- Department of Neurology, The First Affiliated Hospital of Zhengzhou University, Zhengzhou University, Zhengzhou, China
Autoimmune encephalitis (AE) is a severe inflammatory disease of the brain. Patients with AE demonstrate amnesia, seizures, and psychosis. Recent studies have identified numerous associated autoantibodies (e.g., against NMDA receptors (NMDARs), LGI1, etc.) involved in the pathogenesis of AE, and the levels of diagnosis and treatment are thus improved dramatically. However, there are drawbacks of clinical diagnosis and treatment based solely on antibody levels, and thus the application of additional biomarkers is urgently needed. Considering the important role of immune mechanisms in AE development, we summarize the relevant research progress in identifying cerebrospinal fluid (CSF) biomarkers with a focus on cytokines/chemokines, demyelination, and nerve damage.
Introduction
Autoimmune encephalitis (AE) refers to a type of encephalitis caused by an autoimmune inflammatory response, which is characterized by abnormal mental behavior, epilepsy, and memory impairment. Different antibodies may have different clinical manifestations. Early diagnosis of AE is difficult due to atypical clinical symptoms and inconclusive laboratory examination results. So far, AE patients suffer from limited treatment methods and long-lasting treatment cycle, and those who are severely affected also faced with higher treatment cost and mortality. They may also sustain relapse that seriously affects the life quality and causes huge social burden.
As the most prevalent classification method, Graus et al. (1) sorted AE based on antibodies against neuronal cell-surface or synaptic proteins. These antibodies are known as antibodies against intracellular antigens, antibodies against synaptic receptors, antibodies against ion channels, and other cell-surface proteins, respectively. Since the discovery of anti-N-methyl-D-aspartate receptor (NMDAR) encephalitis in 2007, many autoimmune antibodies related to AE have been discovered, leading to a sharp increase in the detection rate compared to that of infectious encephalitis (2–4). The relevant autoantibodies are mainly directed against NMDAR, leucine-rich glioma-inactivated 1 (LGI1), γ-aminobutyric acid A / B receptors (GABAA/B), contact in-associated protein 2 (CASPR2), α-amino-3-hydroxy-5-methyl-4-isoxazolepropionic acid receptor (AMPAR), dipeptidyl-peptidase-like protein-6 (DPPX), and voltage-gated potassium channel (VGKC). Among them, anti-NMDAR encephalitis is the most common and easily recognized form of AE (5–12). How these pathological autoantibodies enter the brain to cause neuropathology and affect neural circuits remains unclear (13). Studies show that viral infection, tumors, and other factors can result in AE (14). Molecular stimulation or induction of antigen release is the most important pathogenesis, leading to the production of autoantibodies. Once these antibodies recognize neuronal receptors or synaptic proteins as foreign epitopes, the antibody-mediated AE is triggered (13, 15, 16). Changes in CD4+ and CD8+ T-cells in peripheral blood and CSF has also been reported using flow cytometry, especially in autoimmune limbic encephalitis (17–20). The diagnosis of AE is difficult during the early stages due to the varied symptoms and atypical results in routine cerebrospinal fluid (CSF) examination (21, 22). Currently, the diagnosis of AE depends on positive antibody detection; however, the absence of antibodies does not exclude the possibility of disease, and the measurement of the antibody level is also difficult to achieve. It is unrealistic to use the antibody detection as a necessary condition for early diagnosis (23, 24). Moreover, since live cells are not conventionally available, antibodies are generally investigated with fixed cells during routine clinical practice, which makes it easy to obtain false results. The antibody levels cannot predict disease prognosis and recurrence as well (1, 5, 25–27). Therefore, it is critical to discover novel inflammatory CNS biomarkers, especially CSF-specific markers, for clinical applications (Figure 1).
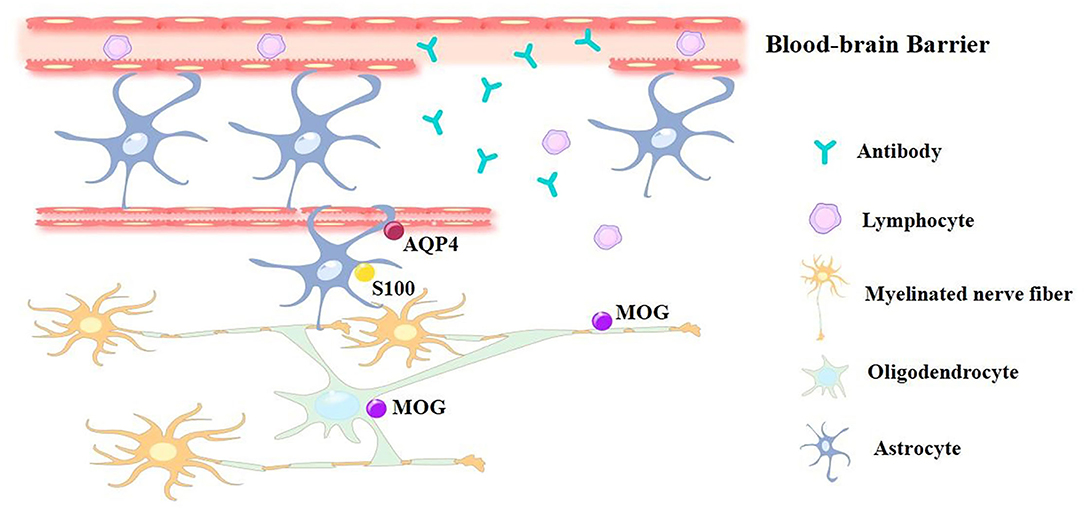
Figure 1. The pathological effect caused by blood-brain barrier damage. The blood-brain barrier damage during AE progress is caused by Th17 cell activation, this leads to antibodies and lymphocytes entering the brain and cerebra-spinal fluid. Traditional methods for AE diagnosis are using CFS to detect relevant positive Ab, while novel approach to diagnose AE are using potential biomarkers, including cytokines/chemokines and molecules like MOG, AQP4 and S100 protein that expressed on nerve cells.
Recent studies have confirmed that both humoral and cellular immunity are involved in the pathogenesis of AE (9, 28–35). In addition to the production of antibodies that can target corresponding nerve cells, neuroimmune-regulatory mechanisms also play an important role in disease progression. B and T lymphocytes are the core of humoral and cellular immunity, respectively, and their differentiation, development, and migration largely depend on cytokines and chemokines. Besides, immune-related demyelination of the nervous system, nerve cell damage, and genetic susceptibility are closely related to the occurrence of autoimmune diseases. This review provides a brief summary of this research in identifying autoimmune CSF markers and related treatments based on the factors mentioned earlier.
Cytokines/Chemokines
Cytokines are associated with the pathogenesis of a variety of autoimmune diseases (36, 37). They are important immunoregulatory factors that participate in both humoral immune responses such as B cell recruitment and differentiation, plasma cell maturation, and antibody secretion, and in cellular immune responses such as T cell differentiation and cytotoxic cell function. Chemokines are small cytokines or signal proteins secreted by various types of cells, which induce directional chemotaxis of nearby immune cells. When the body defends and clears invading pathogens, chemokines direct immune cells toward the sites of infection. Cytokines/chemokines exert multiple effects on many inflammatory cells (Figure 2A), and most of them possess unique characteristics and are elevated in many neuroinflammatory diseases of the CNS, indicating that they may be potential biomarkers (36). They are also important in disrupting the normal blood-brain barrier (BBB) and the subsequent B cell infiltration (13, 38–40). Studies have shown that cytokines and chemokines can be used as biomarkers for the diagnosis of autoimmune diseases and the detection of intrathecal inflammation. As quantifiable indicators, cytokines have the potential to be used as novel biomarkers for the evaluation of AE (41), with the cytokine profile differing depending on the antibody subtype (30). Interleukin-6 (IL-6), IL-17A, C-X-C motif chemokine ligand 10 (CXCL10), and CXCL13 in CSF have been reported to be useful for inflammation detection during the acute phase of AE while IL-15 or chitinase-3-like 1 (CHI3L1) may exhibit chronic disease activity (42). Clinical analyses of chemokine and cytokine levels would help to better understand the immune process of this disease.
