- 1Division of Emergencies and Critical Care, Department of Pain Management and Research, Oslo University Hospital, Oslo, Norway
- 2Faculty of Medicine, Institute of Clinical Medicine, University of Oslo, Oslo, Norway
- 3Mind-Body Lab, Department of Psychology, University of Oslo, Oslo, Norway
- 4Oslo Center for Biostatistics and Epidemiology, Oslo University Hospital, Oslo, Norway
- 5Division of Emergencies and Critical Care, Department of Research and Development, Oslo University Hospital, Oslo, Norway
Importance: Vagus nerve innervation via electrical stimulation and meditative-based diaphragmatic breathing may be promising treatment avenues for fibromyalgia.
Objective: Explore and compare the treatment effectiveness of active and sham transcutaneous vagus nerve stimulation (tVNS) and meditative-based diaphragmatic breathing (MDB) for fibromyalgia.
Design: Participants enrolled from March 2019–October 2020 and randomly assigned to active tVNS (n = 28), sham tVNS (n = 29), active MDB (n = 29), or sham MDB (n = 30). Treatments were self-delivered at home for 15 min/morning and 15 min/evening for 14 days. Follow-up was at 2 weeks.
Setting: Outpatient pain clinic in Oslo, Norway.
Participants: 116 adults aged 18–65 years with severe fibromyalgia were consecutively enrolled and randomized. 86 participants (74%) had an 80% treatment adherence and 107 (92%) completed the study at 2 weeks; 1 participant dropped out due to adverse effects from active tVNS.
Interventions: Active tVNS is placed on the cymba conchae of the left ear; sham tVNS is placed on the left earlobe. Active MDB trains users in nondirective meditation with deep breathing; sham MDB trains users in open-awareness meditation with paced breathing.
Main outcomes and measures: Primary outcome was change from baseline in ultra short-term photoplethysmography-measured cardiac-vagal heart rate variability at 2 weeks. Prior to trial launch, we hypothesized that (1) those randomized to active MDB or active tVNS would display greater increases in heart rate variability compared to those randomized to sham MDB or sham tVNS after 2-weeks; (2) a change in heart rate variability would be correlated with a change in self-reported average pain intensity; and (3) active treatments would outperform sham treatments on all pain-related secondary outcome measures.
Results: No significant across-group changes in heart rate variability were found. Furthermore, no significant correlations were found between changes in heart rate variability and average pain intensity during treatment. Significant across group differences were found for overall FM severity yet were not found for average pain intensity.
Conclusions and relevance: These findings suggest that changes in cardiac-vagal heart rate variability when recorded with ultra short-term photoplethysmography in those with fibromyalgia may not be associated with treatment-specific changes in pain intensity. Further research should be conducted to evaluate potential changes in long-term cardiac-vagal heart rate variability in response to noninvasive vagus nerve innervation in those with fibromyalgia.
Clinical trial registration: https://clinicaltrials.gov/ct2/show/NCT03180554, Identifier: NCT03180554.
Introduction
Fibromyalgia (FM) is a chronic disorder characterized by disabling pain that lasts for 3 months or more in multiple regions of the body and is commonly associated with psychological distress (1). Global prevalence rates for FM are estimated to be 4.9 % in women and 2.9 % in men (2, 3) with a treatment cost of $12-14 billion each year in the United States alone1. Currently available FM treatments provide only modest improvements in pain and minimum improvements in psychophysiological functioning (4–11). The complex etiology of FM makes it continually difficult to develop targeted treatment strategies that are effective, affordable, and safe.
The presence of autonomic nervous system (ANS) dysfunction in those with FM (12, 13) is significantly associated with high self-report pain intensity when compared to pain-free controls (14). A frequently reported indication of ANS dysfunction in FM is low resting-state heart rate variability (HRV) (15). HRV represents the change in the time interval between successive heartbeats and is one of the most important indexes of cardiovascular and autonomic health in clinical and nonclinical populations (16–22). Compared to healthy controls, those diagnosed with FM display significantly lower levels of cardiac vagal tone— specific HRV variables thought to indirectly measure activity of the vagus nerve, the main nerve of the parasympathetic nervous system (19, 23–26).
Vagus nerve stimulation (VNS), which typically involves electrical stimulation of the vagal nerve, is an approved therapy for both refractory epilepsy and treatment-resistant depression (26, 27). Preliminary intervention trials on humans have shown that electrical VNS reduces widespread pain in patients with treatment-resistant FM (28) and reduces sensitivity of mechanically evoked pain in healthy volunteers (29). In addition to electrical-based VNS, cardiorespiratory VNS in the form of meditative-based diaphragmatic breathing (MDB) can also be used to directly influence brain electrical activity mediated by the vagus nerve arising from the diaphragm (30). MDB techniques demonstrate positive analgesic effects for some acute pain conditions but have yet to be determined effective for those with FM (7). These findings suggest that an indirect mediation of pain intensity through changes in cardiac-vagal tone may be a pathway to alleviate pain and disability in FM (31). To date, experimental evidence elucidating the underlying psychophysiological mechanisms is lacking.
