- 1Department of Pediatrics, University of Florida College of Medicine, Gainesville, FL, United States
- 2Department of Health Outcomes and Biomedical Informatics, University of Florida College of Medicine, Gainesville, FL, United States
Spinal muscular atrophy (SMA) is a rare genetic disease that results in progressive neuromuscular weakness. Without therapy, the most common form of the disease, type 1, typically results in death or chronic respiratory failure in the first 2 years of life. Thanks to the recent introduction of newborn screening programs and the discovery of three disease-modifying therapies in the last decade, the outcomes of children with SMA have dramatically improved. Patients are able to achieve many, if not all, of the typical neuromotor milestones, such as sitting, standing and walking, as well as safe oral intake. As the natural history of treated patients is continuously evolving, children with SMA continue to require complex and multidisciplinary care, posing implementation and sustainability challenges. Accordingly, there is a significant need for the application and evaluation of implementation science to address the steps involved in the diagnosis and treatment of patients with SMA, ensuring that all pertinent stakeholders and systems are working effectively to deliver timely and appropriate care. In this manuscript, we discuss the current challenges and gaps in the care for children with SMA, as well as how implementation science can advance this field. In addition, we provide an adapted implementation science framework that includes the main domains and subdomains involved in the care of patients with SMA.
Introduction
Spinal muscular atrophy (SMA) is an autosomal recessive disease with an incidence of approximately 1 in 10,000 live births (1). SMA is caused by biallelic mutations in the Survival Motor Neuron 1 (SMN1) gene resulting in early onset degeneration of α-motor neurons in the anterior horn of the spinal cord with secondary progressive muscle weakness and atrophy (1). Thanks to advances in newborn screening programs, SMA can now be detected in the first week of life. Despite a lack of evidence-based therapies for SMA, the era of precision medicine holds promise for the implementation of advanced therapeutics to treat SMA. If implemented well, precision medicine approaches could have a significant benefit to patients with SMA and other rare diseases. However, biomedical science faces a formidable challenge in evaluating the clinical utility of scientific breakthroughs in rare diseases, especially if it follows the traditional path of innovation development, then efficacy and effectiveness studies, prior to implementation (2).
Background
The best known predictor of SMA severity is the number of copies of Survival Motor Neuron 2 (SMN2), a back-up gene to SMN1, which varies across the population (3). Historically, the disease has been classified into five different types based on achieved neuromotor milestones. Type 0 presents with very severe hypotonia at birth, has a life expectancy of < 6 months and affected infants usually have 1 SMN2 copy. Type 1 is clinically diagnosed in the first six months of life and affected patients are unable to sit or stand and typically have 2 or 3 SMN2 copies. Type 2 has its onset between 6 and 18 months of life, whereby affected children reach the milestone of sitting but do not stand or walk. Patients with type 2 disease have a life expectancy of 10 to 40 years and usually have 3 SMN2 copies. Type 3 presents after 18 months of life, and children are able to sit, stand and walk with assistance, reach adulthood and tend to have 3 to 4 SMN2 copies. Lastly, type 4, the mildest form, is typically diagnosed after the age of 5, whereby patients are able to walk unassisted, are usually diagnosed in adulthood, and carry 4 or more SMN 2 copies (4).
Natural history studies of the most common form, type 1, report a median age at death or the need for non-invasive ventilation of 10.5 months and only 8% of patients survive without respiratory support by 20 months (5). SMA is part of a long list of rare diseases that have benefited from advances in drug development, in part due to the US Orphan Drug Act (1983) that introduced incentives for the pharmaceutical industry to invest in therapies for rare diseases (6, 7). The orphan disease and drug concept follows the principle that people with rare diseases should have equal access to treatment, regardless of the prevalence of the disease. In the specific case of SMA, three therapies have been approved in the past 6 years: nusinersen, onasemnogene-abeparvovec, also known as “gene therapy,” and risdiplam (8–10).
