- 1Department of Neurosurgery, The Third Affiliated Hospital of Wenzhou Medical University, Ruian People's Hospital, Ruian, China
- 2Department of Neurosurgery, Ningbo Branch, Ren Ji Hospital, Shanghai Jiao Tong University School of Medicine, Ningbo, China
- 3Department of Neurosurgery, Hangzhou Ninth People's Hospital, Hangzhou, China
Objective: Hypoxia-inducible factor 1alpha (HIF-1α) functions as a crucial transcriptional mediator in hypoxic and ischemic brain response. We endeavored to assess the prognostic significance of serum HIF-1α in human aneurysmal subarachnoid hemorrhage (aSAH).
Methods: In this prospective, longitudinal, multicenter, and observational study of 257 patients with aSAH and 100 healthy controls, serum HIF-1α levels were quantified. Univariate analyses, followed by multivariate analyses, were performed to discern the relationship between serum HIF-1α levels and severity and delayed cerebral ischemia (DCI) plus poststroke 6-month poor outcome [extended Glasgow outcome scale (GOSE) scores of 1–4]. Predictive efficiency was determined under the receiver operating characteristic (ROC) curve.
Results: There were significantly increased serum HIF-lα levels after aSAH, in comparison to controls (median, 288.0 vs. 102.6 pg/ml; P < 0.001). Serum HIF-lα levels were independently correlated with Hunt–Hess scores [β, 78.376; 95% confidence interval (CI): 56.446–100.305; P = 0.001] and modified Fisher scores (β, 52.037; 95% CI: 23.461–80.614; P = 0.002). Serum HIF-lα levels displayed significant efficiency for discriminating DCI risk [area under ROC curve (AUC), 0.751; 95% CI: 0.687–0.815; P < 0.001] and poor outcome (AUC, 0.791; 95% CI: 0.736–0.846; P < 0.001). Using the Youden method, serum HIF-1α levels >229.3 pg/ml predicted the development of DCI with 92.3% sensitivity and 48.4% specificity and serum HIF-1α levels >384.0 pg/ml differentiated the risk of a poor prognosis with 71.4% sensitivity and 81.1% specificity. Serum HIF-1α levels >229.3 pg/ml were independently predictive of DCI [odds ratio (OR), 3.061; 95% CI: 1.045–8.965; P = 0.041] and serum HIF-1α levels >384.0 pg/ml were independently associated with a poor outcome (OR, 2.907; 95% CI: 1.403–6.024; P = 0.004). The DCI predictive ability of their combination was significantly superior to those of Hunt–Hess scores (AUC, 0.800; 95% CI: 0.745–0.855; P = 0.039) and modified Fisher scores (AUC, 0.784; 95% CI: 0.726–0.843; P = 0.004). The prognostic predictive ability of their combination substantially exceeded those of Hunt–Hess scores (AUC, 0.839; 95% CI: 0.791–0.886; P < 0.001) and modified Fisher scores (AUC, 0.844; 95% CI: 0.799–0.890; P < 0.001).
Conclusion: Elevated serum HIF-lα levels after aSAH, in independent correlation with stroke severity, were independently associated with DCI and 6-month poor outcome, substantializing serum HIF-lα as a potential prognostic biomarker of aSAH.
Introduction
Aneurysmal subarachnoid hemorrhage (aSAH) affects six to nine people per 100,000 per year, accounts for 5% of the hemorrhagic stroke, and is characterized by a high rate of morbidity and mortality (1). In addition to diagnosis and treatment, prognosis prediction is a very important aspect of aSAH therapy (2). Hunt–Hess scale and modified Fisher scale are often used to assess illness severity and predict clinical outcomes of aSAH (3). Extended Glasgow outcome scale (GOSE) is conventionally regarded as a prognostic parameter in some brain injury diseases, including aSAH (4). Delayed cerebral ischemia (DCI) is a usually observed adverse event after aSAH, which is associated with an increased risk of a poor outcome for patients with aSAH (5). Its occurrence may involve large artery vasospasm and/or microcirculatory disturbances by micro vasospasm, microthrombosis, dysfunction of venous outflow, and compression of microvasculature by vasogenic or cytotoxic tissue edema (5). In addition to DCI, early brain injury, which is identified as another pathophysiological event of secondary brain injury after aSAH, involves some key pathophysiological mechanisms, including brain ischemia and hypoxia, thereby inducing neuronal death and finally causing neurologic dysfunction (6). Because peripheral blood is a kind of easily obtained biofluid, biochemical markers in peripheral blood have attracted extensive interest as prognostic parameters of aSAH during recent decades (7–9).
Hypoxia-inducible factor-1 (HIF-1), an oxygen-sensitive transcriptional activator, regulates the expression of a series of genes that facilitate the adaptation to low oxygen tension (hypoxia) in cells and tissues (10). HIF-1alpha (1α) is a subunit (11) whose expressions by neurons and astrocytes were markedly upregulated following experimental SAH, ischemic stroke, or intracerebral hemorrhage (12–15). Up to now, there are inconsistent data available regarding the actual effect of HIF-1α in acute brain injury, whether protective or detrimental (15–21). In a study of 40 patients with acute ischemic stroke, elevated serum HIF-1α levels were closely related to cerebral infarction size (22). Alternatively, in two cohort studies of almost 100 patients with spontaneous intracerebral hemorrhage or severe traumatic brain injury, there was a significant increase in serum HIF-1α levels, which, in intimate correlation with illness severity, independently predicted poor prognosis (Glasgow outcome scale scores of 1–3) at 90 days after injury (23, 24). However, to the best of our knowledge, HIF-1α levels have not been measured in peripheral blood of humans with aSAH. Herein, we undertook a multicenter study to further ascertain whether serum HIF-1α could be associated with the illness severity and long-term clinical outcome of humans with aSAH.
Methods
Study design, subject selection, and ethics approval
This prospective longitudinal observational study was performed from April 2018 to April 2021 at three hospitals, including Ruian People's Hospital, the Third Affiliated Hospital of Wenzhou Medical University; Ningbo Branch, Renji Hospital, Shanghai Jiao Tong University School of Medicine; and Hangzhou Ninth People's Hospital. We consecutively enrolled patients with nontraumatic SAH, who were diagnosed via head computed tomography (CT) scan. Those patients were entered into the study if they followed the inclusion criteria as listed below: (1) first-onset spontaneous SAH, (2) adults (age equal to or more than 18 years), (3) SAH resulting from rupture of a single intracranial aneurysm, (4) hospitalization of 24 h following SAH, and (5) aneurysmal treatment of 48 h after hospital admission. Some patients should be excluded in agreement with the following exclusion criteria: (1) occurrence of aneurysm rebleeding, (2) suspected pseudoaneurysm, (3) previous neurologic diseases, such as stroke, severe head trauma, myasthenia gravis, and intracranial tumors, and (4) other specific diseases or conditions, such as pregnancies, malignancies, chronic heart, liver, kidney, and lung diseases, and other coexisting acute severe illnesses, including acute myocardial infarction and acute lung injury. From April 2020 to April 2021, a group of healthy volunteers was recruited as controls at the preceding three hospitals. Controls were free of other diseases, such as hypertension, diabetes mellitus, and chronic heart disease. Some routine tests, including blood glucose, hemoglobin, and sodium and potassium levels, as well as blood leucocyte, platelet, and neutrophil counts, were normal in controls. The protocol of the current study was established, and the study was carried out in compliance with the Declaration of Helsinki. Also, approval for the protocol of this study was acquired from the Ethical Committees of the aforementioned three hospitals. Patients' relatives or controls themselves signed informed consent for participating in this study.
Data collection and clinical and outcome assessment
Upon entry into the emergency center, we inquired about demographics (age and gender), hospital admission time, vascular risk factors, including two adverse life habits (cigarette smoking and alcohol drinking) and three chronic illnesses (hypertension, diabetes mellitus, and hyperlipidemia), and medication history (use of statins, anticoagulation drugs, and antiplatelet drugs). Hunt–Hess scale was considered as a clinical severity indicator and the modified Fisher scale was regarded as a radiological severity parameter. Aneurysm-related radiological characteristics were obtained using CT angiography or digital subtraction angiography (DSA). Specifically, the position was divided into posterior and anterior circulation, the shape was classified into cystic and others, and the size was assigned into diameters < 10 mm and ≥10 mm. Therapeutic modalities for aneurysms included neurosurgical clipping and endovascular interventional embolization. Two acute adverse events were acute hydrocephalus and intraventricular hemorrhage, which were confirmed via head CT scans. External ventricular drainage was a surgical choice for such adverse events. During in-hospital treatments, DCI was determined in compliance with the previously established criteria as follows: (1) clinical deterioration (namely, a new focal deficit, decrease in the level of consciousness, or both) and/or (2) a new infarct on the head CT scan that was invisible at admission or immediately postoperatively, and cannot be attributed to other causes by means of clinical assessment, imaging of the brain, and appropriate laboratory studies (25). Extended Glasgow outcome scale (GOSE), ranging from 1 to 8, was recorded for assessing functional outcomes of patients with aSAH at 6 months post-injury. GOSE scores of 1–4 were referred to as a poor outcome (26).