The primary aim of this single-blinded randomized sham-controlled clinical trial was to evaluate and compare the effects of two noninvasive and portable means of electrical- and cardiorespiratory-based VNS—transcutaneous vagus nerve stimulation (tVNS) and meditative-based diaphragmatic breathing (MDB)—on photoplethysmography (PPG) measured cardiac vagal HRV and average self-report numeric rating scale (NRS) pain intensity. We hypothesized that adults with FM randomized to receive active MDB or active tVNS would display greater increases in HRV as compared to those randomized to sham MDB or sham tVNS after 2-weeks of treatment. Furthermore, we hypothesized that a change in HRV would be correlated with a change in self-reported NRS pain intensity. Lastly, we hypothesized that active treatments would outperform sham treatments on all clinical pain-related secondary outcomes.
Materials and methods
Setting and procedure
Study design and clinical trial protocol was peer-reviewed and published [see trial protocol (31)]. FM participants were consecutively recruited from, (1) the Department of Pain Management and Research at Oslo University Hospital, in Oslo, Norway; (2) the surrounding Oslo community; and (3) from the south-east region of Norway. Information describing the trial was posted on ClinicalTrials.gov (Identifier: NCT03180554) and CRISITIN (Current Research Information System in Norway). Interested participants were directed to the Oslo University Hospital website where they logged into a secure digital data collection system and filled out a brief self-report inclusion/exclusion form. Participants who met self-reported inclusion criteria received an appointment for both clinical visitation I (CVI) and clinical visitation II (CVII) at the Department of Pain Management and Research, Division of Emergencies and Critical Care, Oslo University Hospital (Figure 1). Inclusion and exclusion criteria were assessed using data from the self-report digital inclusion/exclusion form and an in-person diagnostic screening interview at CVI. Participants enrolled between March 2019 and October 2020. Ethical approval was obtained by the Regional Committee for Medical and Health Research Ethics (REC South-East, Project Number: 2017/7066) in Norway in May 2017.
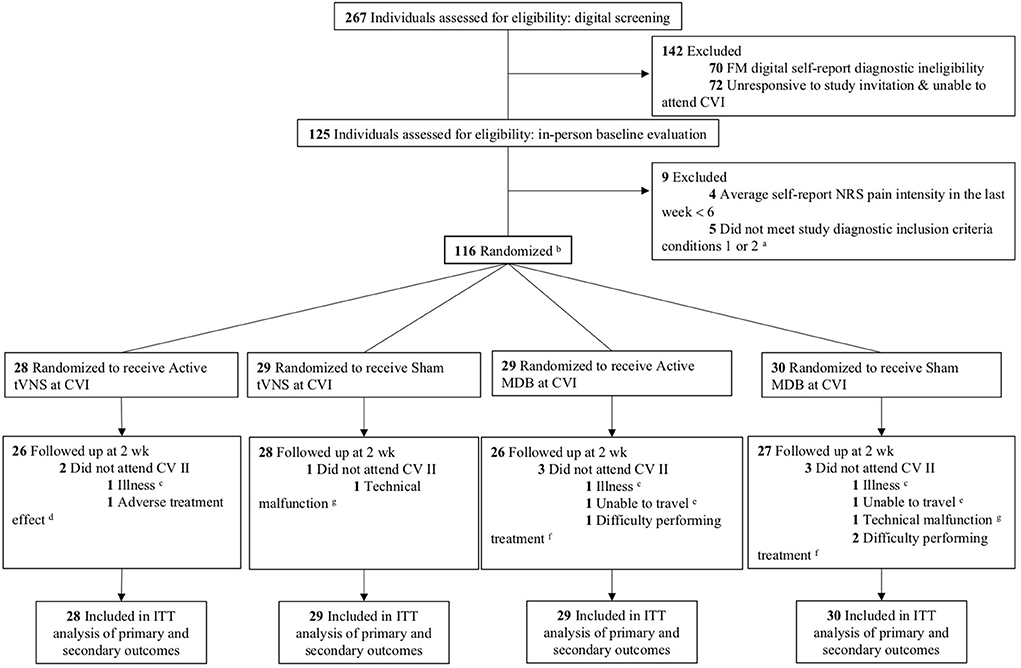
Figure 1. CONSORT flow diagram of study participation. aCondition 1: Widespread pain index (WPI) ≥ 7 and symptom severity scale (SSS) score ≥ 5; Condition 2: WPI of 4–6 and SSS score ≥ 9. bOf the 116 participants randomized, 86 completed 80% of their assigned home treatment (i.e., 23 out of 28 total treatment sessions) which was regarded as adequate treatment adherence; tVNS version 1: 24 participants compliant; tVNS version 2: 25 participants compliant; MDB version 1, 20 participants compliant; MDB version 2, 17 participants compliant. cIllness: tVNS version 1, 1 participant discontinued treatment due to a diagnosis of a comorbid condition; MDB version 1, 1 participant discontinued treatment due to sickness; MDB version 2, discontinued treatment due to excessive fatigue. dAdverse treatment effects: tVNS version 1, 1 participant discontinued treatment due to chest discomfort and additional pain due to tVNS stimulation. eUnable to travel: MDB version 1, 1 participant discontinued treatment due to unknown circumstances and trouble traveling to the clinic; MDB version 2, 1 participant discontinued treatment due to trouble traveling to the clinic. fDifficulty performing treatment: MDB version 1, 1 participant discontinued treatment due to difficulty with following the breathing pattern; MDB version 2, 1 participant discontinued treatment due to difficulty with the meditation posture. gTechnical malfunction: tVNS version 2, 1 participant discontinued treatment due to technical issues with their ear electrode; MDB version 2, 1 participant discontinued treatment due to technical issues with the MDB intervention app and HRV app. CVI, Clinical Visitation I; CVII, Clinical Visitation II; tVNS, transcutaneous vagus nerve stimulation; MDB, Meditative-based diaphragmatic breathing; ITT, intent-to-treat.
