- 1College of Medicine and Veterinary Medicine, University of Edinburgh, Edinburgh, United Kingdom
- 2Centre for Clinical Brain Sciences, University of Edinburgh, Edinburgh, United Kingdom
Introduction: This article documents an emerging body of evidence concerning the neurological effect of polycyclic aromatic hydrocarbon (PAH) exposure with regard to cognitive function and increased risk of neurodegeneration.
Methods: Two electronic databases, PubMed and Web of Science, were systematically searched.
Results: The 37/428 studies selected included outcomes measuring cognitive function, neurobehavioral symptoms of impaired cognition, and pathologies associated with neurodegeneration from pre-natal (21/37 studies), childhood (14/37 studies), and adult (8/37 studies) PAH exposure. Sufficient evidence was found surrounding pre-natal exposure negatively impacting child intelligence, mental development, average overall development, verbal IQ, and memory; externalizing, internalizing, anxious, and depressed behaviors; and behavioral development and child attentiveness. Evidence concerning exposure during childhood and as an adult was scarce and highly heterogeneous; however, the presence of neurodegenerative biomarkers and increased concentrations of cryptic “self” antigens in serum and cerebrospinal fluid samples suggest a higher risk of neurodegenerative disease. Associations with lowered cognitive ability and impaired attentiveness were found in children and memory disturbances, specifically auditory memory, verbal learning, and general memory in adults.
Discussion: Although evidence is not yet conclusive and further research is needed, the studies included supported the hypothesis that PAH exposure negatively impacts cognitive function and increases the risk of neurodegeneration in humans, and recommends considering the introduction of a variable “rural vs. urban” as covariate for adjusting analyses, where the neurological functions affected (as result of our review) are outcome variables.
Introduction
Exposure to air pollution in the environment is now recognized globally by governments, leading research scientists, and civil society as one of the greatest public health hazards of the 21st century (1). Legislation such as “The UK National Air Quality Strategy” (2), and the European Commission's “Fourth Daughter Directive” (3) have introduced standards to monitor and limit levels of air pollutants posing the greatest risk to human health. Polycyclic aromatic hydrocarbons (PAHs) are a group of pollutants included in such legislation. PAHs are atmospheric organic compounds composed of two or more benzene rings arranged in a variety of different configurations. PAH compounds also include functional derivatives of the PAHs only containing carbon and hydrogen atoms (e.g., nitro-PAHs) and the heterocyclic analogs (e.g., aza-arenes) (4). Over 100 different PAHs were already identified by the beginning of the 21st century (4), and now the list exceeds 300, with an exact number still to be determined, as those studied are mainly selected based on the instrumentation available to each research group and reference standards (5). They are discharged from anthropogenic sources (Supplementary Figure 1), involving the incomplete combustion and pyrolysis of hydrocarbons, predominantly found in coal, oil, wood, and petrol. PAHs exist in the atmosphere in a gaseous state or are adsorbed to particulate matter. Over 80% of particulate-bound PAHs are associated with particulate matter of an aerodynamic diameter ≤ 2.5 μm (PM2.5) (6). However, a large number have been also identified in tobacco smoke (5). The study of PAHs and their impact on health has been compounded by their ubiquitousness and the numerous and widespread sources in which they can be found, some of which are also affected by other air pollutants. As PAHs are rather present as part of complex mixtures in air, water, soil, and food, their identification and characterization, for studying their effect on human health, is challenging (5).
Research surrounding PAH exposure and acute short-term health effects in humans has, thus, far focused on vulnerable individuals with pre-existing health conditions: thrombotic effects in individuals with pre-existing coronary heart disease and impaired lung function in asthma sufferers (7). Chronic long-term exposure has implicated PAH's reactive metabolites as having the ability to bind to proteins and DNA and exert carcinogenic effects (8). Such biochemical disruption and cellular damage have been most extensively researched in occupational studies, whereby high exposure has been associated with increased incidence of lung, bladder, skin, and gastrointestinal cancer (8–11). Additionally, decreased immune function, developing cataracts, and having kidney or liver damage, including jaundice, have also been associated with high exposure (5, 12). Whilst extensive research exists surrounding PAH's genotoxic and carcinogenic properties, an emerging body of evidence concerns PAH's neurotoxic effect through the induction of oxidative stress, inflammation (13), and vascular injury within the brain (14). Recently, research has emerged associating PAH exposure with impaired cognitive function and increased risk of neurodegeneration.
To the best of our knowledge, from the large body of literature on the influence of air pollution on human health, the implications of PAH exposure specifically, on cognitive function and neurodegeneration in humans, have not been systematically reviewed. Prior reviews have addressed the implications of PAH exposure on general health (15, 16) and its carcinogenic outcomes (17, 18). The reviews which have made cognitive function and neurodegeneration the outcome of interest include exposures to a vast mixture of air pollutants (19–22). Therefore, we aim to disentangle the unique neurotoxic effect of PAHs in specific age groups and cognitive-related functions to provide evidence for cognitive research and more vigilant monitoring and tighter restrictions on the main sources of emission, tailored to each age group, given the differential factors affecting the various stages of brain development. The Department for Environment, Food and Rural Affairs currently considers annual monitoring of concentrations of one PAH, benzo(a)pyrene (B(a)P), to be a sufficient representation of all atmospheric PAHs, and classifies the potential effect on human health of PAHs collectively, as six compounds, categorized as probably or possibly carcinogenic. No mention is made of the adverse neurological impact (2). A possible explanation is the consideration of concentration levels that constitute a risk for cancer, below which the effect of these pollutants can pass inadvertently. The UK national air quality objective for B(a)P is 0.25 ng m−3. However, emissions of B(a)P have been increasing since 2008 and have exceeded this limit in multiple locations at multiple time points (23). Atmospheric PAH concentrations are subject to seasonal variation and climate (24), as seen in pollution level charts that are used by studies to stratify exposure. While such stratification may add granularity to the data, it is often unrealistic given urban movement and the effect of different local government policies e.g., transportation. A more robust stratification would be to contrast urban and rural areas, where the pollution levels known to widely differ. Therefore, a further aim is to explore the difference in PAH concentration in rural vs. metropolitan areas and the influence this could have on cognitive function and neurodegeneration to inform further studies.
Methods
Eligibility criteria
This review was conducted in line with the PRISMA guidelines (25). Studies included were observational cohort studies of both male and female humans. Time of exposure was inclusive of the gestational period and stretched throughout life until death. Exposure quantification was limited to studies that measured the level of exposure to ambient PAHs or PM2.5 through environmental air sampling or spatiotemporal modeling. Measures of exposure also included concentration of PAH metabolites in urine and dosimetry of PAH-DNA adducts from DNA extracted from white blood cells. Outcomes included involved a formal assessment of cognitive function, neurobehavioral symptoms of impaired cognition, and pathologies associated with neurodegeneration. Reports were limited to published scientific articles written in the English language. No publication dates were imposed. Studies were excluded if they did not fulfill the inclusion criteria, were not in humans, or where PAH exposure was measured as a component of the diet, environmental tobacco smoke (ETS), or traffic related air pollution (TRAP). Exposure through diet and ETS is not an appropriate representation of a major source of atmospheric PAH that can be geographically differential (i.e., in terms of urban vs. rural areas) or influential in both short- and long-term exposure. Moreover, prior research has elucidated contaminating pollutants within TRAP composition detrimentally affecting cognitive function, and the effect of diet-related benzo[a]pyrene, dibenz[a,h]anthracene, and benzo[h]fluoanthracene in human health and cognition (e.g., learning and memory functions). The inclusion of such studies would confound results and prevent us from elucidating the specific impact of ambient PAH on cognition.
Information sources
Studies were identified by searching electronic databases PubMed (1984–2021) and Web of Science (1979–2021). Given the environmental changes seen as the consequence of lockdown policies and movement restrictions mainly in the period April 2020 to December 2021, publications that reflected or analyzed the environmental effect of this “abnormal” period were excluded. “Polycyclic aromatic hydrocarbons” in addition to the following search terms: “brain,” “neurological,” “cognitive,” “cognition,” “neurodegenerative,” “neurodegeneration,” “neurodevelopment,” and “neurodevelopmental” were used to identify articles in both databases. Limitations applied to the search included only the fields “Title” and “Abstract” being searched. In Web of Science, the document type “Articles” was applied. In PubMed, an additional limitation of species, “Humans,” was applied. Eligibility assessment was performed independently in an unbiased standardized manner by one reviewer. Ambiguity concerning the inclusion or exclusion of a study was resolved by a second reviewer being consulted and a consensus taken. Initial screening was performed by reviewing the title and abstract, after which, the full manuscript was reviewed.
Data collection process
A data extraction sheet was developed and pilot tested on five randomly selected included studies, before being refined accordingly. One review author extracted data from the included studies, a second was consulted where ambiguity arose surrounding the appropriate data to extract. One author was contacted through email to provide numerical data that had only been presented graphically. Information extracted from studies comprised sample size, sample characteristics, ratio between sexes, mean age, age range, comorbidities, air pollution component, time of exposure, air pollution data acquisition method, and outcome measure.
Risk of bias in individual studies
The risk of bias was assessed in line with the QUADAS1 guidelines (University of Bristol, 2003). To ascertain the risk of bias within each study included, one reviewer working independently extracted the following information: participant inclusion/exclusion criteria explained, participant withdrawals from the study explained, use of/comparison with a control/low exposure population, confounding variables identified, appropriate method/analysis to adjust for confounding variables, outcome assessors aware of exposure status of study participant, intermediate or unexpected results explained/reported, and whether or not the methods of the study were reproducible.
Methods of analysis
Studies included were divided into four subgroups depending on the time at which the exposure was measured: pre-natal, childhood, adult, and finally childhood + adult. The category childhood + adult included studies of young individuals from a wide age range, some exposed during childhood, and others where exposure extended through to adulthood, not analyzed separately. Subsequently, studies were further divided into categories depending on the outcome measured: cognitive abilities, neurobehavioral development, or neurodegeneration. This subgroup division was conducted to adjust for heterogeneity between studies. The meta-analysis was performed by extracting odds ratios and 95% confidence intervals (CI) for the effect sizes of reported outcomes or calculating them from the parameters and data given in the original publications using the Practical Meta-Analysis Effect Size Calculator by David B. Wilson, from (https://www.campbellcollaboration.org/escalc/html/EffectSizeCalculator-OR-main.php). Results were double-checked using the following online resources: (https://www.gigacalculator.com/calculators/odds-ratio-calculator.php) and effect size converter (https://www.escal.site/). Forest plots were used to visualize differences in effect sizes between studies within the same subgroup.