Measurement of serum HIF-1α levels
Blood samples were promptly obtained upon patients' entry into the emergency department and those of controls were acquired when entering into the study. After blood was centrifugated, serum was aliquoted, and then stored at −80°C until measurements. A commercially available human HIF-1α enzyme-linked immunosorbent assay kit (RapidBio Lab, California, USA) was used for the determination of serum HIF-1α based on the manufacturer's instructions. The determinations of serum HIF-1a were in batches done every 3 months. Serum HIF-1α levels were in duplicate detected by the same technician who was inaccessible to the clinical data, and accordingly, the mean value of two measurements was utilized for further statistical analysis.
Statistical analysis
Statistical analysis was performed using three statistical software, namely, R software (version 3.5.1; https://www.r-project.org), SPSS 19.0 (SPSS Inc., Chicago, IL, USA), and MedCalc 9.6.4.0 (MedCalc Software, Mariakerke, Belgium). Graphs were plotted using GraphPad Prism software version 6.0 for Windows (GraphPad Software, San Diego, California, USA). Qualitative variables, which were shown as frequencies (proportions), were compared between two groups using the Chi-square test or Fisher exact test where appropriate. Normally distributed quantitative variables, which were reported as means (standard deviations, SD), were compared between two groups using an independent t-test. Non-normally distributed quantitative variables, which were presented as medians (percentiles 25th−75th), were compared between two groups using the Mann–Whitney U-test. The Kruskal–Wallis test was performed to be a comparative of serum HIF-1α levels among multiple groups with different GOSE scores, Hunt–Hess scores, or modified Fisher scores. The Spearman test, followed by the multivariate linear regression analysis, was used to ascertain the independent relationships between serum HIF-1α levels and hemorrhagic severity, which were indicated by Hunt–Hess scores and modified Fisher scores. The binary logistic regression models were built to discern predictors, which were in an independent relation with DCI and 6-month poor outcome. The area under the receiver operating characteristic (ROC) curve (AUC) was calculated to reflect the predictive efficiency. Using the Youden method, an optimal cutoff value of serum HIF-1α levels was chosen, thereby generating the corresponding sensitivities and specificities. Using the Z-test, AUCs were compared. Two-tailed P-values of < 0.05 indicated statistically significant differences.
Results
Participants' characteristics
During the study period, 344 nontraumatic patients with aSAH fitted the preset inclusion criteria, and then, a total of 257 patients with aSAH were retained for final analysis after 87 patients were excluded because of the reasons presented in Figure 1. Also, 100 healthy individuals constituted controls. Some baseline characteristics of controls were presented in Table 1. Age, as well as smoker, drinker, and gender percentages, did not significantly differ between patients and controls (all P > 0.05; Table 1).
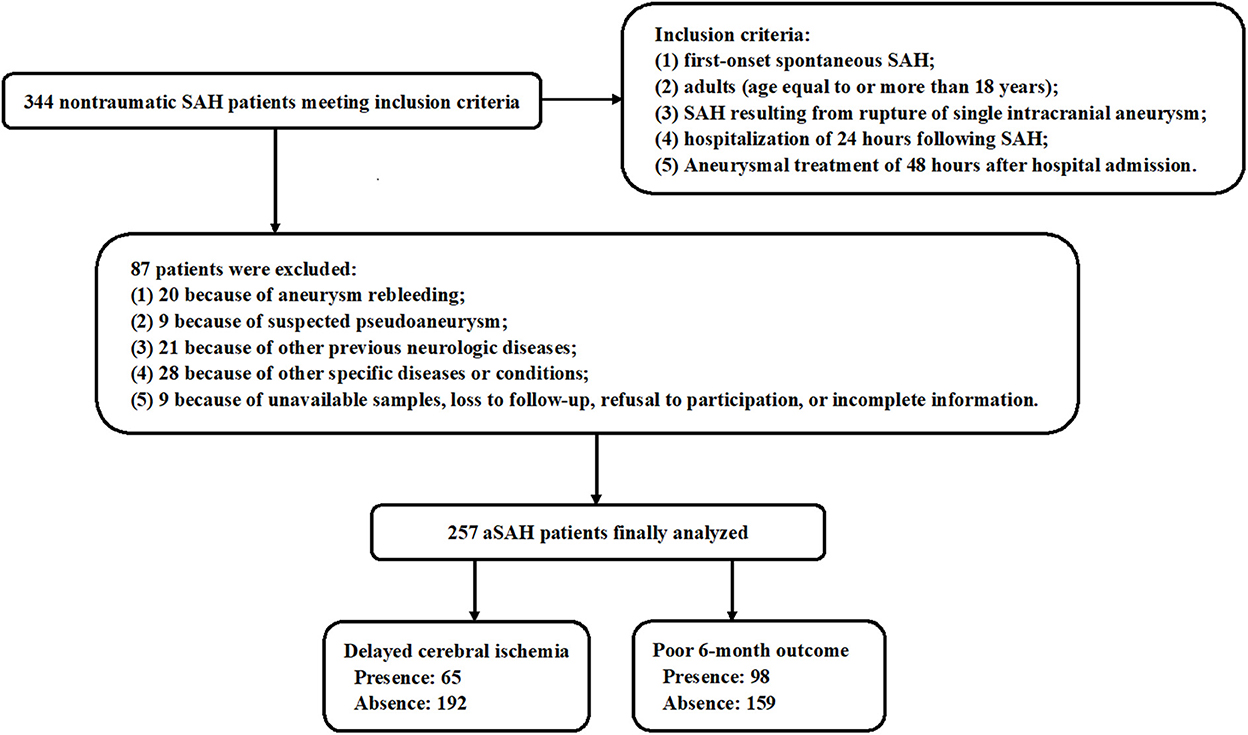
Figure 1. Flow chart for screening patients with aneurysmal subarachnoid hemorrhage. A total of 344 patients with spontaneous aneurysmal subarachnoid hemorrhage obtained an initial assessment according to the inclusion criteria, then 87 patients were removed from this study based on the exclusion criteria, and finally, 257 patients were retained for further analysis. aSAH, aneurysmal subarachnoid hemorrhage.
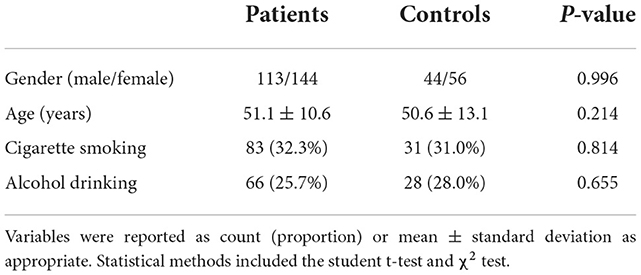
Table 1. Differences in baseline characteristics between healthy controls and patients with aneurysmal subarachnoid hemorrhage.
Among 257 patients with aSAH, there were 113 males and 144 females, and their age ranged from 30 years to 73 years (mean, 51.1 years; SD, 10.6 years). There were 83 cigarette smokers and 66 alcohol drinkers. A total of 53, 23, and 38 patients were inflicted with hypertension, diabetes mellitus, and hyperlipidemia, respectively. In aggregate, 21, 13, and 12 patients previously orally took statins, anticoagulation drugs, and antiplatelet drugs, respectively. Regarding aneurysm-related radiological characteristics, there were 153 aneurysms of < 10 mm in diameter, 207 aneurysms located in the anterior circulation, and 215 cystic aneurysms. Patients were admitted from 0.5 to 24.0 h (median, 10.3 h; lower–upper quartiles, 4.9–14.5 h) after injury. Blood was collected from 1.0 to 26.0 h (median, 11.7 h; percentiles 25th−75th, 6.6–16.3 h) after stroke. Hunt–Hess scores ranged from 1 to 5 (median, 3; lower–upper quartiles, 2–4). Modified Fisher scores ranged from 1 to 4 (median, 2; lower–upper quartiles, 2–3). Hunt–Hess scores of 1, 2, 3, 4, and 5 were found in 41, 59, 86, 57, and 14 patients, respectively. Modified Fisher scores of 1, 2, 3, and 4 were revealed in 46, 115, 72, and 24 patients, respectively. In total, 161 patients underwent an initial endovascular intervention for securing aneurysms and surgical clipping was done in other remainders. Totally, 32 patients were complicated with acute hydrocephalus, 26 patients suffered from intraventricular bleeding, and 33 patients accepted external drainage for the removal of intraventricular bleeding.
Serum HIF-1α levels between patients with aSAH and controls and its correlation with hemorrhagic severity
In Figure 2, patients with aSAH displayed substantially higher serum HIF-1α levels than controls (P < 0.001). In Table 2, serum HIF-1α levels were tightly correlated with other variables, namely, Hunt–Hess scores, modified Fisher scores, acute hydrocephalus, intraventricular hemorrhage, external ventricular drainage, and blood glucose levels (all P < 0.05). The significantly correlated variables were forced into the multivariate linear regression model, and afterward, serum HIF-1α levels were independently correlated with Hunt–Hess scores [β, 78.376; 95% confidence interval (CI): 56.446–100.305; P = 0.001] and modified Fisher scores (β, 52.037; 95% CI: 23.461–80.614; P = 0.002). Just as graphed in Figure 3, serum HIF-1α levels were highly correlated with Hunt–Hess scores and modified Fisher scores, whether they were identified as categorical or continuous variables (all P < 0.001).