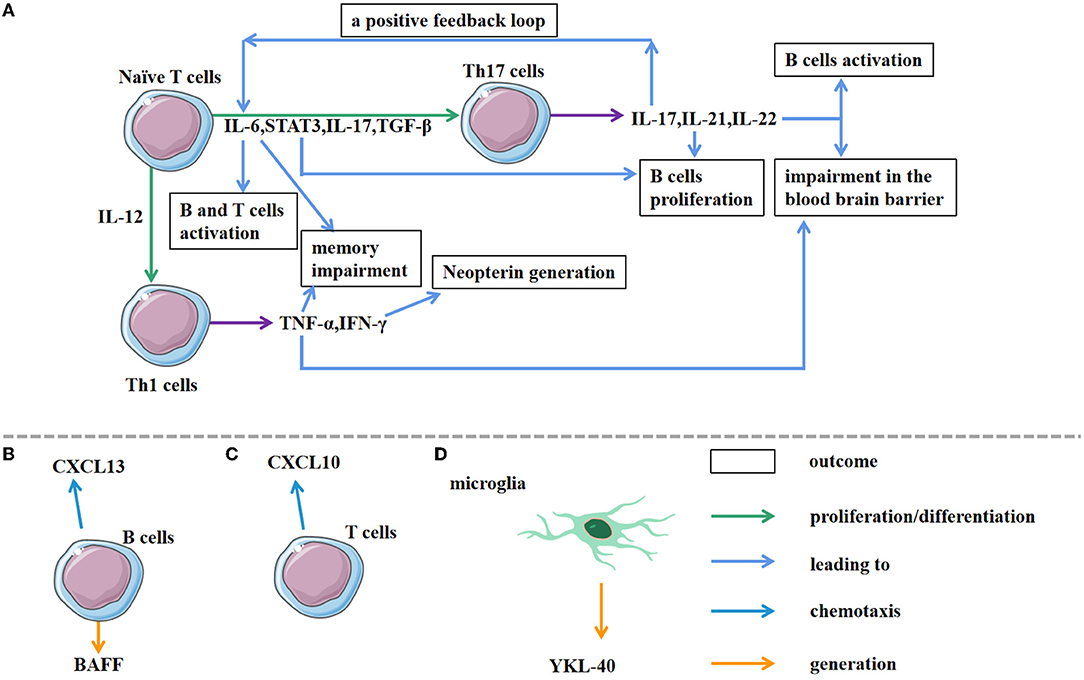
Figure 2. Associated cells involved in AE pathological processes. (A) Under the action of different cytokines, naive T cells differentiate into different Th cells. As a joint result of IL-6, STAT3, IL-17, TGF-β, naive T cells differentiate into Th 17 cells. The subsequent production of IL-17, IL-21, IL-22 by Th 17 cells triggers the immune response and impairs the blood brain barrier. In addition, IL-17A triggers a positive feedback loop of IL-6 signaling, leading to IL-17A/IL-6 co-activation. IL-12 activation can induce Th 1 cell differentiation. Furthermore, Th 1 cells secrete cytokines, such as TNF-α and IFN-γ, resulting in different outcomes. (B,C) As chemokines, CXCL13 and CXCL10 induce directional chemotaxis of B cells and T cells, respectively, towards the target sites. (D) The production of YK-40 by microglia contributes to the early diagnosis.
Factors That Promote the Differentiation and Development of Th17: IL-6, Transforming Growth Factor-β, and Signal Transducer and Activator of Transcription 3 (STAT3)
Impairments in cytokine/chemokine regulation are associated with the occurrence of AE. A large amount of cytokines and chemokines participate in the chemotaxis, differentiation, and development of Th17 cells, which have great significance in the occurrence and progression of a variety of autoimmune diseases (43, 44). Activation of excessive Th17 cells can lead to inflammation and demyelinating diseases of the CNS (45–47). As an inflammatory Th subset, Th17 cells involve in intrathecal synthesis and B cell activation. A previous study has demonstrated Th17 cell accumulation in the CNS of AE patients by comparing the CSF of 60 randomly selected anti-NMDAR encephalitis patients to patients with non-inflammatory diseases as negative control using Fluorescence Activating Cell Sorter (FACS) analysis (28). Animal experiments have shown that Th17 cells can degrade the BBB, allowing lymphocytes, antibodies, and other substances to enter the CNS. The RORγt−/− Th17 deficient mouse model also manifested reduced regulatory T cell numbers and attenuated microglial activation during post infectious basal ganglia encephalitis (BGE), a subset of AE syndromes (13). These findings highlight the key role of Th17 cells during AE activation. Cytokines/chemokines such as IL-6, IL-17 and TGF-β as well as STAT3 are the main factors that can promote the differentiation of CD4+ T cells to Th17 cells (28, 30, 43, 48, 49). IL-6 is a pleiotropic cytokine of great concern in regulating the immune system. As a powerful pro-inflammatory cytokine, it is vital for hosting resistance to pathogens and acute stress. Moreover, IL-6 counts a great deal in the auto antibody production and T cell activation. Therefore, it heavily participates in the inflammatory cascade involving T and B cell (49, 50). STAT3 acts as a carrier during interactions between cytokines and their receptors, which is mainly responsible for maintaining cellular signal transduction. It has been shown to be an important regulator of many anti-apoptotic genes. TGF-β can be produced by a variety of cells to regulate cell growth and differentiation.
Studies have reported that the pro-inflammatory cytokine IL-6 is elevated in the CSF of patients with anti-NMDAR encephalitis (30, 37, 51), but this increase is not remarkable in patients with anti-LGI1 encephalitis (30). IL-6 can attenuate the effects of NMDA-mediated excitatory postsynaptic currents (EPSCs), which contribute to memory impairment of AE patients (52). It can also strongly induce Th17 cell differentiation in vitro and may act independently to promote Th17 development and cause related autoimmunity in vivo (53). TGF-β and STAT3 activation can induce Th17 cell differentiation and participate in immune responses as well. These cytokine/chemokine-induced Th17 cell changes and immune reactions contribute to the occurrence of AE (28).
Cytokines Secreted by Th17cells: IL-17, IL-21, and Tumor Necrosis Factor-α (TNF-α)
Th17 cells promote tissue inflammation by inducing the production of pro-inflammatory cytokines, including IL-17, IL-21, and TNF-α (28, 41, 43, 47, 54–56). As these factors are all products of Th17-immunity which has been reported to be activated in AE, it is likely that they can be candidate AE biomarkers.
IL-17 secreted by Th17 is an early initiation factor of the inflammatory response, considering effects via recruiting granulocytes and macrophages (43, 57). Anti-NMDAR AE patients are usually accompanied by the IL-17 increase in their CSF and serum (30, 33, 44). IL-17 can disrupt the function of tight junctions and promote the passage of inflammatory cells through BBB. It can also promote the secretion of cytokines in a variety of cells, which participate in cell differentiation and development and cause inflammation (58–61). IL-17A may trigger a positive feedback loop of IL-6 signaling through NF-κB and STAT3, leading to the development of autoimmune diseases (62). Thus, IL-17A/IL-6 co-activation in anti-NMDAR encephalitis may be a key factor in the pathogenesis of the disease and the production of intrathecal antibodies (30).
Autocrine regulation causes Th17 cells to secrete IL-21, and this can in turn promote B cell proliferation and differentiate into plasma cells. IL-21 downregulates the functions of FOXP3+ regulatory T cells to enhance autoimmunity (63–65). Jiang et al. collected 32 AE patients, 5 patients with other autoimmune neurological disease, and 10 patients with non-inflammatory disease. A study has found a significantly increased IL-21 level in AE patients compared to control groups and even the detected levels of IL-21 in the CSF were in the low range of the assay (0–6 pg/ml). It is proposed that IL-21 may be a promising surrogate biomarker for the diagnosis of AE (41).
TNF-α is increased in the CSF of patients with anti-NMDAR encephalitis. It can reduce the integrity of the BBB and can be used to diagnose and monitor intrathecal inflammation. Recent studies have confirmed that the appearance of cognitive impairment in patients with AE is associated with TNF-α (51, 52, 66). A localized increase of TNF-α in the hippocampal dentate gyrus activates astrocyte TNF receptor type 1 (TNFR1), which then triggers astrocyte-neuron signaling cascades, leading to sustained functional changes in hippocampal excitatory synapses (66). This illustrates that during pathological conditions including Alzheimer's disease (AD), TNF-α is harmful for memory function and synaptic plasticity, and TNF-α inhibition can be used to effectively manage the disease (67, 68).
Immune-Related Chemokines: CXCL10, CXCL13, C-C Motif Ligand 19, CCL20, and CCL22
Chemokines attracted immune cells to the sites of inflammation and could be responsible for the initial pleocytosis (33). This makes them important in exploring the function of Th17 cells in patients with anti-NMDAR encephalitis (28). CCL19, CCL20, CCL22, CXCL10, CXCL13, and other chemokines are elevated in the CSF of patients with anti-NMDAR encephalitis. Some of these chemokines can increase in CFS cells and result in the early progression of the disease (28). Therefore, chemokines may be potential biomarkers for the diagnosis and monitoring of intrathecal inflammation.
CXCL13 is the main determinant of B cell recruitment during CSF neuro inflammation (Figure 2B) (69). The increased concentration of CSF CXCL13 in patients with anti-NMDAR encephalitis is associated with the synthesis of intrathecal anti-NMDAR antibodies, and thus, it may be a possible biomarker for evaluating the treatment response and prognosis (33, 70). CXCL13 is also the key chemokine that recruits plasma cells; the levels of CXCL13 in CSF are correlated with the abundance of CSF plasma cells or plasmablasts (30, 71, 72). Notably, elevated CXCL13 levels in CSF are specific to anti-NMDAR encephalitis instead of anti-LGI1 encephalitis, which may be due to the different IgG subtypes between the two diseases (30). The persistence or secondary increase of CXCL13 is related to the recurrence of the disease and the limited response to treatment. Second-line treatment is recommended if the CXCL13 level remains high after first-line immunotherapy (70). Studies have suggested that CXCL13 and CXCL10 (a chemokine for T cells) are both elevated in the early-stage anti-NMDAR encephalitis (approximately 40 days), and they are associated with the complexity and early progression of the disease. As the disease develops (approximately half a year), CXCL13 gradually decreases while CXCL10 remains elevated (33), supporting the hypothesis that the B cells take part in abnormal inflammatory activation in the early stages of the disease whereas T cells participate in immune regulation. The appearance of CD4+ T cells in the lesion is induced by CXCL10 (Figure 2C). Therefore, some central chemokines such as CXCL13 and CXCL10, may be latent therapeutic targets for AE. Additionally, cytokines/chemokines like migration inhibitory factor (MIF), CCL2, CCL20, and CCL22 that act as monocytes/macrophages may continue to increase in the CSF of patients with anti-NMDAR encephalitis for several months during the recovery period. For patients with residual behavioral abnormalities, MIF and CCL2 have been reported to persist for 256 days (72). Relative studies also demonstrated that anti-NMDAR patients have a higher concentration of CCL20 and CCL22 using the ELISA assay (28). B cell activating factor (BAFF) is a pivotal indicator of B cell activation and survival, but it has been reported that there is no significant increase in BAFF in the CSF of anti-NMDAR encephalitis patients. The specific role of certain chemokines requires further study.
Other Factors Involved in Immunity: Neopterin, CHI3L1, and Osteopontin (OPN)
Neopterin is a purine-like molecule released by monocytes and macrophages, which is activated by γ-interferon. It is a marker of cellular immune activation and can induce the expression of pro-inflammatory NF-κB, intercellular adhesion molecule-1, cytokines, and other inflammatory mediators (73–75). Neopterin production is closely related to infection, especially viral infection, and the increasing level of neopterin can be seen in various inflammatory and autoimmune CNS diseases. Researchers have found that neopterin is involved in B lymphocyte proliferation, meanwhile, autoantibody production by B lymphocytes and plasma cell infiltrates is found in the CNS of AE patients. As AE is closely linked to autoimmunity and inflammation, neopterin can be a novel biomarker for AE (25, 76). It is noted that, as neopterin has a short half-life, it can be used to monitor the inflammatory activity in patients with acute inflammation or recurrent encephalitis. It is of great importance as early diagnosis of AE depending on existing Ab tests is not realistic.
CHI3L1 (YKL-40) is mainly expressed in microglia, especially during acute and chronic inflammatory reactions (Figure 2D) (77, 78), so, it is considered to be a probable marker of persistent inflammation in many diseases (79–81). As mentioned above, Il-6 participates in Th17-immunity, which is crucial for AE development. IL-6 signaling can stimulate B cell proliferation and up-regulate the expression of the inflammatory marker CHI3L1 (82). An increase in CHI3L1 levels in the brain and/or CSF is seen in various immune-related diseases, especially neurological and neurodegenerative disorders with inflammatory components like multiple sclerosis (MS), AD, and stroke (83–85). Interestingly, the CHI3L1 levels are elevated in the CSF of patients with anti-NMDAR encephalitis and correlated with the clinical MRS score. This suggests that CHI3L1 may be a biomarker for the prognosis of anti-NMDAR encephalitis (51, 82).
OPN is a phosphorylated protein expressed in various cells (86), which is vital in many pathophysiological processes including inflammation and immune responses (87, 88). OPN can induce B cell proliferation and antibody production, and it is also crucial in Th17 cell differentiation. As mentioned earlier, Th17 cells partake in intrathecal antibody synthesis and B cell activation, hence contributing to anti-NMDAR encephalitis (89–91). Therefore, OPN may also be used as a biomarker of anti-NMDAR encephalitis (82).
Nerve Damage and Glial Activity Markers
The occurrence of CNS diseases including AE may lead to nerve damage. For example, by actively participating in AE progression, inflammatory events can directly cause the damage of nerve cells including neurons and oligodendrocytes (92, 93). Patients with AE often present with leukoencephalopathy syndrome (94–97), which occurs simultaneously or in succession (98). As structural elements of neurons and glial cells, the neurofilament light (Nfl), total tau protein (T-tau), and glial fibrillary acidic protein (GFAP) imply neuronal and glial cell damage and death. Their elevation has also been found in the CSF of patients with AE (99–101). Therefore, these markers may indicate abnormal intracranial damage in patients with AE and accordingly guide clinical diagnosis and treatment.
S100 Protein
The S100 protein belongs to the calcium-binding protein family and has two important members, the related homo-dimeric proteins S100A and S100B. S100B is a CNS-specific protein, mainly produced by astrocytes. It is a biochemical marker for brain injury, and the S100B concentration is closely related to the injury degree, treatment efficacy, and prognosis (102, 103). Many factors such as TNF-α can stimulate the release of S100B (104). Studies have manifested that the concentration of S100B in the CSF of patients with anti-NMDAR encephalitis is significantly higher than that in the control group. Additionally, there is a strong correlation between the concentration of S100B in CSF and the MRS score of the patient, suggesting that CSF S100B is related to the prognosis of the disease (105). S100 protein is also detectable in the CSF of patients with anti-DPPX encephalitis (106). Other studies have shown, however, that the concentration of S100B is AE-independent (107). S100A6, on the other hand, belongs to the S100A protein family and can help B lymphocytes to pass through the BBB in AE patients (108).
Progranulin (PGRN)
PGRN is a multifunctional immunomodulatory molecule which is critical in autoimmune diseases such as systemic lupus erythematosus and vasculitis (109). It has a double effect of inflammation, which not only can promote inflammation by regulating the IL-6 signaling pathway, but also can have an anti-inflammatory effect by antagonizing the TNF-α signaling pathway or regulating IL-10 and other signaling pathways (110–112). PGRN can promote the differentiation of CD4+ T cells into regulatory T cells (Tregs) and enhance their functions (111). The level of PGRN is elevated in the CSF of patients with anti-NMDAR encephalitis, indicating that the concentration of PGRN in the CSF may be a prospective biomarker of acute anti-NMDAR encephalitis. In patients with anti-LGI1 encephalitis, on the contrary, the PGRN in the CSF remains normal. Although PGRN is also considered to be a biomarker for certain tumors (such as lymphoma) as well, PGRN is not increased in the serum or CSF of AE patients with paraneoplastic syndrome (113, 114).
Neurofilament Light (Nfl)
Neurofilament light (NfL) chains are scaffolding proteins expressing specifically on the neural skeleton. They have been used as unspecific markers of axonal damage during neurodegeneration and neuroinflammation. To investigate the association between the CSF-Nfl level and AE, researchers collected CSF from AE patients including 37 of anti-NMDAR encephalitis and 16 of anti-LGI1 encephalitis. They found that compared to the control groups and AE patients without Nfl, people who have either of these 2 types of AE accompanied by CSF-Nfl elevation manifested poor diagnosis and prognosis. There is also a strong correlation between CSF-Nfl and AE pathogenesis (115). Moreover, there is also a significant difference among anti-NMDAR AE patients with various etiologies (114, 116). Kammeyer et al. (117) collected 26 plasma and 14 CSF samples of patients with autoimmune neurological disorders, compared to the control group, and patients with active AE show an elevated level of plasma Nfl, implying that it may act as a minimally-invasive biomarker for CNS injury during AE development. Another study also confirmed the opinion that the Nfl level is closely related to disease severity during diagnosis (118).
GFAP
GFAP is a key component of the cytoskeleton during astrocyte development, and it is also an essential filament protein in mature astrocytes (119). As neuronal and glial cell damage markers in CSF, GFAP increased non-specifically in patients with brain disorders (120). During brain injury, full-length GFAP from injured astrocytes entered the subarachnoid CSF, which then circulated to the peripheral blood through direct venous drainage. Researchers gathered clinical data from 25 AE patients, and the follow-up analysis found that the GFAP levels in the CSF of AE patients were moderately elevated during the early stage of disease development. The final outcome (disability at 1 year) strait was associated with the CSF-GFAP levels at all time points (101).
T-Tau
As a representative marker of neuronal and axonal loss, T-tau has long been used for the diagnosis of neurodegeneration. A recent study has revealed a direct connection between AE and atypical tauopathy (121). By comparing the serum and CSF level of T-tau along with MRI data from 13 AE patients and their age-matched controls, Peter Körtvelyessy et al. found that patients with the temporal FLAIR-signal in the MRI and those developing hippocampal sclerosis are prone to have a highly increased T-tau level in CSF (114). Another research collected relevant data from 25 patients hospitalized for AE and followed for 1 year, and a high CSF level of T-tau was found during the acute phase of AE. The final outcome is directly linked to the CSF-T-tau level at around 3 months since the disease onset (101).
Oligoclonal Bands (OCBs)
OCBs are clones of immunoglobulins and can be detected in CSF or serum (122). They are related to several diseases mediated by many factors such as auto antibodies, demyelination, infection, and inflammation. Patients with anti-NMDAR encephalitis and other rarer subtypes like anti-GABABR, anti-AMPAR, and anti-DPPX encephalitis, can show OCB positive in CSF and /or serum, but patients with anti-LGI1, anti-IgLON5, anti-CASPR2, and anti-GlyR antibodies are rarely OCB positive (5, 6, 123–128). Studies have shown that the levels of OCB are correlated with an increase in the CSF cells in AE patients (5). Autoantibody negative encephalitis is a type of AE with seronegative CSF, which caused the antibody testing as non-pathognomonic. The CSF OCB positivity rate is included in the clinical diagnostic criteria for both anti-NMDAR encephalitis and possible AE with negative antibodies (1). The presence of OCBs is related to the late manifestations of anti-NMDAR encephalitis in patients with decreased consciousness, movement disorders, and autonomic symptoms (129). Moreover, OCBs predict the presence of cancer at the time of AE diagnosis. In patients with OCB-positive CSF, strict monitoring of potential malignant tumors should be considered even before knowing the results of the neuro-autoantibody tests (130).
In pediatric patients with anti-NMDAR encephalitis, mirror OCBs develop initially and then progress into intrathecal OCBs, suggesting that these patients develop a systemic autoimmune response in the beginning, which is followed by localized intrathecal antibody synthesis (122). Compared with intrathecal OCBs, mirror OCBs have a lower specificity for CNS inflammation. Mirror OCBs indicate the presence of OCBs in serum and CSF. Recent studies have demonstrated that autoimmune-related CNS disease antibodies are produced in the peripheral circulation (131, 132). Therefore, mirror OCBs should not be ignored, as they may be an important biomarker of inflammatory CNS diseases.