Nusinersen was the first approved drug for SMA. It is an antisense oligonucleotide that binds to the splicing site on the SMN2 pre-mRNA. The modified mRNA is translated into functional SMN protein (10). Onasemnogene-abeparvovec, with the commercial name of Zolgensma® is an adeno-associated viral vector containing the gene that encodes the SMN protein, with an enhancer and promoter for proper gene function. It uses AAV9 or adeno-associated virus 9 to deliver the gene to the affected neurons as a one-time dose. Zolgensma® received orphan drug designation in 2019 (8). The most recent drug approved in 2020, risdiplam, is an mRNA splicing modifier for SMN2 that increases the production of SMN protein (9). Although none of these therapies constitutes a cure, significant improvements in survival, neuromotor function and quality of life are evident (8–10). In addition to these groundbreaking therapies, the recent implementation of newborn screening programs in forty eight states has allowed for early treatment of pre-symptomatic or minimally symptomatic children with SMA (11). However, likely due to the rapid approval of therapies paired with the recent introduction of methods for early diagnosis, a clear gap in implementation has become evident in “real world” settings. Little is known about the long-term implications of these therapies, the ideal healthcare infrastructure required to support their implementation, as well as the medical personnel and resources necessary to provide cost-effective care for these children with complex conditions, given the rapid scientific advancements in the field. Therefore, addressing stakeholder needs, as well as the processes involved in the care of children living with SMA is imperative for optimizing the implementation of SMA interventions and their sustainability.
We herein aim to discuss the main challenges related to the implementation of SMA care, including diagnosis, delivery of therapies, patient outcomes, cost and access, as well as long-term management of SMA.
From diagnosis to the delivery of therapies
In the era of newborn screening programs, SMA can be diagnosed in the first week of life (12). The program was initially included as part of the recommended uniform screening panel (RUSP) in July of 2018 and has been implemented in all but two states in the United States as of September of 2022, covering approximately 98% of new births (11, 13). In the United States, screening programs are administered at the state level and constitute a complex process that involves multiple organizations and time sensitive algorithms to deliver a timely diagnosis. The Newborn screening process for SMA focuses on the identification of exon 7 deletion in the SMN1 gene, with the goal to identify 95% of newborns with SMA (13). The majority of programs use the multiplex testing that is already utilized for severe combined immunodeficiency testing, a disease that has been part of the RUSP since 2010, which consequently decreases the cost of screening (13).
Environmental factors such as size of the state, population demographics, healthcare infrastructure, practice and provider factors, including laboratory personnel, methodology, medical providers' readiness, preparedness of designated diagnostic centers, as well as the infant and caregivers' characteristics and social determinants can influence the process and should be considered when implementing these interventions.
In some states, such as Florida, only SMN1 copy number is reported by the state laboratory (13). Therefore, early referral is required to assess SMN2 copy number through additional laboratory testing, as well as an urgent clinical encounter with a neuromuscular specialist or team of specialists. At the University of Florida Center for Neuromuscular and Rare Diseases, this step currently includes an appointment with Genetics, Neurology and Pulmonology, along with a video-fluoroscopy study for assessment of swallowing dysfunction and physical therapy evaluation of neuromotor milestones with the use of a validated developmental assessment. During the clinical encounter, treatment options are reviewed with the caregivers and if needed, additional laboratory testing is performed to determine which treatment is indicated. Although there are significant practice variations across the United States, in general, the sense of urgency and need for early evaluation is universal (14).
In addition to age requirements, SMN2 copy number, neuromotor and laboratory testing, insurance approval is a common step and often a significant barrier as approval can often take days to weeks. Some payers can be more restrictive than others by imposing additional criteria to those identified by the Food and Drug Administration (15). Unfortunately, delays in the process can be detrimental to neuromotor function. Once approval is obtained, the treatment center is responsible for the administration of the medication(s). In the case of nusinersen, periodic intrathecal injections performed by a certified physician and laboratory monitoring are required. Zolgensma® administration requires daily oral steroid dosing for roughly 2 months, a specialized pharmacy team, as well as frequent laboratory monitoring after drug administration and titration of steroids based on these and clinical parameters.
Although great progress has been made to date, there continues to be a gap in the implementation of newborn screening for SMA. Screening programs have not reached all states and in addition, there is a lack of clear guidance regarding the minimum or ideal personnel necessary for the adequate execution of this process. For example, specialists need to be involved, and there needs to be an acceptable and ideal time to diagnosis and time to therapy (13, 14).
Safety of disease modifying therapies
Various studies have reported on the safety of the aforementioned three medications. However, most of the research focuses on their short-term effects. In the case of nusinersen, intrathecal administration carries its own risks and discomforts. Although generally well tolerated, increased intracranial pressure and emesis requiring intubation during anesthesia have been reported (10, 16). Nusinersen is associated with proteinuria, thrombocytopenia and coagulation abnormalities. These laboratory findings tend to be mild and do not change the clinical management of patients living with SMA.