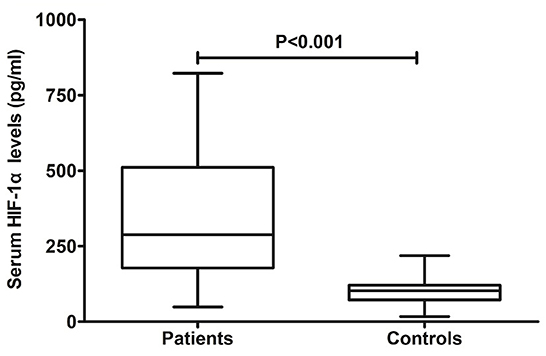
Figure 2. Change of serum hypoxia-inducible factor 1alpha levels after aneurysmal subarachnoid hemorrhage. Serum hypoxia-inducible factor 1alpha levels were significantly elevated in comparison to healthy controls (P < 0.001). HIF-1α denotes hypoxia-inducible factor 1alpha.
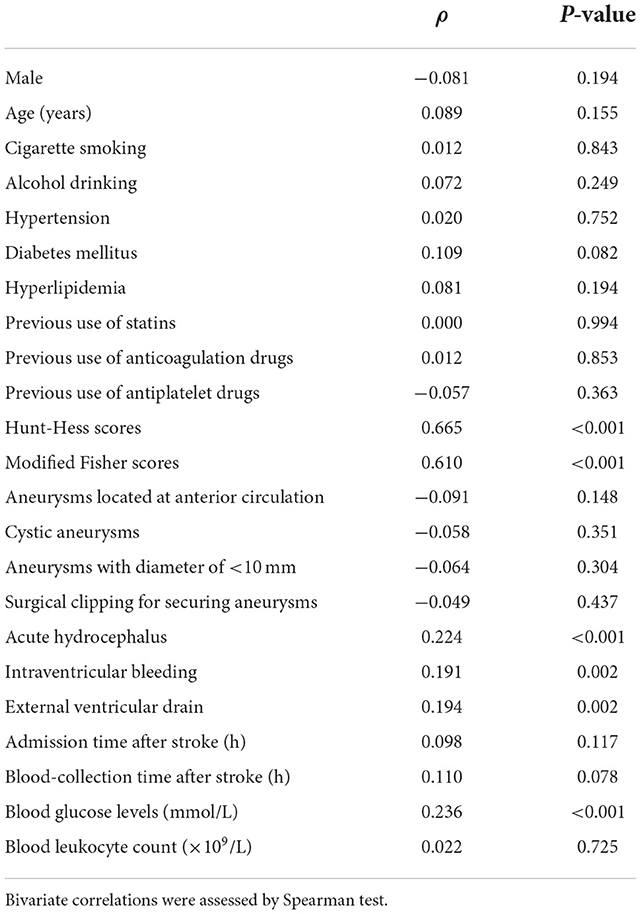
Table 2. Factors related to serum hypoxia-inducible factor 1alpha levels following aneurysmal subarachnoid hemorrhage.
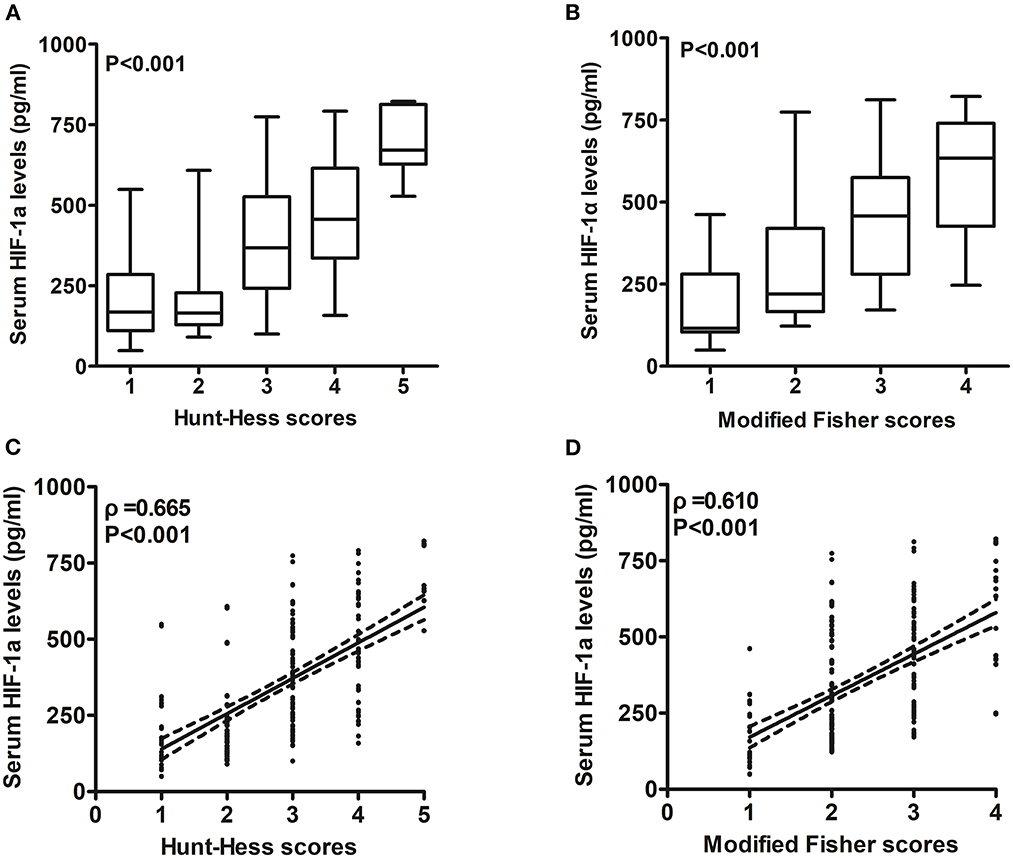
Figure 3. Relationship between serum hypoxia-inducible factor 1alpha levels and illness severity after aneurysmal subarachnoid hemorrhage. (A) Serum hypoxia-inducible factor 1alpha levels among patients with different Hunt–Hess scores. Serum hypoxia-inducible factor 1alpha levels were substantially lowest in patients with Hunt–Hess score of 1, followed by Hunt–Hess scores of 2, 3, and 4, and were markedly highest in those with Hunt–Hess score of 5 (P < 0.001). (B) Serum hypoxia-inducible factor 1alpha levels among patients with different modified Fisher scores. Serum hypoxia-inducible factor 1alpha levels were substantially lowest in patients with modified Fisher scores of 1, followed by modified Fisher scores of 2 and 3, and were markedly highest in those with modified Fisher scores of 4 (P < 0.001). (C) Relation of serum hypoxia-inducible factor 1alpha levels to Hunt–Hess scores. Serum hypoxia-inducible factor 1alpha levels were substantially correlated with Hunt–Hess scores (P < 0.001). (D) Relation of serum hypoxia-inducible factor 1alpha levels to modified Fisher scores. Serum hypoxia-inducible factor 1alpha levels were substantially correlated with modified Fisher scores (P < 0.001). HIF-1α means hypoxia-inducible factor 1alpha.
Serum HIF-1α levels and DCI
A total of 65 patients (25.3%) with DCI were found in this cohort of patients with aSAH. Serum HIF-1α levels were significantly higher in patients with DCI than in those without DCI (P < 0.001; Figure 4A). Under the ROC curve, serum HIF-1α levels distinguished patients at significant risk of DCI (AUC, 0.751; 95% CI: 0.687–0.815; P < 0.001). Using the Youden method, serum HIF-1α levels of >229.3 pg/ml predicted the development of DCI with medium–high sensitivity and specificity values (Figure 4B).