Markers of Synaptic Dysfunction
The increase of synaptic-associated proteins is reported in the CSF of patients with neurodegenerative diseases, suggesting an abnormal synaptic integrity and function during disease pathogenesis. Among them, neurogranin and synaptosomal-associated protein-25 (SNAP-25) are the 2 representative pre- and post-synaptic proteins, respectively (133, 134). To clarify whether these markers can also reflect the progression of antibody-mediated encephalitis (AME), researchers obtained CSF from the diagnosis of 45 patients as AME including the NMDA receptor (n = 34) and LGI1/CASPR-2 (n = 11). By comparing with 39 age- and sex-similar health control, both neurogranin and SNAP-25 were markedly decreased in the CSF of AME patients at presentation. Lower SNAP-25 in prospectively followed patients and higher neurogranin at presentation are associated with greater disease severity (135). The decreased synaptic protein level is probably due to acute synaptic dysfunction and antibody-mediated receptor internalization, which may correlate with disease severity and outcome (135).
Prospects for Treatment
The most common treatment strategies for AE are the first-line treatments such as corticosteroids, IVI g, and plasma exchange as well as the second-line treatments like rituximab, cyclophosphamide, mycophenolate mofetil, and other immunosuppressive medication (38, 136–140). It has been reported that 44% of patients with anti-NMDAR encephalitis have failed first-line treatment, demonstrating that individual patients may have specific immunological characteristics. Compared with non-targeted immunotherapy, targeted immunotherapy has the potential to improve the treatment response, and further research on targeted immunotherapy will be necessary to develop new treatment strategies (141).
Th17 cells are important immune cells involved in the pathogenesis of AE. Inhibiting the differentiation and development of Th17 cells can inhibit the development and progression of inflammation. Studies have shown that the cAMP-response element binding protein (CREB)-regulated transcription coactivator (CRTC2) can promote the differentiation of Th17 cells, therefore, its inhibitors may provide therapeutic benefits for patients with autoimmune diseases. Although there are no reports on inhibiting Th17 cells to treat AE currently, this may be a viable therapeutic strategy for AE in the near future (43). Additionally, IL-2 can constrain the differentiation of Th17 cells, and low-dose IL-2 therapy can be used to treat autoimmune diseases by restoring the balance between Tregs and effector T cells (30, 142). The low-dose IL-2 usage may be a feasible immunotherapy for refractory AE treatment.
Cytokines play a critical role in the autoimmune response. Some cytokine inhibitors, such as anti-IL-6 receptor antibody (tocilizumab), IL-17A inhibitor (secukinumab), and CXCL-10 inhibitor (50, 52, 143, 144) have been shown to block CNS immune feedback pathways and antigen-specific Th17 cell differentiation (145), and they can also effectively treat many autoimmune inflammatory diseases. AE-related cytokine inhibitors and additional cytokines/chemokines involved in AE may be identified as potential targets for future treatments (44, 146–148).
Furthermore, research on NR2B antagonists may yield potential therapeutic options (149) to protect the BBB and exhibit effective therapeutic effects without requiring antigen specificity (150).
Conclusion
Recently, AE has received increasing attention, and the level of AE diagnosis and treatment has dramatically improved. However, there are drawbacks for clinical diagnosis based solely on antibodies; therefore, additional biomarkers are needed to guide diagnosis and treatment. Considering the primary role of the immune mechanism in the pathogenesis of AE, this review summarizes the relevant research progress in identifying CSF biomarkers with a focus on cytokines/chemokines, demyelination, and nerve damage (Table 1). Furthermore, we also provide the latest information to aid the diagnosis and treatment of the disease. Additional research will increase our understanding of AE and improve the level of diagnosis and treatment for this disease.
Author Contributions
Drafting/revision of the manuscript for content and including medical writing for content: SZ, XL, and CM. Drafting/revision of the manuscript for content, including medical writing for content, and article frame design: JT and WM. All authors contributed to the article and approved the submitted version.
Funding
The author(s) disclosed the receipt of the following financial support for the research, authorship, and/or publication of this article: This study was supported by the National Natural Science Foundation of China (Grant 81901300 to CM), the Scientific and Technological Project of Henan Province (Grant SBGJ202003020 to CM), and the Youth Innovation Fund of the First Affiliated Hospital of Zhengzhou University (to SZ).
Conflict of Interest
The authors declare that the research was conducted in the absence of any commercial or financial relationships that could be construed as a potential conflict of interest.
Publisher's Note
All claims expressed in this article are solely those of the authors and do not necessarily represent those of their affiliated organizations, or those of the publisher, the editors and the reviewers. Any product that may be evaluated in this article, or claim that may be made by its manufacturer, is not guaranteed or endorsed by the publisher.
Acknowledgments
The authors are grateful to Liyuan Fan, Tai Wang, and Yun Su for technical support. We thank Prof. Yuming Xu for helpful discussion and insightful comments.
References
1. Graus F, Titulaer MJ, Balu R, Benseler S, Bien CG, Cellucci T, et al. A clinical approach to diagnosis of autoimmune encephalitis. Lancet Neurol. (2016) 15:391–404. doi: 10.1016/S1474-4422(15)00401-9
2. Dubey D, Pittock SJ, Kelly CR, McKeon A, Lopez-Chiriboga AS, Lennon VA, et al. Autoimmune encephalitis epidemiology and a comparison to infectious encephalitis. Ann Neurol. (2018) 83:166–77. doi: 10.1002/ana.25131
3. Esposito S, Principi N, Calabresi P, Rigante D. An evolving redefinition of autoimmune encephalitis. Autoimmun Rev. (2019) 18:155–63. doi: 10.1016/j.autrev.2018.08.009
4. van Coevorden-Hameete MH, de Graaff E, Titulaer MJ, Hoogenraad CC, Sillevis Smitt PA. Molecular and cellular mechanisms underlying anti-neuronal antibody mediated disorders of the central nervous system. Autoimmun Rev. (2014) 13:299–312. doi: 10.1016/j.autrev.2013.10.016
5. Blinder T, Lewerenz J. Cerebrospinal fluid findings in patients with autoimmune encephalitis-a systematic analysis. Front Neurol. (2019) 10:804. doi: 10.3389/fneur.2019.00804
6. Dalmau J, Gleichman AJ, Hughes EG, Rossi JE, Peng X, Lai M, et al. Anti-NMDA-receptor encephalitis: case series and analysis of the effects of antibodies. Lancet Neurol. (2008) 7:1091–8. doi: 10.1016/S1474-4422(08)70224-2
7. Lai M, Huijbers MG, Lancaster E, Graus F, Bataller L, Balice-Gordon R, et al. Investigation of LGI1 as the antigen in limbic encephalitis previously attributed to potassium channels: a case series. Lancet Neurol. (2010) 9:776–85. doi: 10.1016/S1474-4422(10)70137-X
8. van Sonderen A, Thijs RD, Coenders EC, Jiskoot LC, Sanchez E, de Bruijn MA, et al. Anti-LGI1 encephalitis: clinical syndrome and long-term follow-up. Neurology. (2016) 87:1449–56. doi: 10.1212/WNL.0000000000003173
9. Bien CG, Vincent A, Barnett MH, Becker AJ, Blümcke I, Graus F, et al. Immunopathology of autoantibody-associated encephalitides: clues for pathogenesis. Brain. (2012) 135:1622–38. doi: 10.1093/brain/aws082
10. Brenton JN, Goodkin HP. Antibody-mediated autoimmune encephalitis in childhood. Pediatr Neurol. (2016) 60:13–23. doi: 10.1016/j.pediatrneurol.2016.04.004
11. Sinmaz N, Nguyen T, Tea F, Dale RC, Brilot F. Mapping autoantigen epitopes: molecular insights into autoantibody-associated disorders of the nervous system. J Neuroinflammation. (2016) 13:219. doi: 10.1186/s12974-016-0678-4
12. Guevara C, Farias G, Silva-Rosas C, Alarcon P, Abudinen G, Espinoza J, et al. Encephalitis associated to Metabotropic Glutamate Receptor 5 (mGluR5) antibodies in cerebrospinal fluid. Front Immunol. (2018) 9:2568. doi: 10.3389/fimmu.2018.02568
13. Platt MP, Bolding KA, Wayne CR, Chaudhry S, Cutforth T, Franks KM, et al. Th17 lymphocytes drive vascular and neuronal deficits in a mouse model of postinfectious autoimmune encephalitis. Proc Natl Acad Sci U S A. (2020) 117:6708–16. doi: 10.1073/pnas.1911097117
14. Ramanathan S, Al-Diwani A, Waters P, Irani SR. The autoantibody-mediated encephalitides: from clinical observations to molecular pathogenesis. J Neurol. (2019) 268:1689–707. doi: 10.1007/s00415-019-09590-9
15. Armangue T, Spatola A, Vlagea S, Mattozzi M, Cárceles-Cordon E, Martinez-Heras S, et al. Frequency, symptoms, risk factors, and outcomes of autoimmune encephalitis after herpes simplex encephalitis: a prospective observational study and retrospective analysis. Lancet Neurol. (2018) 17:760–72. doi: 10.1016/S1474-4422(18)30244-8
16. Armangue T, Leypoldt F, Málaga L, Raspall-Chaure M, Marti I, Nichter C, et al. Herpes simplex virus encephalitis is a trigger of brain autoimmunity. Ann Neurol. (2014) 75:317–23. doi: 10.1002/ana.24083
17. Dik A, Widman G, Schulte-Mecklenbeck A, Witt JA, Pitsch J, Golombeck KS, et al. Impact of T cells on neurodegeneration in anti-GAD65 limbic encephalitis. Ann Clin Trans Neurol. (2021) 8:2289–301. doi: 10.1002/acn3.51486
18. Helmstaedter C, Hensen N, Leelaarporn P, Schwing K, Oender D, Widman G, et al. Specific B- and T-cell populations are associated with cognition in patients with epilepsy and antibody positive and negative suspected limbic encephalitis. J Neurol. (2021) 268:455–66. doi: 10.1007/s00415-020-10158-1
19. Hansen N, Onder D, Schwing K, Widman G, Leelaarporn P, Prusseit I, et al. CD19+ B-cells in autoantibody-negative limbic encephalitis. Epilepsy Behav. (2020) 106:107016. doi: 10.1016/j.yebeh.2020.107016
20. Hansen N, Schwing K, Önder D, Widman G, Leelaarporn P, Prusseit I, et al. Low CSF CD4/CD8+ T-cell proportions are associated with blood-CSF barrier dysfunction in limbic encephalitis. Epilepsy Behav. (2020) 102:106682. doi: 10.1016/j.yebeh.2019.106682
21. Baumgartner A, Rauer S, Hottenrott T, Leypoldt F, Ufer F, Hegen H, et al. Admission diagnoses of patients later diagnosed with autoimmune encephalitis. J Neurol. (2019) 266:124–32. doi: 10.1007/s00415-018-9105-3
22. Wang R, Guan HZ, Ren HT, Wang W, Hong Z, Zhou D, et al. CSF findings in patients with anti-N-methyl-D-aspartate receptor-encephalitis. Seizure. (2015) 29:137–42. doi: 10.1016/j.seizure.2015.04.005
23. Ricken G, Schwaiger C, Simoni D De, Pichler V, Lang J, Glatter S, et al. Detection methods for autoantibodies in suspected autoimmune encephalitis. Front Neurol. (2018) 9:841. doi: 10.3389/fneur.2018.00841
24. Lascano AM, Vargas MI, Lalive PH. Diagnostic tools for immune causes of encephalitis. Clin Microbiol Infect. (2019) 25:431–6. doi: 10.1016/j.cmi.2018.12.012
25. Fominykh V, Brylev L, Gaskin V, Luzin R, Yakovlev A, Komoltsev I, et al. Neuronal damage and neuroinflammation markers in patients with autoimmune encephalitis and multiple sclerosis. Metab Brain Dis. (2019) 34:1473–85. doi: 10.1007/s11011-019-00452-x
26. Thomas A, Rauschkolb P, Gresa-Arribas N, Schned A, Dalmau JO, Fadul CE, et al. Anti-N-methyl-D-aspartate receptor encephalitis: a patient with refractory illness after 25 months of intensive immunotherapy. JAMA Neurol. (2013) 70:1566–8. doi: 10.1001/jamaneurol.2013.3205
27. Mariotto S, Andreetta F, Farinazzo A, Monaco S, Ferrari S. Persistence of anti-NMDAR antibodies in CSF after recovery from autoimmune encephalitis. Neurol Sci. (2017) 38:1523–4. doi: 10.1007/s10072-017-2958-x
28. Zeng C, Chen L, Chen B, Cai Y, Li P, Yan L, et al. Th17 cells were recruited and accumulated in the cerebrospinal fluid and correlated with the poor prognosis of anti-NMDAR encephalitis. Acta Biochim Biophys Sin. (2018) 50:1266–73. doi: 10.1093/abbs/gmy137
29. Tüzün E, Zhou L, Baehring JM, Bannykh S, Rosenfeld MR, Dalmau J, et al. Evidence for antibody-mediated pathogenesis in anti-NMDAR encephalitis associated with ovarian teratoma. Acta Neuropathol. (2009) 118:737–43. doi: 10.1007/s00401-009-0582-4
30. Byun JI, Lee ST, Moon J, Jung KH, Sunwoo JS, Lim JA, et al. Distinct intrathecal interleukin-17/interleukin-6 activation in anti-N-methyl-d-aspartate receptor encephalitis. J Neuroimmunol. (2016) 297:141–7. doi: 10.1016/j.jneuroim.2016.05.023
31. Dale RC, Pillai S, Brilot F. Cerebrospinal fluid CD19(+) B-cell expansion in N-methyl-D-aspartate receptor encephalitis. Dev Med Child Neurol. (2013) 55:191–3. doi: 10.1111/dmcn.12036
32. Camdessanché JP, Streichenberger N, Cavillon G, Rogemond V, Jousserand G, Honnorat J, et al. Brain immunohistopathological study in a patient with anti-NMDAR encephalitis. Eur J Neurol. (2011) 18:929–31. doi: 10.1111/j.1468-1331.2010.03180.x
33. Liba Z, Kayserova J, Elisak M, Marusic P, Nohejlova H, Hanzalova J, et al. Anti-N-methyl-D-aspartate receptor encephalitis: the clinical course in light of the chemokine and cytokine levels in cerebrospinal fluid. J Neuroinflammation. (2016) 13:55. doi: 10.1186/s12974-016-0507-9
34. Bracher A, Alcalá C, Ferrer J, Melzer N, Hohlfeld R, Casanova B, et al. An expanded parenchymal CD8+ T cell clone in GABA(A) receptor encephalitis. Ann Clin Transl Neurol. (2020) 7:239–44. doi: 10.1002/acn3.50974
35. Venkatesan A, Adatia K. Anti-NMDA-receptor encephalitis: from bench to clinic. ACS Chem Neurosci. (2017) 8:2586–95. doi: 10.1021/acschemneuro.7b00319
36. Kothur K, Wienholt L, Mohammad SS, Tantsis EM, Pillai S, Britton PN, et al. Utility of CSF cytokine/chemokines as markers of active intrathecal inflammation: comparison of demyelinating, Anti-NMDAR and enteroviral encephalitis. PloS ONE. (2016) 11:e0161656. doi: 10.1371/journal.pone.0161656
37. Kothur K, Wienholt L, Brilot F, Dale RC. CSF cytokines/chemokines as biomarkers in neuroinflammatory CNS disorders: a systematic review. Cytokine. (2016) 77:227–37. doi: 10.1016/j.cyto.2015.10.001
38. Martinez-Hernandez E, Horvath J, Shiloh-Malawsky Y, Sangha N, Martinez-Lage M, Dalmau J, et al. Analysis of complement and plasma cells in the brain of patients with anti-NMDAR encephalitis. Neurology. (2011) 77:589–93. doi: 10.1212/WNL.0b013e318228c136
39. Platt MP, Agalliu D, Cutforth T. Hello from the other side: how autoantibodies circumvent the blood-brain barrier in autoimmune encephalitis. Front Immunol. (2017) 8:442. doi: 10.3389/fimmu.2017.00442
40. Bendszus M, Bartsch A, Stoll G. Is the disruption of the blood-brain barrier a prerequisite for cellular infiltration in autoimmune encephalitis? Brain. (2005) 128:E25. doi: 10.1093/brain/awh389
41. Jiang JX, Fewings N, Dervish S, Fois AF, Duma SR, Silsby M, et al. Novel surrogate markers of CNS inflammation in CSF in the diagnosis of autoimmune encephalitis. Front Neurol. (2019) 10:1390. doi: 10.3389/fneur.2019.01390
42. Hansen N, Timäus C. Neues zu biologischen markern bei autoimmunenzephalitis. Zeitschrift für Epileptologie. (2020) 33:288–93. doi: 10.1007/s10309-020-00356-2
43. Hernandez JB, Chang C, LeBlanc M, Grimm D, Le Lay J, Kaestner KH, et al. The CREB/CRTC2 pathway modulates autoimmune disease by promoting Th17 differentiation. Nature Commun. (2015) 6:7216. doi: 10.1038/ncomms8216
44. Ulusoy C, Tüzün E, Kürtüncü M, Türkoglu R, Akman-Demir G, Eraksoy M. et al. Comparison of the cytokine profiles of patients with neuronal-antibody-associated central nervous system disorders. Int J Neurosci. (2012) 122:284–9. doi: 10.3109/00207454.2011.648762
45. Zhu J, Yamane H, Paul WE. Differentiation of effector CD4 T cell populations (*). Annu Rev Immunol. (2010) 28:445–89. doi: 10.1146/annurev-immunol-030409-101212
46. Lovett-Racke AE, Yang Y, Racke MK. Th1 vs. Th17: are T cell cytokines relevant in multiple sclerosis? Biochim Biophys Acta. (2011) 1812:246–51. doi: 10.1016/j.bbadis.2010.05.012
47. Kreymborg K, Etzensperger R, Dumoutier L, Haak S, Rebollo A, Buch T, et al. IL-22 is expressed by Th17 cells in an IL-23-dependent fashion, but not required for the development of autoimmune encephalomyelitis. J Immunol. (2007) 179:8098–104. doi: 10.4049/jimmunol.179.12.8098
48. Tanaka K, Martinez GJ, Yan X, Long W, Ichiyama K, Chi X, et al. Regulation of pathogenic T Helper 17 cell differentiation by steroid receptor coactivator-3. Cell Rep. (2018) 23:2318–29. doi: 10.1016/j.celrep.2018.04.088
49. Guo D, Chen Y, Wang S, Yu L, Shen Y, Zhong H, et al. Exosomes from heat-stressed tumour cells inhibit tumour growth by converting regulatory T cells to Th17 cells via IL-6. Immunology. (2018) 154:132–43. doi: 10.1111/imm.12874
50. Yao X, Huang J, Zhong H, Shen N, Faggioni R, Fung M, et al. Targeting interleukin-6 in inflammatory autoimmune diseases and cancers. Pharmacol Ther. (2014) 141:125–39. doi: 10.1016/j.pharmthera.2013.09.004
51. Chen J, Ding Y, Zheng D, Wang Z, Pan S, Ji T, et al. Elevation of YKL-40 in the CSF of anti-NMDAR encephalitis patients is associated with poor prognosis. Front Neurol. (2018) 9:727. doi: 10.3389/fneur.2018.00727
52. Wang X, Ma C, Liu CY, Li GJ, Zhao D, Han DF, et al. Neuronal NMDAR currents of the hippocampus and learning performance in autoimmune anti-NMDAR encephalitis and involvement of TNF-α and IL-6. Front Neurol. (2019) 10:684. doi: 10.3389/fneur.2019.00684
53. Sonderegger I, Kisielow J, Meier R, King C, Kopf M. IL-21 and IL-21R are not required for development of Th17 cells and autoimmunity in vivo. Eur J Immunol. (2008) 38:1833–8. doi: 10.1002/eji.200838511
54. Sakaguchi R, Chikuma S, Shichita T, Morita R, Sekiya T, Ouyang W, et al. Innate-like function of memory Th17 cells for enhancing endotoxin-induced acute lung inflammation through IL-22. Int Immunol. (2016) 28:233–43. doi: 10.1093/intimm/dxv070
55. Thomas LS, Targan SR, Tsuda M, Yu QT, Salumbides BC, Haritunians T, et al. The TNF family member TL1A induces IL-22 secretion in committed human T(h)17 cells via IL-9 induction. J Leukocyte Biol. (2017) 101:727–37. doi: 10.1189/jlb.3A0316-129R
56. Bălănescu P, Bălănescu E, Bălănescu A. IL-17 and Th17 cells in systemic sclerosis: a comprehensive review. Rom J Intern Med. (2017) 55:198–204. doi: 10.1515/rjim-2017-0027
57. Kumar P, Shih DCW, Lim A, Paleja B, Ling S, Yun LL, et al. Pro-inflammatory, IL-17 pathways dominate the architecture of the immunome in pediatric refractory epilepsy. JCI Insight. (2019) 5:e126337. doi: 10.1172/jci.insight.126337
58. Fujita K, Matsui N, Takahashi Y, Iwasaki Y, Yoshida M, Yuasa T, et al. Increased interleukin-17 in the cerebrospinal fluid in sporadic Creutzfeldt-Jakob disease: a case-control study of rapidly progressive dementia. J Neuroinflammation. (2013) 10:135. doi: 10.1186/1742-2094-10-135
59. Selmi C, Barin JG, Rose NR. Current trends in autoimmunity and the nervous system. J Autoimmun. (2016) 75:20–9. doi: 10.1016/j.jaut.2016.08.005
60. Huppert J, Closhen D, Croxford A, White R, Kulig P, Pietrowski E, et al. Cellular mechanisms of IL-17-induced blood-brain barrier disruption. FASEB J. (2010) 24:1023–34. doi: 10.1096/fj.09-141978
61. Kebir H, Kreymborg K, Ifergan I, Dodelet-Devillers A, Cayrol R, Bernard M, et al. Human TH17 lymphocytes promote blood-brain barrier disruption and central nervous system inflammation. Nat Med. (2007) 13:1173–5. doi: 10.1038/nm1651
62. Ogura H, Murakami M, Okuyama Y, Tsuruoka M, Kitabayashi C, Kanamoto M, et al. Interleukin-17 promotes autoimmunity by triggering a positive-feedback loop via interleukin-6 induction. Immunity. (2008) 29:628–36. doi: 10.1016/j.immuni.2008.07.018
63. Leonard WJ, Spolski R. Interleukin-21: a modulator of lymphoid proliferation, apoptosis and differentiation. Nat Rev Immunol. (2005) 5:688–98. doi: 10.1038/nri1688
64. Spolski R, Leonard WJ. Interleukin-21: a double-edged sword with therapeutic potential. Nat Rev Drug Discov. (2014) 13:379–95. doi: 10.1038/nrd4296
65. Brandt K, Singh PB, Bulfone-Paus S, Rückert R. Interleukin-21: a new modulator of immunity, infection, and cancer. Cytokine Growth Factor Rev. (2007) 18:223–32. doi: 10.1016/j.cytogfr.2007.04.003
66. Habbas S, Santello M, Becker D, Stubbe H, Zappia G, Liaudet N, et al. Neuroinflammatory TNFα impairs memory via astrocyte signaling. Cell. (2015) 163:1730–41. doi: 10.1016/j.cell.2015.11.023
67. Carta AR, Frau L, Pisanu A, Wardas J, Spiga S, Carboni E, et al. Rosiglitazone decreases peroxisome proliferator receptor-γ levels in microglia and inhibits TNF-α production: new evidences on neuroprotection in a progressive Parkinson's disease model. Neuroscience. (2011) 194:250–61. doi: 10.1016/j.neuroscience.2011.07.046
68. Giuliani F, Vernay A, Leuba G, Schenk F. Decreased behavioral impairments in an Alzheimer mice model by interfering with TNF-alpha metabolism. Brain Res Bull. (2009) 80:302–8. doi: 10.1016/j.brainresbull.2009.07.009
69. Schmidt C, Plate A, Angele B, Pfister HW, Wick M, Koedel U, et al. A prospective study on the role of CXCL13 in lyme neuroborreliosis. Neurology. (2011) 76:1051–8. doi: 10.1212/WNL.0b013e318211c39a
70. Leypoldt F, Höftberger R, Titulaer MJ, Armangue T, Gresa-Arribas N, Jahn H, et al. Investigations on CXCL13 in anti-N-methyl-D-aspartate receptor encephalitis: a potential biomarker of treatment response. JAMA Neurol. (2015) 72:180–6. doi: 10.1001/jamaneurol.2014.2956
71. Kowarik MC, Cepok S, Sellner J, Grummel V, Weber MS, Korn T, et al. CXCL13 is the major determinant for B cell recruitment to the CSF during neuroinflammation. J Neuroinflammation. (2012) 9:93. doi: 10.1186/1742-2094-9-93
72. Omae T, Saito Y, Tsuchie H, Ohno K, Maegaki Y, Sakuma H, et al. Cytokine/chemokine elevation during the transition phase from HSV encephalitis to autoimmune anti-NMDA receptor encephalitis. Brain Dev. (2018) 40:361–5. doi: 10.1016/j.braindev.2017.12.007
73. Hagberg L, Cinque P, Gisslen M, Brew BJ, Spudich S, Bestetti A, et al. Cerebrospinal fluid neopterin: an informative biomarker of central nervous system immune activation in HIV-1 infection. AIDS Res Ther. (2010) 7:15. doi: 10.1186/1742-6405-7-15
74. Dale RC, Brilot F. Biomarkers of inflammatory and auto-immune central nervous system disorders. Curr Opin Pediatr. (2010) 22:718–25. doi: 10.1097/MOP.0b013e3283402b71
75. Hoffmann G, Wirleitner B, Fuchs D. Potential role of immune system activation-associated production of neopterin derivatives in humans. Inflamm Res. (2003) 52:313–21. doi: 10.1007/s00011-003-1181-9
76. Dale RC, Brilot F, Fagan E, Earl J. Cerebrospinal fluid neopterin in paediatric neurology: a marker of active central nervous system inflammation. Dev Med Child Neurol. (2009) 51:317–23. doi: 10.1111/j.1469-8749.2008.03225.x
77. Rathcke CN, Vestergaard H. YKL-40, a new inflammatory marker with relation to insulin resistance and with a role in endothelial dysfunction and atherosclerosis. Inflamm Res. (2006) 55:221–7. doi: 10.1007/s00011-006-0076-y
78. Craig-Schapiro R, Perrin RJ, Roe CM, Xiong C, Carter D, Cairns NJ, et al. YKL-40: a novel prognostic fluid biomarker for preclinical Alzheimer's disease. Biol Psychiatry. (2010) 68:903–12. doi: 10.1016/j.biopsych.2010.08.025
79. Tanwar MK, Gilbert MR, Holland EC. Gene expression microarray analysis reveals YKL-40 to be a potential serum marker for malignant character in human glioma. Cancer Res. (2002) 62:4364–8.
80. Bonneh-Barkay D, Wang G, Starkey A, Hamilton RL, Wiley CA. In vivo CHI3L1 (YKL-40) expression in astrocytes in acute and chronic neurological diseases. J Neuroinflammation. (2010) 7:34. doi: 10.1186/1742-2094-7-34
81. Alcolea D, Vilaplana E, Pegueroles J, Montal V, Sánchez-Juan P, González-Suárez A, et al. Relationship between cortical thickness and cerebrospinal fluid YKL-40 in predementia stages of Alzheimer's disease. Neurobiol Aging. (2015) 36:2018–23. doi: 10.1016/j.neurobiolaging.2015.03.001
82. Zhao J, Wang C, Zhang Y, Sun R, Wang H, Li G, et al. Elevated CHI3L1 and OPN levels in patients with anti-N-methyl-d-aspartate receptor encephalitis. J Neuroimmunol. (2019) 334:577005. doi: 10.1016/j.jneuroim.2019.577005
83. Comabella M, Fernández M, Martin R, Rivera-Vallvé S, Borrás E, Chiva C, et al. Cerebrospinal fluid chitinase 3-like 1 levels are associated with conversion to multiple sclerosis. Brain. (2010) 133:1082–93. doi: 10.1093/brain/awq035
84. Olsson B, Lautner R, Andreasson U, Öhrfelt A, Portelius E, Bjerke M, et al. CSF and blood biomarkers for the diagnosis of Alzheimer's disease: a systematic review and meta-analysis. Lancet Neurol. (2016) 15:673–84. doi: 10.1016/S1474-4422(16)00070-3
85. Hjalmarsson C, Bjerke M, Andersson B, Blennow K, Zetterberg H, Aberg ND, et al. Neuronal and glia-related biomarkers in cerebrospinal fluid of patients with acute ischemic stroke J Cent Nerv Syst Dis. (2014) 6:51–8. doi: 10.4137/JCNSD.S13821
86. Chiodoni C, Colombo MP, Sangaletti S. Matricellular proteins: from homeostasis to inflammation, cancer, and metastasis. Cancer Metastasis Rev. (2010) 29:295–307. doi: 10.1007/s10555-010-9221-8
87. Rittling SR, Singh R. Osteopontin in immune-mediated diseases. J Dent Res. (2015) 94:1638–45. doi: 10.1177/0022034515605270
88. Sun Y, Yin XS, Guo H, Han RK, He RD, Chi LJ, et al. Elevated osteopontin levels in mild cognitive impairment and Alzheimer's disease. Mediators Inflamm. (2013) 2013:615745. doi: 10.1155/2013/615745
89. Wang KX, Denhardt DT. Osteopontin: role in immune regulation and stress responses. Cytokine Growth Factor Rev. (2008) 19:333–45. doi: 10.1016/j.cytogfr.2008.08.001
90. Agah E, Zardoui A, Saghazadeh A, Ahmadi M, Tafakhori A, Rezaei N, et al. Osteopontin (OPN) as a CSF and blood biomarker for multiple sclerosis: a systematic review and meta-analysis. PloS ONE. (2018) 13:e0190252. doi: 10.1371/journal.pone.0190252
91. Carecchio M, Comi C. The role of osteopontin in neurodegenerative diseases. J Alzheimers Dis. (2011) 25:179–85. doi: 10.3233/JAD-2011-102151
92. Mayo L, Quintana FJ, Weiner HL. The innate immune system in demyelinating disease. Immunol Rev. (2012) 248:170–87. doi: 10.1111/j.1600-065X.2012.01135.x
93. Perlman S, Zhao J. Roles of regulatory T cells and IL-10 in virus-induced demyelination. J Neuroimmunol. (2017) 308:6–11. doi: 10.1016/j.jneuroim.2017.01.001
94. Kruer MC, Koch TK, Bourdette DN, Chabas D, Waubant E, Mueller S, et al. NMDA receptor encephalitis mimicking seronegative neuromyelitis optica. Neurology. (2010) 74:1473–5. doi: 10.1212/WNL.0b013e3181dc1a7f
95. Lekoubou A, Viaccoz A, Didelot A, Anastasi A, Marignier R, Ducray F, et al. Anti-N-methyl-D-aspartate receptor encephalitis with acute disseminated encephalomyelitis-like MRI features. Eur J Neurol. (2012) 19:e16–7. doi: 10.1111/j.1468-1331.2011.03617.x
96. Takeda A, Shimada H, Tamura A, Yasui M, Yamamoto K, Itoh K, et al. A case of anti-N-methyl-d-aspartate receptor encephalitis with multiple sclerosis-like demyelinated lesions. Mult Scler Relat Disord. (2014) 3:391–7. doi: 10.1016/j.msard.2013.09.005
97. Gahr M, Lauda F, Wigand ME, Connemann BJ, Rosenbohm A, Tumani H, et al. Periventricular white matter lesion and incomplete MRZ reaction in a male patient with anti-N-methyl-D-aspartate receptor encephalitis presenting with dysphoric mania. BMJ Case Rep. (2015) 2015:bcr2014209075. doi: 10.1136/bcr-2014-209075
98. Titulaer MJ, Höftberger R, Iizuka T, Leypoldt F, McCracken L, Cellucci T, et al. Overlapping demyelinating syndromes and anti–N-methyl-D-aspartate receptor encephalitis. Ann Neurol. (2014) 75:411–28. doi: 10.1002/ana.24117
99. Yang X, Huang Q, Yang H, Liu S, Chen B, Liu T, et al. Astrocytic damage in glial fibrillary acidic protein astrocytopathy during initial attack. Mult Scler Relat Disord. (2019) 29:94–9. doi: 10.1016/j.msard.2019.01.036
100. Iorio R, Damato V, Evoli A, Gessi M, Gaudino S, Lazzaro VDi, et al. Clinical and immunological characteristics of the spectrum of GFAP autoimmunity: a case series of 22 patients. J Neurol Neurosurg Psychiatry. (2018) 89:138–46. doi: 10.1136/jnnp-2017-316583
101. Constantinescu R, Krýsl D, Bergquist F, Andrén K, Malmeström C, Asztély F, et al. Cerebrospinal fluid markers of neuronal and glial cell damage to monitor disease activity and predict long-term outcome in patients with autoimmune encephalitis. Eur J Neurol. (2016) 23:796–806. doi: 10.1111/ene.12942
102. Batlle M, Ferri L, Andrade C, Ortega FJ, Vidal-Taboada JM, Pugliese M, et al. Astroglia-microglia cross talk during neurodegeneration in the rat hippocampus. Biomed Res Int. (2015) 2015:102419. doi: 10.1155/2015/102419
103. Persson L, Hårdemark HG, Gustafsson J, Rundström G, Mendel-Hartvig I, Esscher T, et al. S-100 protein and neuron-specific enolase in cerebrospinal fluid and serum: markers of cell damage in human central nervous system. Stroke. (1987) 18:911–8. doi: 10.1161/01.STR.18.5.911
104. Edwards MM, Robinson SR. TNF alpha affects the expression of GFAP and S100B: implications for Alzheimer's disease. J Neural Transm. (2006) 113:1709–15. doi: 10.1007/s00702-006-0479-5
105. Liu B, Xie Z, Liu G, Gu Y, Pan S, Wang H, et al. Elevated neuron-specific enolase and S100 calcium-binding protein B concentrations in cerebrospinal fluid of patients with anti-N-methyl-d-aspartate receptor encephalitis. Clin Chim Acta. (2018) 480:79–83. doi: 10.1016/j.cca.2018.01.016
106. Stoeck K, Carstens PO, Jarius S, Raddatz D, Stöcker W, Wildemann B, et al. Prednisolone and azathioprine are effective in DPPX antibody-positive autoimmune encephalitis. Neurol Neuroimmunol Neuroinflamm. (2015) 2:e86. doi: 10.1212/NXI.0000000000000086
107. Sveinsson O, Granqvist M, Forslin Y, Blennow K, Zetterberg H, Piehl F, et al. Successful combined targeting of B- and plasma cells in treatment refractory anti-NMDAR encephalitis. J Neuroimmunol. (2017) 312:15–8. doi: 10.1016/j.jneuroim.2017.08.011
108. Tsai MH, Lin CH, Tsai KW, Lin MH, Ho CJ, Lu YT, et al. S100A6 promotes B lymphocyte penetration through the blood-brain barrier in autoimmune encephalitis. Front Genet. (2019) 10:1188. doi: 10.3389/fgene.2019.01188
109. Thurner L, Preuss KD, Fadle N, Regitz E, Klemm P, Zaks M, et al. Progranulin antibodies in autoimmune diseases. J Autoimmun. (2013) 42:29–38. doi: 10.1016/j.jaut.2012.10.003
110. Muynck L De, Van Damme P. Cellular effects of progranulin in health and disease. J Mol Neurosci. (2011) 45:549–60. doi: 10.1007/s12031-011-9553-z
111. Jian J, Li G, Hettinghouse A, Liu C. Progranulin: a key player in autoimmune diseases. Cytokine. (2018) 101:48–55. doi: 10.1016/j.cyto.2016.08.007
112. Cenik B, Sephton CF, Kutluk Cenik B, Herz J, Yu G. Progranulin: a proteolytically processed protein at the crossroads of inflammation and neurodegeneration. J Biol Chem. (2012) 287:32298–306. doi: 10.1074/jbc.R112.399170
113. Arechavaleta-Velasco F, Perez-Juarez CE, Gerton GL, Diaz-Cueto L. Progranulin and its biological effects in cancer. Med Oncol. (2017) 34:194. doi: 10.1007/s12032-017-1054-7
114. Körtvelyessy P, Prüss H, Thurner L, Maetzler W, Vittore-Welliong D, Schultze-Amberger J, et al. Biomarkers of neurodegeneration in autoimmune-mediated encephalitis. Front Neurol. (2018) 9:668. doi: 10.3389/fneur.2018.00668
115. Nissen MS, Ryding M, Nilsson AC, Madsen JS, Olsen DA, Halekoh U, et al. CSF-neurofilament light chain levels in NMDAR and LGI1 encephalitis: a national cohort study. Front Immunol. (2021) 12:719432. doi: 10.3389/fimmu.2021.719432
116. Macher S, Zrzavy T, Höftberger R, Altmann P, Pataraia E, Zimprich F, et al. Longitudinal measurement of cerebrospinal fluid neurofilament light in anti-N-methyl-D-aspartate receptor encephalitis. Eur J Neurol. (2021) 28:1401–5. doi: 10.1111/ene.14631
117. Kammeyer R, Mizenko C, Sillau S, Richie A, Owens G, Nair KV, et al. Evaluation of plasma neurofilament light chain levels as a biomarker of neuronal injury in the active and chronic phases of autoimmune neurologic disorders. Front Neurol. (2022) 13:689975. doi: 10.3389/fneur.2022.689975
118. Guasp M, Martín-Aguilar L, Sabater L, Bioque M, Armangué T, Martínez-Hernández E, et al. Neurofilament light chain levels in anti-NMDAR encephalitis and primary psychiatric psychosis. Neurology. (2022) 98:e1489–98. doi: 10.1212/WNL.0000000000200021
119. Middeldorp J, Hol EM. GFAP in health and disease. Prog Neurobiol. (2011) 421–43. doi: 10.1016/j.pneurobio.2011.01.005
120. Blennow K, Hampel H, Weiner M. Zetterberg H. Cerebrospinal fluid and plasma biomarkers in Alzheimer disease. Nat Rev Neurol. (2010) 6:131–44. doi: 10.1038/nrneurol.2010.4
121. Sabater L, Gaig C, Gelpi E, Bataller L, Lewerenz J, Torres-Vega E, et al. A novel non-rapid-eye movement and rapid-eye-movement parasomnia with sleep breathing disorder associated with antibodies to IgLON5: a case series, characterisation of the antigen, and post-mortem study. Lancet Neurol. (2014) 13:575–86. doi: 10.1016/S1474-4422(14)70051-1
122. Sinclair AJ, Wienholt L, Tantsis E, Brilot F, Dale RC. Clinical association of intrathecal and mirrored oligoclonal bands in paediatric neurology. Dev Med Child Neurol. (2013) 55:71–5. doi: 10.1111/j.1469-8749.2012.04443.x
123. Huda S, Wong SH, Pettingill P, O'Connell D, Vincent A, Steiger M, et al. An 11-year retrospective experience of antibodies against the voltage-gated potassium channel (VGKC) complex from a tertiary neurological centre. J Neurol. (2015) 262:418–24. doi: 10.1007/s00415-014-7588-0
124. Gastaldi M, Arbasino C, Dallocchio C, Diamanti L, Bini P, Marchioni E, et al. NMDAR encephalitis presenting as akinesia in a patient with Parkinson disease. J Neuroimmunol. (2019) 328:35–7. doi: 10.1016/j.jneuroim.2018.12.002
125. DeFelipe-Mimbrera A, Masjuan J, Corral Í, Villar LM, Graus F, García-Barragán N, et al. Opsoclonus-myoclonus syndrome and limbic encephalitis associated with GABAB receptor antibodies in CSF. J Neuroimmunol. (2014) 272:91–3. doi: 10.1016/j.jneuroim.2014.04.009
126. Wijntjes J, Bechakra M, Schreurs MWJ, Jongen JLM, Koppenaal A, Titulaer MJ, et al. Pruritus in anti-DPPX encephalitis. Neurol Neuroimmunol Neuroinflamm. (2018) 5:e455. doi: 10.1212/NXI.0000000000000455
127. Morales-Briceño H, Cruse B, Fois AF, Lin MW, Jiang J, Banerjee D, et al. IgLON5-mediated neurodegeneration is a differential diagnosis of CNS Whipple disease. Neurology. (2018) 90:1113–5. doi: 10.1212/WNL.0000000000005679
128. Simabukuro MM, Sabater L, Adoni T, Cury RG, Haddad MS, Moreira CH, et al. Sleep disorder, chorea, and dementia associated with IgLON5 antibodies. Neurol Neuroimmunol Neuroinflamm. (2015) 2:e136. doi: 10.1212/NXI.0000000000000136
129. Irani SR, Bera K, Waters P, Zuliani L, Maxwell S, Zandi MS, et al. N-methyl-D-aspartate antibody encephalitis: temporal progression of clinical and paraclinical observations in a predominantly non-paraneoplastic disorder of both sexes. Brain. (2010) 133:1655–67. doi: 10.1093/brain/awq113
130. Iorio R, Damato V, Spagni G, Della Marca G, Vollono C, Masi G, et al. Clinical characteristics and outcome of patients with autoimmune encephalitis: clues for paraneoplastic aetiology. Eur J Neurol. (2020) 27:2062–71. doi: 10.1111/ene.14325
131. Irani SR, Vincent A. Autoimmune encephalitis – new awareness, challenging questions. Discov Med. (2011) 11:449–58.
132. Vincent A, Bien CG, Irani SR, Waters P. Autoantibodies associated with diseases of the CNS: new developments and future challenges. Lancet Neurol. (2011) 10:759–72. doi: 10.1016/S1474-4422(11)70096-5
133. Brinkmalm A, Brinkmalm G, Honer WG, Frölich L, Hausner L, Minthon L, et al. SNAP-25 is a promising novel cerebrospinal fluid biomarker for synapse degeneration in Alzheimer's disease. Mol Neurodegener. (2014) 9:53. doi: 10.1186/1750-1326-9-53
134. Kester MI, Teunissen CE, Crimmins DL, Herries EM, Ladenson JH, Scheltens P, et al. Neurogranin as a cerebrospinal fluid biomarker for synaptic loss in symptomatic Alzheimer disease. JAMA Neurol. (2015) 72:1275–80. doi: 10.1001/jamaneurol.2015.1867
135. Day GS, Yarbrough MY, Körtvelyessy P, Prüss H, Bucelli RC, Fritzler MJ, et al. Prospective quantification of CSF biomarkers in antibody-mediated encephalitis. Neurology. (2021) 96:e2546–57. doi: 10.1212/WNL.0000000000011937
136. Peschl P, Bradl M, Höftberger R, Berger T, Reindl M. Oligodendrocyte glycoprotein: deciphering a target in inflammatory demyelinating diseases. Front Immunol. (2017) 8:529. doi: 10.3389/fimmu.2017.00529
137. McKay JH, Dimberg EL, Lopez Chiriboga AS. A systematic review of gamma-aminobutyric acid receptor type B autoimmunity. Neurol Neurochir Pol. (2019) 53:1–7. doi: 10.5603/PJNNS.a2018.0005
138. Dalmau J, Lancaster E, Martinez-Hernandez E, Rosenfeld MR, Balice-Gordon R. Clinical experience and laboratory investigations in patients with anti-NMDAR encephalitis. Lancet Neurol. (2011) 10:63–74. doi: 10.1016/S1474-4422(10)70253-2
139. Dalmau J, Rosenfeld MR. Autoimmune encephalitis update. Neuro Oncol. (2014) 16:771–8. doi: 10.1093/neuonc/nou030
140. Vollmer TL, McCarthy M. Autoimmune encephalitis: a more treatable tragedy if diagnosed early. Neurology. (2016) 86:1655–6. doi: 10.1212/WNL.0000000000002641
141. McKeon A. The importance of early and sustained treatment of a common autoimmune encephalitis. Lancet Neurol. (2013) 12:123–5. doi: 10.1016/S1474-4422(12)70319-8
142. Lim JA, Lee ST, Moon J, Jun JS, Park BS, Byun JI, et al. New feasible treatment for refractory autoimmune encephalitis: low-dose interleukin-2. J Neuroimmunol. (2016) 299:107–11. doi: 10.1016/j.jneuroim.2016.09.001
143. Dale RC. Interleukin-6 blockade as rescue therapy in autoimmune encephalitis. Neurotherapeutics. (2016) 13:821–3. doi: 10.1007/s13311-016-0471-1
144. Lee WJ, Lee ST, Moon J, Sunwoo JS, Byun JI, Lim JA, et al. Tocilizumab in autoimmune encephalitis refractory to rituximab: an institutional cohort study. Neurotherapeutics. (2016) 13:824–32. doi: 10.1007/s13311-016-0442-6
145. Serada S, Fujimoto M, Mihara M, Koike N, Ohsugi Y, Nomura S, et al. IL-6 blockade inhibits the induction of myelin antigen-specific Th17 cells and Th1 cells in experimental autoimmune encephalomyelitis. Proc Natl Acad Sci U S A. (2008) 105:9041–6. doi: 10.1073/pnas.0802218105
146. Mease PJ, McInnes IB, Kirkham B, Kavanaugh A, Rahman P, van der Heijde D, et al. Secukinumab inhibition of interleukin-17A in patients with psoriatic arthritis. N Engl J Med. (2015) 373:1329–39. doi: 10.1056/NEJMoa1412679
147. Wasilewska A, Winiarska M, Olszewska M, Rudnicka L. Interleukin-17 inhibitors A new era in treatment of psoriasis and other skin diseases. Postepy Dermatol Alergol. (2016) 33:247–52. doi: 10.5114/ada.2016.61599
148. Frieder J, Kivelevitch D, Menter A. Secukinumab: a review of the anti-IL-17A biologic for the treatment of psoriasis. Ther Adv Chronic Dis. (2018) 9:5–21. doi: 10.1177/2040622317738910
149. Paoletti P, Bellone C, Zhou Q. NMDA receptor subunit diversity: impact on receptor properties, synaptic plasticity and disease. Nat Rev Neurosci. (2013) 14:383–400. doi: 10.1038/nrn3504
Keywords: Autoimmune encephalitis (AE), biomarker, cerebrospinal fluid, cytokines/chemokines, demyelination, nerve damage
Citation: Zhang S, Mao C, Li X, Miao W and Teng J (2022) Advances in Potential Cerebrospinal Fluid Biomarkers for Autoimmune Encephalitis: A Review. Front. Neurol. 13:746653. doi: 10.3389/fneur.2022.746653
Received: 24 July 2021; Accepted: 20 June 2022;
Published: 22 July 2022.
Edited by:
Ming Jin Lim, Guy's and St Thomas' NHS Foundation Trust, United KingdomReviewed by:
Erdem Tüzün, Istanbul University, TurkeyNiels Hansen, University Medical Center Goettingen, Germany
Amanda Piquet, University of Colorado Anschutz Medical Campus, United States
Copyright © 2022 Zhang, Mao, Li, Miao and Teng. This is an open-access article distributed under the terms of the Creative Commons Attribution License (CC BY). The use, distribution or reproduction in other forums is permitted, provided the original author(s) and the copyright owner(s) are credited and that the original publication in this journal is cited, in accordance with accepted academic practice. No use, distribution or reproduction is permitted which does not comply with these terms.
*Correspondence: Junfang Teng, jftengzdyfy@126.com; Wang Miao, miaowang721@126.com
†These authors have contributed equally to this work