Participants and blinding
Participants were 18–65 years of age with severe FM that persisted at least 3 months or more with an average self-report NRS pain intensity of 6-10. A full list of study exclusion criteria can be found in trial protocol (31). Participants provided both oral and written informed consent for participation. Active and sham treatment allocation was concealed from the participants and testing administrators—both were told that they will provide/receive two different versions of nerve stimulation at different locations on the ear (for the tVNS group) or that there are two breathing techniques that are being explored (for the MDB group). The testing administrators introduced either “Version 1” (active) or “Version 2” (sham) of the respective treatment interventions. The principal investigators were blinded to patient treatment allocation as well as the randomization.
Randomization
Participants were consecutively randomized to one of the four treatment groups which were ran in parallel: tVNS #1 (active); tVNS #2 (sham); MDB #1 (active); MDB #2 (sham). The computer-generated randomized allocation sequencer was imported into an electronic data capture system and made available to testing administrators. The randomization was stratified by sex (male = 1, female = 2) and cardioactive medications (yes = 1, no = 0) with varying block size within strata. Participants were permitted to continue taking any previously prescribed pain medications during the trial period.
Interventions
Both treatment types (MDB or tVNS) and versions (active or sham) were self-delivered for 15 min in the morning upon waking and 15 min at night before bed for a total duration of 2-weeks [see intervention details in (31)]. Participants received a step-by-step training session and instruction manual on how to self-deliver their assigned treatment at home for the 14-day period at Clinical Visitation I (CVI). Treatment adherence was evaluated at the end of CVII by testing administrators by a combination of HRV recording sessions and respiratory data (for active and sham MDB).
Active and sham MDB was delivered via an android smartphone-app program (32) and compatible CE-approved respiratory gating device (31). Active MDB guided participants in nondirective meditation (33) and slow diaphragmatic breathing (6 breadths/min) (17) whereas sham MDB instructed participants in focused paced breathing (22, 34, 35) at 12 breadths/min—the normal resting respiration rate for an adult (36). Active and sham auricular transcutaneous vagus nerve stimulation (tVNS) was delivered utilizing two titanium electrodes connected to a portable electrical stimulator (22, 37–39). Participants placed the bipolar stimulation electrode within the conchae of the left ear (active tVNS) or on the center of the left earlobe (40, 41) (sham tVNS). A detailed description of tVNS and MDB delivery is described in (31).
Follow-up
Testing administrators collected data in-person at baseline (CVI) and post-intervention follow-up (CVII).
Measures
FM diagnostic information and cardiovascular information was obtained at baseline (Table 1). Primary and secondary outcome measures were collected at baseline and post-intervention follow-up.
