.2017.0004
48. Karris MA, Smith DM. Tissue-specific HIV-1 infection: why it matters. Future Virol. (2011) 6:869–82. doi: 10.2217/fvl.11.48
49. Swanstrom R, Coffin J. HIV-1 pathogenesis: the virus. Cold Spring Harbor Perspect Med. (2012) 2:a007443. doi: 10.1101/cshperspect.a007443
50. Stevenson M. Role of myeloid cells in HIV-1-host interplay. J Neurovirol. (2015) 21:242–8. doi: 10.1007/s13365-014-0281-3
51. Pahwa S, Deeks S, Zou S, Tomitch N, Miller-Novak L, Caler E, et al. NIH workshop on HIV-associated comorbidities, coinfections, and complications: summary and recommendation for future research. J Acquir Immune Defic Syndr. (2021) 86:11–8. doi: 10.1097/QAI.0000000000002528
52. Serrano-Villar S, Sainz T, Lee SA, Hunt PW, Sinclair E, Shacklett BL, et al. HIV-infected individuals with low CD4/CD8 ratio despite effective antiretroviral therapy exhibit altered T cell subsets, heightened CD8+ T cell activation, and increased risk of non-AIDS morbidity and mortality. PLoS Pathog. (2014) 10:e1004078. doi: 10.1371/journal.ppat.1004078
53. Utay NS, Hunt PW. Role of immune activation in progression to AIDS. Curr Opin HIV AIDS. (2016) 11:131–7. doi: 10.1097/COH.0000000000000242
54. Wang RJ, Moore J, Moisi D, Chang EG, Byanyima P, Kaswabuli S, et al. HIV infection is associated with elevated biomarkers of immune activation in Ugandan adults with pneumonia. PLoS ONE. (2019) 14:e0216680. doi: 10.1371/journal.pone.0216680
55. Deeks SG, Archin N, Cannon P, Collins S, Jones RB, de Jong M, et al. International, research priorities for an HIV cure: International AIDS Society Global Scientific Strategy 2021. Nat Med. (2021) 27:2085-98. doi: 10.1038/s41591-021-01590-5
56. Lewin SR, Attoye T, Bansbach C, Doehle B, Dube K, Dybul M, et al. Sunnylands Working, Multi-stakeholder consensus on a target product profile for an HIV cure. Lancet HIV. (2021) 8:e42–50. doi: 10.1016/S2352-3018(20)30234-4
57. Hellmuth J, Muccini C, Colby DJ, Kroon E, de Souza M, Crowell TA, et al. Central nervous system safety during brief analytic treatment interruption of antiretroviral therapy within 4 human immunodeficiency virus remission trials: an observational study in acutely treated people living with human immunodeficiency virus. Clin Infect Dis. (2021) 73:e1885–92. doi: 10.1093/cid/ciaa1344
58. Henrich TJ, Hanhauser E, Marty FM, Sirignano MN, Keating S, Lee TH, et al. Antiretroviral-free HIV-1 remission and viral rebound after allogeneic stem cell transplantation: report of 2 cases. Ann Intern Med. (2014) 161:319–27. doi: 10.7326/M14-1027
59. Lundgren JD, Babiker A, El-Sadr W, Emery S, Grund B, Neaton JD., Strategies for Management of Antiretroviral Therapy Study Group, et al. Inferior clinical outcome of the CD4+ cell count-guided antiretroviral treatment interruption strategy in the SMART study: role of CD4+ Cell counts and HIV RNA levels during follow-up. J Infect Dis. (2008) 197:1145–55. doi: 10.1086/529523
60. Baker JV, Henry WK, Neaton JD. The consequences of HIV infection and antiretroviral therapy use for cardiovascular disease risk: shifting paradigms. Curr Opin HIV AIDS. (2009) 4:176–82. doi: 10.1097/COH.0b013e328329c62f
61. revised classification system for HIV infection and expanded surveillance case definition for AIDS among adolescents and adults. MMWR Recomm Rep. (1992) 41:1-19.
