Erratum: Protocol for a cross-sectional study: effects of a multiple sclerosis relapse therapy with methylprednisolone on offspring neurocognitive development and behavior (MS-children)
- 1Department of Neurology, Jena University Hospital, Jena, Germany
- 2Department of Neurology, St. Josef Hospital, Ruhr University Bochum, Bochum, Germany
Introduction: Multiple Sclerosis (MS) is the most common neuroimmunological disease in women of childbearing age. Current MS therapy consists of immunomodulatory relapse prevention with disease-modifying therapies (DMTs) and acute relapse therapy with the synthetic glucocorticoid (GC) methylprednisolone (MP). As most DMTs are not approved for use during pregnancy, treatment is usually discontinued, increasing the risk for relapses. While MP therapy during pregnancy is considered relatively save for the fetus, it may be detrimental for later cognitive and neuropsychiatric function. The underlying mechanism is thought to be an epigenetically mediated desensitization of GC receptors, the subsequent increase in stress sensitivity, and a GC-mediated impairment of brain development. The aim of this study is to investigate the associations of fetal MP exposure in the context of MS relapse therapy with later cognitive function, brain development, stress sensitivity, and behavior.
Methods and Analysis: Eighty children aged 8–18 years of mothers with MS will be recruited. Forty children, exposed to GC in utero will be compared to 40 children without fetal GC exposure. The intelligence quotient will serve as primary outcome. Secondary outcomes will include attention, motor development, emotional excitability, Attention-Deficit Hyperactivity Disorder-related symptoms, and behavioral difficulties. The Trier Social Stress Test will test stress sensitivity, EEG and MRI will assess functional and structural brain development. To determine underlying mechanisms, DNA methylation of the GC receptor gene and the H19/IGF2 locus and changes in the microbiome and the metabolome will be investigated. Primary and secondary outcomes will be analyzed using linear regression models. Time-variant outcomes of the stress test will be analyzed in two mixed linear models exploring overall activity and change from baseline.
Ethics and Dissemination: This study was approved by the participating institutions' ethics committees and results will be presented in accordance with the STROBE 2007 Statement.
Trial Registration: https://clinicaltrials.gov/ct2/show/NCT04832269?id=ZKSJ0130
Introduction
Background
Multiple sclerosis (MS) is one of the most common neurological diseases in young adults, usually occurring between the ages of 20 and 40 years, affecting an estimated 2.8 million patients worldwide (1). Approximately two thirds of these patients are women, mainly of childbearing age (2). Therapy for MS consists of disease-modifying therapy (DMT) for relapse prophylaxis with immunomodulatory substances and relapse therapy with methylprednisolone (MP). As most immunomodulators are not approved for use during pregnancy, treatment is usually discontinued with a subsequent risk increase for relapses (3). While the risk for relapses especially during the first and second trimester is comparable to the prepartum period and thus not an uncommon event, the risk decreases by ~70% during the third trimester (4). Based on these data and the prevalence rate for MS in women of childbearing age (1), we estimate that in Germany ~5,500 MS-relapses occur annually during a pregnancy.
European and US guidelines recommend the use of high-dose MP to treat severe, disabling relapses anytime during pregnancy with a risk-benefit-evaluation between gestational weeks 8 and 11 (5). This treatment is considered relatively harmless for the fetus since no severe physical development disorders have been found at birth (3, 6). However, studies with offspring antenatally exposed to the synthetic GC betamethasone (BM) to enhance fetal lung maturation due to a threat of premature labor suggest a risk for increased stress sensitivity and neuropsychiatric disorders in later life (7, 8). These effects of antenatal GC-exposure on offspring outcomes have been found at all periods of pregnancy (9). Remarkably, the treatment regime used in obstetric indications consists of only 2 × 8–12 mg BM 24 h apart as compared to the 500–1,000 mg MP over 3 – 5 days used to treat an MS relapse. Considering the ~six-fold higher biological activity of BM compared with MP (10), the total dose of GC in the treatment of MS relapses is up to 50-fold higher than for obstetric indications. In a previous study from our workgroup, which is the basis for the present study, we could show that antenatal exposure to 2 × 8 mg BM to enhance fetal lung maturation resulted in multidimensional changes in neurodevelopment and stress-sensitivity in full-term offspring at 8–9 years of age compared to children not exposed to BM (11, 12). This included an intelligence quotient (IQ), which was on average 10 points lower in the exposed group than in the non-exposed group, changes in autonomic activity, attention-deficit hyperactivity disorder (ADHD)-like symptoms and changes in electrocortical activation during a cognitive task. In a follow-up study with 16-year-olds antenatally exposed to BM, we found changes in measures of structural brain development, specifically alterations in gyrification and a reduced cortical thickness in MRI scans (publication in preparation). Combined, these findings have led to a change in German obstetric guidelines already, which now call for a more critical indication for the induction of lung maturation (13, 14).
The adverse long-term effects of antenatal GC exposure may be the result of both a programming of the function of the hypothalamic-pituitary-adrenal axis (HPAA) and maturational effects of GCs on functional and structural brain development. The relationship between adverse environmental influences such as an intrauterine exposure to supraphysiological levels of GCs during critical periods of development and the health of the offspring in later life is the basis of the “Fetal Programming” or “Developmental Origins of Health and Disease (DOHaD)” hypothesis (15, 16). Until the 3rd trimester, the fetus is incapable of synthesizing cortisol and relatively protected from maternal cortisol because the placental enzyme 11ß-HSD 2 inactivates 80–90 % of maternal cortisol to cortisone (17). In contrast to cortisol, most synthetic GCs with the exception of MP are no substrates for 11ß-HSD2 and cross the placenta without inactivation (18). However, even though MP is a substrate for 11ß-HSD 2, high bolus doses of MP as used in MS-relapse therapy rapidly saturate this enzyme and reach the fetus in pharmacological concentrations (6, 19). Once in the fetal circulation, even short-term elevated levels of GCs can permanently change patterns of gene expression and cellular function via epigenetic mechanisms (20). Permanent desensitization of GC receptors involved in negative feedback regulation of the HPAA via DNA methylation of the promoter region of the GC receptor gene NR3C1 may lead to a life-long hyperactivity of the HPAA and, thus, to increased stress sensitivity in later life (21, 22). Hyperactivity of the HPAA is associated with stress-related neuropsychiatric disorders, including anxiety, depression, and ADHD (21, 22).
Increased levels of GCs induce accelerated tissue differentiation and maturation at the expense of tissue growth (23). While this effect is utilized to induce lung maturation in fetuses at risk of premature birth (24), it occurs in all organ systems and is reflected in a reduced birth weight following antenatal GC treatment (25). Out of all organ systems, the developing brain is particularly vulnerable to elevated GC concentrations due to its complex sequence of precisely coordinated steps of maturation and its intrinsic plasticity (26), a basic property of the brain enabling learning and adaptation. Supraphysiological concentrations of GCs change fetal brain development via antiproliferative effects on neural stem and progenitor cells (27). The subsequent changes in neuronal connections and myelination (28, 29) may contribute to the sustained changes in cognitive and cerebral functioning. Increased concentrations of GCs may also affect fetal brain development indirectly via changes in the composition of the maternal gut microbiota (30, 31), which in turn modulates the fetal gut microbiota (32, 33). The gut microbiome communicates with the central nervous system via an integrated, bidirectional communication pathway which i.a., involves inflammatory cytokines, neuromodulators and neurotransmitters (17, 34, 35). Consequently, disturbances in this communication system, the so-called “gut-brain” axis, have been associated with a number of neurological and psychiatric diseases, such as depression, anxiety disorders, and attention deficit hyperactivity disorder (17, 32, 36, 37).
While no studies examining the long-term effects on the offspring exist, current MS guidelines consider relapse therapy using MP during pregnancy safe for the fetus. Based on findings regarding long-term consequences of antenatal BM exposure, we hypothesize however, that this may not be the case. Based on the up to 50-fold cumulative dose of GCs in MS relapse treatment and its more prolonged administration compared to obstetric indications, we aim to investigate whether relapse treatment with MP in MS patients during pregnancy affects structural and functional brain development, cognition and behavior as well as stress sensitivity in the antenatally exposed offspring.
Research Aims and Hypotheses
This study is based on two hypotheses: Fetal exposure to MP during MS relapse therapy leads to epigenetically mediated
a. disturbances in functional and structural brain development and
b. a long-term change in stress sensitivity of the offspring.
The resulting changes in brain function are associated with neuropsychiatric abnormalities as well as cognitive and behavioral changes in later life.
The primary objective of this cross-sectional study is to elucidate associations of antenatal MP exposure with cognitive performance in children aged 8–18 years compared to non-exposed children of mothers with MS. As secondary objectives, this study will investigate the association of cognitive development and behavioral problems with underlying changes in stress sensitivity and functional and structural brain development. To clarify potential epigenetic mechanisms underpinning these changes in stress sensitivity and functional and structural brain development, DNA methylation at the promotor region of the GC receptor gene NR3C1 and at the H19/IGF2 locus, which controls expression of the insulin-like growth factor 2 (IGF2), the major growth hormone during development (38), will be investigated. To elucidate further potential mechanisms, changes in the composition of the microbiome will be examined.
This study is expected to advance the understanding of developmental programming effects of antenatal high-dosage GC exposure. Another major aim of this study is to add data to the debate around evidence-based decision-making regarding (1) the continuation of a DMT during pregnancy vs. the potential long-term risks of relapse therapy with MP for the child's cognitive and neuropsychiatric health in later life, and (2) the risks of relapse treatment with MP for the child vs. the risks of an untreated relapse for the mother. There is an accumulating amount of real world data on the safety of continuing a DMT during pregnancy primarily with interferons or glatiramer acetate, which may be safer for mother and child than a potentially necessary relapse treatment with MP.
Methods and Analysis
Study Design
This two-center, observational, cross-sectional study aims to recruit N = 80 children of mothers with MS. The exposed group (n = 40) will consist of children who were antenatally exposed to MP as part of maternal MS relapse therapy, while the control group (n = 40), will consist of children of mothers with MS, who were not exposed to MP. Assessment will take place at the University Hospital in Jena (UKJ) and the Ruhr University Bochum (RUB). The first participant was included on 19/10/2020 and the study is anticipated to continue until the end of 2024.
Sample Size
The aforementioned study by some of the authors (11) investigated cognitive performance, as measured by an IQ test, in two groups of 39 healthy children at the age of 8 years. Considering the lower biological activity of MP compared to BM, the cohort in the present study was exposed to a GC-dose up to 50-fold higher than in the study on the effects of BM. The higher dosage of MP will most likely expose a significant effect in two groups of the same size as the previous study.
As the present study uses a different IQ test, the planned sample size is further based on the standardized effect size Cohen's d, as a multiple of the standard deviation. The previous study found a mean difference in IQ score of 10.5 points (SD = 14.0). A two-sample t-test (α = 0.05) would have sufficient power of 82% (d = 0.71) to 87% (d = 0.75) with two groups of 35 children. This takes into account potential non-evaluability of five participants per group, e.g., in the case of artifacts or attrition. The planned regression models include seven variables, which, based on the rule of ten leads to a sufficient number of cases to produce robust results (39).
Sample Characteristics and Recruitment
Children aged 8–18 years, of any sex and ethnicity are eligible to participate. Based on the children we identified for the group exposed to GC, control children are matched 1:1 by age and sex. A physician presents verbal and written information about the study, its aims, the procedure, and data management to the participants and their guardian(s) and answers any remaining questions. Children are included if they speak German and they and their guardian(s) are willing and able to consent to their participation.
Exclusion criteria for both groups comprise preterm birth (before 34th week of gestation), a birth weight below the 5th percentile, and perinatal complications, such as cerebral hemorrhage, neonatal intensive care requiring ventilation, or any further antenatal therapy with GCs beyond MS relapse therapy. Participants are further excluded from the study in case of maternal substance abuse during pregnancy or serious illness in the child which makes assessment impossible, such as an intellectual disability, or long-term medication with GCs, e.g., in asthma.
Recruitment takes place in the UKJ's close cooperation with the RUB, the host of the German MS and Pregnancy Registry, and several other MS centers across Germany. A search algorithm was developed to identify eligible participants for the MP-exposed and the control group within the respective hospital-specific data management systems. Further recruitment efforts are directed toward online advertisements on MS-specific websites and UKJ's public outreach channels.
Outcome Measures
Basic sociodemographic and medical data related to the pregnancy, birth and the mother's MS are collected from all participants (see Table 1). Combined with a questionnaire on stressful life events during pregnancy, this serves as a proxy for the mother's stress level during pregnancy. The child's current overall stress level is estimated using a questionnaire on stressful life events in the past 12 months (see Table 1). Time between GC exposure and assessment will be calculated using the child's age and the pregnancy week at the time of treatment. The primary outcome measure of this study is the child's global cognitive ability, as measured by a standardized IQ-test, i.e., the Reynolds Intellectual Assessment Scales and Screening (RIAS) (40). Secondary behavioral outcomes, such as motor development, attention, emotional excitability, ADHD-associated symptoms, and behavioral difficulties in multiple categories are measured via neuropsychological assessment, and self-, and informant-reported questionnaires. Additional secondary outcomes are obtained during the Trier Social Stress Test for children (TSST-C), an established standardized protocol to test stress sensitivity (41). These outcome measures comprise of the neuroendocrine (salivary cortisol) and autonomic stress response (salivary alpha-amylase concentration as a measure of sympathetic activity (42)) and heart rate variability (HRV) indices. To capture subjective stress levels during the TSST-C, the participant fills out an anxiety questionnaire (43) before and after the test. As measures of neurodevelopment, activation in the EEG during rest and a cognitive task included in the TSST-C and MRI-based markers of structural brain development are used. With the use of peripheral blood (leukocytes), and buccal mucosa cells, potential epigenetic mechanisms underlying changes in cognition and behavior as well as in stress sensitivity will be determined (see Table 2). For exploratory mechanistic analysis, the composition of the microbiome (stool sample), metabolome (EDTA plasma) and elementome (hair sample) will be examined.
Procedure
Based on logistical considerations, eligible participants are invited to two study days (see Figure 1). On one of the days, neuropsychological testing (NPT) and the TSST-C take place (NPT day), while an MRI scan is carried out on the other (MRI day). For scheduling reasons, a few days may lie in between visits.
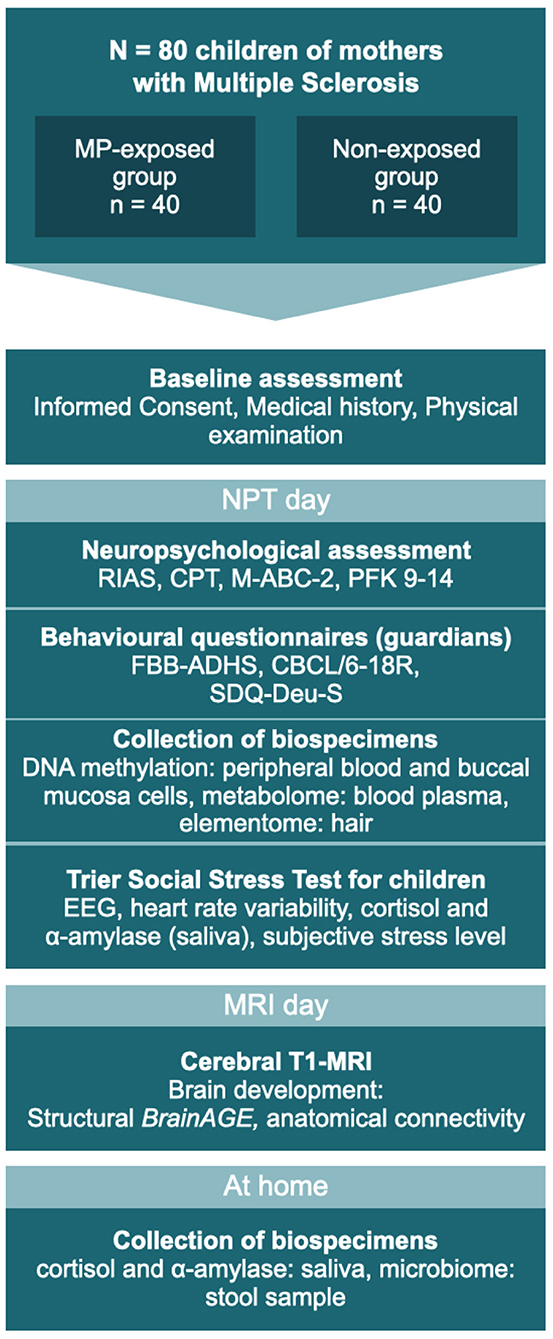
Figure 1. The two study days: testing sequence for all participants. MP, methylprednisolone; NPT, Neuropsychological testing; RIAS, Reynolds Intellectual Assessment Scales and Screening; CPT, Continuous Performance Test; M-ABC-2, Movement Assessment Battery for Children – Second Edition; PFK 9-14, German personality questionnaire; FBB-ADHS, German questionnaire for attention deficit hyperactivity disorder; CBCL/6-18, Child Behavior Checklist for children aged 6–18 years; SDQ-Deu-S, Strengths and Difficulties Questionnaire. MRI and NPT days may occur in reverse order.
On the NPT day, basic clinical and demographic data are recorded and the accompanying guardian is asked to assess their child's behavior using several well-established questionnaires (see Tables 1, 2). The participating child takes part in a set of four neuropsychological assessments of cognition and behavior (see Table 2), performed by a psychologist blinded to the participant's status of antenatal MP exposition. Before the participant is released for a 60-min break, hair, saliva, and buccal specimens are collected. The entire procedure takes ~120–180 min.
Following the collection of blood (see Table 2), stress sensitivity is examined using the TSST-C, an instrument to generate a psychophysiological stress response in the standardized setting of a simulated examination. See Figure 2 for a schematic representation of the procedure. The participant is equipped with EEG and ECG electrodes, a blood pressure monitor, and a pulse oximeter for continuous measurements during the TSST-C. Saliva samples are collected to measure cortisol and alpha-amylase concentrations throughout the test (see Figure 2 and Table 2 for scheduling of sampling). The TSST-C begins with an “active rest” phase of 15 min, during which the participant watches a slow-paced animal documentary, sitting down. In a separate room, a two-person examination committee then present the participant with a story, before leaving the room for 5 min, giving the participant time to prepare an “exciting and compelling” ending to the story. The participant is then asked to continue telling the story (stress phase I), after which the participant is instructed to verbalize serial subtractions of age-appropriate difficulty (stress phase II). When the participant makes a mistake, the examiner interrupts them with a prompt to start over. The stress phases last a combined 10 min, after which the participant receives positive feedback from the committee. In total, the TSST-C takes ~130 min. After the TSST-C, the participants are handed a kit for the at-home collection of a further 3 saliva samples, to capture the HPAA activity throughout a normal day. The kit also includes material to collect a stool sample for the analysis of the microbiome.
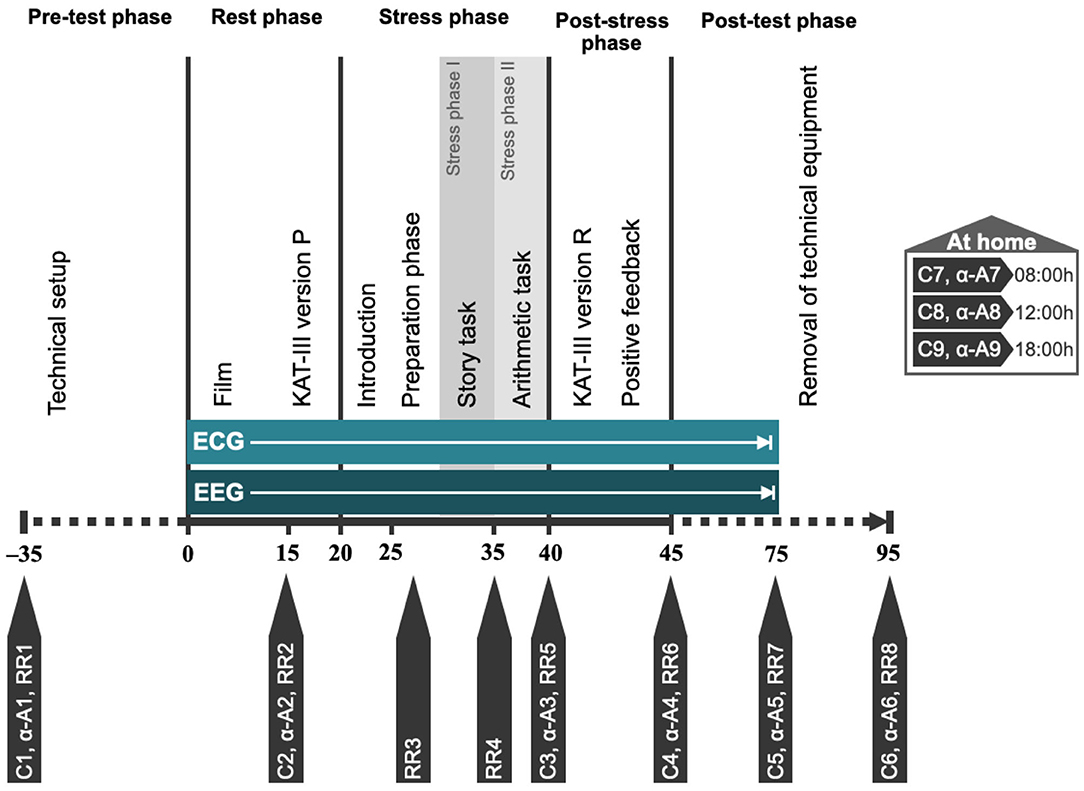
Figure 2. Schematic representation of TSST-C procedure. C1–C9, saliva swabs 1–9 (cortisol); α-A1–α-A9, saliva swabs 1–9 (alpha-amylase); RR1–RR8, blood pressure measurements 1–8; KAT-III, German anxiety scale for children (Kinder-Angst-Test-III) – versions P (prospective) and R (retrospective).
On the MRI day, a volumetric cranial MRI scan in T1 standard sequence is obtained from the participant. Diffusion tensor imaging (DTI) is used to determine the anatomical connectome. Including participant education, the entire procedure is estimated to take 50 min.
Data Storage and Management
All data are recorded in a pseudonymised form. In accordance with EU data protection legislation, both study centers maintain a secured patient identification list with full names, addresses, and dates of birth. Any personal information is archived for at least 10 years after inclusion of the last participant.
The collection, management and use of human biological specimens in this study is in accordance with national and EU legislation. Any transfer of specimens between the two centers will take place in accordance with the Oviedo Convention (Convention on Human Rights and Biomedicine – CETS 164) and Recommendation of the Committee of Ministers to Member States on research on biological materials of human origin (adopted by the Committee of Ministers on 15 March 2006 at the 958th Meeting of Ministerial Deputies). Due to the vulnerable nature of a cohort of children, we further implement directive 95/46/EC and working group 8/2010 opinion, article 29 and take the high importance of data protection in vulnerable populations into careful consideration.
Part of the initial data collection uses hard copy files, which are stored in a secure location and later digitized using an electronic case report form. All digitized data are stored on a safe server managed by the Center for Clinical Studies at the University hospital in Jena.
Data Analysis Plan
For the analysis of the primary outcome, exposed and non-exposed groups will be compared in terms of their performance on the IQ test using a linear regression model taking into account potential confounders, including antenatal exposure to MP, point in time of exposure, maternal age at birth, birth weight, and socioeconomic background. Exploratory analysis of secondary outcomes will be performed with a linear regression model. Those outcomes measured repeatedly throughout the course of the TSST-C will be evaluated as time series data with a linear mixed model, with time as a further independent factor. For this analysis, two models will be fitted to explore (1) overall mean activity throughout the TSST-C, as well as (2) the change from baseline, in which the stress response will be adjusted to the baseline value.
Methodological Challenges
Recruitment of eligible participants presents the main challenge for this study. However, based on estimations of the prevalence of MS in Germany as well as pregnancy and relapse rates, our sample size represents only ~0.8% of the total target population in Germany. The close cooperation with the German MS and Pregnancy Registry, founded by members of the study group, gives us access to a database of more than 3,000 pregnancies of women with MS. Several MS clinics and surgeries across Germany, as well as the German Society for MS (DMSG) support recruitment efforts by sharing flyers and online adverts with their patients or members, and searching their databases for potential participants. The ongoing COVID-19 pandemic presents another challenge which slowed down recruitment and testing, particularly in the first quarter of 2021. In accordance with the rules and recommendations of the German government, we have implemented a strict hygiene plan for participants and researchers to continue with recruitment.
The TSST-C is vulnerable to subjective factors in the examination committee and environmental factors. The procedure is guided by detailed protocols and pilot runs, to ensure standardization within and between study centers. The same investigator performs neuropsychological testing in both study centers, granting further consistency of conditions.
To account for the vulnerability of the study population and potential anxiety around measures such as MRI or blood sampling, we devised detailed, child-friendly participant education material. The children's guardians are usually present and the child is offered a topical local anesthetic in preparation for the drawing of blood.
In this study, antenatal or postnatal stressors may act as confounders. Maternal psychosocial stress during pregnancy has been shown to elicit increased maternal and fetal cortisol levels (54, 55). It is further associated with an increase in the offspring stress response, disturbed motor and structural brain development and an increased risk of cognitive, behavioral, and emotional problems in later life, such as autism spectrum disorders, ADHD, depression and schizophrenia (for reviews see (9, 55)). MS relapses are not only treated with GCs but also are a major psychological stressor. However, as relapses during pregnancy rarely go untreated, there is no realistic way of mitigating this confounder using an untreated MS control group. Due to the retrospective nature of this study, there is no reliable method to assess perceived psychological stress level beyond major stressful life events, at a minimum of 8 years in the past. The mother's stress level during pregnancy is thus estimated using a questionnaire based on the Stress and Adversity Inventory (STRAIN) (56), which was adapted to this study's context. Additionally, the children fill out a questionnaire regarding subjective and objective stress in the past 12 months (see Table 1).
Ethics and Dissemination
This study is conducted with the approval of the ethics committees of Friedrich-Schiller University Jena (reference: 2020-1668-3-BO) and the medical faculty of Ruhr University Bochum (reference: 21-7192 BR). The results are going to be published in relevant peer-reviewed journals, presented in accordance with the STROBE 2007 Statement (57).
Author Contributions
VK: writing of the manuscript, study implementation, and data collection. MS: study conception and design and critical manuscript revision. ST and KH: critical manuscript revision and study implementation. FR: study conception, design, study implementation, and critical manuscript revision. MD: study conception, design, critical manuscript revision, study implementation, and data collection.
Funding
This study is supported by the Grant for Multiple Sclerosis Innovation 2020 (MerckHealthcare KGaA). The funder was not involved in the study design, collection, analysis, interpretation of data, the writing of this article or the decision to submit it for publication.
Conflict of Interest
The authors declare that the research was conducted in the absence of any commercial or financial relationships that could be construed as a potential conflict of interest.
Publisher's Note
All claims expressed in this article are solely those of the authors and do not necessarily represent those of their affiliated organizations, or those of the publisher, the editors and the reviewers. Any product that may be evaluated in this article, or claim that may be made by its manufacturer, is not guaranteed or endorsed by the publisher.
Acknowledgments
We would like to thank Dr. phil. Carolin Ligges for her expertise and council regarding psychological testing and research with children.
References
1. Browne PD, Chandraratna C, Angood H, Tremlett C, Baker Taylor BV, et al. Atlas of Multiple Sclerosis 2013: A growing global problem with widespread inequity. Neurology. (2014) 83:1022–4. doi: 10.1212/WNL.0000000000000768
2. Kingwell E, Marriott JJ, Jette N, Pringsheim T, Makhani N, Morrow SA, et al. Incidence and prevalence of multiple sclerosis in Europe: a systematic review. BMC Neurol. (2013) 13:1–13. doi: 10.1186/1471-2377-13-128
3. Thöne J, Thiel S, Gold R, Hellwig K. Treatment of multiple sclerosis during pregnancy – safety considerations. Expert Opin Drug Saf. (2017) 16:523–34. doi: 10.1080/14740338.2017.1311321
4. Confavreux C, Hutchinson M, Hours MM, Cortinovis-Tourniaire PT. Moreau, and PiMS Group Rate of pregnancy-related relapse in multiple sclerosis. N Engl J Med. (1998) 339:285–91. doi: 10.1056/NEJM199807303390501
5. Dobson R, Dassan P, Roberts M, Giovannoni G, Nelson-Piercy C, Brex PA, et al. consensus on pregnancy in multiple sclerosis:‘Association of British Neurologists' guidelines. Pract Neurol. (2019) 19:106–14. doi: 10.1136/practneurol-2018-002060
6. Lockshin MD, Sammaritano LR. Corticosteroids during pregnancy. Scand J Rheumatol. (1998) 27:136–8. doi: 10.1080/03009742.1998.11720789
7. Alexander N, Rosenlöcher F, Stalder T, Linke J, Distler W, Morgner J, et al. Impact of antenatal synthetic glucocorticoid exposure on endocrine stress reactivity in term-born children. J Clin Endocrinol Metabol. (2012) 97:3538–44. doi: 10.1210/jc.2012-1970
8. Ilg L, Kirschbaum C, Li SC, Rosenlöcher F, Miller R, Alexander N. Persistent Effects of Antenatal Synthetic Glucocorticoids on Endocrine Stress Reactivity From Childhood to Adolescence. J Clin Endocrinol Metabol. (2019) 104:827–34. doi: 10.1210/jc.2018-01566
9. Van den Bergh BR., van den Heuvel MI, Lahti M, Braeken M, de Rooij SR, Entringer S, et al. Prenatal developmental origins of behavior and mental health: the influence of maternal stress in pregnancy. Neurosci Biobehav Rev. (2020) 117:26–64. doi: 10.1016/j.neubiorev.2017.07.003
10. Liu D, Ahmet A, Ward L, Krishnamoorthy P, Mandelcorn ED, Leigh R, et al. A practical guide to the monitoring and management of the complications of systemic corticosteroid therapy. Allergy Asthma Clin Immunol. (2013) 9:30. doi: 10.1186/1710-1492-9-30
11. Rakers F, Schleußner E, Muth I, Hoyer D, Rupprecht S, Schiecke K, et al. Impact of antenatal glucocorticoid exposure on activity of the stress system, cognition and behavior in 8–9-year-old children: a clinical cohort study. (in preparation).
12. Schwab M, Heinemann S, Schwab K, Thoms I, Rupprecht S, Hoyer H, et al. Effects of Prenatal Betamathasone (BM) Exposure on Cortical Activity at the Age of Eight Years-A Pilot Study. Reprod Sci, (2010) 17: 292A−293A.
13. Berger R, Abele H, Bahlmann F, Bedei I, Doubek K, Felderhoff-Müser U, et al. Prävention und Therapie der Frühgeburt. Leitlinie der DGGG, OEGGG und SGGG (S2k-Niveau, AWMF-Registernummer 015/025, Februar 2019)–Teil 1 mit Empfehlungen zur Epidemiologie, Ätiologie, Prädiktion, primären und sekundären Prävention der Frühgeburt. Zeitschrift für Geburtshilfe und Neonatologie. (2019) 223: 304–316. doi: 10.1055/a-0979-1028
14. Berger R, Abele H, Bahlmann F, Bedei I, Doubek K, Felderhoff-Müser U, et al. Prävention und Therapie der Frühgeburt. Leitlinie der DGGG, OEGGG und SGGG (S2k-Niveau, AWMF-Registernummer 015/025, Februar 2019)–Teil 2 mit Empfehlungen zur tertiären Prävention der Frühgeburt und zum Management des frühen vorzeitigen Blasensprungs. Zeitschrift für Geburtshilfe und Neonatologie. (2019) 223:373–94. doi: 10.1055/a-1008-8730
15. Barker DJ. The fetal and infant origins of adult disease. BMJ. (1990) 301:1111. doi: 10.1136/bmj.301.6761.1111
16. Gillman MW. Developmental origins of health and disease. N Engl J Med. (2005) 353:1848. doi: 10.1056/NEJMe058187
17. Rakers FS, Rupprecht M, Dreiling C, Bergmeier OWitte W, Schwab M. Transfer of maternal psychosocial stress to the fetus. Neurosci Biobehav Rev. (2020) 117:185–97. doi: 10.1016/j.neubiorev.2017.02.019
18. Benediktsson R, Calder AA, Edwards CR, Seckl JR. Placental 11β-hydroxysteroid dehydrogenase: a key regulator of fetal glucocorticoid exposure. Clin Endocrinol. (1997) 46:161–6. doi: 10.1046/j.1365-2265.1997.1230939.x
19. Anderson GG, Rotchell Y, Kaiser DG. Placental transfer of methylprednisolone following maternal intravenous administration. Am J Obstet Gynecol. (1981) 140:699–701. doi: 10.1016/0002-9378(81)90207-6
20. Cao-Lei L, De Rooij S, King S, Matthews S, Metz G, Roseboom T, et al. Prenatal stress and epigenetics. Neurosci Biobehav Rev. (2020) 117:198–210. doi: 10.1016/j.neubiorev.2017.05.016
21. Harris A, Seckl J. Glucocorticoids, prenatal stress and the programming of disease. Horm Behav. (2011) 59:279–89. doi: 10.1016/j.yhbeh.2010.06.007
22. Moisiadis VG, Matthews SG. Glucocorticoids and fetal programming part 1: Outcomes. Nat Rev Endocrinol. (2014) 10:391. doi: 10.1038/nrendo.2014.73
23. Liggins G. The role of cortisol in preparing the fetus for birth. Reprod Fertil Dev. (1994) 6:141–50. doi: 10.1071/RD9940141
24. Garbrecht MR, Klein JM, Schmidt TJ, Snyder JM. Glucocorticoid metabolism in the human fetal lung: implications for lung development and the pulmonary surfactant system. Biol Neonate. (2006) 89:109–19. doi: 10.1159/000088653
25. Bloom SL, Sheffield JS, McIntire DD, Leveno KJ. Antenatal dexamethasone and decreased birth weight. Obstet Gynecol. (2001) 97:485–90. doi: 10.1097/00006250-200104000-00001
26. Uno H, Eisele S, Sakai A, Shelton S, Baker E, DeJesus O, et al. Neurotoxicity of glucocorticoids in the primate brain. Horm Behav. (1994) 28:336–48. doi: 10.1006/hbeh.1994.1030
27. Carson R, Monaghan-Nichols AP, DeFranco DB, Rudine AC. Effects of antenatal glucocorticoids on the developing brain. Steroids. (2016) 114:25–32. doi: 10.1016/j.steroids.2016.05.012
28. Antonow-Schlorke I, Helgert A, Gey C, Coksaygan T, Schubert H, Nathanielsz PW, et al. Adverse effects of antenatal glucocorticoids on cerebral myelination in sheep. Obstet Gynecol. (2009) 113:142–51. doi: 10.1097/AOG.0b013e3181924d3b
29. Scheinost D, Sinha R, Cross SN, Kwon SH, Sze G, Constable RT, et al. Does prenatal stress alter the developing connectome? Pediatr Res. (2017) 81:214–26. doi: 10.1038/pr.2016.197
30. Huang EY, Inoue T, Leone VA, Dalal S, Touw K, Wang Y, et al. Using corticosteroids to reshape the gut microbiome: implications for inflammatory bowel diseases. Inflamm Bowel Dis. (2015) 21:963–72. doi: 10.1097/MIB.0000000000000332
31. Zhao H, Jiang X, Chu W. Shifts in the gut microbiota of mice in response to dexamethasone administration. Int Microbiol. (2020) 23:565–73. doi: 10.1007/s10123-020-00129-x
32. Jašarević E, Howard CD, Morrison K, Misic A, Weinkopff T, Scott P, et al. The maternal vaginal microbiome partially mediates the effects of prenatal stress on offspring gut and hypothalamus. Nat Neurosci. (2018) 21:1061–71. doi: 10.1038/s41593-018-0182-5
33. Vuong HE, Pronovost GN, Williams DW, Coley EJ, Siegler EL, Qiu A, et al. The maternal microbiome modulates fetal neurodevelopment in mice. Nature. (2020) 586:281–6. doi: 10.1038/s41586-020-2745-3
34. Cerritelli F, Frasch MG, Antonelli MC, Viglione C, Vecchi S, Chiera M, et al. A Review on the Vagus Nerve and Autonomic Nervous System During Fetal Development: Searching for Critical Windows. Front Neurosci. (2021) 15:1184. doi: 10.3389/fnins.2021.721605
35. Garzoni L, Faure C, Frasch MG. Fetal cholinergic anti-inflammatory pathway and necrotizing enterocolitis: the brain-gut connection begins in utero. Front Integr Neurosci. (2013) 7:57. doi: 10.3389/fnint.2013.00057
36. Glover V, O'Donnell KJ, O'Connor TG, Fisher J. Prenatal maternal stress, fetal programming, and mechanisms underlying later psychopathology—a global perspective. Dev Psychopathol. (2018) 30:843–54. doi: 10.1017/S095457941800038X
37. Vuong HE, Yano JM, Fung TC, Hsiao EY. The microbiome and host behavior. Annu Rev Neurosci. (2017) 40:21–49. doi: 10.1146/annurev-neuro-072116-031347
38. Baker J, Liu JP, Robertson EJ, Efstratiadis A. Role of insulin-like growth factors in embryonic and postnatal growth. Cell. (1993) 75:73–82. doi: 10.1016/S0092-8674(05)80085-6
39. Austin PC, Steyerberg EW. The number of subjects per variable required in linear regression analyses. J Clin Epidemiol. (2015) 68:627–36. doi: 10.1016/j.jclinepi.2014.12.014
40. Hagmann-Von A, Grob A. Reynolds Intellectual Assessment Scales and Screening. Deutschsprachige Adaptation der Reynolds Intellectual Assessment Scales (RIAS) & des Reynolds Intellectual Screening Test (RIST) von Cecil R. Reynolds und Randy W. Kamphaus. In: Huber H, Hogrefe AG, . Bern: Hogrefe (2014).
41. Kirschbaum C, Pirke KM, Hellhammer DH. The ‘Trier Social Stress Test'–a tool for investigating psychobiological stress responses in a laboratory setting. Neuropsychobiology. (1993) 28:76–81. doi: 10.1159/000119004
42. Nater UM, Rohleder N. Salivary alpha-amylase as a non-invasive biomarker for the sympathetic nervous system: current state of research. Psychoneuroendocrinology. (2009) 34:486–96. doi: 10.1016/j.psyneuen.2009.01.014
44. Steinhausen HC, Winkler Metzke C. Die Zürcher Lebensereignis-Liste (ZLEL): Ergebnisse einer Schweizer epidemiologischen Untersuchung. Kindheit und Entwicklung. (2001) 10:47–55. doi: 10.1026/0942-5403.10.1.47
45. Knye M, Roth N, Westhus W, Heine A. Continuous Performance Test: CPT. 1st ed. Göttingen: Hogrefe (2004).
46. Henderson SE, Sudgen DA, Barnett AL. Movement Assessment Battery for Children - 2 (M-ABC-2; German edition). 4th ed, In: F. Petermann. Frankfurt: Pearson Assessment (2015).
47. Seitz W, Rausche A. Persönlichkeitsfragebogen für Kinder zwischen 9 und 14 Jahren: PFK 9-14. 5th ed. Göttingen: Hogrefe (2004).
48. Döpfner M, Görtz-Dorten A. Diagnostik-System für psychische Störungen nach ICD-10 und DSM-5 für Kinder-und Jugendliche (DISYPS-III). 1st ed. Bern: Hogrefe (2017).
49. Döpfner M, Plück J, Kinnen C, Achenbach TM. Deutsche Schulalter-Formen der Child Behavior Checklist von Thomas M. Achenbach: Elternfragebogen über das Verhalten von Kindern und Jugendlichen (CBCL/6-18R). 1st ed. Göttingen: Hogrefe (2014).
50. Lohbeck A, Schultheiß J, Petermann F, Petermann U. Die Deutsche selbstbeurteilungsversion des strengths and difficulties questionnaire (SDQ-Deu-S). Diagnostica. (2015) 61:222–35. doi: 10.1026/0012-1924/a000153
51. Schmidt K, Schwab M, Eiselt M, Kott M, Hoyer D, Zwiener U. Nonlinear modeling of different fetal and neonatal behaviorial states. Pathophysiology. (1998) 1001:257. doi: 10.1016/S0928-4680(98)81300-X
52. Franke K, Gaser C. Ten years of BrainAGE as a neuroimaging biomarker of brain aging: what insights have we gained? Front Neurol. (2019) 10:789. doi: 10.3389/fneur.2019.00789
53. Ambeskovic M, Fuchs E, Beaumier P, Gerken M, Metz GA. Hair trace elementary profiles in aging rodents and primates: links to altered cell homeodynamics and disease. Biogerontology. (2013) 14:557–67. doi: 10.1007/s10522-013-9464-1
54. Rakers F, Bischoff S, Schiffner R, Haase M, Rupprecht S, Kiehntopf M, et al. Role of catecholamines in maternal-fetal stress transfer in sheep. Am J Obstet Gynecol. (2015) 213:684. e1–684. e9. doi: 10.1016/j.ajog.2015.07.020
55. Van den Bergh BR, Dahnke R, Mennes M. Prenatal stress and the developing brain: Risks for neurodevelopmental disorders. Dev Psychopathol. (2018) 30:743–62. doi: 10.1017/S0954579418000342
56. Slavich GM, Shields GS. Assessing lifetime stress exposure using the Stress and Adversity Inventory for Adults (Adult STRAIN): an overview and initial validation. Psychosom Med. (2018) 80:17. doi: 10.1097/PSY.0000000000000534
Keywords: Multiple sclerosis, glucocorticoids, methylprednisolone, cognition, pituitary-adrenal axis
Citation: Kozik V, Schwab M, Thiel S, Hellwig K, Rakers F and Dreiling M (2022) Protocol for a Cross-Sectional Study: Effects of a Multiple Sclerosis Relapse Therapy With Methylprednisolone on Offspring Neurocognitive Development and Behavior (MS-Children). Front. Neurol. 13:830057. doi: 10.3389/fneur.2022.830057
Received: 06 December 2021; Accepted: 17 March 2022;
Published: 26 April 2022.
Edited by:
Elisabeth Gulowsen Celius, Oslo University Hospital, NorwayReviewed by:
Martin Gerbert Frasch, University of Washington, United StatesAlice Mariottini, University of Florence, Italy
Copyright © 2022 Kozik, Schwab, Thiel, Hellwig, Rakers and Dreiling. This is an open-access article distributed under the terms of the Creative Commons Attribution License (CC BY). The use, distribution or reproduction in other forums is permitted, provided the original author(s) and the copyright owner(s) are credited and that the original publication in this journal is cited, in accordance with accepted academic practice. No use, distribution or reproduction is permitted which does not comply with these terms.
*Correspondence: Valeska Kozik, valeska.kozik@med.uni-jena.de
†These authors have contributed equally to this work