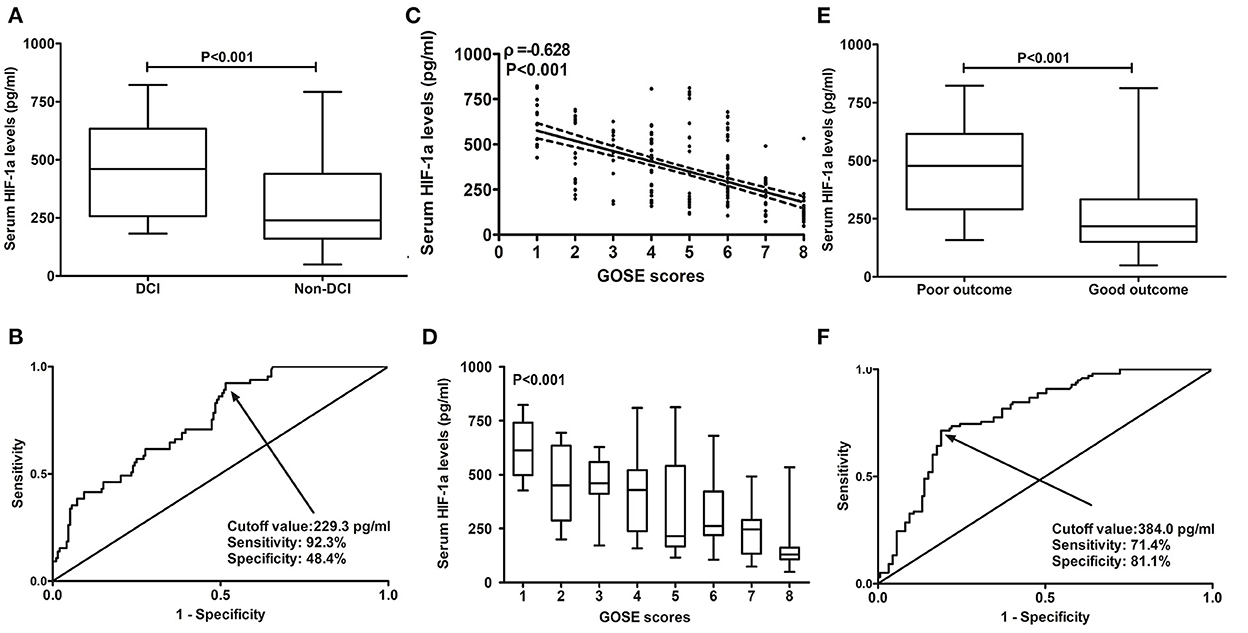
Figure 4. Predictive ability of serum hypoxia-inducible factor 1alpha levels for patients at risk of delayed cerebral ischemia and 6-month poor outcome after aneurysmal subarachnoid hemorrhage. (A) Serum hypoxia-inducible factor 1alpha levels between patients with delayed cerebral ischemia and those not presenting with delayed cerebral ischemia. Serum hypoxia-inducible factor 1 alpha levels were profoundly higher in patients with delayed cerebral ischemia than in other remainders (P < 0.001). (B) Discriminatory power of serum hypoxia-inducible factor 1alpha levels for risk of delayed cerebral ischemia. Serum hypoxia-inducible factor 1alpha levels substantially distinguished the development of delayed cerebral ischemia (P < 0.001). Using the Youden method, an optimal cutoff value was chosen, which produced the corresponding sensitivity and specificity values. (C) Relation of serum hypoxia-inducible factor 1alpha levels to extended Glasgow outcome scale scores. Serum hypoxia-inducible factor 1alpha levels were substantially correlated with extended Glasgow outcome scale scores (P < 0.001). (D) Serum hypoxia-inducible factor 1alpha levels among patients with different extended Glasgow outcome scale scores. Serum hypoxia-inducible factor 1alpha levels were substantially highest in patients with extended Glasgow outcome scale score of 1, followed by extended Glasgow outcome scale scores of 2–7, and were markedly lowest in those with extended Glasgow outcome scale score of 8 (P < 0.001). (E) Serum hypoxia-inducible factor 1alpha levels between patients with poor outcomes and those with good outcomes. Serum hypoxia-inducible factor 1 alpha levels were profoundly higher in patients with poor outcomes than in those with good outcomes (P < 0.001). (F) Distinguishable value of serum hypoxia-inducible factor 1alpha levels for poor outcomes. Serum hypoxia-inducible factor 1alpha levels pronouncedly discriminated poor outcomes (P < 0.001). Using the Youden method, an optimal cutoff value was selected, which yielded the corresponding sensitivity and specificity values. HIF-1α, hypoxia-inducible factor 1alpha; GOSE, extended Glasgow outcome scale; DCI, delayed cerebral ischemia.
In Table 3, as compared to patients not presenting with DCI, those suffering from DCI exhibited substantially elevated Hunt–Hess scores, modified Fisher scores, and blood glucose (all P < 0.05), as well as had significantly higher percentages of serum HIF-1α levels of >229.3 pg/ml, acute hydrocephalus, intraventricular hemorrhage, and external ventricular drainage (all P < 0.05). Thereafter, the aforementioned variables were forced into the binary logistic regression model, and subsequently, it was shown that serum HIF-1α levels of >229.3 pg/ml [odds ratio (OR), 3.061; 95% CI: 1.045–8.965; P = 0.041], Hunt–Hess scores (OR, 1.972; 95% CI: 1.233–3.155; P = 0.005), and modified Fisher scores (OR, 1.977; 95% CI: 1.167–3.351; P = 0.011) were the three independent predictors of DCI after aSAH.
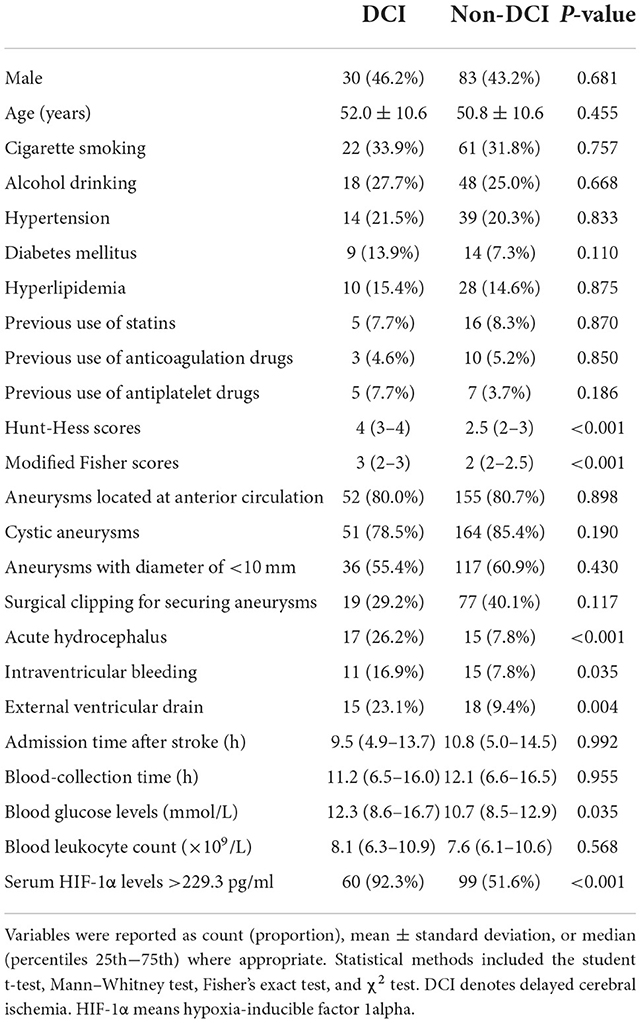
Table 3. Factors in relation to delayed cerebral ischemia following aneurysmal subarachnoid hemorrhage.
A nomogram was drawn to obtain a more comprehensive view of the relationship between DCI risk and other independent predictors, namely, Hunt–Hess scores, modified Fisher scores, and serum HIF-1α levels of >229.3 pg/ml (Figure 5). The calibration curve of the nomogram for distinguishing DCI risk showed that the mean absolute error was 0.020 (Figure 6). The combined binary logistic regression model was built, which included Hunt–Hess scores, modified Fisher scores, and serum HIF-1α levels of >229.3 pg/ml (using Hosmer and Lemeshow test, P = 0.908). Subsequently, serum HIF-1α levels of >229.3 pg/ml combined with Hunt–Hess scores and modified Fisher scores had AUC at 0.832 (95% CI: 0.780–0.884). In Figure 7, the DCI predictive ability of their combination was significantly superior to those of Hunt–Hess scores (AUC, 0.800; 95% CI: 0.745–0.855; P = 0.039) and modified Fisher scores (AUC, 0.784; 95% CI: 0.726–0.843; P = 0.004).
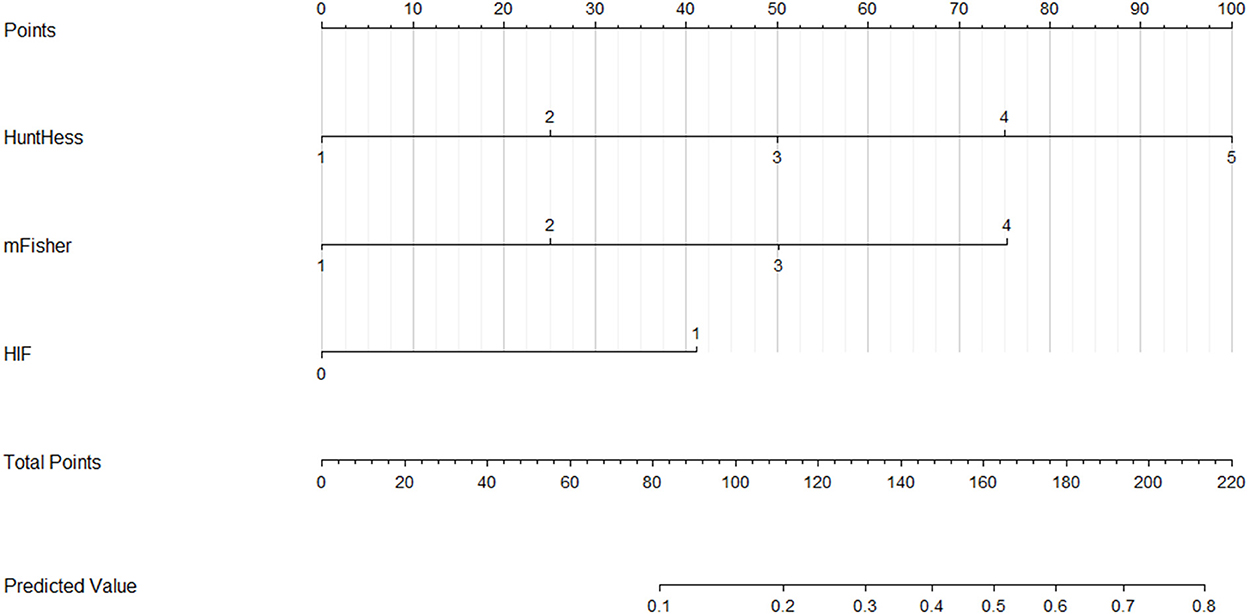
Figure 5. Nomogram for predicting delayed cerebral ischemia among patients with aneurysmal subarachnoid hemorrhage. Serum hypoxia-inducible factor 1alpha levels combined with Hunt–Hess scores and modified Fisher scores significantly discriminated against patients at risk of delayed cerebral ischemia. For HIF, “1” indicates serum hypoxia-inducible factor 1alpha levels >229.3 pg/ml and “0” means serum hypoxia-inducible factor 1alpha levels <229.3 pg/ml. HuntHess, Hunt–Hess scores; mFisher, modified Fisher scores; HIF, hypoxia-inducible factor 1alpha.