62. Navia BA, Jordan BD, Price RW. The AIDS dementia complex: I. Clin Features Annals Neurol. (1986) 19:517–24. doi: 10.1002/ana.410190602
63. Valcour V, Chalermchai T, Sailasuta N, Marovich M, Lerdlum S, Suttichom D, et al. Central nervous system viral invasion and inflammation during acute HIV infection. J Infect Dis. (2012) 206:275–82. doi: 10.1093/infdis/jis326
64. Miller F, Afonso PV, Gessain A, Ceccaldi PE. Blood-brain barrier and retroviral infections. Virulence. (2012) 3:222–9. doi: 10.4161/viru.19697
65. Wu DT, Woodman SE, Weiss JM, McManus CM, D'Aversa TG, Hesselgesser J, et al. Mechanisms of leukocyte trafficking into the CNS. J Neurovirol. (2000) 6(Supp 1):S82–5.
66. Coffin J, Swanstrom R. HIV pathogenesis: dynamics and genetics of viral populations and infected cells. Cold Spring Harbor Perspect Med. (2013) 3:a012526. doi: 10.1101/cshperspect.a012526
67. Burdo TH, Lackner A, Williams KC. Monocyte/macrophages and their role in HIV neuropathogenesis. Immunol Rev. (2013) 254:102–13. doi: 10.1111/imr.12068
68. Honeycutt JB, Wahl A, Baker C, Spagnuolo RA, Foster J, Zakharova O, et al. Macrophages sustain HIV replication in vivo independently of T cells. J Clin Investig. (2016) 126:1353–66. doi: 10.1172/JCI84456
69. Kruize Z, Kootstra NA. The role of macrophages in HIV-1 persistence and pathogenesis. Front Microbiol. (2019) 10:e2828. doi: 10.3389/fmicb.2019.02828
70. Leon-Rivera R, Veenstra M, Donoso M, Tell E, Eugenin EA, Morgello S, et al. Central Nervous System (CNS) viral seeding by mature monocytes and potential therapies to reduce CNS viral reservoirs in the cART Era. mBio. (2021) 12:e03633-20. doi: 10.1128/mBio.03633-20
71. Schnell G, Joseph S, Spudich S, Price RW, Swanstrom R. HIV-1 replication in the central nervous system occurs in two distinct cell types. PLoS Pathog. (2011) 7:e1002286. doi: 10.1371/journal.ppat.1002286
72. Schnell G, Price RW, Swanstrom R, Spudich S. Compartmentalization and clonal amplification of HIV-1 variants in the cerebrospinal fluid during primary infection. J Virol. (2010) 84:2395–407. doi: 10.1128/JVI.01863-09
73. Strain MC, Letendre S, Pillai SK, Russell T, Ignacio CC, Gunthard HF, et al. Genetic composition of human immunodeficiency virus type 1 in cerebrospinal fluid and blood without treatment and during failing antiretroviral therapy. J Virol. (2005) 79:1772–88. doi: 10.1128/JVI.79.3.1772-1788.2005
74. Price RW, Spudich S. Antiretroviral therapy and central nervous system HIV type 1 infection. J Infect Dis. (2008) 3(Suppl. 3):S294–306. doi: 10.1086/533419
75. Spudich SS, Nilsson AC, Lollo ND, Liegler TJ, Petropoulos CJ, Deeks SG, et al. Cerebrospinal fluid HIV infection and pleocytosis: relation to systemic infection and antiretroviral treatment. BMC Infect Dis. (2005) 5:e98. doi: 10.1186/1471-2334-5-98
76. Sturdevant CB, Joseph SB, Schnell G, Price RW, Swanstrom R, Spudich S. Compartmentalized replication of R5 T cell-tropic HIV-1 in the central nervous system early in the course of infection. PLoS Pathogens. (2015) 11:e1004720. doi: 10.1371/journal.ppat.1004720
77. de Almeida SM, Rotta I, de Pereira AP, Tang B, Umlauf A, Ribeiro CEL., HIV Neurobehavioral Research Center (HNRC) Group, et al. Cerebrospinal fluid pleocytosis as a predictive factor for CSF and plasma HIV RNA discordance and escape. J Neurovirol. (2020) 26:241–51. doi: 10.1007/s13365-020-00828-1
78. Ellis RJ, Gamst AC, Capparelli E, Spector SA, Hsia K, Wolfson T, et al. Cerebrospinal fluid HIV RNA originates from both local CNS and systemic sources. Neurology. (2000) 54:927–36. doi: 10.1212/WNL.54.4.927