Results
Study selection
The search of Web of Science and PubMed provided a total of 490 citations. After adjusting for duplicates, 428 remained. Subsequent screening of the title and abstract resulted in a further 289 being discarded. Of the remaining 139, a further 102 were excluded upon further examination of the full manuscript and application of inclusion criteria. One study (26) reported data from a previous study (27). Sixteen were reviews and did not include any primary data, 47 reported outcomes not relevant to cognitive function or neurodegeneration, and in 38 the recorded exposure to PAH was not in keeping with the specified criteria, resulting in a total of 37 studies included in this review. Subgroup division resulted in 21 pre-natal exposure studies, 15 concerning cognitive development outcomes, and 13 on neurobehavioral development, seven included measures of both. From 11 childhood exposure studies, three were on general cognition, five on neurobehavioral development, and three on neurodegeneration. There were also five adult exposure studies all with outcomes of general cognition, and three childhood + adult studies all with measures of neurodegeneration. One study included measured outcomes for both pre-natal and childhood exposure (28) and two studies involved two different study cohorts: one exposed only during childhood and the other included a mix of childhood and adult-exposed subjects (29, 30). Figure 1 depicts the flow chart for study inclusion and subgroup division. The full dataset can be found at the following link: https://doi.org/10.7488/ds/3031.
Study characteristics
The 37 studies involved populations from nine countries (Figure 2). Study population characteristics including sample size and mean age (±SD) are displayed in Figure 3.
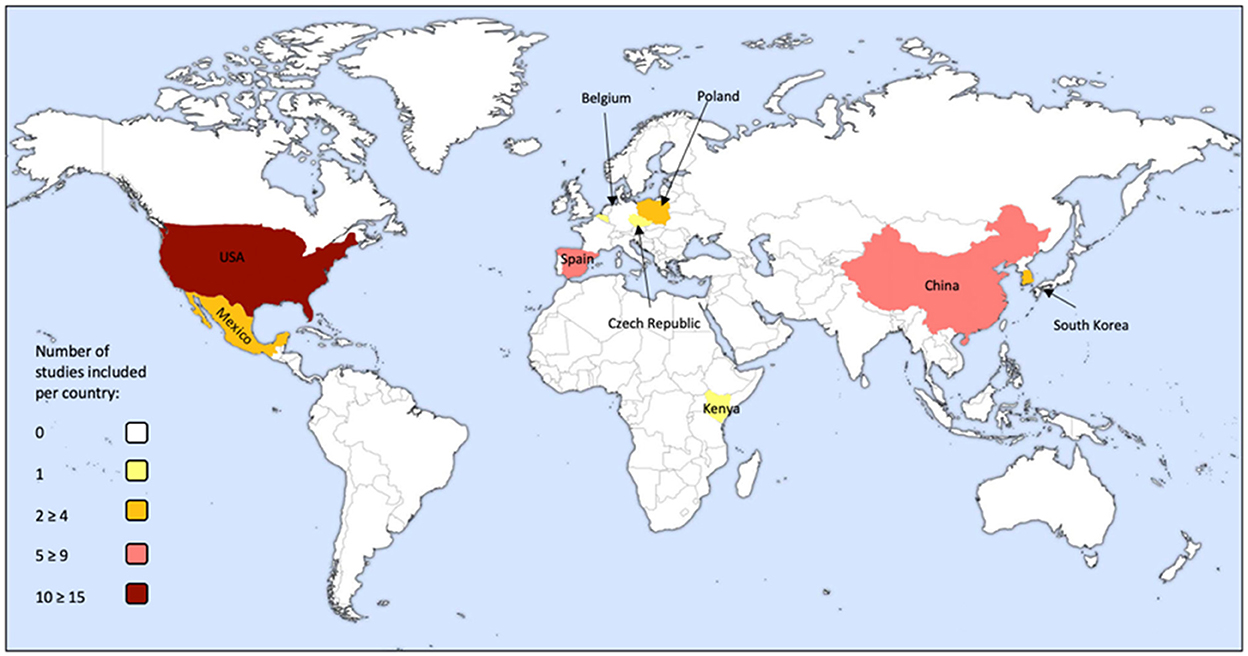
Figure 2. Global distribution of the population cohorts in each of the 37 studies included in this review (figure made using: Biorender.com).
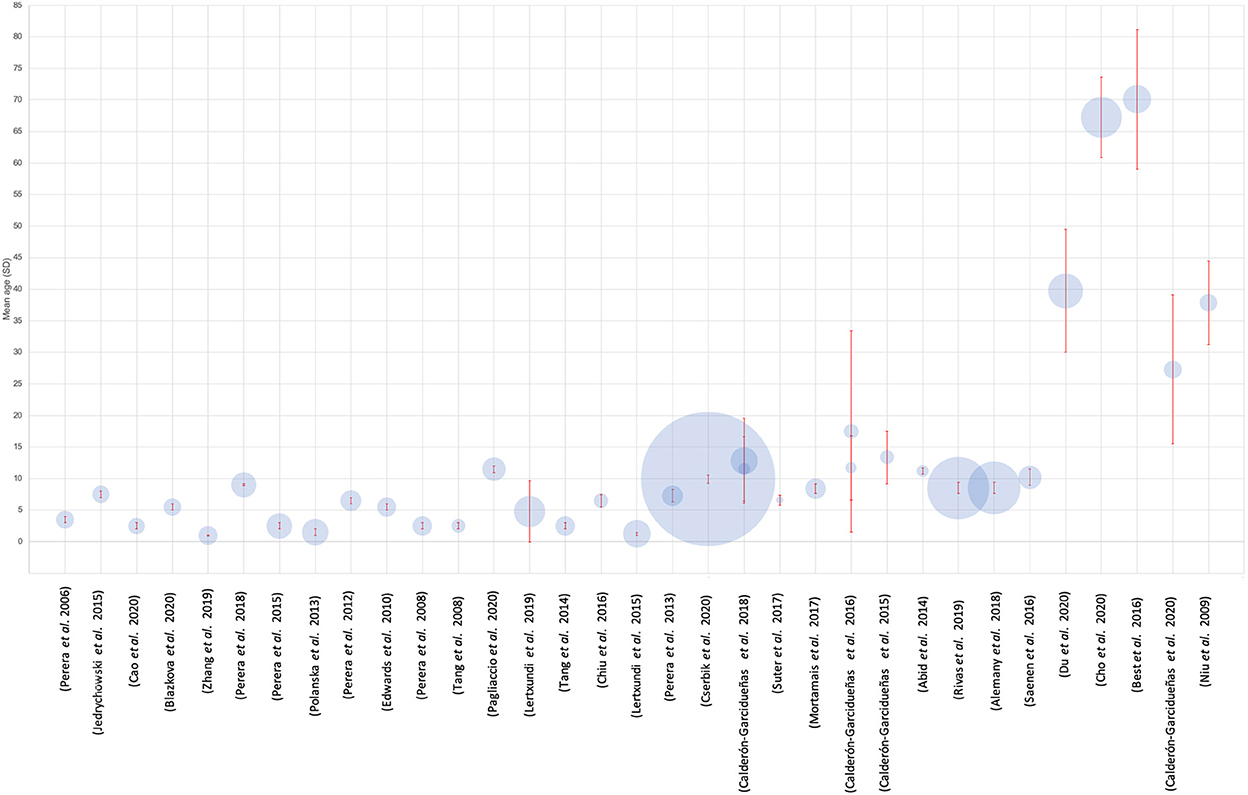
Figure 3. Characteristics of the study population involved in each study. Circle size is representative of the sample size. Red bars indicate mean age and SD. Four studies were omitted from this analysis due to insufficient data (28, 34, 43, 60).
Eleven studies included cohorts from the USA. Six of them selected participants from the Columbia Center for Children's Environmental Health cohort; however, each study selected different subgroups of the population and measured different outcomes (31–36). Two studies selected a subgroup of participants from the National Health and Nutrition Examination Survey 2001–2002 (NHANES) (37), one of which included additional participants from the NHANES 2003–2004 cohort (38). The remaining three studies involved cohorts from the Childhood Autism Risks from Genetics and the Environment Study (28), the Adolescent Brain Cognitive Development Study (39), and the Asthma Coalition on Community, Environment and Social Stress project (40). Eight studies reported results from populations in China. One study involved a Taiyuan population (41) in addition to two selecting different subgroups from the Taiyuan Mother and Child Cohort Study (42, 43). Three involved populations were from Tongliang (27, 44, 45), and the remaining two were from Shanxi province (46) and Qingdao City (47).
Five studies involved populations from Spain. Two involved a subgroup from the Infancia y Medio Ambiente Project (48, 49) and three studies from the Brain Development and Air Pollution Ultrafine Particles in School Children project (50–52).
Four studies reported on populations in Poland, three including participants from the Krakow Study (53–55) and one from the Polish Mother and Child Cohort Study (56). Four studies reported on populations in Mexico. All involved Mexico City residents (57), where two refer to six Mexican cities (29, 58), and another included details of three small Mexican cities (30). Two studies involved a Korean population (59, 60). Further individual studies included populations from the Czech Republic (61), Kenya (62), and Belgium (63).
Exposure assessment
Of the 37 studies included, seven measured exposure through environmental PAH sampling, five by environmental PM2.5 sampling, seven by PM2.5 spatiotemporal modeling, 10 by concentrations of PAH metabolites in urine, and eight using dosimetry to measure PAH-DNA adducts (Figure 4).
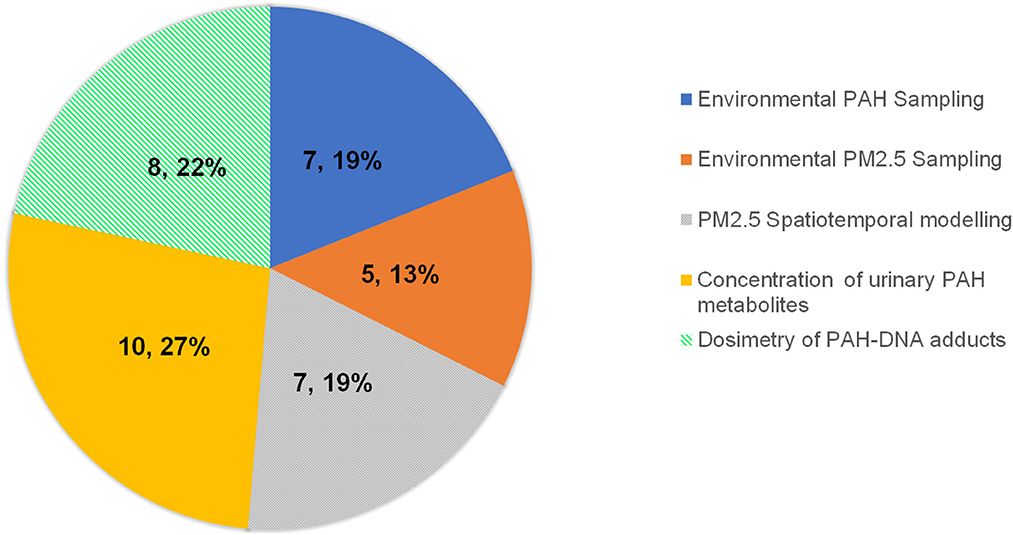
Figure 4. Pie chart representing the proportion of included studies measuring exposure to PAH as a measure of environmental PAH sampling, environmental PM2.5 sampling, PM2.5 spatiotemporal modeling, concentration of urinary PAH metabolites, and dosimetry of PAH-DNA adducts.
Outcome assessments
Outcomes included 21 different tests measuring cognitive function, nine different tests measuring neurobehavioral symptoms of impaired cognition, and three different measures of pathologies associated with neurodegeneration (Figure 5).
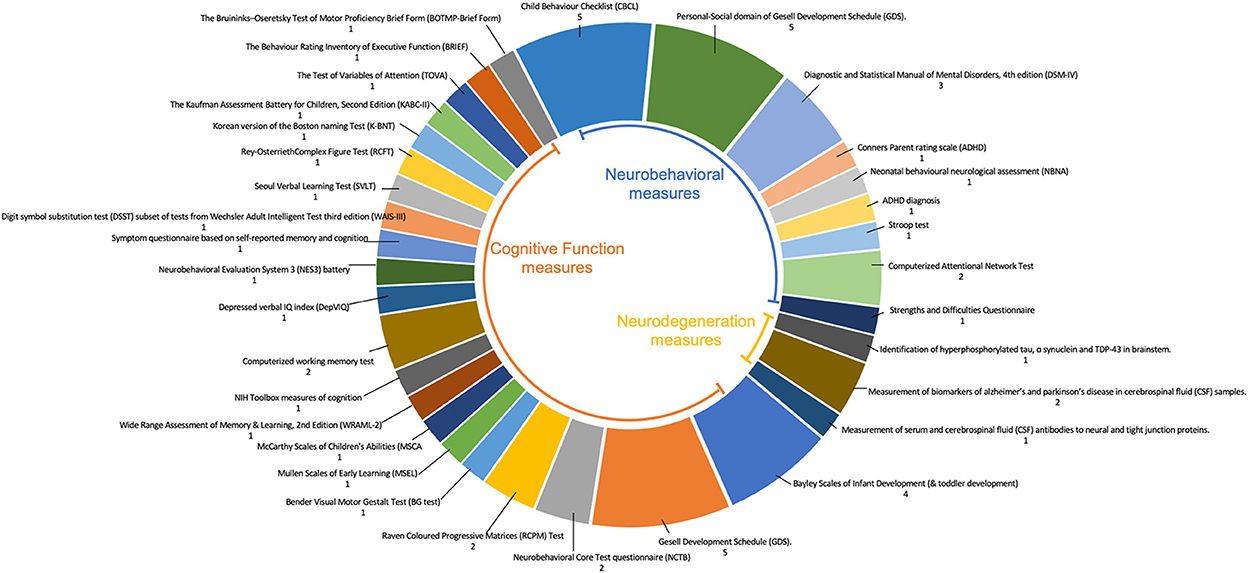
Figure 5. Pie chart representing the number of studies using different tests to measure outcomes. Measures include cognitive function, neurobehavioral symptoms of impaired cognition, and pathologies associated with neurodegeneration.
Pre-natal exposure
Association between pre-natal PAH exposure and cognitive abilities in childhood
Children with a high pre-natal PAH exposure were found to have a delay in overall child intelligence [OR = 1.75, 95% CI, 1.11–2.71) (54), mental development [OR = 0.65 (32)], and average overall development (27, 44) (OR = 0.84, 95% CI, 0.52–1.36; OR = 1.85, 95% CI, 1.13–3.01, respectively). Specifically, the greatest negative effects reported were in verbal IQ (OR = 3.45, 95% CI, 0.95–12.49) (53) and language (OR = 5.99, 95% CI, 1.88–19.02) (47). However, the latter could not be confirmed in five out of six studies (27, 42, 44, 45, 56). Two studies analyzed the effect of PAH on general cognitive abilities with contradictory results: one (31) reported a negative effect (OR = 2.89, 95% CI, 1.33–6.25) while another (56) reported no effect. PAH effect on impaired motor development was inconclusive, as confirmed by four studies (27, 42, 44, 45) (OR = 0.95, 95% CI, 0.58–1.53; OR = 1.91, 95% CI, 1.22–2.97; OR = 1.63, 95% CI, 1.00–2.65; OR = 1.82, 95% CI, 3.21–1.03, respectively), whereas three others could not confirm it. No association was found between PAH exposure and developmental motor ability (56), fine and gross motor abilities (47), and psychomotor abilities (31). Only one study reported the effect of PAH and reduced adaptive development (27) (OR = 1.77, 95% CI, 1.09–2.88) while four out of five studies reported no association with adaptive domains (42, 44, 45, 47). Size effects reported by the studies mentioned are graphically represented in Figure 6 and listed in Table 1.
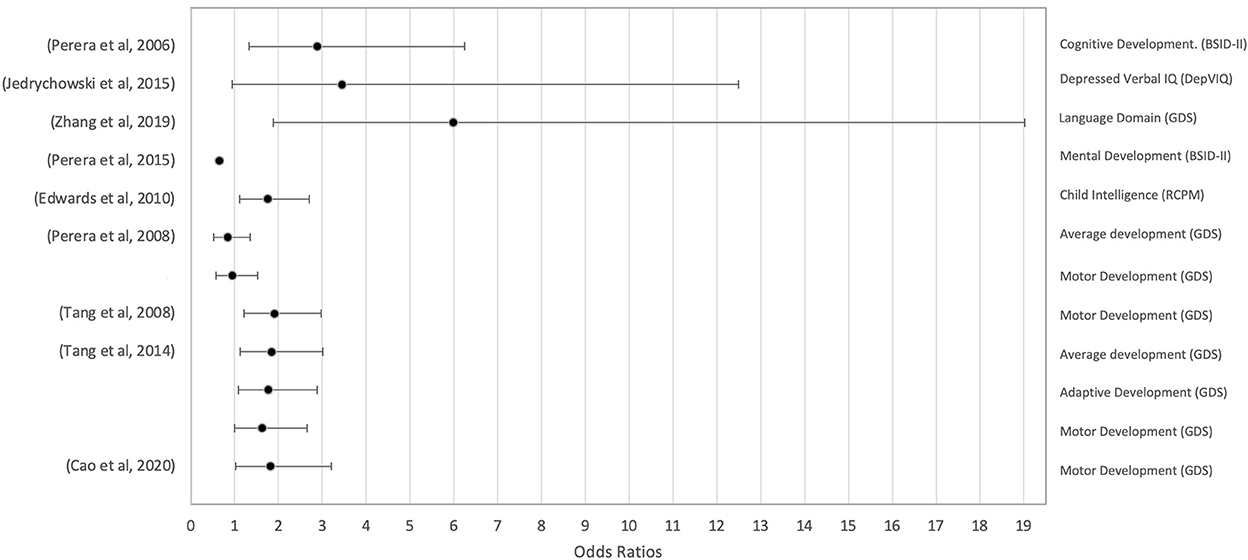
Figure 6. Forest plot of the calculated odds ratios (OR) and 95% confidence intervals (95% CI) for the association between pre-natal exposure to PAH and measures of cognitive development. Bayley Scales of Infant Development—revised (BSID-II), Depressed Verbal IQ Index (DepVIQ), Gesell Development Schedule (GDS), Raven Colored Progressive Matrices Test (RCPM), Wechsler Intelligence Scale for Children IV (WISC-IV). One study had insufficient data to calculate 95% CI (32).
Association between pre-natal PAH exposure and neurobehavioral development
Children with a high pre-natal PAH exposure were found to exhibit externalizing and internalizing behavioral problems (OR = 2.49, 95% CI, 1.57–3.95; OR = 2.39, 95% CI, 1.51–3.79, respectively) (55), and infants exhibited a decrease in behavioral development (OR = 2, 95% CI, 1.27–3.15) (43). Associations with anxious/depressed behavior were found in three out of four studies (33, 34, 55) (OR = 8.89, 95% CI, 1.7–46.51; OR = 8.14, 95% CI, 1.21–54.94; OR = 1.7, 95% CI, 1.08–2.68, respectively), with no association found by one study (36). Three out of five studies reported a negative effect on children's attentiveness (33, 35, 36) (OR = 1.34, 95% CI, 0.85–1.83; OR = 2.02, 95%, 1.35–3.03; OR = 3.79, 95% CI, 1.14–12.66) whilst two (34, 55) reported no effect. The report from one study (55) about the effect of both withdrawn/depressed and aggressive behavior (OR = 2, 95% CI, 1.27–3.16; OR = 2.29, 95% CI, 1.45–3.62, respectively) was contradicted by another study (36) that reported no effect for either. The latter (36) did, however, report the effect of impaired thought problems (OR = 1.95, 95% CI, 1.3–2.91) which was contradicted by the former (55). Only one out of seven studies reported an association between PAH and social problems (55) (OR = 1.57, 95% CI, 1.00–2.48), and the remaining six reported no effect (27, 36, 42, 44, 45, 47). Two studies (36, 55) found no effect on rule breaking behavior or somatic complaints. One study (35) reported no associations with attention deficit hyperactivity disorder (ADHD) index scores or hyperactive compulsive behavior, nor did another from the same research group (31) regarding total behavioral problems. Studies reporting neurobehavioral effects are reported in Table 2, and effect sizes are depicted in Figure 7.
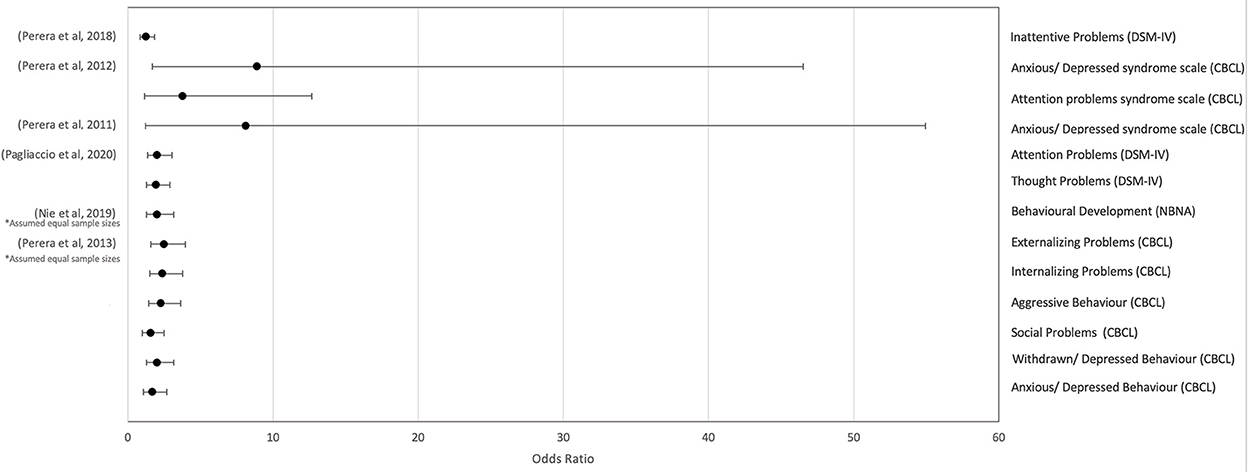
Figure 7. Forest plot of the calculated odds ratios (OR) and 95% confidence intervals (95% CI) for the association between pre-natal exposure to PAH and measures of neurobehavioral development. Gesell Development Schedule, Diagnostic (GDS) and Statistical Manual of Mental Disorders, 4th Edition (DSM-IV), Child Behavior Checklist (CBCL), Neonatal Behavioral Neurological Assessment (NBNA). *Sample sizes were assumed equal.
Association between pre-natal PM2.5 exposure and cognitive abilities and neurobehavioral development in childhood
A study (40) examined high PM2.5 exposure during early, mid, and late pregnancy with measures of full-scale IQ score, inattentiveness, and adverse memory performance. Boys highly exposed during late pregnancy exhibit lower IQ and inattentiveness when exposure was from mid to late pregnancy. Girls highly exposed during early to mid pregnancy exhibited adverse memory performance. No effect was reported for the remaining domains analyzed by this study (40).
The finding of impaired motor development (48) was not supported by a subsequent study conducted by the same group (49), which reported, however, impaired memory in boys (49). From studies analyzing the impact of pre-natal PM2.5 exposure on cognition and neurobehavioral development (Table 3), no effect was found on visual-motor functioning (61), general cognitive ability (28, 49), mental status (48), non-verbal intelligence (61), adaptive function or autism spectrum disorder (28), nor on verbal, perceptive manipulative, and numeric development (49).
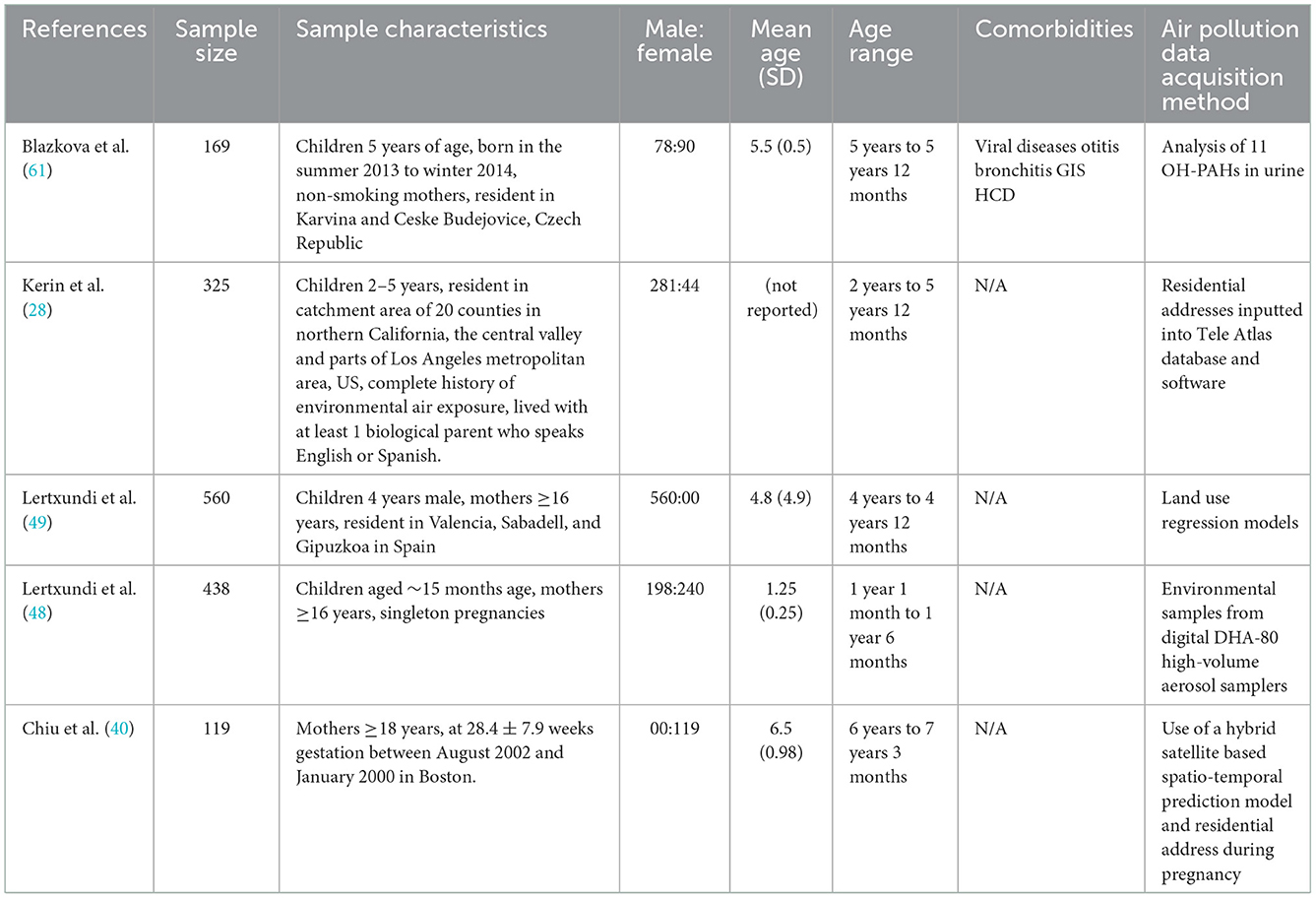
Table 3. Studies with measured pre-natal PM2.5 exposure on cognitive abilities and neurobehavioral development in childhood.
Childhood
Association between childhood PAH exposure and cognitive abilities and neurobehavioral development
Children exposed to high levels of PAH post-natally exhibited lower general cognitive ability and delayed impaired memory (62). Increased inattentiveness was reported by two studies (50, 62), but this finding was contradicted by one study (51). The negative effect of post-natal PAH exposure was not observed in all memory domains. A study (50) found an association between impaired working numeric memory but not on working verbal memory. Short-term memory was not found affected either (62).
The association with ADHD diagnosis reported by one study (38) was not supported by two other studies (50, 51). Neither study found an effect on learning performance (38, 62) or an association with visual spatial skills, non-verbal test performance, executive function, motor performance (62), or behavioral problems (50). Studies reporting childhood PAH exposure can be found in Table 4.
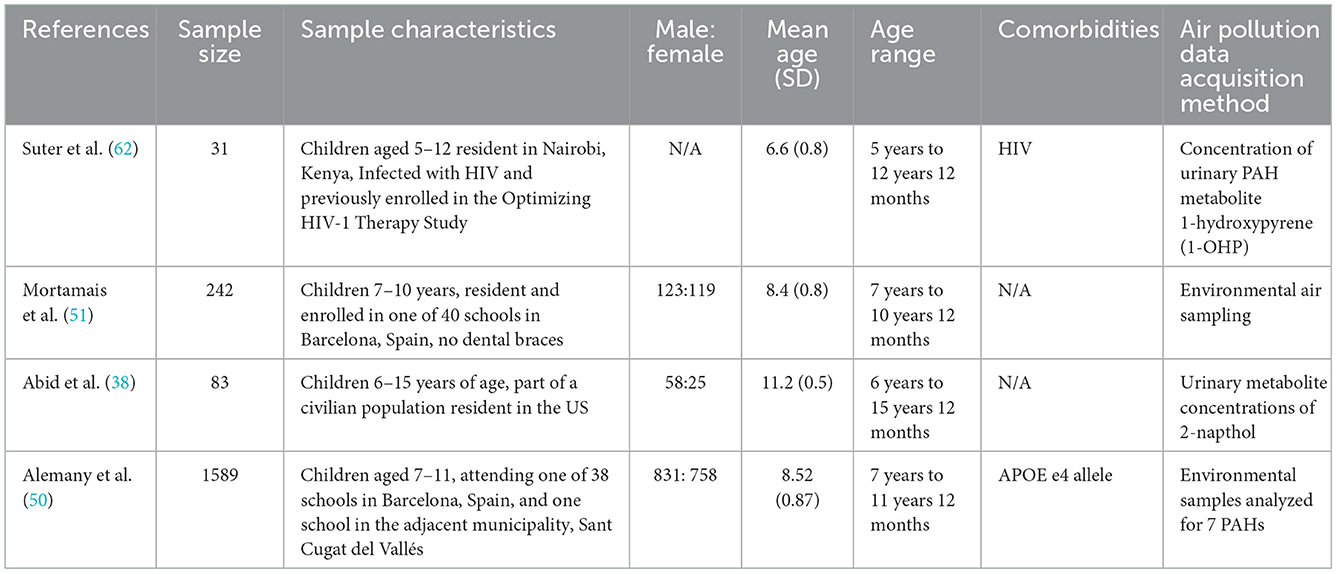
Table 4. Studies with measured childhood PAH exposure on cognitive abilities and neurobehavioral development.
Association between childhood PM2.5 exposure and cognitive abilities and neurobehavioral development
From the four studies reporting post-natal PM2.5 exposure on general cognition and neurobehavior (Table 5), one reported that children exposed to high levels of PM2.5 post-natally displayed impaired selective and sustained attention (63), but this finding was contradicted by two studies (39, 52) reporting no effect on inattentiveness or attention and executive function, respectively. A study's report of impaired visual information processing speed (63) was again contradicted by another (39) reporting no association with processing speed. No association was found with working memory (39, 52), episodic memory, language (39), cognitive ability, adaptive function, or autism spectrum disorder (28).
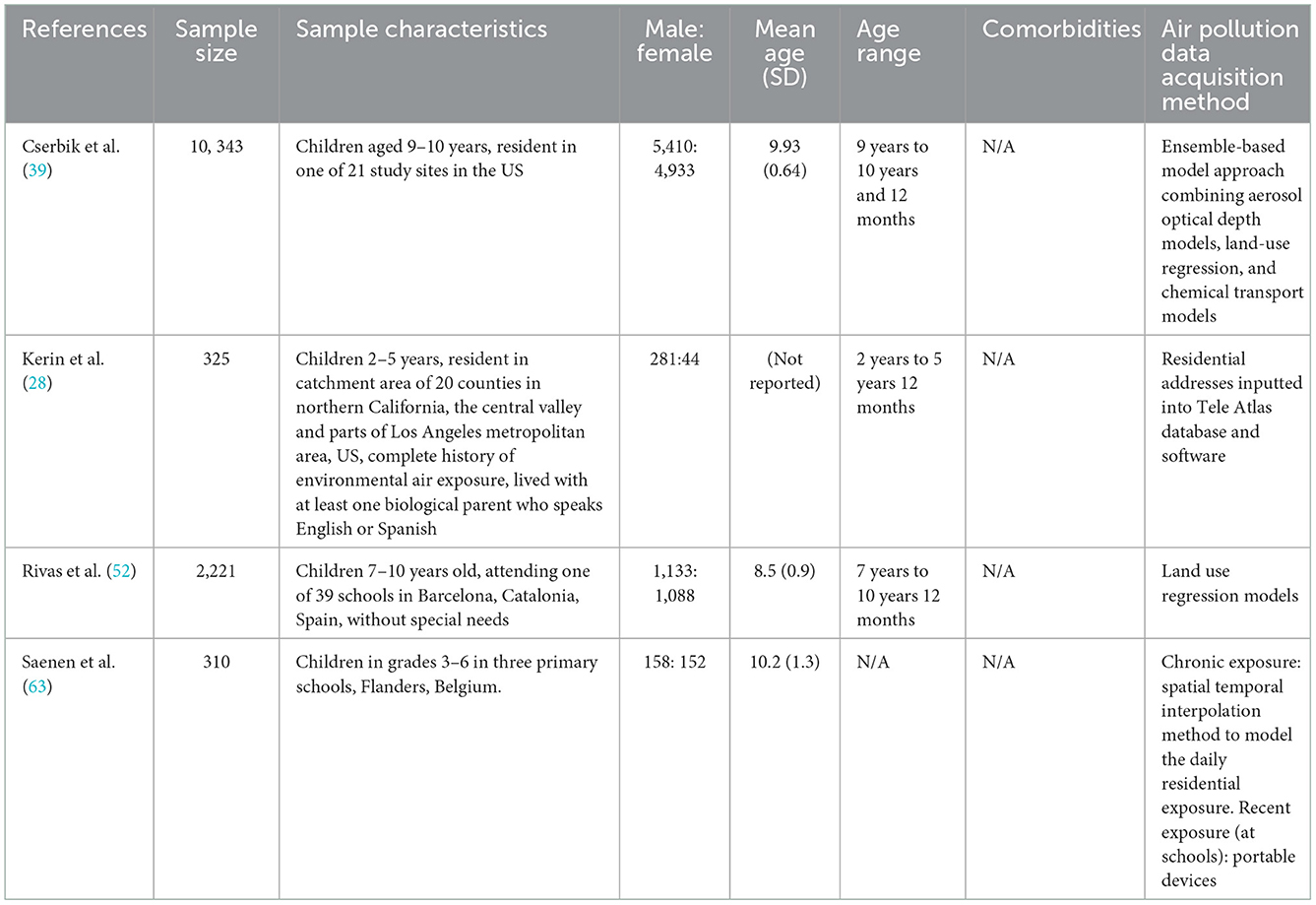
Table 5. Studies with measured childhood PM2.5 exposure on cognitive abilities and neurobehavioral development.
Association between childhood PM2.5 exposure and neurodegeneration
Only three studies by the same research group analyzed post-natal PM2.5 exposure to neurodegeneration (Table 6). Two of them found that children highly exposed to PM2.5 post-natally exhibited lower amyloid beta protein fragment 1–42 (Aβ1 − 42) and brain-derived neurotrophic factor (BDNF) (29, 30), with one finding, in addition, higher interferon (IFN) γ concentrations in cerebrospinal fluid (CSF) (30). No effect was found with regard to concentrations of biomarkers: non-phosphorylated tau (non-p-tau), vitamin D, tau phosphorylated at threonine 181 (30), cellular prion protein, total tau, interleukin (IL) β, leptin (29, 30), total alpha- synuclein (α-synuclein), oligodendrocyte α-synuclein, hyperphosphorylated tau, tumor necrosis factor alpha, IL 2, IL 6, IL 10, or monocyte chemoattractant protein-1 (MCP-1) (29). Of 33 antibodies to neural and tight junction proteins, actin immunoglobulin G (IgG), occludin/zonulin (OZ) immunoglobulin A (IgA), OZ IgG, myelin oligodendrocyte glycoprotein (MOG) IgG, MOG immunoglobulin M (IgM), myelin basic protein (MBP) IgA, MBP IgG, astrocytic protein (S-100) IgG, S-100 IgM and cerebellar antigen (CEREB) IgG in serum, and MBP antibodies in CSF were higher in children exposed to high levels of PAH compared to controls (58).
Adult
Association between adult PAH exposure and cognitive abilities
Adults highly exposed to PAH exhibited impaired auditory memory (41, 46), individual accounts of memory disturbances (60), and impaired verbal learning and memory (59). However, there was no effect on working memory and executive function, visuospatial memory/attention and planning (59), or visual perception memory (41, 46).
There was one account of impaired cognitive disturbances (60), which was contradicted by two reports of no association with cognitive dysfunction (37, 59) and by additional individual accounts of no effect in approximate number system functioning (41) (i.e., digit span, digit symbol, number of dots tests), confrontational word retrieval, verbal fluency, delayed reaction time between congruent and incongruent stimuli, visual attention, and task switching (59). Mood state, attention/response speed, manual dexterity, or perceptual motor speed were not found associated with PAH exposure in adulthood (41, 46). However, it must be noted that two investigations were population studies (37, 59), while the other three (41, 46, 60) were occupational health studies on brain effects of PAHs in coke ovens (41, 46) or oil spill (60) workers, who are exposed to very high levels of PAHs, especially high molecular weight compounds including benzo(a)pyrene and other compounds with five to six or more hydrocarbon rings. A summary of the population characteristics of the five studies exploring adult PAH exposure and general cognition can be found in Table 7.
Childhood and adult
Association between childhood and adult PM2.5 exposure and neurodegeneration
Details from the three studies reporting on cohorts inclusive of participants exposed to PM2.5 only during childhood and some participants through to adulthood can be found in Table 8. In a cohort of mixed exposure to PM2.5, the presence of neurodegenerative biomarkers phosphorylated tau (p-tau), α-synuclein, and transactive response DNA-binding protein 43 (TDP-43) was confirmed in brainstems (57). The faster increase in concentrations with regard to the age of non-p-tau in CSF was also associated with increased exposure (30). However, no association was found with regard to the concentration of total and oligomer α-synuclein in CSF (29).
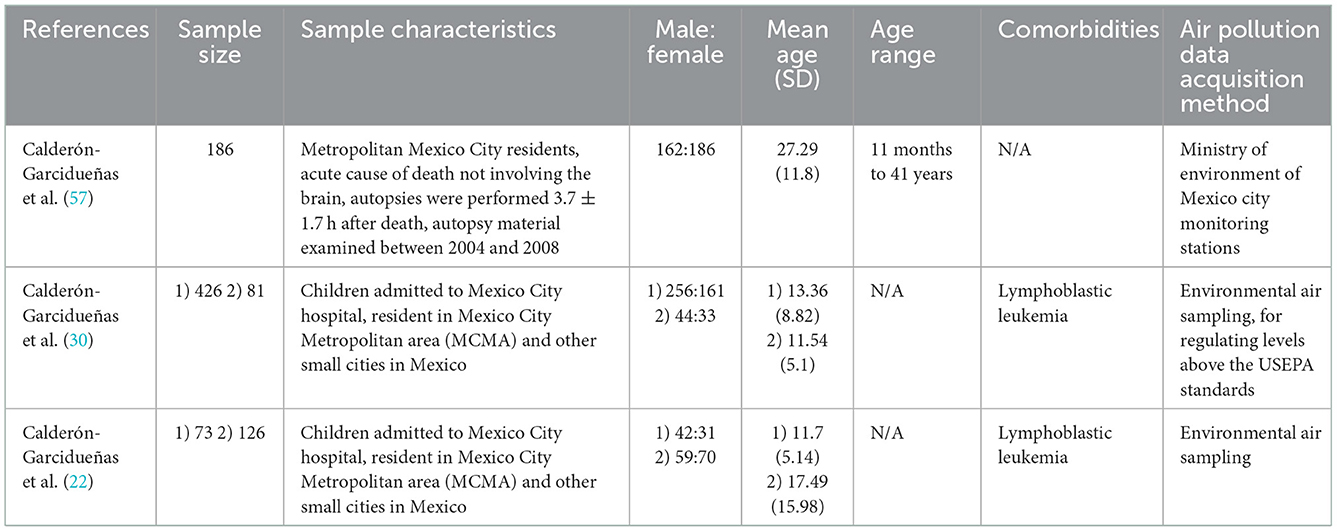
Table 8. Studies with measured cohorts inclusive of childhood and adult PM2.5 exposure on neurodegeneration.
Risk of bias within studies
All studies included were of high quality with reproducible accounts of the method employed to assess relevant outcomes, and the inclusion/exclusion criteria used to select the study population were explained in sufficient detail (refer to detailed QUADAS tool responses in the publicly available data at https://datashare.ed.ac.uk/handle/10283/3892). Where applicable, all studies provided explanations for participant withdrawal, which were unrelated to both the exposure and the outcome being measured and reported intermediate or unexpected results. Approximately 54% of studies involved the use of a comparison with a low exposure or control population either by dichotomizing exposure data or using a demographically matched control population. The remaining 46% of studies assessed PAH exposure as a continuous variable. All studies correctly identified confounding variables, and the method and analysis were adjusted accordingly. There was, however, a considerable risk of information bias amongst studies, with only 16% of studies reporting the outcome assessor to be blinded and unaware of the exposure status of the study participant. Seventy-three percent of the studies provided no indication as to whether they were or not blinded, and in 11% the outcome assessors were confirmed not blinded.
Discussion
This review found sufficient evidence that pre-natal PAH exposure negatively impacts cognitive function with specific regard to child intelligence, mental development, verbal IQ, memory impairment, average overall development, child attentiveness, behavioral development, and externalizing, internalizing, anxious, and depressed behavioral problems.
Evidence concerning exposure during childhood and as an adult with cognitive function was insufficient to conduct a meta-analysis, due to a reduced number of studies, low consistency, and high heterogeneity in results. However, associations can be observed such as exposure during childhood with lowered cognitive ability, impaired child attentiveness, and exposure as an adult manifesting in memory disturbances with specific regard to auditory memory and verbal learning and memory.
Studies concerning PAH exposure during childhood and as an adult were scarce, but an increased risk of neurodegeneration was found through the presence of neurodegenerative biomarkers and increased concentrations of cryptic “self” antigens in serum and CSF, indicative of the neuroinflammatory pathology which precedes Alzheimer's disease (AD) and Parkinson's disease (PD).
It is known that some pathways of aryl-hydrocarbon neurotoxicity are common for PAHs, TCDD, dioxin-like agents, polyphenols, and similar xenobiotics. A review of the neuropathological mechanisms of PAHs highlights that these, together with their metabolites, may cross the blood–brain barrier causing neurological abnormalities that may include neuronal damage, impaired neurotransmitter regulation, parasympathetic dysregulation, and neurodegeneration (65). Preclinical studies hint at a common neuropathological mechanism of PAH action being the binding of these compounds to the aryl-hydrocarbon receptor (AhR), a cytosolic transcription factor that initiates a complex pathway leading to alteration of gene regulation. AhR is also present in neural cells and can be involved in the mechanisms leading to PAH-induced neurological disorders (65).
This review differentially addressed the neurological impact of PAHs in three different domains, namely, cognitive abilities, neurobehavioral development, and neurodegeneration, and can be used as evidence for policy surrounding the monitoring of PAHs specifically. In addition, it raises awareness of the potentially confounding effect that different ambient PAH concentrations, in metropolitan and rural settings, can have on research assessing outcomes concerned with cognitive function and neurodegeneration in studies. It was not possible, however, to conclude on the differential impact of PAHs acquired mainly from outdoor sources from those acquired from indoor sources.
A previous review on the impact of PM2.5 in disease incidence did not stratify patients by age nor considered differences between urban and rural areas, rather stratifying studies by the pollution level in which the country was considered (i.e., “lightly polluted” vs. “heavily polluted”) (64). Other reviews have highlighted general adverse health conditions such as chronic asthma, increased incidence of premature death and hospital admissions (15), and kidney and liver damage (16). Some focused specifically on the carcinogenic properties and resulting incidence of the lung (17), urinary tract (18), and skin and gastrointestinal tract cancers (16). Those that focused on the neurological impact of air pollution concerned a diverse mixture of compounds. One specifically focused on non-communicable diseases and the roles of nitrogen dioxide (NO2), nitrogen oxide species (NOx), carbon monoxide (CO), and PM2.5 (19). Another (20) raises awareness concerning ambient pollution's adverse effect on cognitive decline and impairment, concurring with findings from (22), where the emphasis was on ozone, PM2.5, and PM10. A study (21) reported NO2, NOx, black carbon, and PMs as potential risk factors for AD, PD, and multiple sclerosis. Despite the outcomes assessed being orientated toward neurological health, the exposures measured either include multiple pollutants or compounds NO2, NOx, CO, PM, or black carbon, around which extensive research already exists and has culminated in tight air quality restrictions and monitoring, which is closely adhered to by governing bodies. This review raises awareness of the neurological impact PAH has, independent of other pollutants, the importance of which is paramount with the current health impacts of PAHs in the UK air quality strategy detailed as “possibly” or “probably” carcinogenic, detracting from the seriousness of their impact on neurological human health (2). This review proceeds to categorize outcomes into subgroups depending on the time of exposure to provide further insight into the demographics of the individuals most vulnerable to the pollution levels reported and to differentiate between the areas of cognitive function and neurodegeneration most impacted, elucidating the potential mechanisms of neurotoxicity. The observation that the most profound effect of PAH exposure culminates from the pre-natal period is in keeping with prior research, showing the fetal brain to be more vulnerable to environmental toxic insult than the adult. The increased permeability of the not yet fully formed blood–brain barrier combined with the rapid brain growth during the second trimester means the period of most intense construction and brain architecture is also the time the brain is most vulnerable to the passage of toxins and neurotoxicity (66). Overall, this review has systematically located, summarized, and meta-analyzed evidence about the potent neurotoxicity of direct or indirect exposure to PAHs across the human lifespan, highlighting the need for more well-designed epidemiological studies.
Limitations
Studies included in the analysis were limited to those written in the English language. Publication bias and selective reporting within studies cannot be discarded, nor can indexing issues, in which the search terms may have failed to identify relevant studies.
The study populations included only originated from nine countries, of which the UK was not one. Findings are therefore limited to the environments and seasonal variations in climate found in these countries, and no specific recommendations for the UK, where the present review was conducted, can be made. The studies included also involved the use of different subgroup samples from the same large cohort, due to the necessity and availability of a limited number of longitudinal study databases. Sampling bias cannot, therefore, be disregarded.
Other polluting aryl-hydrocarbons present in the air, in particulate matter (PM2.5) and diets, such as 2,3,7,8-tetrachlorodibenzo-p-dioxin (TCDD) and its congeners, dibenzofurans and dioxin-like polychlorinated biphenyls, have been reported to induce similar neurotoxicity and neurological disorders to PAHs. The concomitant exposure to these compounds, which are ubiquitously present as persistent organic pollutants, could have confounded the measured effects of PAHs reported in the studies reviewed. If the primary sources did not disentangle their effects, it is possible that some of the meta-analyzed results embody added effects of these aryl-hydrocarbons on ambient PAHs.
To adjust for heterogeneity, studies were stratified depending on the time point of exposure and outcome assessed; however, this did not account for heterogeneity between evaluators and instruments used, mainly due to the limited number of sources analyzed. In addition to this, the use of five different measures to quantify levels of PAH exposure, as well as the inclusion of quantification of PM2.5 as a measure, resulted in heterogeneity in exposure measurement instruments and the inclusion of potential contaminating compounds within PM2.5, which would confound results. Finally, there were insufficient data to calculate 95% CI for one study (32), and the request for numeric data from another study received no response (60); hence, the report of an effect on memory and cognitive disturbances was inferred from a figure with no confirmation from the raw data.
Future research
The role of biological sex in the neurotoxic effects of PAH exposure requires further investigation. Sex stratification of data concerning memory impairment in pre-natally exposed populations was contradictory. Further accounts of memory impairment following both childhood and adult exposure should be dichotomized to examine sensitivity between sexes. Pre-natal PAH exposure's effect on motor development was an area of controversy. Additional research is required in this domain to eliminate ambiguity. Individual reports of a lack of association with motor performance and perceptual motor speed, respectively, were inadequate to clarify such controversy or draw any conclusions.
In addition to this, a more thorough examination of the timescale of PAH exposure is needed, utilizing a smaller scale to determine critical windows.
Stratification by pregnancy term elucidated differential full-scale IQ, inattentiveness, and memory performance results. No effect on the concentration of non-p-tau in CSF was reported following childhood exposure, however when looked at in a mixed cohort of childhood and adult exposure, as association in relation to age progression was reported, indicative of a critical window of exposure.
Furthermore, gene-environment interactions need further analysis, for example PAHs effect on the brain of genetically susceptible populations, such as carriers of the APOE4 allele.
Repeated future analysis on longitudinal cohorts is required to examine the impact of sustained high PAH exposure or subsequent markedly reduced exposure, the effects such fluctuations can have on cognitive function and neurodegeneration, and whether some adverse effects from pre-natal or early life exposure are recoverable.
Future research should identify and analyze the individual contributions and specific synergistic combinations of PAHs on neurological health. This would differentiate and determine the most neurotoxic PAHs and provide evidence for updates in policy, requiring the monitoring of additional PAHs, rather than only B(a)P. Additional research into the threshold at which PAH is capable of exerting neurotoxic effects would inform policy, with scientific backing to implement a safe limit with regard to neurological health and update the limit of 0.25 ng.m−3 B(a)P, which was set only with regard to carcinogenic properties. Furthermore, more studies are needed concerning populations in the UK, to account for the local environmental, climate, and seasonal variations capable of altering PAH's neurotoxic properties.
Data availability statement
The datasets presented in this study can be found in online repositories. The name of the repository and accession number can be found below: Edinburgh DataShare, https://datashare.ed.ac.uk/, https://doi.org/10.7488/ds/3031.
Author contributions
All authors listed have made a substantial, direct, and intellectual contribution to the work and approved it for publication.
Funding
JH was funded by the College of Medicine and Veterinary Medicine at The University of Edinburgh and MV was funded by The Row Fogo Charitable Trust (Grant No. BROD.FID3668413). The study was conducted at the Dementia Research Institute at the University of Edinburgh, which receives its funding from UK DRI Ltd. Company No. 11045257 | Charity No. 1179589, funded by the UK MRC, Alzheimer's Society and Alzheimer's Research UK. This study has been also supported by the EPSRC-funded project Lifecourse of Place: how environments throughout life can support healthy aging (Ref ES/T003669/1). None of the funding bodies mentioned were involved in the study design, collection, analysis, interpretation of data, the writing of this article or the decision to submit it for publication.
Conflict of interest
The authors declare that the research was conducted in the absence of any commercial or financial relationships that could be construed as a potential conflict of interest.
Publisher's note
All claims expressed in this article are solely those of the authors and do not necessarily represent those of their affiliated organizations, or those of the publisher, the editors and the reviewers. Any product that may be evaluated in this article, or claim that may be made by its manufacturer, is not guaranteed or endorsed by the publisher.
Supplementary material
The Supplementary Material for this article can be found online at: https://www.frontiersin.org/articles/10.3389/fneur.2022.1052333/full#supplementary-material
Supplementary Figure 1. Anthropogenic sources (industrial, mobile, domestic and agricultural sources) of PAH release into the environment (figure made using: Biorender.com).
Abbreviations
AD, Alzheimer's disease; ADHD, Attention deficit hyperactivity disorder; APOE4, Apolipoprotein E4; Aβ1 − 42, Amyloid beta protein fragment 1–42; α-synuclein, Alpha-synuclein; B[a]P, Benzo[a]pyrene; BDNF, Brain-derived neurotrophic factor; CEREB IgG, Cerebellar antigen;CO, Carbon monoxide; CSF, Cerebrospinal fluid; ETS, Environmental tobacco smoke; IFN γ, Interferon gamma; IgA, Immunoglobulin A; IgG, Immunoglobulin G; IgM, Immunoglobulin M; IL β, Interleukin beta; IL 2, Interleukin 2; IL 6, Interleukin 6; IL 10, Interleukin 10; IQ, Intelligence quotient; MBP, Myelin basic protein; MBP IgA, Myelin basic protein immunoglobulin A; MBP IgG, Myelin basic protein immunoglobulin G; MCP-1, Monocyte chemoattractant protein-1; MOG IgG, Myelin oligodendrocyte glycoprotein immunoglobulin G; MOG IgM, Myelin oligodendrocyte glycoprotein immunoglobulin A; NHANES, National Health and Nutrition Examination Survey; Non-p-tau, Non-phosphorylated tau; NOx, Nitrogen oxide species; NO2, Nitrogen dioxide; OZ IgA, Occludin/zonulin immunoglobulin A; OZ IgG, Occludin/zonulin immunoglobulin G; PAH, Polycyclic aromatic hydrocarbons; PAH-DNA adducts, Polycyclic aromatic hydrocarbon- DNA adducts; PD, Parkinson's disease; PM, Particulate matter; PM2.5, Particulate matter with an aerodynamic diameter > 2.5 micrometers; PM10, Particulate matter with an aerodynamic diameter > 10 micrometers; p-tau, Phosphorylated tau; S-100 IgG, Astrocytic protein immunoglobulin G; S-100 IgM, Astrocytic protein immunoglobulin M; TDP-43, Transactive response DNA binding protein 43; TRAP, Traffic-related air pollution.
Footnotes
References
1. Cohen AJ, Brauer M, Burnett R, Anderson HR, Frostad J, Estep K, et al. Estimates and 25-year trends of the global burden of disease attributable to ambient air pollution: an analysis of data from the Global Burden of Diseases Study 2015. Lancet. (2017) 389:1907–18. doi: 10.1016/S0140-6736(17)30505-6
2. Department for Environment Food and Rural Affairs. The Air Quality Strategy for England, Scotland, Wales and Northern Ireland. Norwich: HMSO (2011).
3. The European Parliament and The European Council of the European Union. Directive 2004/107/EC of the European Parliament and of the Council of 15December 2004 relating to arsenic, cadmium, mercury, nickel and polycyclic aromatic hydrocarbons in ambient air. Brussels: The European Parliament and The Council of the European Union (2015).
4. Agency for Toxic Substances and Disease Registry (ATSDR). Toxicological profile for Polycyclic Aromatic Hydrocarbons (PAHs). Atlanta, GA: U.S. Department of Health and Human Services, Public Health Service (1995).
5. Menicini E, Bocca B. Polyciclic Aromatic hydrocarbons. In:Encyclopedia of Food Sciences and Nutrition. 2nd, ed. Academic Press; Elsevier (2003). p. 4616–25. doi: 10.1016/B0-12-227055-X/00939-1
6. Hassanvand MS, Naddafi K, Faridi S, Nabizadeh R, Sowlat MH, Momeniha F, et al. Characterization of PAHs and metals in indoor/outdoor PM10/PM2.5/PM1 in a retirement home and a school dormitory. Sci Total Environ. (2015) 527–8:100–10. doi: 10.1016/j.scitotenv.2015.05.001
7. ACGIH. Polycyclic Aromatic Hydrocarbons (PAHs) Biologic Exposure Indices (BEI) Cincinnati. Cincinnati, OH: American Conference of Governmental Industrial Hygienists (ACGIH) (2005).
8. Bach PB, Kelley MJ, Tate RC, McCrory DC. Screening for lung cancer: a review of the current litrature. Chest. (2003) 123:1. doi: 10.1378/chest.123.1_suppl.72S
9. Boffetta P, Jourenkova N, Gustazsson P. Cancer risk from occupational and environmental exposure to polycyclic aromatic hydrocarbons. Cancer Causes Control. (1997) 8:3. doi: 10.1023/A:1018465507029
10. Diggs DL, Harris KL, Rekhadevi PV, Ramesh A. Tumor microsomal metabolism of the food toxicant, benzo(a)pyrene, in ApcMin mouse model of colon cancer. Tumour Biol. (2012) 33:1–12. doi: 10.1007/s13277-012-0375-6
11. Olsson AC, Fevotte J, Fletcher T, Cassidy A, Mannetje A, Zaridze D, et al. (2010). Occupational exposure to polycyclic aromatic hydrocarbons and lung cancer risk: a multicenter study in Europe. Occup Environ Med. (2010) 67:98–103. doi: 10.1136/oem.2009.046680
12. Kuo C-Y, Chien PS, Kuo W-C, Wei C-T, Rau J-Y. Comparison of polycyclic aromatic hydrocarbon emissions on gasoline- and diesel-dominated routes. Environ Monit Asses. (2012) 185:5749–61. doi: 10.1007/s10661-012-2981-6
13. Saunders CR, Das SK, Ramesh A, Shockley DC, Mukherjee S. Benzo(a)pyrene-induced acute neurotoxicity in the F-344rat: role of oxidative stress. J Appl Toxicol. (2006) 26:427–38. doi: 10.1002/jat.1157
14. Hartz AMS, Bauer B, Block ML, Hong J-S, Miller DS. Diesel exhaust particles induce oxidative stress, proinflammatory signaling, and P-glycoprotein up-regulation at the blood-brain barrier. FASEB J. (2008) 22:2723–33. doi: 10.1096/fj.08-106997
15. Abdel-Shafy H, Mansour MS. A review on polycyclic aromatic hydrocarbons. Environmental impact, effect on human health and remediation. Egypt J Petroleum. (2016) 25:107–23. doi: 10.1016/j.ejpe.2015.03.011
16. Kim KH, Ara JS, Kabir E, Brown RJ. A review of airborne polycyclic aromatic hydrocarbons (PAHs) and their human health effects. Environ Int. (2013) 60:71–80. doi: 10.1016/j.envint.2013.07.019
17. Armstrong B, Hutchinson E, Unwin J, Fletcher T. Lung cancer risk after exposure to polycyclic aromatic hydrocarbons. A review and meta-analysis. Environ Health Perspect. (2004) 112:970–8. doi: 10.1289/ehp.6895
18. Bosetti C, Boffetta, P, La Vecchia, C. Occupational exposures to polycyclic aromatic hydrocarbons, and respiratory and urinary tract cancers: a quantitative review to 2005. Ann Oncol. (2007) 431–46. doi: 10.1093/annonc/mdl172
19. Peters R, Ee N, Peters J, Booth A, Mudway I. Air pollution and dementia: a systematic review. J Alzheimers Dis. (2019) 70:145–64. doi: 10.3233/JAD-180631
20. Schikowski T, Altug H. The role of air pollution in cognitive impairment and decline. Neurochem Int. (2020) 136:1–4. doi: 10.1016/j.neuint.2020.104708
21. Kilian J, Kitazawa M. The emerging risk of exposure to air pollution on cognitive decline and Alzheimer's disease- Evidence from epidemiological and animal studies. Biomed J. (2018) 41:141–62. doi: 10.1016/j.bj.2018.06.001
22. Calderón-Garcidueñas L, Leray E, Heydarpour P, Torres-Jardón R, Reis J. Air pollution, a rising environmental risk factor for cognition, neuroinflammation and neurodegeneration. The clinical impact on children and beyond. Rev Neurol. (2016) 172:69–80. doi: 10.1016/j.neurol.2015.10.008
23. Department Department of the Environment Food and Rural Affairs. Air Pollution in the UK 2019. Norwich: HMSO (2020).
24. Finardi S, Radice P, Cecinato A, Gariazzo C, Gherardi M, Romagnoli P. Seasonal variation of PAHs concentration and source attribution through diagnostic ratios analysis. Urban Clim. (2017) 22:19–34. doi: 10.1016/j.uclim.2015.12.001
25. Liberati A, Altman DG, Tetzlaff J, Mulrow C, Gøtzsche PC, Loannidis JPA, et al. The PRISMA statement for reporting systematic reviews and meta-analyses of studies that evaluate healthcare interventions: explaination and elaboration. Br Med J. (2009) 339:1–14. doi: 10.1136/bmj.b2700
26. Tang D, Lee J, Muirhead L, Li TY, Qu L, Yu J, et al. Molecular and neurodevelopmental benefits to children of closure of a coal burning power plant in China. PLoS ONE. (2014) 9:e91966. doi: 10.1371/journal.pone.0091966
27. Tang D, Li TY, Chow JC, Kulkarni SU, Watson JG, Ho SSH, et al. Air pollution effects on fetal and child development: a cohort comparison in China. Environ Pollut. (2014) 185:90–6. doi: 10.1016/j.envpol.2013.10.019
28. Kerin T, Volk H, Li W, Lurmann F, Eckel S, McConnell R, et al. Association between air pollution exposure, cognitive and adaptive function, and ASD severity among children with autism spectrum disorder. J Autism Dev Disord. (2017) 48:137–50. doi: 10.1007/s10803-017-3304-0
29. Calderón-Garcidueñas L, Avila- Ramírez J, Calderón-Garcidueñas A, Gonzáles-Heredia T, Acuña- Ayala H, Chao C, et al. Cerebrospinal fluid biomarkers in highly exposed PM2.5 urbanites: the risk of Alzheimer's and Parkinson's Diseases in Young Mexico City Residents. J Alzheimers Dis. (2016) 54:597–613. doi: 10.3233/JAD-160472
30. Calderón-Garcidueñas L, Mukherjee PS, Waniek K, Holzer M, Chao C, Thompson C, et al. Non-phosphorylated Tau in cerebrospinal fluid is a marker of Alzheimer's Disease continuum in young urbanites exposed to air pollution. J Alzheimers Dis. (2018) 66:1437–51. doi: 10.3233/JAD-180853
31. Perera F, Rauh V, Whyatt RM, Tsai WY, Tang D, Diaz D. Effect of pre-natal exposure to airborne polycyclic aromatic hydrocarbons on neurodevelopment in the first 3 years of life among inner-city children. Environ Health Perspect. (2006) 114:1287–98. doi: 10.1289/ehp.9084
32. Perera F, Phillips DH, Wang Y, Roen E, Herbstman J, Rauh V, et al. Pre-natal exposure to polycyclic aromatic hydrocarbons/aromatics, BDNF and child development. Environ Res. (2015) 142:602–8. doi: 10.1016/j.envres.2015.08.011
33. Perera F, Tang D, Wang S, Vishnevetsky J, Zhang B, Diaz D. Prenatal polycyclic aromatic hydrocarbon (PAH) exposure and child behavior at age 6–7 years. Environ Health Perspect. (2012) 120:921–30. doi: 10.1289/ehp.1104315
34. Perera F, Wang S, Vishnevetsky J, Zhang B, Cole K, Tang D, et al. Polycyclic aromatic hydrocarbons–aromatic DNA adducts in cord blood and behaviour scores in New York City children. Environ Health Perspect. (2011) 119:1176–81. doi: 10.1289/ehp.1002705
35. Perera F, Wheelock K, Wang Y, Tang D, Margolis AE, Badia G, et al. Combined effects of prenatal exposure to polycyclic aromatic hydrocarbons and material hardship on child ADHD behavior problems. Environ Res. (2018) 160:505–13. doi: 10.1016/j.envres.2017.09.002
36. Pagliaccio D, Herbstman JB, Perera F, Tang D, Goldsmith J, Peterson BS, et al. Prenatal exposure to polycyclic aromatic hydrocarbons modifies the effects of early life stress on attention and thought problems in late childhood. J Child Psychol Psychiatry. (2020) 61:1253–65. doi: 10.1111/jcpp.13189
37. Best EA, Juaez-Colunga E, James K, LeBlanc WG, Serdar B. Biomarkers of exposure to polycyclic aromatic hydrocarbons and cognitive function among elderly in the United States. National Health and Nutrition Examination Survey: 2001–2002. PLoS ONE. (2016) 11:e0147632. doi: 10.1371/journal.pone.0147632
38. Abid Z, Roy A, Herbstman JB, Ettinger AS. Urinary polycyclic aromatic hydrocarbon metabolites and attention/deficit hyperactivity disorder, learning disability, and special education in U.S. children aged 6 to 15. J Environ Public Health. (2014) 2014:1–10. doi: 10.1155/2014/628508
39. Cserbik D, Chen JC, McConnell R, Berhane K, Sowell ER, Schwartz J, et al. Fine particulate matter exposure during childhood relates to hemispheric- specific differences in brain structure. Environ Int. (2020) 143: 1–11. doi: 10.1289/isee.2020.virtual.O-OS-592
40. Chiu YM, Hsu HL, Coull BA, Bellinger DC, Kloog I, Schartz J, et al. Prenatal particulate air pollution and neurodevelopment in urban children: examining sensitive windows and sex-specific associations. Environ Int. (2016) 87:56–65. doi: 10.1016/j.envint.2015.11.010
41. Niu Q, Zhang H, Li X, Li M. Benzo(a)pyrene-induced neurobehavioral function and neurotransmitter alterations in coke oven workers. Occup Environ Med. (2010) 67:444–8. doi: 10.1136/oem.2009.047969
42. Cao X, Li J, Cheng L, Deng Y, Li Y, Yan Z, et al. The associations between prenatal exposure to polycyclic aromatic hydrocarbon metabolites, umbilical cord blood mitochondrial DNA copy number, and children's neurobehavioral development. Environ Pollut. (2020) 265:1–10. doi: 10.1016/j.envpol.2020.114594
43. Nie J, Li J, Cheng L, Deng Y, Li Y, Yan Z, et al. Prenatal polycyclic aromatic hydrocarbons metabolites, cord blood telomere length, and neonatal neurobehavioral development. Environ Res. (2019) 174:105–13. doi: 10.1016/j.envres.2019.04.024
44. Perera F, Li T, Zhou Z, Yuan T, Chen Y, Qu L. Benefits of reducing prenatal exposure to coal-burning pollutants to children's neurodevelopment in China. Environ Health Perspect. (2008) 116:1396–403. doi: 10.1289/ehp.11480
45. Tang D, Li T, Liu JJ, Zhou Z, Yuan T, Chen Y. Effects of prenatal exposure to coal-burning pollutants on children's development in China. Environ Health Perspect. (2008) 116:674–83. doi: 10.1289/ehp.10471
46. Du J, Pan B, Cao X, Li J, Yang J, Nie J. Urinary polycyclic aromatic hydrocarbon metabolites, peripheral blood mitochondrial DNA copy number and neurobehavioral function in coke oven workers. Chemosphere. (2020) 261:1–11. doi: 10.1016/j.chemosphere.2020.127628
47. Zhang Y, Yang Y, Zhang Q, Cui J, Rahaman A, Huang X, et al. Effect of Benzo(a)pyrene-DNA adduct in cord blood on the neurodevelopment of 12-month-old infants in Qingdao City. Neuropsychiatr Dis Treat. (2019) 15:3351–7. doi: 10.2147/NDT.S219244
48. Lertxundi A, Baccini M, Lertxundi N, Fano E, Aranbarri A, Martínez MD, et al. Exposure to fine particle matter, nitrogen dioxide and benzene during pregnancy and cognitive and psychomotor developments in children at 15 months of age. Environ Int. (2015) 80:33–40. doi: 10.1016/j.envint.2015.03.007
49. Lertxundi A, Andiarena A, Martinez MD, Ayerdi M, Murcia M, Estarlich M, et al. Prenatal exposure to PM2.5 and NO2 and sex-dependent infant cognitive and motor development. Environ Res. (2019) 174:114–21. doi: 10.1016/j.envres.2019.04.001
50. Alemany S, Vilor-Tejedor N, Garcia-Esteban R, Bustamante M, Dadvand P, Esnaola M, et al. Traffic-related air pollution, APOE E4 status, and neurodevelopmental outcomes among school children enrolled in the BREATHE Project (Catalonia, Spain). Environ Health Perspect. (2018) 126:1–11. doi: 10.1289/EHP2246
51. Mortamais M, Pujol J, van Drooge BL, Macia D, Martinez-Vilavella G, Reynes C, et al. Effect of exposure to polycyclic aromatic hydrocarbons on basal ganglia and attention-deficit hyperactivity disorder symptoms in primary school children. Environ Int. (2017) 105:12–9. doi: 10.1016/j.envint.2017.04.011
52. Rivas I, Basagana X, Cirach M, Lopez-Vicente M, Suades-Gonzalez E, Garcia-Esteban R, et al. Association between early life exposure to air pollution and working memory and attention. Environ Health Perspect. (2019) 127:1–11. doi: 10.1289/EHP3169
53. Jedrychowski W, Perera F, Camann D, Spengler J, Butscher M, Mroz E, et al. Prenatal exposure to polycyclic aromatic hydrocarbons and cognitive dysfunction in children. Environ Sci Pollut Res. (2015) 22:3631–9. doi: 10.1007/s11356-014-3627-8
54. Edwards SC, Jedrychowski W, Butscher M, Camann D, Kieltyka A, Mroz E. Prenatal exposure to airborne polycyclic aromatic hydrocarbons and children's intelligence at 5 years of age in a prospective cohort study in Poland. Environ Health Perspect. (2010) 118:1–9. doi: 10.1289/ehp.0901070
55. Perera F, Wang S, Rauh V, Zhou H, Stigter L, Camann D, et al. Prenatal exposure to air pollution, maternal psychological distress, and child behavior. Pediatrics. (2013) 132:1284–94. doi: 10.1542/peds.2012-3844
56. Polanska K, Hanke W, Sobala W, Trzcinka-Ochocka M, Ligocka D, Brzeznicki S, et al. Developmental effects of exposures to environmental factors: the Polish mother and child cohort study. BioMed Res Int. (2013) 2013:1–11. doi: 10.1155/2013/629716
57. Calderón-Garcidueñas L, González-Maciel A, Reynoso-Robles R, Hammond J, Kulesza R, Lachmann I, et al. Quadruple abnormal protein aggregates in brainstem pathology and exogenous metal-rich magnetic nanoparticles (and engineered Ti-rich nanorods). The substantia nigrae is a very early target in young urbanites and the gastrointestinal tract a key brainstem portal. Environ Res. (2020) 191:1–17. doi: 10.1016/j.envres.2020.110139
58. Calderón-Garcidueñas L, Vojdani A, Blaurock-Busch E, Busch Y, Friedle A, Franco-Lira M, et al. Air pollution and children: neural and tight junction antibodies and combustion metals, the role of barrier breakdown and brain immunity in neurodegeneration. J Alzheimers Dis. (2015) 43:1037–58. doi: 10.3233/JAD-141365
59. Cho J, Sohn J, Noh J, Jang H, Kim W, Cho SK, et al. Association between exposure to polycyclic aromatic hydrocarbons and brain cortical thinning: the environmental pollution-induced neurological effects (EPINEF) study. Sci Total Environ. (2020) 737:1–9. doi: 10.1016/j.scitotenv.2020.140097
60. Ha M, Kwon H, Cheong HK, Lim S, Yoo SJ, Kim EJ, et al. Urinary metabolites before and after clean-up and subjective symptoms in volunteer participants in clean-up of the Hebei Spirit oil spill. Sci Total Environ. (2012) 429:167–73. doi: 10.1016/j.scitotenv.2012.04.036
61. Blazkova B, Pastorkova A, Solansky I, Veleminsky M Jr, Veleminsky M, Urbancova K, et al. Effect of polycyclic aromatic hydrocarbons exposure on cognitive development in 5 years old children. Brain Sci. (2020) 10:619–30. doi: 10.3390/brainsci10090619
62. Suter MK, Karr CJ, John-Stewart GC, Gomez LA, Moraa H, Nyatika D, et al. Implications of combined exposure to household air pollution and HIV on neurocognition in children. Environ Res Public Health. (2018) 15:163–76. doi: 10.3390/ijerph15010163
63. Saenen ND, Provost EB, Viaene MK, Vanpoucke C, Lefebvre W, Vrijens K, et al. Nawrot TS. Recent versus chronic exposure to particulate matter air pollution in association with neurobehavioral performance in a panel study of primary schoolchildren. Environ Int. (2016) 95:112–9. doi: 10.1016/j.envint.2016.07.014
64. Fu P, Guo X, Cheung FMH, Yung KKL. The association between PM2.5 exposure and neurological disorders: a systematic review and meta-analysis. Sci Total Environ. (2019) 655:1240–8. doi: 10.1016/j.scitotenv.2018.11.218
65. Olasehinde TA, Olaniran AO. Neurotoxicity of polycyclic aromatic hydrocarbons: a systematic mapping and review of neuropathological mechanisms. Toxics. (2022) 10:417. doi: 10.3390/toxics10080417
Keywords: polycyclic aromatic hydrocarbon (PAH), cognition, neurological, neurodegeneration, neurobehavioral, meta-analysis, systematic literature search
Citation: Humphreys J and Valdés Hernández MdC (2023) Impact of polycyclic aromatic hydrocarbon exposure on cognitive function and neurodegeneration in humans: A systematic review and meta-analysis. Front. Neurol. 13:1052333. doi: 10.3389/fneur.2022.1052333
Received: 23 September 2022; Accepted: 06 December 2022;
Published: 10 January 2023.
Edited by:
Pamela J. Lein, University of California, Davis, United StatesReviewed by:
Alexandra Economou, National and Kapodistrian University of Athens, GreeceEduard Rodriguez-Farre, Institute of Biomedical Research of Barcelona (CSIC), Spain
Copyright © 2023 Humphreys and Valdés Hernández. This is an open-access article distributed under the terms of the Creative Commons Attribution License (CC BY). The use, distribution or reproduction in other forums is permitted, provided the original author(s) and the copyright owner(s) are credited and that the original publication in this journal is cited, in accordance with accepted academic practice. No use, distribution or reproduction is permitted which does not comply with these terms.
*Correspondence: Maria del C. Valdés Hernández, bS52YWxkZXMtaGVybmFuQGVkLmFjLnVr