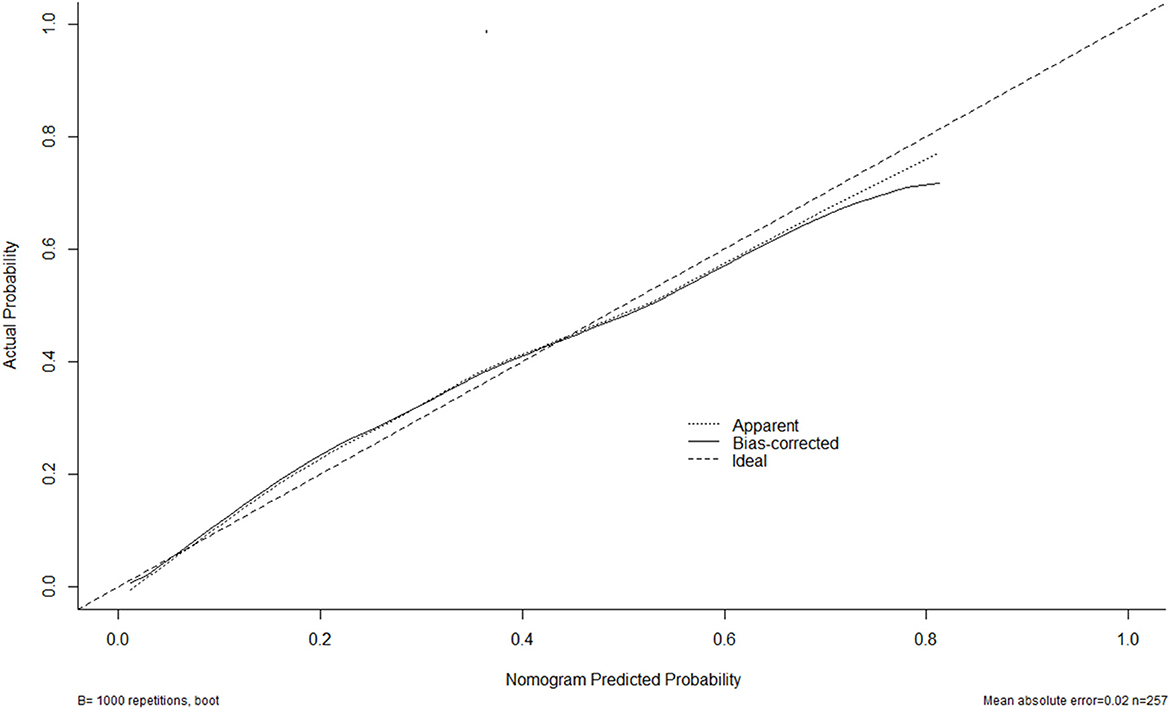
Figure 6. Calibration curves of the nomogram for predicting delayed cerebral ischemia among patients with aneurysmal subarachnoid hemorrhage. The x-axis denotes the predicted delayed cerebral ischemia. The y-axis denotes the actual delayed cerebral ischemia. The diagonal dotted line means a perfect prediction using an ideal model. The solid line represents the performance of the nomogram, of which the closer fit to the diagonal dotted line indicates the better prediction of the nomogram.
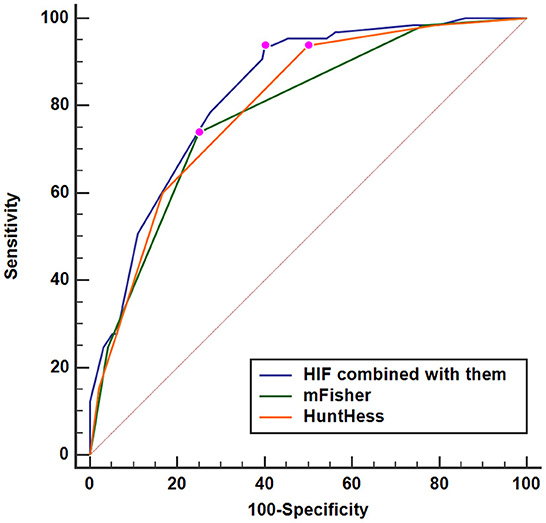
Figure 7. Predictive ability with respect to serum hypoxia-inducible factor 1alpha levels combined with Hunt–Hess scores and modified Fisher scores for the risk of delayed cerebral ischemia. Serum HIF-1α levels combined with Hunt–Hess scores and modified Fisher scores had significantly increased predictive ability for the risk of delayed cerebral ischemia, as compared to Hunt–Hess scores and modified Fisher scores alone (P < 0.05). HuntHess, Hunt–Hess scores; mFisher, modified Fisher scores; HIF, hypoxia-inducible factor 1alpha.
Serum HIF-1α levels and 6-month poor prognosis
At 6 months following aSAH, GOSE scores ranged from 1 to 8, with a median value of 5 (lower–upper quartiles, 4–7), and a total of 16, 29, 17, 36, 31, 61, 27, and 40 patients exhibited GOSE scores of 1, 2, 3, 4, 5, 6, 7, and 8, respectively. In Figure 4C, serum HIF-1α levels were substantially and inversely correlated with 6-month GOSE scores (P < 0.001), and in Figure 4D, they were statistically significantly highest in patients with the development of GOSE score of 1, followed by GOSE scores of 2, 3, 4, 5, 6, and 7, and were pronouncedly lowest in those presented with GOSE score of 8 (P < 0.001).
In aggregate, 98 patients (38.1%) experienced a poor prognosis (GOSE scores 1–4) at 6 months after aSAH. Just as presented in Figure 4E, serum HIF-1α levels were substantially elevated in patients with GOSE scores of 1–4 in comparison with those suffering from GOSE scores of 5–8 (P < 0.001). Under the ROC curve, serum HIF-1α levels substantially distinguished patients at risk of a poor prognosis (AUC, 0.791; 95% CI: 0.736–0.846; P < 0.001). Using the Youden method, serum HIF-1α levels of >384.0 pg/ml differentiated the risk of a poor prognosis with medium–high sensitivity and specificity values (Figure 4F).
Using univariate analysis, as compared to patients with good outcomes, those with poor outcomes showed substantially increased blood glucose levels, Hunt–Hess scores, and modified Fisher scores (all P < 0.05; Table 4), as well as had significantly increased percentages of serum HIF-1α levels of >384.0 pg/ml, previous use of anticoagulation drugs, diabetes mellitus, intraventricular hemorrhage, and hydrocephalus (all P < 0.05; Table 4). Afterward, the binary logistic regression model was established, where the preceding significant variables were contained, and it was demonstrated that Hunt–Hess scores, modified Fisher scores, and serum HIF-1α levels of >384.0 pg/ml independently predicted the development of 6-month poor prognosis after aSAH with OR values of 2.086 (95% CI: 1.302–3.342; P = 0.002), 4.467 (95% CI: 2.421–8.244; P = 0.001), and 2.907 (95% CI: 1.403–6.024; P = 0.004), respectively.
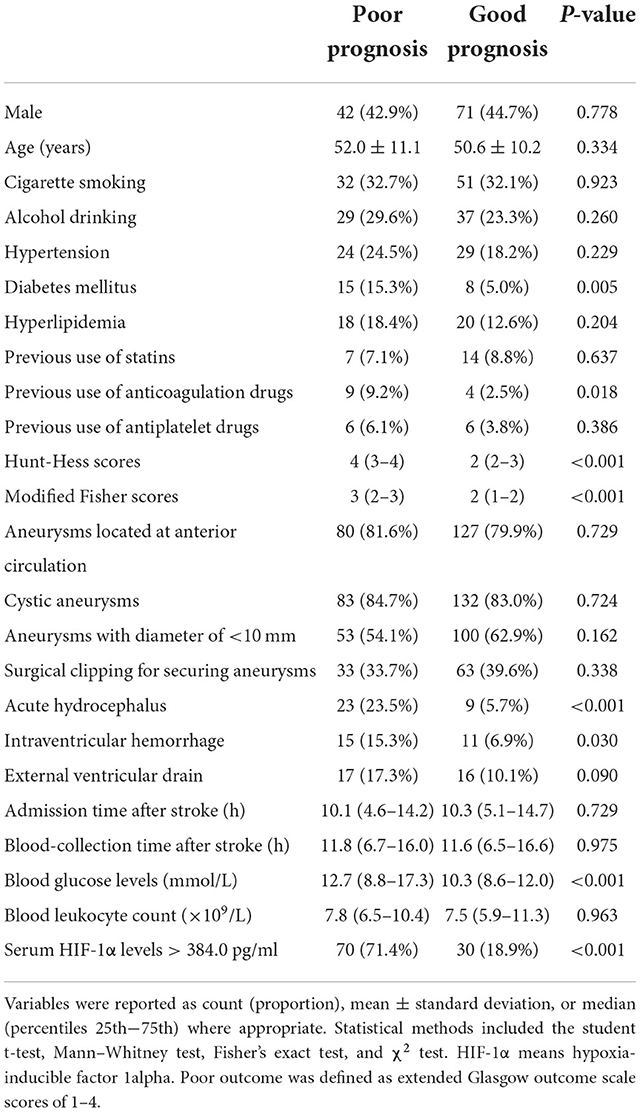
Table 4. Factors in correlation with 6-month poor prognosis following aneurysmal subarachnoid hemorrhage.