Primary outcome
Resting-state photoplethysmography (PPG)-measured HRV data was obtained via an Android App utilized in prior research trials (42–44) and validated with the Polar H7 device and electrocardiography (ECG) (45) at CVI and CVII. HRV recordings of 1 min were used to assess cardiac-vagal HRV parameters: the root mean square of successive differences (rMSSD), high-frequency (HF) HRV, and the percentage of successive RR intervals that differ by more than 50 ms (pNN50). PPG-measured HRV is considered a reliable means of computing HRV if the correct procedural methods of recording are implemented in trials (46): each participant was instructed to remain seated for at least 5 min prior to taking the first HRV measurement to acclimatize; acclimatization reduces HRV changes due to posture changes (47, 48) and help reduce confounds driven by participant test anxiety and changes in respiration (18, 49, 50). Immediately following the 5 min acclimatization period, three 1- min HRV recordings were taken on the tip of the right index finger of each participant separated by 1-min intervals [recording procedure is provided in (31)]. Due to the impact of attentive states and test anxiety on respiratory frequency and HRV recordings (51), the beginning and end of each of the three HRV recordings were not announced. An average of the last two recordings was used as the baseline measure.
Secondary outcomes
Testing administrators conducted a FM clinical interview at CVI and in CVII. Participants were guided through an electronic version of the 2016 revision to the 2010/2011 FM diagnostic criteria form (52) which computes an overall FM severity (0–31 point scale, where higher numbers indicated more severe pain status) composed of a widespread pain index (WPI) score and a symptom severity scale (SSS) score. The numeric rating scale (NRS) (53) was used to assess average pain intensity in the last week at both CVI and CVII. Participants chose a number between 0 and 10 that best described their pain intensity—zero indicates “no pain at all” whereas the upper limit represents “the worst pain ever possible.”
Participant-reported outcome measures in the form of questionnaires were completed electronically by each participant at the end of CVI and CVII [for a complete list of questionnaires see (31)]. The Credibility/Expectancy Questionnaire (CEQ) was delivered at CVI and measures a participant's expectations about the efficacy of a particular treatment and whether they think that the treatment is credible or not; it is composed of six items which are scored on a 9-point scale ranging from “not at all logical,” “somewhat logical,” and “very logical.” Items 4 and 6 ask the participant how they feel and how they think the administered treatment will improve their overall health state regarding their pain on a 0–100% scale, where 0% represents “no improvement” whereas 100% represents “total improvement.”
Adverse events
Adverse events were identified during intervention period and by follow-up testing administrator questions about significant discomfort, technical usability issues, or harm of any kind caused by the interventions.
Sample size
A sample size of at least 84 participants (21 in each group) was needed to detect meaningful differences between our four groups at 2 weeks (54, 55). Effect size distributions of 0.25, 0.5, and 0.9 were interpreted as small, medium, and large effects (after rounding to the closest 0.05). To account for possible participant dropout during the trial, we aimed to recruit a minimum of 112 participants (28 participants per group) which would correlate to a statistical power of 0.9. A final population of N =116 were formally enrolled and randomized (Figure 1) for the study. This recommended sample size is based upon the effect size distributions and was tailored to our specific study to appropriately power the investigation and make it more likely to better replicate and derive true effect size estimates for both HRV and NRS pain intensity (55).
Statistical analyses
The analyses were performed with STATA/SE for Windows, Version 16.1 and IBM SPSS Statistics version 27 (56, 57). Characteristics of the four groups were described by means and standard deviations or medians and quartile ranges for continuous variables. Frequencies and percentages were given for categorical variables/data. Missing data after baseline were handled with last observation carried forward method as specified in the protocol. Associations between changes in HRV and pain were examined by correlation analyses—strengths of the intercorrelations were assessed by Pearson's and Spearman's correlation coefficients. A linear mixed model for continuous outcomes was used to fit two data points (week 0, week 2) per patient to include all patients in the analysis in accordance with the Intention to Treat principle (ITT).
Difference in change from baseline between the four groups with and without adjusting for baseline was tested by using two linear mixed effect models for continuous outcome data and a likelihood ratio test. For the random part of the linear mixed effect model, participant identifier or identifier and time, were specified as the random variables. In the linear mixed model with two random variables (identifier and time), an exchangeable variance-covariance structure was specified. For the fixed part of the mixed model, group and time and interaction between group and time were specified to test whether the change differed between the groups over time with and without adjusting for baseline values. Highly skewed (non-normally distributed) variables were logarithmically transformed before fitting the mixed models. The analyses of average changes between groups over time were carried out for the compliant groups (n= 86) and full sample (N= 116).
Results
Among 267 individuals expressing interest in study participation and screened for eligibility, 116 were enrolled and randomized (Figure 1). Of the 116 participants randomized, 86 (74%) in total completed 80% of their assigned home treatment (i.e., 23 out of 28 total treatment sessions) which was regarded as adequate treatment adherence (see Figure 1 for 80% compliancy numbers by treatment group) and 107 (92%) were seen at CVII for post-treatment follow-up.