Zolgensma® administration is performed through intravenous infusion, which may require the placement of a central line due to the known abnormal vascularity of affected patients (17, 18). The treatment is associated with transaminitis, steroid responsive hepatotoxicity, complement mediated thrombocytopenia, and thrombotic microangiopathy that recently resulted in a fatality (19–22). An additional problem that has not been uniformly addressed is the variability in the administration of routine vaccinations to infants during steroid administration, with some families choosing to avoid immunizations during steroid treatment, putting the child at risk for fatal, preventable infections.
Risdiplam has been associated with diarrhea, rash, respiratory infections, including pneumonia during clinical trials and animal studies reported potentially reversible fertility problems and retinal toxicity (9, 23, 24). Lastly, although not studied as part of clinical trials, many patients have received two or more therapies with reported higher levels of transaminases in cases that have received Zolgensma® after nusinersen (25).
Long term safety data are scarce given the relative short duration of post marketing approval. Animal studies have raised concern about the risks related to AAV viruses and potential harm related to SMN overexpression at high doses, but literature in humans is lacking (26, 27). Safety monitoring should be the responsibility of the health care teams and pharmaceutical companies, with participation from health insurance companies and governmental agencies, creating a challenging but ideal opportunity for the application of implementation science frameworks.
Patient outcomes
The efficacy of these medications is evident; however, with three medications on the market, 1:1 comparative effectiveness studies are not feasible and are considered unethical at this point. Although rarely used in rare diseases after drug approval, a Bayesian adaptive trial design could be an option to assess the effect of combination therapies, Althought this design does not fully mitigate the ethical concerns about randomization to the inferior treatment, it reduces the likelihood that patients are allocated to the inferior treatment arm (28). A match-adjusted indirect comparative study of nusinersen vs. Zolgensma® using information from clinical trials (total sample of 82 patients) showed a favorable effect toward Zolgensma®, but the small sample and differences across populations limited the generalizability of the results (29). Interestingly, many patients have received two or three therapies as part of their clinical care but it is unclear which patients see more benefit from this approach (30).
It is worth mentioning that although most studies focus on neuromotor milestones, ventilator free time and overall survival, little is known about the efficacy of these therapies in other outcomes such as improvement of dysphagia, degree of sleep disorder breathing and scoliosis (31). Careful use of registries can assist health care professionals and patients when making decisions regarding evaluations and interventions, while optimizing resources.
Cost and access
Substantial costs are involved in the treatment of SMA, resulting from the newborn screen program implementation to the actual drug dosing, as well as costs associated with short- and long-term monitoring. The novel therapies themselves involve significant costs that are certainly restrictive in many countries. Nusinersen, the first drug approved has a cost of approximately $750,000 during the first year of treatment and $375,000 annually thereafter (32). The cost of a single life-time dose of Zolgensma® is $2.1 million. To date, over 2000 patients have been dosed worldwide (33). Lastly, risdiplam has a weight-based cost that can reach $340,000 annually (34).
Early work has evaluated the cost effectiveness of newborn screening for SMA (35). A study by Shih et al. in Australia demonstrated that newborn screening coupled with gene therapy improved the survival and quality of life for infants with SMA and was cost effective (36). However, given the high price tag and variations in the degree of improvement, particularly in advanced disease, the economic burden of treatment needs to be weighed against expected benefits.
In addition, cost of care beyond therapy administration should be considered, but long-term outcome data are very limited. A literature review estimated the annual cost of care for patients with type 1 SMA, exclusive of drug costs, to range from $75,047 to $196,429 (37). Although research in this area is limited due to the rarity of the disease, it is clear that the care of SMA patients poses a challenge for health care systems, insurance companies, and governments. Evaluating the long-term cost, benefits and burden of these interventions requires transparent data sharing among centers and health care organizations.
The role for implementation science
The application of implementation science in the field of rare diseases, including neuromuscular disorders is limited, mainly due to the lack of successful therapies. Although each disease affects a small number of patients by definition, rare diseases collectively impact more than 350 million patients globally (38). With most of these diseases caused by monogenic mutations, it is very likely that many cell and gene therapies will receive approval in the near future. Application of implementation science in SMA could serve as a model for other rare conditions that will soon have disease specific treatments.