79. Hagberg L, Price RW, Zetterberg H, Fuchs D, Gisslen M. Herpes zoster in HIV-1 infection: the role of CSF pleocytosis in secondary CSF escape and discordance. PLoS ONE. (2020) 15:e0236162. doi: 10.1371/journal.pone.0236162
80. Marra CM, Maxwell CL, Collier AC, Robertson KR, Imrie A. Interpreting cerebrospinal fluid pleocytosis in HIV in the era of potent antiretroviral therapy. BMC Infect Dis. (2007) 7:e37. doi: 10.1186/1471-2334-7-37
81. Alakkas A, Ellis RJ, Watson CW, Umlauf A, Heaton RK, Letendre S, et al. White matter damage, neuroinflammation, and neuronal integrity in HAND. J Neurovirol. (2019) 25:32–41. doi: 10.1007/s13365-018-0682-9
82. Aylward EH, Henderer JD, McArthur JC, Brettschneider PD, Harris GJ, Barta PE, et al. Reduced basal ganglia volume in HIV-1-associated dementia: results from quantitative neuroimaging. Neurology. (1993) 43:2099–104. doi: 10.1212/WNL.43.10.2099
83. Berger JR, Nath A, Greenberg RN, Andersen AH, Greene RA, Bognar A, et al. Cerebrovascular changes in the basal ganglia with HIV dementia. Neurology. (2000) 54:921–6. doi: 10.1212/WNL.54.4.921
84. Joseph SB, Arrildt KT, Swanstrom AE, Schnell G, Lee B, Hoxie JA, et al. Quantification of entry phenotypes of macrophage-tropic HIV-1 across a wide range of CD4 densities. J Virol. (2014) 88:1858–69. doi: 10.1128/JVI.02477-13
85. Churchill M, Nath A. Where does HIV hide? A focus on the central nervous system. Curr Opin HIV AIDS. (2013) 8:165–9. doi: 10.1097/COH.0b013e32835fc601
86. Li GH, Maric D, Major EO, Nath A. Productive HIV infection in astrocytes can be established via a nonclassical mechanism. AIDS. (2020) 34:963–78. doi: 10.1097/QAD.0000000000002512
87. Price RW, Brew B, Sidtis J, Rosenblum M, Scheck AC, Cleary P. The brain in AIDS: central nervous system HIV-1 infection and AIDS dementia complex. Science. (1988) 239:586–92. doi: 10.1126/science.3277272
88. Anesten B, Yilmaz A, Hagberg L, Zetterberg H, Nilsson S, Brew BJ, et al. Blood-brain barrier integrity, intrathecal immunoactivation, and neuronal injury in HIV. Neurol Neuroimmunol Neuroinflamm. (2016) 3:e300. doi: 10.1212/NXI.0000000000000300
89. Caligaris G, Trunfio M, Ghisetti V, Cusato J, Nigra M, Atzori C, et al. Blood-brain barrier impairment in patients living with HIV: predictors and associated biomarkers. Diagnostics. (2021) 11:867. doi: 10.3390/diagnostics11050867
90. Mellgren A, Antinori A, Cinque P, Price RW, Eggers C, Hagberg L, et al. Cerebrospinal fluid HIV-1 infection usually responds well to antiretroviral treatment. Antivir Ther. (2005) 10:701–7. doi: 10.1177/135965350501000607
91. Burbelo PD, Price RW, Hagberg L, Hatano H, Spudich S, Deeks SG, et al. Anti-human immunodeficiency virus antibodies in the cerebrospinal fluid: evidence of early treatment impact on central nervous system reservoir? J Infect Dis. (2018) 217:1024–1032. doi: 10.1093/infdis/jix662
92. Manji H, Jager HR, Winston A. HIV, dementia and antiretroviral drugs: 30 years of an epidemic. J Neurol Neurosurg Psychiatry. (2013) 84:1126–37. doi: 10.1136/jnnp-2012-304022
93. Handoko R, Spudich S. Treatment of central nervous system manifestations of HIV in the current Era. Semin Neurol. (2019) 39:391–8. doi: 10.1055/s-0039-1688915
94. Canestri A, Lescure FX, Jaureguiberry S, Moulignier A, Amiel C, Marcelin AG, et al. Discordance between cerebral spinal fluid and plasma HIV replication in patients with neurological symptoms who are receiving suppressive antiretroviral therapy. Clin Infect Dis. (2010) 50:773–8. doi: 10.1086/650538
95. Ferretti F, Gisslen M, Cinque P, Price RW. Cerebrospinal fluid HIV escape from antiretroviral therapy. Curr HIV/AIDS Rep. (2015) 12:280–8. doi: 10.1007/s11904-015-0267-7