Treatment groups were similar in baseline characteristics and current cardioactive medication use. Significant between-group differences were found for hormonal contraceptive use among women and two items on the CEQ regarding improvement in function (Table 1). The mean (SD) pain intensity in the past week at baseline was 6.72 (0.74) for the total population indicating severe intensity. No significant differences were found across treatment groups at baseline for any cardiac vagal HRV variables. A pairwise Spearman correlation analysis (Table 2) performed for the entire FM population (N = 116) which explored associative changes from CVI to CVII among HRV and pain variables showed that NRS average pain intensity in the last week had no significant correlations with changes in HRV.
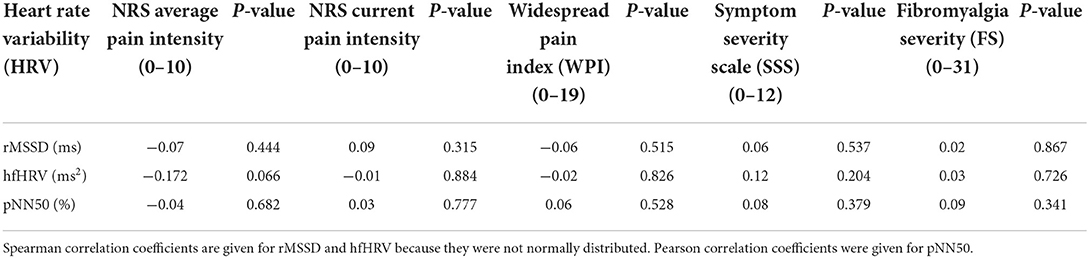
Table 2. Pairwise correlations of change from clinical visitation I to clinical visitation II between HRV, FM pain variables, and psychological distress (N = 116).
Primary outcome
There were no significant differences found across treatment groups in any of the cardiac-vagal variables of interest (Table 3A).
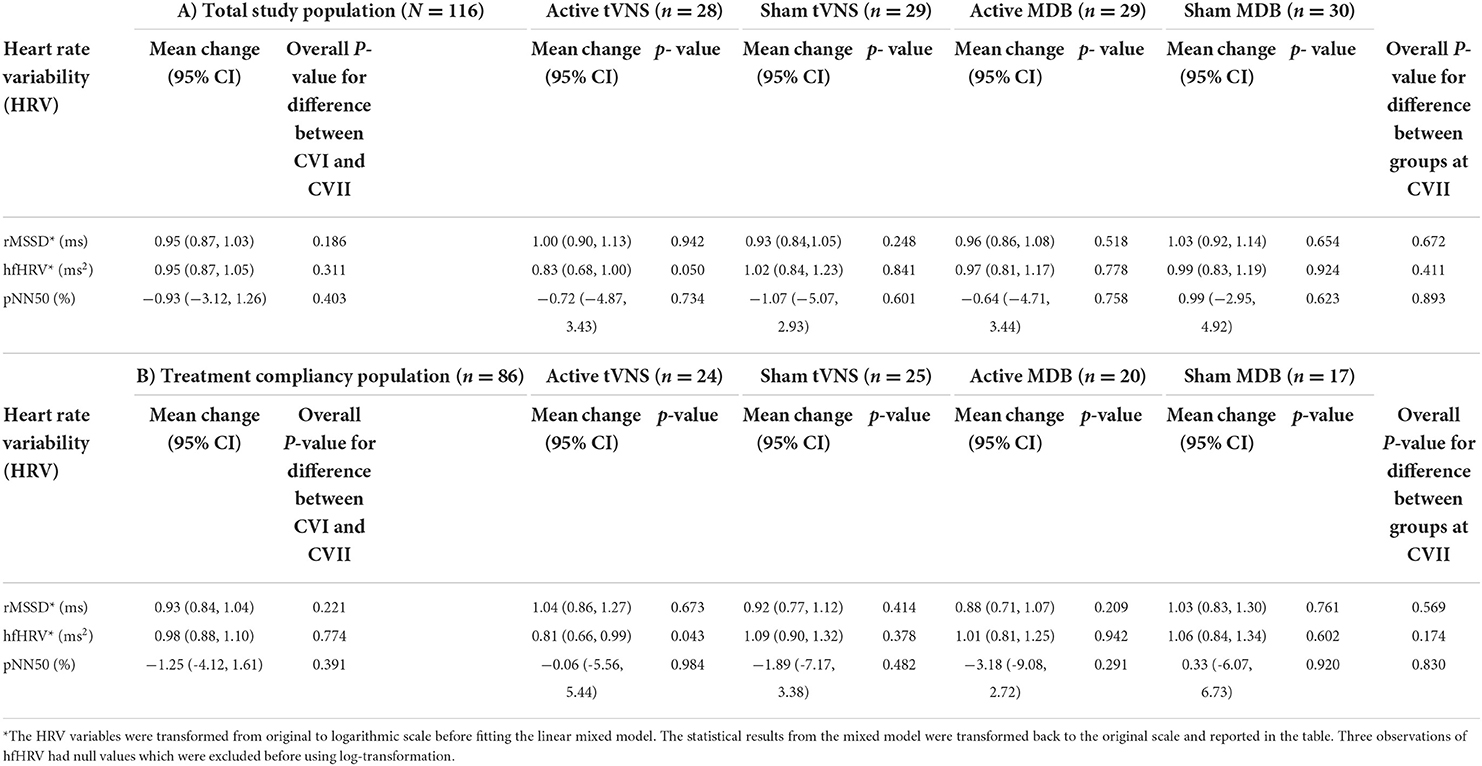
Table 3. Primary outcome: Mean (95% CI) differences between treatment groups at 2 wk follow-up for HRV and total average change in HRV from baseline to follow-up for total study population (A; N = 116) and treatment compliancy population (B; n = 86) (Imputed analyses adjusted for baseline differences).