A nomogram was plotted to get a more comprehensive view of the relationship between a poor prognosis and other independent predictors, namely, Hunt–Hess scores, modified Fisher scores, and serum HIF-1α levels of >384.0 pg/ml (Figure 8). The calibration curve of the nomogram for predicting a poor prognosis showed that the mean absolute error was 0.018 (Figure 9). The combined binary logistic regression model was built, which included Hunt–Hess scores, modified Fisher scores, and serum HIF-1α levels of >384.0 pg/ml (using Hosmer and Lemeshow test, P = 0.604). Subsequently, serum HIF-1α levels of >384.0 pg/ml combined with Hunt–Hess scores and modified Fisher scores had AUC at 0.900 (95% CI: 0.862–0.938). In Figure 10, the prognostic predictive ability of their combination was significantly superior to those of Hunt–Hess scores (AUC, 0.839; 95% CI: 0.791–0.886; P < 0.001) and modified Fisher scores (AUC, 0.844; 95% CI: 0.799–0.890; P < 0.001).
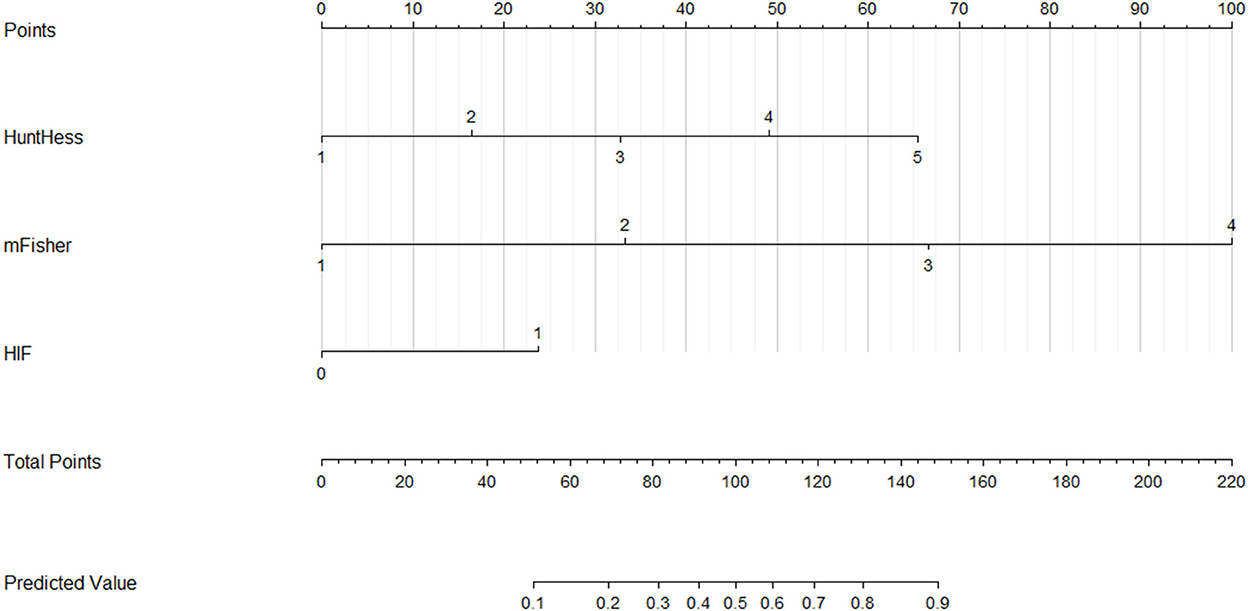
Figure 8. Nomogram for predicting poor outcome after aneurysmal subarachnoid hemorrhage. Serum hypoxia-inducible factor 1alpha levels combined with Hunt–Hess scores and modified Fisher scores significantly differentiated poor outcomes. For HIF, “1” indicates serum hypoxia-inducible factor 1alpha levels >384.0 pg/ml and “0” means serum hypoxia-inducible factor 1alpha levels < 384.0 pg/ml. HuntHess, Hunt–Hess scores; mFisher, modified Fisher scores; HIF, hypoxia-inducible factor 1alpha.
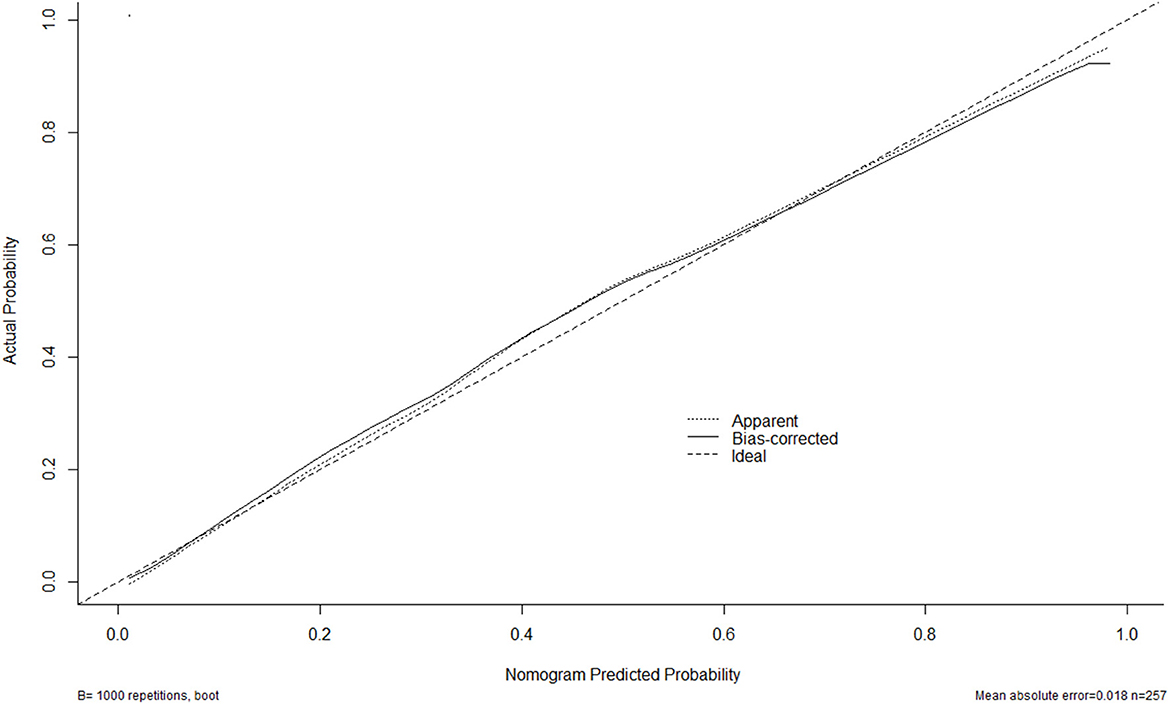
Figure 9. Calibration curves of the nomogram for predicting poor outcome after aneurysmal subarachnoid hemorrhage. The x-axis denotes the predicted poor outcome. The y-axis denotes the actual poor outcome. The diagonal dotted line means a perfect prediction using an ideal model. The solid line represents the performance of the nomogram, of which the closer fit to the diagonal dotted line indicates the better prediction of the nomogram.
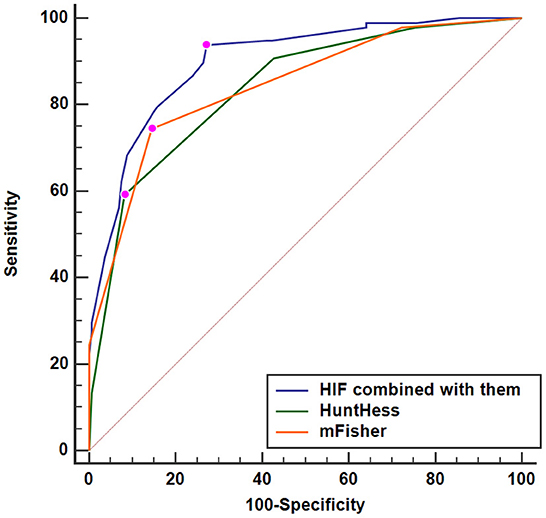
Figure 10. Predictive ability with respect to serum hypoxia-inducible factor 1alpha levels combined with Hunt–Hess scores and modified Fisher scores for poor outcome. Serum HIF-1α levels combined with Hunt–Hess scores and modified Fisher scores displayed substantially elevated discriminatory capability for poor outcome in comparison to Hunt–Hess scores and modified Fisher scores alone (P < 0.01). HuntHess, Hunt–Hess scores; mFisher, modified Fisher scores; HIF, hypoxia-inducible factor 1alpha.
Discussion
To the best of our knowledge, this is the first series for investigating circulating HIF-1α levels in humans with aSAH. Subsequently, we found some intriguing results that (1) there was a substantial increase of serum HIF-1α levels after aSAH, which were independently correlated with illness severity indicated by Hunt–Hess scores and modified Fisher scores, (2) serum HIF-1α levels independently predicted DCI and 6-month poor outcome after aSAH, and (3) serum HIF-1α levels combined with Hunt–Hess scores and modified Fisher scores displayed significantly higher predictive ability for DCI and poor 6-month outcome than Hunt–Hess scores and modified Fisher scores alone under the ROC curve. Such data were strongly supportive of the notion that serum HIF-1α may serve as a useful prognostic biomarker of aSAH and can aid in severity assessment and prognostic prediction after a human with aSAH.
Hypoxia-inducible factor 1alpha is a crucial upstream transcriptional factor in response to hypoxia (10). HIF-1α may act as a double-edged sword to participate in pathophysiological processes after acute brain injury (15–21). Some experimental data have demonstrated that HIF-1α may confer brain detrimental effects via increasing neuronal apoptosis and disrupting blood–brain barrier permeability (15–17). Contrarily, other experimental studies have shown that HIF-1α may harbor brain-protective potential and the protective mechanisms may be related to HIF-1α-induced angiogenesis and glycolytic metabolism (18–21). HIF-1α expression by rat-cultured cortical neurons was upregulated after being deprived of oxygen and glucose (27). Also, HIF-1α messenger RNA was induced in the ischemic brain penumbra of rats with permanent middle cerebral artery occlusion (28). Similarly, the HIF-1α protein was obviously expressed in perihematomal neurons of rats with intracerebral hemorrhage (14). Moreover, HIF-1α was expressed predominantly in neurons of rats subjected to endovascular perforation to cause aSAH (15). Overall, HIF-1α may be mainly derived from neurons after acute brain injury. Because HIF-1α, functioning as a transcription factor, is localized in the intracellular compartment (12, 13), hypothetically, in response to acute brain injury, HIF-1α in the extracellular compartment may not be secreted from neurons but be released from destroyed neurons. We found a substantial elevation of serum HIF-1α levels after aSAH. It is deduced that HIF-1α in peripheral blood of this group of patients with aSAH might at least partly originate from hemorrhage-injured neurons.
The neurovascular unit is composed of various interacting cells, including neurons, astrocytes, microglia, pericytes, endothelial cells, and vascular smooth muscle cells (29). Neurovascular unit components interact and balance microenvironments, thereby ensuring stable neuronal function (30). HIF-1α is extensively expressed in the neurovascular unit and mediates the transcriptional expression of more than 100 genes under hypoxic conditions (31). These HIF-1a target genes are implicated in various processes, including inflammation, cell metabolism, proliferation, survival, death, cytoskeletal structure formation, cell adhesion, and movement (32). Thus, HIF-1α exerts cell type-specific actions and its phenotype becomes more complex. Specifically, HIF-1α harbored neuroprotective potentials via protecting cerebrovascular function, lessening cerebral edema, and inhibiting neuronal apoptosis after experimental aSAH (33–35); in contrast, HIF-1α conferred detrimental properties via disrupting the blood–brain barrier, increasing brain edema, and aggravating neuroinflammation in animals with aSAH (36–39). Overall, such divergent effects of HIF-1α in aSAH may be attributed to the diversity of downstream targets, which function in different cell types within the neurovascular unit. Also, such differences in the functioning of HIF-1α between cell types and in different environments make it a challenging therapeutic target in aSAH.
Up to now, there have been three clinical studies regarding the relationship between serum HIF-1α levels and the prognosis of humans with acute brain injury, where elevated serum HIF-1α levels were highly correlated with cerebral infarction size in 40 patients with acute ischemic stroke (22), were independently associated with admission Glasgow outcome scale scores and 90-day poor outcome in 97 patients with acute spontaneous intracerebral hemorrhage (23), and were independently predictive of 90-day poor prognosis in 104 patients with severe traumatic brain injury (24). In the current study of patients with aSAH, a multicenter study was conducted, the sample number was increased to 257 patients, the follow-up time was extended to 6 months after injury and its associations with both severity and prognosis were verified using multivariate analyses, and the combined model was built for prognostic prediction. Our data demonstrated that elevated serum HIF-1α levels, in strong correlation with Hunt–Hess scores and modified Fisher scores, were independently associated with DCI risk and long-term poor clinical outcomes. Of note, a combined model, containing serum HIF-1α levels, Hunt–Hess scores, and modified Fisher scores, displayed higher predictive ability for DCI development or poor functional outcome following aSAH in comparison to Hunt–Hess scores and modified Fisher scores alone. In summary, serum HIF-1α may represent a potential prognostic biochemical marker of aSAH.
There are several limitations of this study. First, although our data were demonstrated in a multicenter study, which included a medium sample size of patients with aSAH, a larger cohort study is needed to validate the conclusions. Second, except for intraventricular hemorrhage and hydrocephalus, some other acute adverse events, such as epilepsy, hyponatremia, and pulmonary infection, have not been recorded in this study. Thus, a future study is warranted, which will contain more acute adverse affairs. Finally, serum HIF-1α levels were determined at hospital admission of patients with aSAH. Thus, its dynamic changes in serum levels are unclear, which will be explored in future.
Conclusion
To the best of our knowledge, this is the first series for investigating circulating HIF-1α levels in humans with aSAH. In this multicenter study, we demonstrate that elevated serum HIF-1α levels are strongly correlated with illness severity and are tightly associated with DCI and long-term functional outcomes of aSAH. Interestingly, serum HIF-1α levels combined with Hunt–Hess scores and modified Fisher scores have a higher predictive ability for DCI risk or poor functional outcome after aSAH, as compared to Hunt–Hess scores and modified Fisher scores alone. Hence, serum HIF-1α may display the prognostic role and be of clinical value in severity assessment and outcome prediction of aSAH.
Data availability statement
The raw data supporting the conclusions of this article will be made available by the authors, without undue reservation.
Ethics statement
The studies involving human participants were reviewed and approved by the Ruian People's Hospital, Ningbo Branch, Ren Ji Hospital, Shanghai Jiao Tong University School of Medicine, and Hangzhou Ninth People Hospital. The patients/participants provided their written informed consent to participate in this study.
Author contributions
All authors listed have made a substantial, direct, and intellectual contribution to the work and approved it for publication.
Funding
This work was financially supported by a grant from the Wenzhou Science and Technology Plan Project (No. Y20210930).
Acknowledgments
The authors thank all staff for their technical support and are grateful to all participants for their providing blood samples.
Conflict of interest
The authors declare that the research was conducted in the absence of any commercial or financial relationships that could be construed as a potential conflict of interest.
Publisher's note
All claims expressed in this article are solely those of the authors and do not necessarily represent those of their affiliated organizations, or those of the publisher, the editors and the reviewers. Any product that may be evaluated in this article, or claim that may be made by its manufacturer, is not guaranteed or endorsed by the publisher.
Abbreviations
aSAH, aneurysmal subarachnoid hemorrhage; AUC, area under curve; CI, confidence interval; GOSE, extended Glasgow outcome scale; OR, odds ratio; ROC, receiver operating characteristic; DCI, delayed cerebral ischemia; HIF-1α, hypoxia-inducible factor 1alpha.
References
1. Rouanet C, Silva GS. Aneurysmal subarachnoid hemorrhage: current concepts and updates. Arq Neuropsiquiatr. (2019) 77:806–14. doi: 10.1590/0004-282x20190112
2. Lai X, Zhang W, Ye M, Liu X, Luo X. Development and validation of a predictive model for the prognosis in aneurysmal subarachnoid hemorrhage. J Clin Lab Anal. (2020) 34:e23542. doi: 10.1002/jcla.23542
3. Fang Y, Lu J, Zheng J, Wu H, Araujo C, Reis C, et al. Comparison of aneurysmal subarachnoid hemorrhage grading scores in patients with aneurysm clipping and coiling. Sci Rep. (2020) 10:9199. doi: 10.1038/s41598-020-66160-0
4. Aldrich EF, Higashida R, Hmissi A, Le EJ, Macdonald RL, Marr A, et al. Thick and diffuse cisternal clot independently predicts vasospasm-related morbidity and poor outcome after aneurysmal subarachnoid hemorrhage. J Neurosurg. (2020) 134:1553–61. doi: 10.3171/2020.3.JNS193400
5. Suzuki H, Kanamaru H, Kawakita F, Asada R, Fujimoto M, Shiba M. Cerebrovascular pathophysiology of delayed cerebral ischemia after aneurysmal subarachnoid hemorrhage. Histol Histopathol. (2021) 36:143–58. doi: 10.14670/HH-18-253
6. Osgood ML. Aneurysmal subarachnoid hemorrhage: review of the pathophysiology and management strategies. Curr Neurol Neurosci Rep. (2021) 21:50. doi: 10.1007/s11910-021-01136-9
7. Pan J, Lao L, Shen J, Huang S, Zhang T, Fan W, et al. Utility of serum NOX4 as a potential prognostic biomarker for aneurysmal subarachnoid hemorrhage. Clin Chim Acta. (2021) 517:9–14. doi: 10.1016/j.cca.2021.02.007
8. Yang X, Peng J, Pang J, Wan W, Zhong C, Peng T, et al. The association between serum macrophage migration inhibitory factor and delayed cerebral ischemia after aneurysmal subarachnoid hemorrhage. Neurotox Res. (2020) 37:397–405. doi: 10.1007/s12640-019-00072-4
9. Wang C, Kou Y, Han Y, Li X. Early serum calprotectin (S100A8/A9) predicts delayed cerebral ischemia and outcomes after aneurysmal subarachnoid hemorrhage. J Stroke Cerebrovasc Dis. (2020) 29:104770. doi: 10.1016/j.jstrokecerebrovasdis.2020.104770
10. Ke Q, Costa M. Hypoxia-inducible factor-1 (HIF-1). Mol Pharmacol. (2006) 70:1469–80. doi: 10.1124/mol.106.027029
11. Semenza GL. Defining the role of hypoxia-inducible factor 1 in cancer biology and therapeutics. Oncogene. (2010) 29:625–34. doi: 10.1038/onc.2009.441
12. Ramamoorthy P, Shi H. Ischemia induces different levels of hypoxia inducible factor-1α protein expression in interneurons and pyramidal neurons. Acta Neuropathol Commun. (2014) 2:51. doi: 10.1186/2051-5960-2-51
13. Ramamoorthy P, Xu G, Shi H. Expression of hypoxia inducible factor 1alpha is protein kinase A-dependent in primary cortical astrocytes exposed to severe hypoxia. Neurochem Res. (2019) 44:258–68. doi: 10.1007/s11064-018-2516-9
14. Jiang Y, Wu J, Keep RF, Hua Y, Hoff JT, Xi G. Hypoxia-inducible factor-1alpha accumulation in the brain after experimental intracerebral hemorrhage. J Cereb Blood Flow Metab. (2002) 22:689–96. doi: 10.1097/00004647-200206000-00007
15. Wang Z, Meng CJ, Shen XM, Shu Z, Ma C, Zhu GQ, et al. Potential contribution of hypoxia-inducible factor-1α, aquaporin-4, and matrix metalloproteinase-9 to blood-brain barrier disruption and brain edema after experimental subarachnoid hemorrhage. J Mol Neurosci. (2012) 48:273–80. doi: 10.1007/s12031-012-9769-6
16. Ding JY, Kreipke CW, Speirs SL, Schafer P, Schafer S, Rafols JA. Hypoxia-inducible factor-1alpha signaling in aquaporin upregulation after traumatic brain injury. Neurosci Lett. (2009) 453:68–72. doi: 10.1016/j.neulet.2009.01.077
17. Higashida T, Peng C, Li J, Dornbos D 3rd, Teng K, Li X, et al. Hypoxia-inducible factor-1α contributes to brain edema after stroke by regulating aquaporins and glycerol distribution in brain. Curr Neurovasc Res. (2011) 8:44–51. doi: 10.2174/156720211794520251
18. Li YQ, Hui ZR, Tao T, Shao KY, Liu Z, Li M, et al. Protective effect of hypoxia inducible factor-1α gene therapy using recombinant adenovirus in cerebral ischaemia-reperfusion injuries in rats. Pharm Biol. (2020) 58:438–46. doi: 10.1080/13880209.2020.1762667
19. Lv B, Li F, Fang J, Xu L, Sun C, Han J, et al. Hypoxia inducible factor 1α promotes survival of mesenchymal stem cells under hypoxia. Am J Transl Res. (2017) 9:1521–9.
20. Zhu T, Zhan L, Liang D, Hu J, Lu Z, Zhu X, et al. Hypoxia-inducible factor 1α mediates neuroprotection of hypoxic postconditioning against global cerebral ischemia. J Neuropathol Exp Neurol. (2014) 73:975–86. doi: 10.1097/NEN.0000000000000118
21. Huang T, Huang W, Zhang Z, Yu L, Xie C, Zhu D, et al. Hypoxia-inducible factor-1α upregulation in microglia following hypoxia protects against ischemia-induced cerebral infarction. Neuroreport. (2014) 25:1122–8. doi: 10.1097/WNR.0000000000000236
22. Xue L, Chen H, Lu K, Huang J, Duan H, Zhao Y. Clinical significance of changes in serum neuroglobin and HIF-1α concentrations during the early-phase of acute ischemic stroke. J Neurol Sci. (2017) 375:52–7. doi: 10.1016/j.jns.2017.01.039
23. Cai Y, Zhuang YK, Wu XY, Dong XQ, Du Q, Yu WH, et al. Serum hypoxia-inducible factor 1alpha levels correlate with outcomes after intracerebral hemorrhage. Ther Clin Risk Manag. (2021) 17:717–26. doi: 10.2147/TCRM.S313433
24. Lv QW, Zheng ZQ, Zhang H, Guo M, Shen LJ. Serum hypoxia-inducible factor 1alpha emerges as a prognostic factor for severe traumatic brain injury. Clin Chim Acta. (2021) 522:77–82. doi: 10.1016/j.cca.2021.08.017
25. Frontera JA, Fernandez A, Schmidt JM, Claassen J, Wartenberg KE, Badjatia N, et al. Defining vasospasm after subarachnoid hemorrhage: what is the most clinically relevant definition? Stroke. (2009) 40:1963–8. doi: 10.1161/STROKEAHA.108.544700
26. Wilson L, Boase K, Nelson LD, Temkin NR, Giacino JT, Markowitz AJ, et al. A manual for the Glasgow Outcome Scale-Extended Interview. J Neurotrauma. (2021) 38:2435–46. doi: 10.1089/neu.2020.7527
27. Ruscher K, Isaev N, Trendelenburg G, Weih M, Iurato L, Meisel A, et al. Induction of hypoxia inducible factor 1 by oxygen glucose deprivation is attenuated by hypoxic preconditioning in rat cultured neurons. Neurosci Lett. (1998) 254:117–20. doi: 10.1016/S0304-3940(98)00688-0
28. Bergeron M, Yu AY, Solway KE, Semenza GL, Sharp FR. Induction of hypoxia-inducible factor-1 (HIF-1) and its target genes following focal ischaemia in rat brain. Eur J Neurosci. (1999) 11:4159–70. doi: 10.1046/j.1460-9568.1999.00845.x
29. Hawkins BT, Davis TP. The blood-brain barrier/neurovascular unit in health and disease. Pharmacol Rev. (2005) 57:173–85. doi: 10.1124/pr.57.2.4
30. McConnell HL, Mishra A. Cells of the blood-brain barrier: an overview of the neurovascular unit in health and disease. Methods Mol Biol. (2022) 2492:3–24. doi: 10.1007/978-1-0716-2289-6_1
31. Powis G, Kirkpatrick L. Hypoxia inducible factor-1alpha as a cancer drug target. Mol Cancer Ther. (2004) 3:647–54. doi: 10.1158/1535-7163.647.3.5
32. He Q, Ma Y, Liu J, Zhang D, Ren J, Zhao R, et al. Biological functions and regulatory mechanisms of hypoxia-inducible factor-1α in ischemic stroke. Front Immunol. (2021) 12:801985. doi: 10.3389/fimmu.2021.801985
33. Oguzoglu AS, Senol N, Asci H, Erzurumlu Y, Gulle K, Savran M, et al. Pregabalin protects brain tissue from subarachnoid hemorrhage by enhancing HIF-1α/eNOS signaling and VEGF production. World Neurosurg. (2021) 152:e713–20. doi: 10.1016/j.wneu.2021.06.011
34. Milner E, Johnson AW, Nelson JW, Harries MD, Gidday JM, Han BH, et al. HIF-1α mediates isoflurane-induced vascular protection in subarachnoid hemorrhage. Ann Clin Transl Neurol. (2015) 2:325–37. doi: 10.1002/acn3.170
35. Dong Y, Li Y, Feng D, Wang J, Wen H, Liu D, et al. Protective effect of HIF-1α against hippocampal apoptosis and cognitive dysfunction in an experimental rat model of subarachnoid hemorrhage. Brain Res. (2013) 1517:114–21. doi: 10.1016/j.brainres.2013.04.024
36. Wu C, Hu Q, Chen J, Yan F, Li J, Wang L, et al. Inhibiting HIF-1α by 2ME2 ameliorates early brain injury after experimental subarachnoid hemorrhage in rats. Biochem Biophys Res Commun. (2013) 437:469–74. doi: 10.1016/j.bbrc.2013.06.107
37. Hu Q, Du Q, Yu W, Dong X. 2-Methoxyestradiol alleviates neuroinflammation and brain edema in early brain injury after subarachnoid hemorrhage in rats. Front Cell Neurosci. (2022) 16:869546. doi: 10.3389/fncel.2022.869546
38. Yan J, Chen C, Lei J, Yang L, Wang K, Liu J, et al. 2-methoxyestradiol reduces cerebral vasospasm after 48 hours of experimental subarachnoid hemorrhage in rats. Exp Neurol. (2006) 202:348–56. doi: 10.1016/j.expneurol.2006.06.009
Keywords: aneurysm, subarachnoid hemorrhage, hypoxia-inducible factor 1alpha, delayed cerebral ischemia, prognosis, severity
Citation: Cai Y-Y, Zhuang Y-K, Wang W-J, Jiang F, Hu J-M, Zhang X-L, Zhang L-X and Lou X-H (2022) Potential role of serum hypoxia-inducible factor 1alpha as a biomarker of delayed cerebral ischemia and poor clinical outcome after human aneurysmal subarachnoid hemorrhage: A prospective, longitudinal, multicenter, and observational study. Front. Neurol. 13:1072351. doi: 10.3389/fneur.2022.1072351
Received: 18 October 2022; Accepted: 22 November 2022;
Published: 08 December 2022.
Edited by:
Jean-charles Sanchez, Université de Genéve, SwitzerlandReviewed by:
Xin-Jiang Yan, Quzhou City People's Hospital, ChinaQuan Du, Zhejiang Chinese Medical University, China
Copyright © 2022 Cai, Zhuang, Wang, Jiang, Hu, Zhang, Zhang and Lou. This is an open-access article distributed under the terms of the Creative Commons Attribution License (CC BY). The use, distribution or reproduction in other forums is permitted, provided the original author(s) and the copyright owner(s) are credited and that the original publication in this journal is cited, in accordance with accepted academic practice. No use, distribution or reproduction is permitted which does not comply with these terms.
*Correspondence: Xiao-Hui Lou, d3pyYWx4aEAxNjMuY29t