Core concepts in implementation science include a focus on evaluating multilevel contextual factors (e.g., policy, organizational, and individual factors) that influence the implementation process, the evaluation strategies for implementing evidence-based practices, and the application of frameworks to guide the full spectrum of translational research. In particular, determinant frameworks, such as the consolidated framework for implementation research (CFIR) provide an ideal platform for the understanding of barriers and enablers that affect implementation outcomes in SMA (39, 40). The identification of specific constructs related to the intervention, in this case the diagnosis and treatment of patients with SMA, the inner and outer settings, patient characteristics and processes allows for a systematic approach to these multidimensional matters. CFIR could be used to guide the continuous improvement of newborn screening programs, both at the institutional level and state level.
Informed by the CFIR and other implementation science frameworks, a comprehensive framework has recently been proposed to address the specific needs of precision medicine implementation efforts. Given the complexity of genomic medicine and the recognition that existing frameworks may lack sufficient flexibility to address the full spectrum of clinical and research needs, the Genomic Medicine and Integrative Research (GMIR) framework was developed by the clinical sequencing evidence generating research consortium (CSER) and the Implementing Genomics in Practice (IGNITE) network, both consortia funded by the NIH (41). This framework sheds light on priority research domains and factors across multiple levels, including affected individuals, providers, health systems and the social environment, a platform that can accommodate the challenges of precision medicine (41). Although to date no framework fits the specific needs of patients with SMA, an adaptation of GMIR outlining the steps for the implementation of interventions can be used to inform the care of children with SMA (Figure 1) (41). This framework highlights the contextual factors that facilitate early diagnosis and treatment. In addition, the GMIR provides a detailed overview of the different interconnected subdomains representing the processes and outcomes related to SMA, ensuring that all aspects related to treatment implementation and sustainability are continuously assessed, including those pertinent to the patient, their caregivers and the community, in response to changes in healthcare and industry policies.
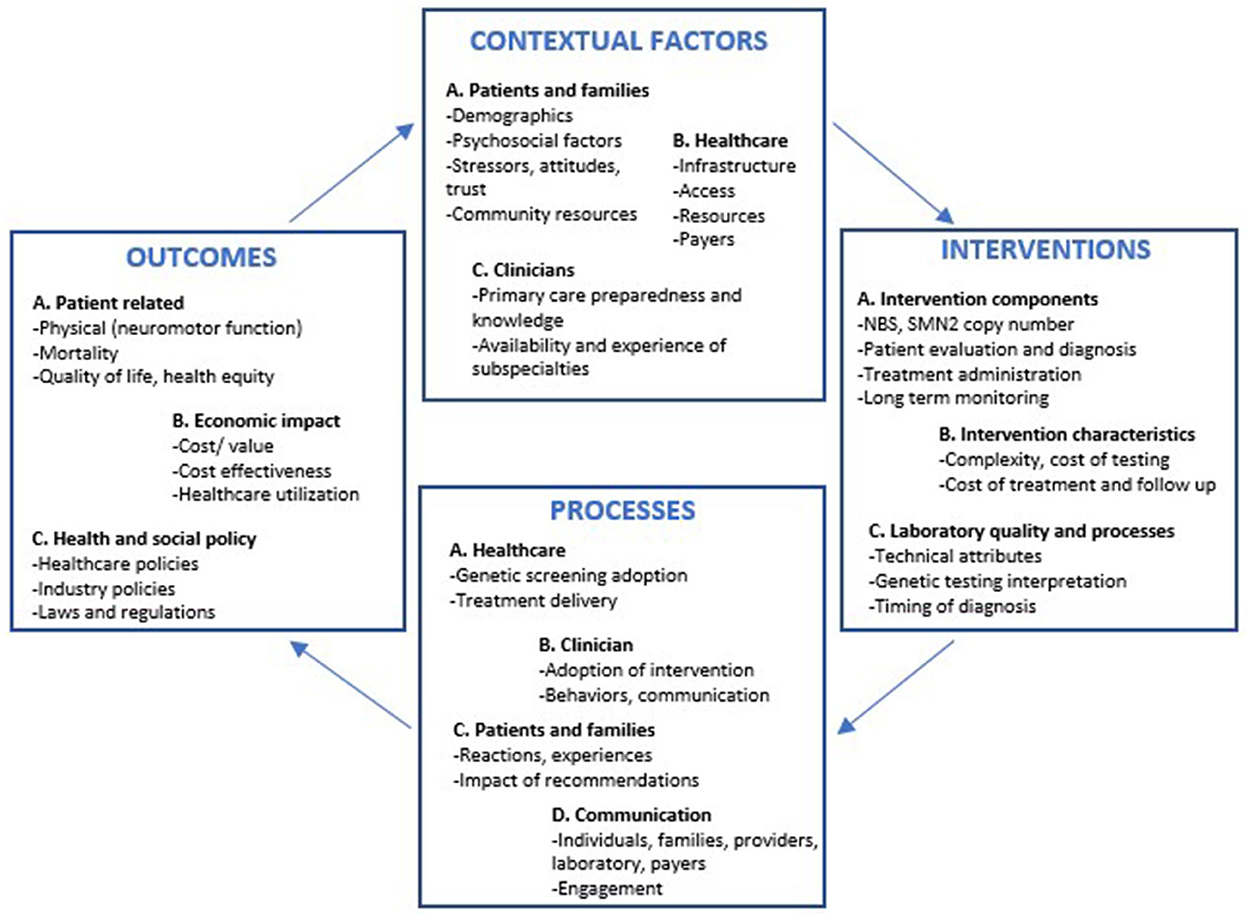
Figure 1. GMIR framework adapted for infants with spinal muscular atrophy diagnosed via newborn screening programs. NBS, Newborn screening; SMN2, Survival motor neuron 2 gene.
For example, D'Silva et al. propose a model of care for the diagnosis and delivery of Zolgensma® based on experience in Australia (42). Despite the lack of an overarching implementation science framework, specific implementation strategies are recommended to address the challenges that result from variations in diagnostic modalities, AAV9 testing, coordination of stakeholders, geographical location and workforce utilization (42).
While determinants frameworks are critical to guide the study of implementation barriers and facilitators, evaluation frameworks are necessary to measure implementation success. One such framework is the RE-AIM (reach, efficacy, adoption, implementation and maintenance) model, a widely applied framework to determine the overall impact of an intervention at the individual and population based level (43). RE-AIM can successfully serve as a tool for the evaluation of newborn screening programs and patient registries, as these interventions require the involvement of a range of organizations and personnel. If adequately performed, the application of RE-AIM and other evaluation frameworks can prove to be a powerful tool in the field of SMA and other rare diseases.
Conclusions
Although the implementation of newborn screening and the opportunity to treat minimally symptomatic infants have, without a doubt, led to improvements in the devastating outcomes of patients with SMA, variation in the execution of the diagnostic and treatment processes pose remarkable challenges. Once a tragical disease, SMA is now a chronic condition with multiple phenotypes and evolving medical needs. With the common goal to provide optimal care for patients with SMA, diagnostic and treatment centers should work together along with patients and their families, clinicians, pharmaceutical companies and governmental agencies to ensure not only timely treatment and follow up, but also the development of the processes necessary for the evaluation of outcomes beyond efficacy and safety, such as cost-effectiveness and program sustainability. These and other strategies can play a key role in facilitating the development of effective and reproducible models for the care of children with rare diseases that will benefit from similar approaches in the upcoming years. Well planned implementation strategies will also allow stakeholders to learn about additional features such as the identification of new phenotypes, detection of unknown consequences of novel therapies, comorbidities and outcomes, that would otherwise be almost impossible to evaluate given the limited number of patients, while developing cost effective protocols for state-of-the-art care and detailed long-term surveillance.
Data availability statement
The original contributions presented in the study are included in the article/supplementary material, further inquiries can be directed to the corresponding author.
Author contributions
CL-A, BB, and RS contributed to the conception and design of the work. CL-A drafted the manuscript. BB and RS performed a critical review of the manuscript. All authors reviewed and approved the final version of the manuscript.
Funding
This work was supported in part by a University of Florida Children's Miracle Network grant.
Conflict of interest
The authors declare that the research was conducted in the absence of any commercial or financial relationships that could be construed as a potential conflict of interest.
Publisher's note
All claims expressed in this article are solely those of the authors and do not necessarily represent those of their affiliated organizations, or those of the publisher, the editors and the reviewers. Any product that may be evaluated in this article, or claim that may be made by its manufacturer, is not guaranteed or endorsed by the publisher.
References
1. Kolb SJ, Coffey CS, Yankey JW, Krosschell K, Arnold WD, Rutkove SB, et al. Natural history of infantile-onset spinal muscular atrophy. Ann Neurol. (2017) 82:883–91. doi: 10.1002/ana.25101
2. Chambers DA, Feero WG, Khoury MJ. Convergence of implementation science, precision medicine, and the learning health care system: a new model for biomedical research. JAMA. (2016) 315:1941–2. doi: 10.1001/jama.2016.3867
3. Farrar MA, Vucic S, Johnston HM, du Sart D, Kiernan MC. Pathophysiological insights derived by natural history and motor function of spinal muscular atrophy. J Pediatr. (2013) 162:155–59. doi: 10.1016/j.jpeds.2012.05.067
4. Butchbach ME. Copy Number variations in the survival motor neuron genes: implications for spinal muscular atrophy and other neurodegenerative diseases. Front Mol Biosci. (2016) 3:7. doi: 10.3389/fmolb.2016.00007
5. Finkel RS, McDermott MP, Kaufmann P, Darras BT, Chung WK, Sproule DM, et al. Observational study of spinal muscular atrophy type I and implications for clinical trials. Neurology. (2014) 83:810–7. doi: 10.1212/WNL.0000000000000741
6. Kerpel-Fronius S, Baroutsou V, Becker S, Carlesi R, Collia L, Franke-Bray B, et al. Development and use of gene therapy orphan drugs-ethical needs for a broader cooperation between the pharmaceutical industry and society. Front Med (Lausanne). (2020) 7:608249. doi: 10.3389/fmed.2020.608249
7. The Orphan Drug Act Implementation and Impact. Office of Inspector General (2022). Available online at: https://oig.hhs.gov/oei/reports/oei-09-00-00380.pdf (accessed November 27, 2022).
8. Mendell JR, Al-Zaidy S, Shell R, Arnold WD, Rodino-Klapac LR, Prior TW, et al. Single-dose gene-replacement therapy for spinal muscular atrophy. N Engl J Med. (2017) 377:1713–22. doi: 10.1056/NEJMoa1706198
9. Darras BT, et al. Risdiplam-Treated Infants with Type 1 Spinal Muscular Atrophy versus Historical Controls. N Engl J Med. (2021) 385:427–35. doi: 10.1056/NEJMoa2102047
10. Finkel RS, Mercuri E, Darras BT, Connolly AM, Kuntz NL, Kirschner J, et al. Nusinersen versus sham control in infantile-onset spinal muscular atrophy. N Engl J Med. (2017) 377:1723–32. doi: 10.1056/NEJMoa1702752
11. CureSMA. Newborn screening for SMA. (2022). Available online at: https://www.curesma.org/newborn-screening-for-sma/ (accessed November 27, 2022).
12. Glascock J, Sampson J, Haidet-Phillips A, Connolly A, Darras B, Day J, et al. Treatment algorithm for infants diagnosed with spinal muscular atrophy through newborn screening. J Neuromuscul Dis. (2018) 5:145–58. doi: 10.3233/JND-180304
13. Hale K, Ojodu J, Singh, S. Landscape of Spinal muscular atrophy newborn screening in the United States: 2018–2021. Int J Neonatal Screen. (2021) 7:33. doi: 10.3390/ijns7030033
14. Hale JE, Darras BT, Swoboda KJ, Estrella E, Chen JYH, Abbott MA, et al. Massachusetts' findings from statewide newborn screening for spinal muscular atrophy. Int J Neonatal Screen 7. (2021). doi: 10.3390./ijns7020026
15. Margaretos NM, Bawa K, Engmann NJ, Chambers JD. Patients' access to rare neuromuscular disease therapies varies across US private insurers. Orphanet J Rare Dis. (2022) 17:36. doi: 10.1186/s13023-022-02182-3
16. Goedeker NL, Gibbons JL, Varadhachary AS, Connolly AM, Zaidman CM. Laboratory monitoring of nusinersen safety. Muscle Nerve. (2021) 63:902–5. doi: 10.1002/mus.27217
17. Somers E, Lees RD, Hoban K, Sleigh JN, Zhou H, Muntoni F, et al. Vascular defects and spinal cord hypoxia in spinal muscular atrophy. Ann Neurol. (2016) 79:217–30. doi: 10.1002/ana.24549
18. Kichula EA, Proud CM, Farrar MA, Kwon JM, Saito K, Desguerre I, et al. Expert recommendations and clinical considerations in the use of onasemnogene abeparvovec gene therapy for spinal muscular atrophy. Muscle Nerve. (2021) 64:413–27. doi: 10.1002/mus.27363
19. Salabarria SM, Norman SL, Coleman KE, Berthy JA, Elder ME, Leon-Astudillo CE, et al. Systemic AAV delivery activates the classical complement pathway leading to thrombotic microangiopathy (Conference presentation). American Society of Gene and Cell Therapy Conference, Virtual, United States (2021).
20. Day JW, Mendell JR, Mercuri E, Finkel RS, Strauss KA, Kleyn A, et al. Clinical trial and postmarketing safety of onasemnogene abeparvovec therapy. Drug Saf. (2021) 44:1109–19. doi: 10.1007/s40264-021-01107-6
21. Chand DH, Zaidman C, Arya K, Millner R, Farrar MA, Mackie FE, et al. Thrombotic microangiopathy following onasemnogene abeparvovec for spinal muscular atrophy: a case series. J Pediatr. (2020) 231:265–8. (2021). doi: 10.1016/j.jpeds.2020.11.054
22. Guillou J, de Pellegars A, Porcheret F, Frémeaux-Bacchi V, Allain-Launay E, Debord C, et al. Fatal thrombotic microangiopathy case following adeno-associated viral smn gene therapy. Blood Adv. (2022) 6:4266–270. doi: 10.1182/bloodadvances.2021006419
23. Information EP. Evrysdi Prescribing Information: Important Safety Information. (2020). Available online at: https://www.evrysdi-hcp.com/ (accessed November 27, 2022).
24. Sergott RC, Amorelli GM, Baranello G, Barreau E, Beres S, Kane S, et al. Risdiplam treatment has not led to retinal toxicity in patients with spinal muscular atrophy. Ann Clin Transl Neurol. (2021) 8:54–65. doi: 10.1002/acn3.51239
25. Waldrop MA, Karingada C, Storey MA, Powers B, Iammarino MA, Miller NF, et al. Gene therapy for spinal muscular atrophy: safety and early outcomes. Pediatrics. (2020) 146:729. doi: 10.1542/peds.2020-0729
26. Van Alstyne M, Tattoli I, Delestrée N, Recinos Y, Workman E, Shihabuddin LS, et al. Gain of toxic function by long-term AAV9-mediated SMN overexpression in the sensorimotor circuit. Nat Neurosci. (2021) 24:930–40. doi: 10.1038/s41593-021-00827-3
27. Hinderer C, Katz N, Buza EL, Dyer C, Goode T, Bell P, et al. Severe toxicity in nonhuman primates and piglets following high-dose intravenous administration of an adeno-associated virus vector expressing human SMN. Hum Gene Ther. (2018) 29:285–98. doi: 10.1089/hum.2018.015
28. Williamson SF, Jacko P, Villar SS, Jaki TA. Bayesian adaptive design for clinical trials in rare diseases. Comput Stat Data Anal. (2016) 113:136–53. doi: 10.1016/j.csda.2016.09.006
29. Bischof M, Lorenzi M, Lee J, Druyts E, Balijepalli C, Dabbous O, et al. Matching-adjusted indirect treatment comparison of onasemnogene abeparvovec and nusinersen for the treatment of symptomatic patients with spinal muscular atrophy type 1. Curr Med Res Opin. (2021) 37:1719–30. doi: 10.1080/03007995.2021.1947216
30. Oechsel KF, Cartwright MS. Combination therapy with onasemnogene and risdiplam in spinal muscular atrophy type 1. Muscle Nerve. (2021) 64:487–90. doi: 10.1002/mus.27375
31. van der Heul AMB, Cuppen I, Wadman RI, Asselman F, Schoenmakers MAGC, van de Woude DR, et al. Feeding and swallowing problems in infants with spinal muscular atrophy type 1: an observational study. J Neuromuscul Dis. (2020) 7:323–30. doi: 10.3233/JND-190465
32. (ICER). I.f.C.a.E.R. Spinraza and Zolgensma for spinal muscular atrophy: effectivenes and value. Inst Clin Econ Rev. (2019).
33. Novartis. New Zolgensma Data Demonstrate Age-Appropriate Development When Used Early, Real-World Benefit in Older Children and Durability 5+ Years Post-Treatment. Available online at: https://www.novartis.com/news/media-releases/new-zolgensma-data-demonstrate-age-appropriate-development-when-used-early-real-world-benefit-older-children-and-durability-5-years-post-treatment (accessed November 27, 2022).
34. Pharmaphorum. Roche Takes on Pricey Rivals as FDA Approves SMA Drug. Available online at: https://pharmaphorum.com/news/roche-takes-on-pricey-rivals-as-fda-approves-sma-drug/ (accessed November 27, 2022).
35. Jalali A, Rothwell E, Botkin JR, Anderson RA, Butterfield RJ, Nelson RE, et al. Cost-effectiveness of nusinersen and universal newborn screening for spinal muscular atrophy. J Pediatr. (2020) 227:274–80 e272. doi: 10.1016/j.jpeds.2020.07.033
36. Shih ST, Farrar MA, Wiley V, Chambers G. Newborn screening for spinal muscular atrophy with disease-modifying therapies: a cost-effectiveness analysis. J Neurol Neurosurg Psychiatry. (2021). doi: 10.1136/jnnp-2021-326344
37. Dangouloff T, Botty C, Beaudart C, Servais L, Hiligsmann M. Systematic literature review of the economic burden of spinal muscular atrophy and economic evaluations of treatments. Orphanet J Rare Dis. (2021) 16:47. doi: 10.1186/s13023-021-01695-7
38. Tambuyzer E, Vandendriessche B, Austin CP, Brooks PJ, Larsson K, Miller Needleman KI, et al. Therapies for rare diseases: therapeutic modalities, progress and challenges ahead. Nat Rev Drug Discov. (2020) 19:93–111. doi: 10.1038/s41573-019-0049-9
39. Nilsen P. Making sense of implementation theories, models and frameworks. Implement Sci. (2015) 10:53. doi: 10.1186/s13012-015-0242-0
40. Damschroder LJ, Aron DC, Keith RE, Kirsh SR, Alexander JA, Lowery JC. Fostering implementation of health services research findings into practice: a consolidated framework for advancing implementation science. Implement Sci. (2009) 4:50. doi: 10.1186/1748-5908-4-50
41. Horowitz CR, Orlando LA, Slavotinek AM, Peterson J, Angelo F, Biesecker B, et al. The genomic medicine integrative research framework: a conceptual framework for conducting genomic medicine research. Am J Hum Genet. (2019) 104:1088–96. doi: 10.1016/j.ajhg.2019.04.006
42. D'Silva AM, Holland S, Kariyawasam D, Herbert K, Barclay P, Cairns A, et al. Onasemnogene abeparvovec in spinal muscular atrophy: an Australian experience of safety and efficacy. Ann Clin Transl Neurol. (2022) 9:339–50. doi: 10.1002/acn3.51519
Keywords: spinal muscular atrophy (SMA), gene therapy, implementation science, nusinersen, risdiplam, onasemnogene-abeparvovec, newborn screening program
Citation: Leon-Astudillo C, Byrne BJ and Salloum RG (2022) Addressing the implementation gap in advanced therapeutics for spinal muscular atrophy in the era of newborn screening programs. Front. Neurol. 13:1064194. doi: 10.3389/fneur.2022.1064194
Received: 07 October 2022; Accepted: 21 November 2022;
Published: 12 December 2022.
Edited by:
Gabriele Siciliano, University of Pisa, ItalyReviewed by:
Arlene D'Silva, University of New South Wales, AustraliaCopyright © 2022 Leon-Astudillo, Byrne and Salloum. This is an open-access article distributed under the terms of the Creative Commons Attribution License (CC BY). The use, distribution or reproduction in other forums is permitted, provided the original author(s) and the copyright owner(s) are credited and that the original publication in this journal is cited, in accordance with accepted academic practice. No use, distribution or reproduction is permitted which does not comply with these terms.
*Correspondence: Carmen Leon-Astudillo, Y2xlb25hc3R1ZGlsbG9AdWZsLmVkdQ==