Per-protocol analyses
No significant across-group differences were found in any of the cardiac-vagal variables of interest for participants who achieved 80% treatment compliancy (a completion of 23 out of 28 total treatment sessions) (n = 86) (Table 3B).
Secondary outcomes
Significant across group differences were found for current pain intensity with significant within-group changes in those randomized to active tVNS (−0.82; 95% CI, −1.32−0.31) and sham tVNS (−0.86; 95% CI, −1.36–−0.36) (Table 4). Significant across group differences were also found for overall FM severity. No significant across group differences were found for FM symptom severity, widespread pain, or average pain intensity in the last week.
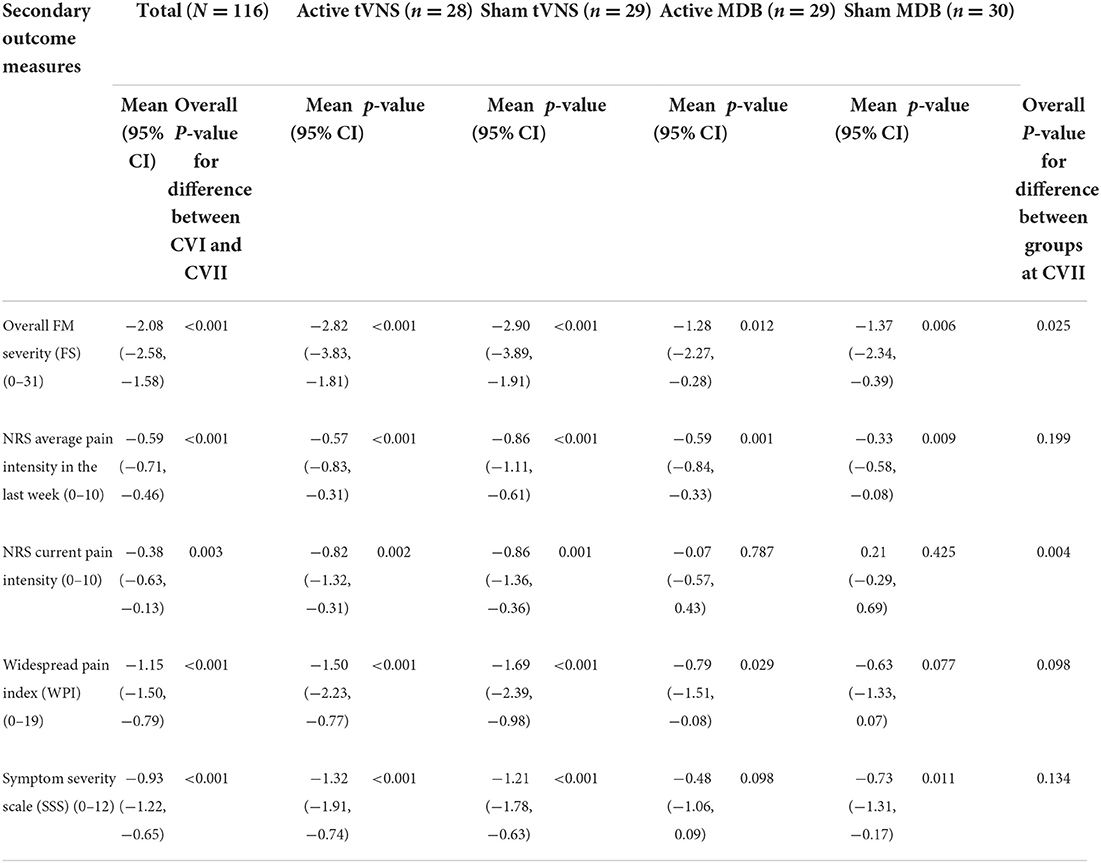
Table 4. Mean (95% CI) differences between treatment groups at 2 wk follow-up and total average change from baseline to follow-up for secondary outcomes (Imputed analyses adjusted for baseline differences; N = 116).
Adverse events
One participant randomized to receive active tVNS experienced chest discomfort and additional pain due to the tVNS stimulation and decided to discontinue treatment. No other serious adverse events were reported in this trial.
Discussion
The aim of our randomized controlled clinical trial was to establish and compare the efficacy of active and sham versions of tVNS and MDB on cardiac vagal tone and average pain intensity in adults diagnosed with severe FM. Contrary to our primary hypothesis, no significant across-group differences in cardiac vagal tone were found. Furthermore, contrary to our second hypothesis, no significant associations were found between changes in cardiac-vagal HRV and changes in average NRS pain intensity during treatment in our entire FM population; active treatments did not outperform sham treatments for clinical pain-related secondary outcomes. However, significant group differences were found for overall FM severity and current pain intensity at post-treatment follow-up.
The presence of significant pain reductions in the absence of significant across-group differences in cardiac-vagal HRV variables may challenge the current notion that rMSSD and/or hfHRV are reliable indexes of cardiac vagal activity (19, 24, 25, 58). Recent preliminary findings in rats (59) and in healthy subjects (60) indicate that there is no correlation between cardiac vagal HRV and tonic vagal activity or vagal stimulation over time (61). These findings may suggest three important implications: (1) that clinical measures of HRV may not represent vagal activity; (2) the term “vagal tone” may be misleading; and/or (3) rMSSD may only reflect a small subset of cardiac vagal afferent activity.
Utilizing ultra short-term (<5 min) PPG methods for evaluating cardiac-vagal HRV could have aided in increasing participant treatment compliancy and regimen (62) while also decreasing overall clinical visitation time and associated costs (63). However, it may have been insufficient in detecting potentially significant autonomic changes in participants before, during, and after treatment. Long-term HRV recordings still represent the typical reference standard for predicting health outcomes whereas short-term values are proxies of long-term values with unknown predictive validity; therefore, ultra-short HRV measurements could be considered as “proxies of proxies” (63). Despite the fact that our HRV recording method, instrumentation, and procedure has been validated with both the Polar H7 device and electrocardiography (ECG) (42–45) evaluating HRV utilizing classic 5-min ECG recording windows during pre and post clinical visitations could have aided in determining whether the observed changes in pain intensity were associated with changes in HRV (64). Based on clinical theories such as the vagal-tank theory, longer vagus nerve innervation treatment methods for those with FM may be needed to detect a significant perturbance of low HRV levels and help understand how the ≪vagal tank≫ sustains self-regulatory efforts to build a higher resting cardiac vagal control over time, yet this is speculative (65).
Significant changes in overall FM severity were found in all groups. Only those who received active or sham tVNS displayed significant changes in current NRS pain intensity as compared to those randomized to active or sham MDB, yet the clinical significance of these findings are questionable: overall FM severity is based on a 0–31 point scale (52) whereas current pain intensity is known to (1) drastically fluctuate daily in those with FM; (2) is mediated by psychological distress in relation to physical functioning; and (3) is therefore not considered a reliable direct indicator of treatment efficacy (66). Furthermore, the overall effects of active and sham versions of tVNS in our trial were quite similar.
Observational fMRI analyses (40, 67) showed that active tVNS stimulation at the cymba conchae and sham tVNS stimulation of the earlobe resulted in similar overlapping BOLD changes in cortical areas. These observational results may provide support for our clinical findings which showed an overall nonsignificant difference of clinical efficacy between active and sham tVNS indicating that the terms “active” and “sham” tVNS stimulation could be somewhat misleading (68). More pronounced effects in favor of active respiratory biofeedback as compared to sham (34, 36, 69, 70) have been found in previous clinical trials (71–76). However, similar effects for changes in overall FM severity and average NRS self-report pain intensity for active and sham MDB in this trial challenges the significant differentiable effects demonstrated in prior literature.
Strengths and limitations
There are several important limitations to consider. Firstly, sociodemographic characteristics including education, annual income, and employment were not collected in this trial and could have provided pertinent information for describing our findings. Secondly, even though this study was practiced as a double-blinded clinical trial, it was formally categorized as a single-blind trial because there was no way of ensuring that either the testing administrators or the participants held any prior insights into active and inactive MDB/tVNS treatment protocols. Furthermore, to sufficiently detect a difference between groups in HRV as it relates to NRS pain intensity, a sample size between 30 and 77 (depending on the HRV metric of interest) is typically recommended (77); subgroups are commonly employed within designs that have been suggested to require 20 participants per cell (54). However, due to the exploratory nature of this investigation, our sample size determination may not have been ideal to detect clinically significant differences between our groups. Lastly, it is important to note our choice of methods regarding 1-min HRV recording windows. Prior research (78) has found that 10 s and 1 min rMSSD values correlate with 5 min rMSSD values. In general, HRV parameters that predominantly reflect parasympathetic cardiac modulation (rMSSD and pNN50) can be reliably measured using 1 min recordings; recordings of 1 min should be seen as the absolute minimum to obtain reliable hfHRV assessment (17, 79–81). Given that our primary HRV parameter of interest was rMSSD as it pertains to cardiac vagal function, utilizing 1-min recording windows was acceptable (17, 45, 82).
In addition to the apparent limitations of this trial, there are several important study strengths to be noted. Reducing clinical visitation time, HRV recording time, and daily treatment delivery time may have been a significant contributing factor to increasing treatment compliancy and achieving high clinical visitation attendance; MDB was self-delivered with a novel respiratory-gating device able to monitor the respiratory dynamics of each participant (35, 83–86). Noninvasive tVNS treatment was utilized to increase patient safety, usability, and decrease risk for adverse events (38). It has been proposed (87) that short- term meditative-based practices could have a more promising effect upon clinical outcomes as compared to continual long-term meditation practices (such as the 8-week mindfulness-based stress reduction program, MBSR) for specific vulnerable populations (88). tVNS treatment durations commonly range from 30 min to 2hours per session (89, 90). However, to match dosage and vagus nerve innervation across all groups, tVNS and MDB were practiced for 30 min per day split into two 15 min sessions; delivering the treatments in this manner may have helped increase treatment useability and compliancy but may have also changed the way in which the vagus nerve imparts clinical effects over time.
Conclusions
Meditative-based diaphragmatic breathing and vagus nerve stimulation had no significant across-group effects on ultra short-term PPG-measured cardiac vagal tone among adults with FM. No significant across group differences were found for changes in average self-report NRS pain intensity; active MDB as well as sham tVNS resulted in the largest improvements in average pain intensity at 2-weeks when compared to sham MDB and active tVNS. The absence of significant across-group changes in ultra short-term PPG-measured cardiac vagal tone in response to vagal-innervation treatments in those with FM may indicate that more reliable long-term recordings procedures should be utilized and/or that longer (i.e., >2-weeks) vagus nerve innervation treatment methods should be delivered. However, finding significant across-group differences for overall FM severity and current pain intensity may indicate that meditative-based diaphragmatic breathing and vagus nerve stimulation provide differentiable yet complementary approaches to noninvasive FM pain management. Further research should be conducted on evaluating long-term noninvasive vagus nerve innervation methods for treating FM due to their useability, portability, and potential effectiveness.
Data availability statement
In accordance with institutional guidelines, anonymized data is available upon request with a corresponding application describing the intent and justification(s) for investigative analysis.
Ethics statement
The studies involving human participants were reviewed and approved by Regional Committees for Medical Research Ethics South East Norway. The patients/participants provided their written informed consent to participate in this study.
Author contributions
The manuscript was drafted by CP. Study was conceptualized and designed by CP and HJ. Critical revision of the manuscript for important intellectual content was conducted by CP, AS, LR, and HJ. Blinded statistical analysis was performed by LD. This study was conducted as CP's Ph.D. Fellowship with the principal supervision of HJ. Co-supervision of AS and LR. Data acquisition, analysis, and interpretation of data was performed by all authors. All authors contributed to the article and approved the submitted version.
Funding
Funding for a doctorate fellowship was received from the South-East Regional Health Authority, Norway (Project Number: 2017/766) to conduct and analyze data from this study. The funding source had no role in the design and conduct of the study; collection, management, analysis, and interpretation of the data; preparation, review, or approval of the manuscript; and decision to submit the manuscript for publication.
Acknowledgments
We would like to thank the administrative staff working at the Department of Pain Management and Research at Oslo University Hospital for aiding in participant scheduling and visitations. We would also like to thank all research assistants assigned to this project for their help in participant data collection and testing.
Conflict of interest
The authors declare that the research was conducted in the absence of any commercial or financial relationships that could be construed as a potential conflict of interest.
Publisher's note
All claims expressed in this article are solely those of the authors and do not necessarily represent those of their affiliated organizations, or those of the publisher, the editors and the reviewers. