- 1Department of Anesthesiology, Critical Care and Pain Medicine, Boston Children's Hospital, Boston, MA, United States
- 2Department of Anaesthesia, Harvard Medical School, Boston, MA, United States
- 3Divisions of Genetics and Genomics, Department of Pediatrics, Boston Children's Hospital, Boston, MA, United States
- 4Department of Paediatrics, Harvard Medical School, Boston, MA, United States
Several neurological disorders may be amenable to treatment with gene-targeting therapies such as antisense oligonucleotides (ASOs) or viral vector-based gene therapy. The US FDA has approved several of these treatments; many others are in clinical trials. Preclinical toxicity studies of ASO candidates have identified dose-dependent neurotoxicity patterns. These include degeneration of dorsal root ganglia, the cell bodies of peripheral sensory neurons. Quantitative sensory testing (QST) refers to a series of standardized mechanical and/or thermal measures that complement clinical neurologic examination in detecting sensory dysfunction. QST primarily relies on patient self-report or task performance (i.e., button-pushing). This brief report illustrates individualized pragmatic approaches to QST in non-verbal subjects receiving early phase investigational intrathecal drug therapies as a component of clinical trial safety protocols. Three children with neurodevelopmental disorders that include Neuronal Ceroid Lipofuscinosis Type 7, Ataxia-Telangiectasia, and Epilepsy of Infancy with Migrating Focal Seizures are presented. These case studies discuss individualized testing protocols, accounting for disease presentation, cognitive and motor function. We outline specific considerations for developing assessments for detecting changes in sensory processing in diverse patient groups and safety monitoring trials of early phase investigational intrathecal drug therapies. QST may complement information obtained from the standard neurologic examination, electrophysiologic studies, skin biopsies, and imaging. QST has limitations and challenges, especially in non-verbal subjects, as shown in the three cases discussed in this report. Future directions call for collaborative efforts to generate sensory datasets and share data registries in the pediatric neurology field.
Introduction
Precision medicine is an emerging area for disease prevention and treatment strategies that takes into account individual variability in genes and environment. A growing number of neurological disorders may be amenable to precision medicine using treatment with gene-targeting therapies such as antisense oligonucleotides (ASOs) or viral vector-based gene therapy. The US Food and Drug Administration (FDA) and European Medicines Agencies (EMA) have approved several of these treatments for clinical applications; many others are in clinical trials.
Recent advances in ASO drug development have resulted in a rapid transition from bench-to-bedside candidates with the FDA recently approving 12 ASO therapies between the years 2016 and 2020 alone (1). All of these therapies were optimized for treatment of orphan diseases where druggable genetic targets are present only in small-to-moderate proportions of patients. Preclinical toxicity studies of ASO candidates in rodents and non-human primates have identified dose-dependent neurotoxicity patterns. These include acute and subacute dysfunction, inflammation, and degeneration of dorsal root ganglia, the cell bodies of peripheral sensory neurons (2, 3). It is critical to ensure these therapies are safe and effective in order to translate therapies into the clinic.
Clinicians are faced with challenges in identification and measurement of drug-related neurotoxicity in patients with neurological disorders using non-invasive bedside approaches. Quantitative sensory testing (QST) refers to a series of standardized measures that complement clinical neurologic examination in detecting sensory dysfunction (4–7). QST primarily relies on patient self-report or task performance (i.e., button-pushing). Little has been described of how best to design clinical trial protocols that accommodate patients with neurological disorders who are unable to provide self-report or have reduced functional capacity. This brief report illustrates individualized pragmatic approaches to QST in non-verbal subjects undergoing treatment with novel ASOs as a component of clinical trial safety protocols.
Pharmacovigilance in Clinical Trials
Monitoring patient safety and drug toxicity during clinical trials is a critical component in drug development. Optimal safety biomarkers are quantitative and permit within-subject comparisons and group comparisons relative to age-specific norms. Developing broadly applicable safety biomarkers is challenging for patients with rare neurologic disorders. Sample sizes are small (or even N-of-1), and there is heterogeneity among different neurologic disorders or even participants with the same condition.
Quantitative Sensory Testing
Hyposensitivity (elevated sensory thresholds) occurs with sensory loss, due to injury or disease (8, 9) or temporarily by local anesthesia (10, 11) or analgesia (12, 13). Hypersensitivity (lowered sensory thresholds) can occur with many types of neuropathic pain (5, 14, 15).
QST provides insight into large myelinated Aδ and small unmyelinated C fiber function (16). Typically, a calibrated stimulus is applied to the skin in graded intensities, and the patient's perception is recorded. Stimulus modalities can be mechanical (i.e., monofilaments, pin-prick, vibratory), thermal (i.e., Peltier thermode), or electrical (17, 18). Outcome measures include stimulus detection (present/absent) and stimulus intensity (self-reported rating).
Adult QST protocols are standardized and studied extensively. A widely used protocol involves a battery of 13 non-invasive assessments, developed by the German Neuropathic Pain network (DFNS) (5). A DFNS-QST profile of two body areas can be obtained within 1-h in adults with neuropathic pain (4).
Standardized protocols permit comparison of one individual to normative values and patients with neuropathic conditions using multicenter databases (5, 6, 14, 19–23). Patient stratification according to QST profile has been proposed to identify responders in analgesic trials (24). QST has been used in over 1,000 patients with malignancies to investigate large and small fiber neuropathies associated with chemotherapy agents, including bortezomib, vincristine, taxanes, and platinum compounds (25–28).
QST Protocols in Children
Modifications to the DFNS protocol have been made for typically developing children as young as 6 years old. Reference values have been reported for age and gender (19, 22). Our research group has extensive experience in sensory profiling. We have developed QST protocols for typically-developing children (29), children and adolescents with juvenile idiopathic arthritis (30), complex regional pain syndrome (31–33), and scoliosis (34); and adult volunteers receiving novel local anesthetics in Phase-1 clinical trials (10).
For term and preterm neonates, infants, and non-verbal children, surrogate approaches to sensory evaluation have recorded stimulus-evoked behaviors (i.e., facial expressions or nocifensive leg flexion withdrawal response following cutaneous mechanical stimulation) (35–38). We recently examined longitudinal trends in nocifensive withdrawal responses among typically developing infants and infants undergoing prolonged intensive care for treatment of esophageal atresia (36).
Limited information has been reported regarding QST protocol design in non-verbal children. From PubMed search, we could not identify publications describing protocols for sensory assessments during drug trials for children with communicative, cognitive, and motor constraints.
Case Examples
The examples below illustrate individualized testing protocols, accounting for disease presentation, cognitive and motor function for three children receiving spinal administration of a personalized ASO in an N-of-1 protocol (3). These patients have medically complex congenital neurodevelopmental disorders that present with varying degrees of neurodegeneration and marked functional impairment. To provide the reader with context, children with medical complexity often require substantial medical care, specialized therapy and educational support, and have varying severity of functional limitations that require tracheostomy, feeding tube or a wheelchair (39, 40).
The importance of monitoring sensory function and performing QST in these patients was motivated in part by preclinical spinal toxicology studies. These preclinical studies showed a safe behavioral and neuropathologic profile in the dose ranges chosen (based on scaling models) for clinical administration (3, 41, 42). At higher doses, animals showed varying degrees of dorsal root ganglion inflammation and nerve injury (3). For all cases, QST was performed to identify potential signs of drug-associated nerve injury, i.e., loss of response to tactile stimulation as a result of nerve damage; the results of QST remained stable throughout treatment.
Methods Overview
Lower extremities were tested because animal studies show more behavioral and histologic signs of toxicity around lumbar than thoracic or cervical spinal levels. Baseline assessments before treatment, and repeated tests pre- and post-drug administration were performed. The duration of treatments ranged from 12 to 36 months.
The primary outcome measure was the lowest mechanical stimulus intensity required to evoke nocifensive behavior (Case 1; Case 3) or verbal and non-verbal cues (Case 2) when applied to the foot. In all cases caregivers were present during the assessments and they engaged with the physiologist in order to help relax the patient or providing feedback about responses as needed.
All studies were conducted with local ethics approval and written informed parent consent.
6-Year-Old Girl with Neuronal Ceroid Lipofuscinosis Type 7
Patient 1 had typical neurodevelopment through infancy. By age 3, she began to develop a progressive decline of cognitive and motor function, blindness, seizures, and spasticity. At age 6, whole genome sequencing led to a diagnosis of neuronal ceroid lipofuscinosis Type 7 (3). At evaluation, the patient was non-verbal with significant developmental delay and was unable to walk unassisted. Testing with the use of Vineland Adaptive Behavior Scales (Vineland-II) showed low scores for Communication, Daily Living Skills, Socialization and Motor Skill domains, with declines in 7 of 11 neurologic and neuropsychological subscores at baseline. Gross Motor Function Measure-88 scores were 0 on the “Crawling and Kneeling,” “Standing,” and “Walking, Running and Jumping” domains at baseline.
• Means of communication: Patient 1 interacted with the world by shifting attention (i.e., becoming quiet and still while listening). She demonstrated frustration through making fussy vocalizations and becoming more active.
• Study optimization: We opted to stimulate the left side because the patient had a broader range of motion, making leg flexion withdrawal feasible. Musical distraction techniques (familiar movie soundtrack) and parent interaction (soothing singing) were used while performing the test to help induce relaxation and reduce spurious leg movement. The patient was assessed while awake for all study time-points.
• Interpretation: Figure 1A shows baseline sensory threshold data collected across the duration of ASO drug treatment. Sensory thresholds remained stable throughout. The study site was relocated to a second site part-way through the study. Sensory threshold data collected at study site 1 and site 2 (by site-specific sensory physiologists) showed no clinical or statistically significant differences. Collectively, these data suggest reliability of the test across time and testing locations.
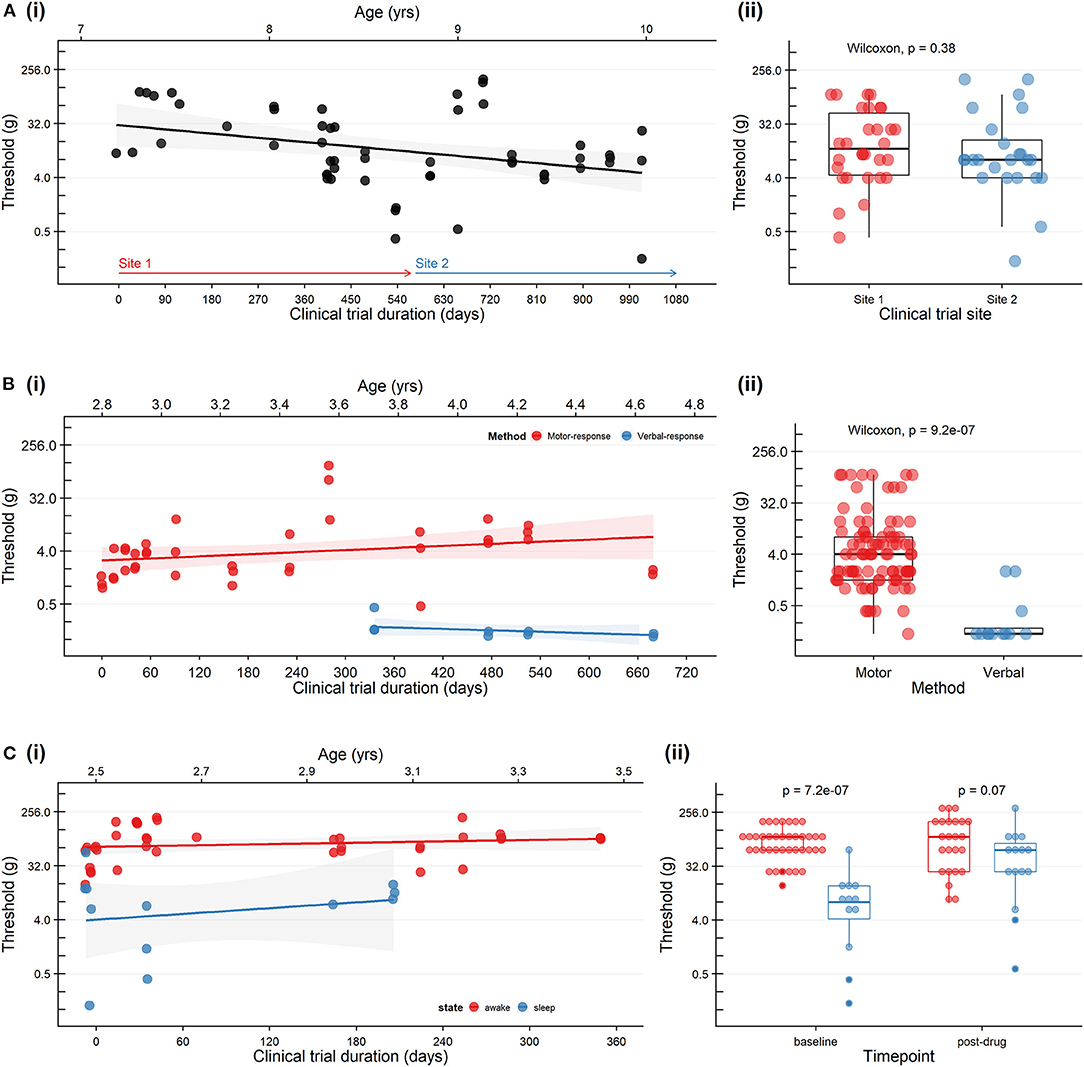
Figure 1. Trends in cutaneous mechanical threshold in three patients with neurodegenerative disorders receiving a personalized investigational intrathecal ASO. (A) A patient with Neuronal Ceroid Lipofuscinosis Type 7 who was studied at two study sites. (i) Baseline sensory thresholds recorded on the day of drug dosing (prior to drug administration). Study site 1 (red arrow) was relocated to a second site (blue arrow) on Day 603. (ii) Baseline sensory threshold data collected at site 1 and site 2 (by site-specific sensory physiologists) showing no clinical or statistically significant differences (Wilcoxon). (B) A patient with Ataxia-Telangiectasia during developmental progression from preverbal to verbal status. Leg flexion withdrawal or feet movement was used consistently over 10 months of the study (motor response: red). At age 3.8 years the patient was able to provide verbal responses as the primary outcome measure (verbal response: blue) and therefore we modifed the protocol to improve engagement and responses. (i) Baseline sensory thresholds recorded on the day of drug dosing (prior to drug administration). (ii) Differences between sensory threshold with verbal and motor responses (Wilcoxon). (C) A patient with Epilepsy of Infancy with Migrating Focal Seizures who exhibited considerable state-dependent variability in the sensory threshold. (i) Baseline sensory thresholds were recorded on the day of drug administration (prior to drug administration) (wakefulness: red; sleep: blue). (ii) Sensory threshold data showing lower sensitivity to mechanical stimulation during wakefulness (red) compared to sleep (blue) at baseline (Wilcoxon). Pre-dose and post-dose sensory thresholds are included in the plot; no statistical difference in pre-drug vs. post-drug sensory thresholds (Wilcoxon, p = 0.53). Sensory thresholds were established by applying von Frey hair monofilaments to the plantar surface of the foot with increasing stimulus intensity. For all plots: each dot represents an individual trial; the scatter plots with a black line and shaded area represent a linear regression fit, and 95% confidence boundaries describing the relationship between time and threshold, and boxplots represent min, max, median, 25th, and 75th percentile.
3-Year-Old Girl with Ataxia-Telangiectasia
Patient 2 underwent newborn screening, which showed a risk for severe combined immunodeficiency. At 7-months, she was diagnosed with ataxia-telangiectasia. At evaluation, at age 2.8 years, she presented with delays in speech and adaptive skills, and mild ataxia. Testing with the use of Vineland Adaptive Behavior Scales (Vineland-III) showed moderately low scores (3rd percentile) at baseline indicating that the patient was behind peers in development. Peabody Developmental Motor Scale (PDMS-2) scores were low for locomotion (2nd percentile), stationary motor (9th percentile) and object manipulation (9th percentile) at baseline.
• Means of communication: Patient 2 used non-verbal communication to indicate “yes” and “no,” vocalized to identify objects, and signs and gestures to indicate distress and frustration.
• Study optimization: The challenges faced were in ensuring stimulus-response specificity and patient engagement. We opted to (1) perform multiple trains of stimuli by applying each stimulus three times in three groups and documenting a positive response when 2/3 groups elicited a response; (2) alternate stimulus application location across the feet, so stimuli were “unexpected” (increasing attention and preventing habituation); (3) utilize relaxation techniques (play or eating); (4) protocol adaptations accordingly with developmental gains Verbal and non-verbal cues were considered positive responses, e.g., immediate changes in attention, leg withdrawal. The patient was assessed while awake for all study time-points.
• Interpretation: Figure 1B shows thresholds over time. Note that over the study time period, at age 3.8 years, verbal abilities improved, and she was converted from behavioral observation to adaptive self-report. Baseline sensory thresholds recorded on the day of drug dosing were consistent over time. As expected, the use of verbal-report as a means to communicate sensation provided a more sensitive compared to behavioral observation; both measures were stable over time.
18-Month-Old Girl with Epilepsy of Infancy with Migrating Focal Seizures
Patient 3 developed unrelenting seizures from day 4 of life onward. At age 4-months, she was diagnosed with a potassium channel mutation causing epilepsy of infancy with migrating focal seizures. At evaluation, the patient was preverbal with significant developmental delay and presented multiple seizures a day. Vineland Adaptive Behavior Scales (Villand-III) scores were low (<1st percentile) in all domains and indicated profound developmental delays across all domains at baseline. Motor evaluation showed severe gross hypotonia thoughout with minimal to no head control appreciated in any positioning; she required assistance for all mobility.
• Means of communication: Patient 3 had difficulty engaging in fundamental skills for attending to her environment and eliciting parent support for her needs. Parents were able to report behavious that suggested patient was hungry (i.e., lip smacking) or unsettled (i.e., groaning, startle-response).
• Study optimization: Being of toddler-age, the patient was often napping during the time window allocated for baseline data collection (i.e., hours prior to drug administration). We opted to take measurements when possible during both wakefulness and sleep states to ensure the measures were not floored.
• Interpretation: Sensory thresholds were stable over time but considerably lower during natural sleep compared to awake state (Figure 1C). Although her threshold during while sleep are lower they were consistent over time.
Discussion
Pros and Cons of Sensory Testing: Feasibility and Reliability
Various types of assessments can be performed to understand neurophysiologic function including QST (measurement of sensory thresholds), electrophysiologic testing (nerve fiber excitability), and recording of cortical sensory-evoked potentials. Here we have outlined individualized pragmatic approaches to QST in non-verbal research-participants undergoing treatment with novel ASOs as a component of clinical trial safety protocols. We have attempted to identify the most reliable sensory testing parameters given the patient age, state, disease presentation and cognitive ability. There are several advantages to our approach for use in these challenging patient populations.
One of the biggest challenges when working with pre/non-verbal populations lies in ensuring reliability of testing. The real-world data provided in this paper exemplifies how factors such as age and state can influence variability outcomes. We note that there is very little data available in this field, and as such, have largely drawn from our own experiences in this field. Where concerns over reliability of testing arise, it may be appropriate to complement sensory evaluation with more direct measures of nerve function. Alternative approaches include nerve conduction, electromyography, and cortical sensory-evoked potentials.
Electrophysiologic studies including nerve conduction velocity (NCV) and electromyography (EMG) have well-established interpretations in evaluation of peripheral nerve functioning. Conduction velocity in the upper extremities and lower extremities (ulnar, sural nerve stimulation) can be evaluated. Recording from one muscle supplied by the nerve distal to the site of stimulation is performed using surface electrodes, and/or a needle electrode. Slowing of nerve conduction velocity and reductions in amplitude usually indicate the presence of lesions affecting the axon of the peripheral nerve or loss of axons, respectively (43). Importantly, while NVC/EMG involves evaluation of nerve conduction in the largest and fastest myelinated fibers, it is insensitive to Að* and C-fiber dysfunction. Following proximal nerve injury or dysfunction, there is a time lag of several days before early changes are observed in NCV/EMG.
Cortical sensory-evoked potentials may provide additional objective information. Recording electrodes are placed over the scalp, and cutaneous stimuli are delivered. Cortical responses are analyzed in a similar approach to NCV/EMG. Laser, contact-heat, and intraepidermal electrical stimulation are the three main types of stimuli that can be used reliably. Thermal modalities primarily investigate small-diameter Að* fiber dysfunction (rather than C-fibers), and the central lesions involving the spinothalamic tract (44). Intraepidermal electrical stimulation preferentially activates large-diameter AB fibers rather than small-diameter Að* or C fibers (44). Both laser and contact-heat stimuli are associated with risk of burning at the cutaneous site of stimulation. As with NCV/EMG, these methods can cause discomfort so it is important that children have a sense of what to expect, and to implement these approaches on an as needs basis rather than for each time-point (which could be between 2 and 4+ times over a 24 h study period).
Pros and Cons of Sensory Testing: Sensory and Motor Function Degradation
Challenges faced when designing a sensory testing study in a neurologically challenged population is in adapting to disease progression. Many genetic neurologic disorders are associated with neuropathies that may progress over time. Here, we opt to utilize the flexion withdrawal reflex as an outcome measure because of its primitive nature. However, for some patients, spasticity may develop and make it difficult to identify what is evoked vs. spontaneous limb movement. In this scenario, we recommend applying multiple stimuli and testing on multiple occasions.
We additionally provide a protocol that aims to generate objective and quantitative data. In pre- or non-verbal populations, experienced care givers may be used as a proxy for self-report although this approach is confounded by many challenges and biases. For example, patients may exhibit pain behaviors when they do not have pain and make it difficult to discern signs of pain, or observers may overestimate pain when unblinded to the application of a painful stimulus (45). However, when meeting the families for the first time we do ask parents/caregivers to provide us with insight of how patient communicate discomfort and we use these cues when assessing responses.
We show that our protocol can be used as a paragmatic approach to evaluate patient safety and drug toxicity during clinical trials were there is an potential risk for spinal toxicity. We additionally highlight the importance of interpreting the sensory testing results with other clinical examination assessments such as those performed by a neurologist and the physical therapist.
Pragmatic Testing Considerations
We propose provisional recommendations for performing QST in children with neurologic disorders receiving early phase investigational intrathecal drug therapies based on our initial experience with N-of-1 trials.
General Protocol Composition
• Protocol design must balance obtaining necessary information while limiting session duration and exposure to noxious stimuli; e.g., adults might complete a QST battery lasting 1-h, whereas 5-year-olds might only tolerate a mechanical detection paradigm lasting 15-min.
• Documentation of experimental variables, e.g., patient positioning, sleep state, and distraction technique (Supplementary Figure 1).
• Documentation of degree of developmental disability across language, cognitive and motor domains (as appropriate).
Cognitive Level (Ability to Understand the Test)
• For neonates, young infants, or older children with developmental delay or neurologic regression who cannot provide self-report, mechanically evoked leg flexion withdrawal responses offer an indirect measure of sensory responsiveness. The outcome measure is the lowest mechanical stimulus intensity required to produce a response.
• Age-varying changes in mechanical threshold must be considered. The threshold required to evoke the reflex rapidly increases during the first 2 months of life and then changes very little up the first year (35, 36, 38).
Motor Function (Ability to Move in Response to a Stimulus)
• Patients with adequate understanding of the test but limited ability to verbalize the responses can be aided with alternative response cues, e.g., clicking a button or eye-blinking.
• Patients with movement disorders (e.g., spasticity or dystonia) presenting with stiff or restless legs make it difficult to evoke withdrawal responses. Optimizing positioning and distraction techniques facilitate relaxation and cooperation.
Cooperation (Ability to Withstand Multiple Repetitive Stimuli)
• Attention span and concentration affect the quality of the results by increasing data variability. Cooperation is enhanced in a quiet, calming environment and engaging through play or distraction (e.g., parental engagement or watching TV).
Baseline Measures
We recommend performing:
• A series of baseline evaluations on successive days that reflect changes in a patient's state and acknowledges that a degree of learned behavior will occur with longitudinal studies.
• Quality assurance tests where multiple assessors or study sites are involved.
Conclusions
We have outlined specific considerations for developing individualized assessments for detecting changes in sensory processing in diverse patient groups and safety monitoring in N-of-1 trials of novel medications (Table 1). QST (either using self-report or nocifensive limb movements) is convenient for repeated bedside measurements. Changes due to mechanical injury, neurotoxicity, or reversible drug effect can be detected immediately, without the time delay of NCV/EMG. QST may therefore complement information obtained from the standard neurologic examination, electrophysiologic studies, skin biopsies, and imaging. QST has limitations and challenges, especially in non-verbal participants, as shown in the three cases discussed above. Future directions call for collaborative efforts to generate sensory datasets and share data registries in the pediatric field.
Data Availability Statement
The raw data supporting the conclusions of this article will be made available by the authors, without undue reservation.
Ethics Statement
The studies involving human participants were reviewed and approved by Boston Children's Hospital Institutional Review Board. Written informed consent to participate in this study was provided by the participants' legal guardian/next of kin. Written informed consent was obtained from the minor(s)' legal guardian/next of kin for the publication of any potentially identifiable images or data included in this article.
Author Contributions
CB, LC, and CD conceptualized and wrote the manuscript. LC, CD, CB, and TY critically revised the manuscript. All authors contributed to the article and approved the submitted version.
Funding
This work is supported by the Sara Page Mayo Endowment for Pediatric Pain Research and Treatment, and by the Cardiovascular & Critical Care Cluster of Clinical Research Excellence at Boston Children's Hospital.
Conflict of Interest
The authors declare that the research was conducted in the absence of any commercial or financial relationships that could be construed as a potential conflict of interest.
Publisher's Note
All claims expressed in this article are solely those of the authors and do not necessarily represent those of their affiliated organizations, or those of the publisher, the editors and the reviewers. Any product that may be evaluated in this article, or claim that may be made by its manufacturer, is not guaranteed or endorsed by the publisher.
Supplementary Material
The Supplementary Material for this article can be found online at: https://www.frontiersin.org/articles/10.3389/fneur.2022.664710/full#supplementary-material
References
1. Dhuri K, Bechtold C, Quijano E, Pham H, Gupta A, Vikram A, et al. Antisense oligonucleotides: an emerging area in drug discovery and development. J Clin Med. (2020) 9:2004. doi: 10.3390/jcm9062004
2. Frazier KS. Antisense oligonucleotide therapies: the promise and the challenges from a toxicologic pathologist's perspective. Toxicol Pathol. (2015) 43:78–89. doi: 10.1177/0192623314551840
3. Kim J, Hu C, Moufawad El Achkar C, Black LE, Douville J, Larson A, et al. Patient-customized oligonucleotide therapy for a rare genetic disease. N Engl J Med. (2019) 381:1644–52. doi: 10.1056/NEJMoa1813279
4. Rolke R, Magerl W, Campbell KA, Schalber C, Caspari S, Birklein F, et al. Quantitative sensory testing: a comprehensive protocol for clinical trials. Eur J Pain. (2006) 10:77–88. doi: 10.1016/j.ejpain.2005.02.003
5. Rolke R, Baron R, Maier C, Tölle TR, Treede R-D, Beyer A, et al. Quantitative sensory testing in the German research network on neuropathic pain (DFNS): standardized protocol and reference values. Pain. (2006) 123:231–43. doi: 10.1016/j.pain.2006.01.041
6. Smith SM, Dworkin RH, Turk DC, Baron R, Polydefkis M, Tracey I, et al. The potential role of sensory testing, skin biopsy, and functional brain imaging as biomarkers in chronic pain clinical trials: IMMPACT considerations. J Pain. (2017) 18:757–77. doi: 10.1016/j.jpain.2017.02.429
7. Koulouris AE, Edwards RR, Dorado K, Schreiber KL, Lazaridou A, Rajan S, et al. Reliability and validity of the boston bedside quantitative sensory testing battery for neuropathic pain. Pain Med. (2020) 21:2336–47. doi: 10.1093/pm/pnaa192
8. Rolke R, Rolke S, Vogt T, Birklein F, Geber C, Treede R-D, et al. Hand-arm vibration syndrome: clinical characteristics, conventional electrophysiology and quantitative sensory testing. Clin Neurophysiol. (2013) 124:1680–8. doi: 10.1016/j.clinph.2013.01.025
9. Münster T, Eckl S, Leis S, Göhring-Waldeck G, Ihmsen H, Maihöfner C. Characterization of somatosensory profiles in patients with Crohn's disease. Pain Pract. (2015) 15:265–71. doi: 10.1111/papr.12182
10. Lobo K, Donado C, Cornelissen L, Kim J, Ortiz R, Peake RWA, et al. A phase 1, dose-escalation, double-blind, block-randomized, controlled trial of safety and efficacy of neosaxitoxin alone and in combination with 0.2% bupivacaine, with and without epinephrine, for cutaneous Anesthesia. Anesthesiology. (2015) 123:873–85. doi: 10.1097/ALN.0000000000000831
11. Garibyan L, Cornelissen L, Sipprell W, Pruessner J, Elmariah S, Luo T, et al. Transient alterations of cutaneous sensory nerve function by noninvasive cryolipolysis. J Invest Dermatol. (2015) 135:2623–31. doi: 10.1038/jid.2015.233
12. Siegenthaler A, Schliessbach J, Vuilleumier PH, Juni P, Zeilhofer HU, Arendt-Nielsen L, et al. Linking altered central pain processing and genetic polymorphism to drug efficacy in chronic low back pain. BMC Pharmacol Toxicol. (2015) 16:23. doi: 10.1186/s40360-015-0023-z
13. Schliessbach J, Siegenthaler A, Bütikofer L, Vuilleumier P, Jüni P, Stamer U, et al. Predicting drug efficacy in chronic low back pain by quantitative sensory tests. Eur J Pain. (2018) 22:973–88. doi: 10.1002/ejp.1183
14. Maier C, Baron R, Tölle TR, Binder A, Birbaumer N, Birklein F, et al. Quantitative sensory testing in the German research network on neuropathic pain (DFNS): somatosensory abnormalities in 1236 patients with different neuropathic pain syndromes. Pain. (2010) 150:439–50. doi: 10.1016/j.pain.2010.05.002
15. Vollert J, Attal N, Baron R, Freynhagen R, Haanpää M, Hansson P, et al. Quantitative sensory testing using DFNS protocol in Europe: an evaluation of heterogeneity across multiple centers in patients with peripheral neuropathic pain and healthy subjects. Pain. (2016) 157:750–8. doi: 10.1097/j.pain.0000000000000433
16. Beissner F, Brandau A, Henke C, Felden L, Baumgärtner U, Treede R-D, et al. Quick discrimination of adelta and C fiber mediated pain based on three verbal descriptors. PLoS ONE. (2010) 5:e12944. doi: 10.1371/journal.pone.0012944
17. Savic G, Frankel HL, Jamous MA, Jones PW, King NKK. Sensitivity to change of the cutaneous electrical perceptual threshold test in longitudinal monitoring of spinal cord injury. Spinal Cord. (2011) 49:439–44. doi: 10.1038/sc.2010.123
18. Hughes SW, Basra M, Chan C, Parr C, Wong F, Gomez S, et al. Capsaicin-induced changes in electrical pain perception threshold can be used to assess the magnitude of secondary hyperalgesia in humans. Pain Med. (2020) 21:2830–8. doi: 10.1093/pm/pnaa082
19. Blankenburg M, Boekens H, Hechler T, Maier C, Krumova E, Scherens A, et al. Reference values for quantitative sensory testing in children and adolescents: developmental and gender differences of somatosensory perception. Pain. (2010) 149:76–88. doi: 10.1016/j.pain.2010.01.011
20. Pfau DB, Krumova EK, Treede R-D, Baron R, Toelle T, Birklein F, et al. Quantitative sensory testing in the German research network on neuropathic pain (DFNS): reference data for the trunk and application in patients with chronic postherpetic neuralgia. PAIN. (2014) 155:1002–15. doi: 10.1016/j.pain.2014.02.004
21. González-Duarte A, Lem-Carrillo M, Guerrero-Torres L. Normative values of quantitative sensory testing in Hispanic Latino population. Brain Behav. (2016) 6:e00466. doi: 10.1002/brb3.466
22. van den Bosch GE, van Dijk M, Tibboel D, Valkenburg AJ. Thermal quantitative sensory testing in healthy Dutch children and adolescents standardized test paradigm and Dutch reference values. BMC Pediatr. (2017) 17:77. doi: 10.1186/s12887-017-0827-7
23. Neziri AY, Scaramozzino P, Andersen OK, Dickenson AH, Arendt-Nielsen L, Curatolo M. Reference values of mechanical and thermal pain tests in a pain-free population. Eur J Pain. (2011) 15:376–83. doi: 10.1016/j.ejpain.2010.08.011
24. Reimer M, Forstenpointner J, Hartmann A, Otto JC, Vollert J, Gierthmühlen J, et al. Sensory bedside testing: a simple stratification approach for sensory phenotyping. Pain Rep. (2020) 5:e820. doi: 10.1097/PR9.0000000000000820
25. Timmins HC, Li T, Kiernan MC, Horvath LG, Goldstein D, Park SB. Quantification of small fiber neuropathy in chemotherapy-treated patients. J Pain. (2020) 21:44–58. doi: 10.1016/j.jpain.2019.06.011
26. Dougherty PM, Cata JP, Burton AW, Vu K, Weng H-R. Dysfunction in multiple primary afferent fiber subtypes revealed by quantitative sensory testing in patients with chronic vincristine-induced pain. J Pain Symptom Manage. (2007) 33:166–79. doi: 10.1016/j.jpainsymman.2006.08.006
27. Boyette-Davis JA, Cata JP, Zhang H, Driver LC, Wendelschafer-Crabb G, Kennedy WR, et al. Follow-up psychophysical studies in bortezomib-related chemoneuropathy patients. J Pain. (2011) 12:1017–24. doi: 10.1016/j.jpain.2011.04.008
28. Cata JP, Weng H-R, Burton AW, Villareal H, Giralt S, Dougherty PM. Quantitative sensory findings in patients with bortezomib-induced pain. J Pain. (2007) 8:296–306. doi: 10.1016/j.jpain.2006.09.014
29. Meier PM, Berde CB, DiCanzio J, Zurakowski D, Sethna NF. Quantitative assessment of cutaneous thermal and vibration sensation and thermal pain detection thresholds in healthy children and adolescents. Muscle Nerve. (2001) 24:1339–45. doi: 10.1002/mus.1153
30. Cornelissen L, Donado C, Kim J, Chiel L, Zurakowski D, Logan DE, et al. Pain hypersensitivity in juvenile idiopathic arthritis: a quantitative sensory testing study. Pediatr Rheumatol Online J. (2014) 12:39. doi: 10.1186/1546-0096-12-39
31. Sethna NF, Meier PM, Zurakowski D, Berde CB. Cutaneous sensory abnormalities in children and adolescents with complex regional pain syndromes. Pain. (2007) 131:153–61. doi: 10.1016/j.pain.2006.12.028
32. Meier PM, Zurakowski D, Berde CB, Sethna NF. Lumbar sympathetic blockade in children with complex regional pain syndromes: a double blind placebo-controlled crossover trial. Anesthesiology. (2009) 111:372–80. doi: 10.1097/ALN.0b013e3181aaea90
33. Lebel A, Becerra L, Wallin D, Moulton EA, Morris S, Pendse G, et al. fMRI reveals distinct CNS processing during symptomatic and recovered complex regional pain syndrome in children. Brain. (2008) 131:1854–79. doi: 10.1093/brain/awn123
34. O'Neill N, Lins L, Hedequist D, Hresko T, Emans J, Karline L, et al. Quantitative sensory changes following posterior spinal fusion in adolescent idiopathic scoliosis. Pediatrics (2021) 147:804–5. doi: 10.1542/peds.147.3_MeetingAbstract.804
35. Cornelissen L, Fabrizi L, Patten D, Worley A, Meek J, Boyd S, et al. Postnatal temporal, spatial and modality tuning of nociceptive cutaneous flexion reflexes in human infants. PLoS ONE. (2013) 8:e76470. doi: 10.1371/journal.pone.0076470
36. Underwood E, Gabard-Durnam L, Tao A, Hensch T, Nelson C, Berde C, et al. Development of Nociceptive & Tactile Spinal Sensory Circuits During the First Year of Life in Human Infants. Amsterdam: Rescheduled to virtual presentation (2020).
37. Abdulkader HM, Freer Y, Fleetwood-Walker SM, McIntosh N. Bodily progression of motor responses to increasing mechanical force stimulation in the newborn infant and the effect of heel prick. Neonatology. (2008) 94:38–44. doi: 10.1159/000112948
38. Andrews K, Fitzgerald M. Cutaneous flexion reflex in human neonates: a quantitative study of threshold and stimulus-response characteristics after single and repeated stimuli. Dev Med Child Neurol. (1999) 41:696–703. doi: 10.1017/S0012162299001425
39. Cohen E, Kuo DZ, Agrawal R, Berry JG, Bhagat SKM, Simon TD, et al. Children with medical complexity: an emerging population for clinical and research initiatives. Pediatrics. (2011) 127:529–38. doi: 10.1542/peds.2010-0910
40. Berry JG, Agrawal R, Kuo DZ, Cohen E, Risko W, Hall M, et al. Characteristics of hospitalizations for patients who use a structured clinical care program for children with medical complexity. J Pediatr. (2011) 159:284–90. doi: 10.1016/j.jpeds.2011.02.002
41. Rigo F, Chun SJ, Norris DA, Hung G, Lee S, Matson J, et al. Pharmacology of a central nervous system delivered 2'-O-methoxyethyl-modified survival of motor neuron splicing oligonucleotide in mice and nonhuman primates. J Pharmacol Exp Ther. (2014) 350:46–55. doi: 10.1124/jpet.113.212407
42. Passini MA, Bu J, Richards AM, Kinnecom C, Sardi SP, Stanek LM, et al. Antisense oligonucleotides delivered to the mouse CNS ameliorate symptoms of severe spinal muscular atrophy. Sci Transl Med. (2011) 3:72ra18. doi: 10.1126/scitranslmed.3001777
43. Mallik A, Weir AI. Nerve conduction studies: essentials and pitfalls in practice. BMJ. (2005) 76(Suppl. 2):ii23–31. doi: 10.1136/jnnp.2005.069138
44. Lefaucheur J-P. Clinical neurophysiology of pain. In: Levin KH, Chauvel P, editors. Handbook of Clinical Neurology, Vol. 161. Elsevier (2019). p. 121–48. doi: 10.1016/B978-0-444-64142-7.00045-X
Keywords: pain measurement, antisense oligonucleotides, neurodevelopmental disorders, clinical trials, quantitative sensory testing, patient safety, personalized medicine, intrathecal
Citation: Cornelissen L, Donado C, Yu TW and Berde CB (2022) Modified Sensory Testing in Non-verbal Patients Receiving Novel Intrathecal Therapies for Neurological Disorders. Front. Neurol. 13:664710. doi: 10.3389/fneur.2022.664710
Received: 05 February 2021; Accepted: 14 January 2022;
Published: 10 February 2022.
Edited by:
Ali Yadollahpour, The University of Sheffield, United KingdomReviewed by:
Suellen Walker, University College London, United KingdomTim F. Oberlander, University of British Columbia, Canada
Copyright © 2022 Cornelissen, Donado, Yu and Berde. This is an open-access article distributed under the terms of the Creative Commons Attribution License (CC BY). The use, distribution or reproduction in other forums is permitted, provided the original author(s) and the copyright owner(s) are credited and that the original publication in this journal is cited, in accordance with accepted academic practice. No use, distribution or reproduction is permitted which does not comply with these terms.
*Correspondence: Laura Cornelissen, laura.cornelissen@childrens.harvard.edu; Carolina Donado, carolina.donadorincon@childrens.harvard.edu
†These authors have contributed equally to this work