96. Peluso MJ, Ferretti F, Peterson J, Lee E, Fuchs D, Boschini A, et al. Cerebrospinal fluid HIV escape associated with progressive neurologic dysfunction in patients on antiretroviral therapy with well controlled plasma viral load. AIDS. (2012) 26:1765–74. doi: 10.1097/QAD.0b013e328355e6b2
97. Trunfio M, Pinnetti C, Foca E, Bai F, Maffongelli G, Celani L, et al. Cerebrospinal fluid HIV-1 escape according to different thresholds and underlying comorbidities: is it time to assess the definitions? AIDS. (2019) 33:759–62. doi: 10.1097/QAD.0000000000002091
98. Winston A, Antinori A, Cinque P, Fox HS, Gisslen M, Henrich TJ, et al. Defining cerebrospinal fluid HIV RNA escape: editorial review AIDS. AIDS. (2019) 33(Suppl. 2):S107–11. doi: 10.1097/QAD.0000000000002252
99. Ferretti F, Gianotti N, Lazzarin A, Cinque P. Central nervous system HIV infection in “less-drug regimen” antiretroviral therapy simplification strategies. Semin Neurol. (2014) 34:78–88. doi: 10.1055/s-0034-1372345
100. Mastrangelo A, Turrini F, de Zan V, Caccia R, Gerevini S, Cinque P. Symptomatic cerebrospinal fluid escape. AIDS. (2019) 33(Suppl. 2):S159–69. doi: 10.1097/QAD.0000000000002266
101. Gray F, Lescure FX, Adle-Biassette H, Polivka M, Gallien S, Pialoux G, et al. Encephalitis with infiltration by CD8+ lymphocytes in HIV patients receiving combination antiretroviral treatment. Brain Pathol. (2013) 23:525–33. doi: 10.1111/bpa.12038
102. Lescure FX, Moulignier A, Savatovsky J, Amiel C, Carcelain G, Molina JM, et al. CD8 encephalitis in HIV-infected patients receiving cART: a treatable entity. Clin Infect Dis. (2013) 57:101–8. doi: 10.1093/cid/cit175
103. Lucas SB, Wong KT, Nightingale S, Miller RF. HIV-Associated CD8 encephalitis: a UK case series and review of histopathologically confirmed cases. Front Neurol. (2021) 12:e628296. doi: 10.3389/fneur.2021.628296
104. Eden A, Fuchs D, Hagberg L, Nilsson S, Spudich S, Svennerholm B, et al. HIV-1 viral escape in cerebrospinal fluid of subjects on suppressive antiretroviral treatment. J Infect Dis. (2010) 202:1819–25. doi: 10.1086/657342
105. Eden A, Nilsson S, Hagberg L, Fuchs D, Zetterberg H, Svennerholm B, et al. Asymptomatic cerebrospinal fluid HIV-1 viral blips and viral escape during antiretroviral therapy: a longitudinal study. J Infect Dis. (2016) 214:1822–1825. doi: 10.1093/infdis/jiw454
106. Price RW, Deeks SG. Antiretroviral drug treatment interruption in human immunodeficiency virus-infected adults: Clinical and pathogenetic implications for the central nervous system. J Neurovirol. (2004) 10(Suppl. 1):44–51. doi: 10.1080/753312752
Keywords: Africa, HIV, inflammation, central nervous system (CNS), cerebrospinal fluid (CSF), neuroimmunology, antiretroviral therapy (ART)
Citation: Meyer AC, Njamnshi AK, Gisslen M and Price RW (2022) Neuroimmunology of CNS HIV Infection: A Narrative Review. Front. Neurol. 13:843801. doi: 10.3389/fneur.2022.843801
Received: 27 December 2021; Accepted: 10 May 2022;
Published: 14 June 2022.
Edited by:
Richard Idro, Makerere University, UgandaReviewed by:
Victor Musiime, Makerere University, UgandaDilraj Singh Sokhi, Sheffield Teaching Hospitals NHS Foundation Trust, United Kingdom
Copyright © 2022 Meyer, Njamnshi, Gisslen and Price. This is an open-access article distributed under the terms of the Creative Commons Attribution License (CC BY). The use, distribution or reproduction in other forums is permitted, provided the original author(s) and the copyright owner(s) are credited and that the original publication in this journal is cited, in accordance with accepted academic practice. No use, distribution or reproduction is permitted which does not comply with these terms.
*Correspondence: Richard W. Price, cmljaGFyZC5wcmljZSYjeDAwMDQwO3Vjc2YuZWR1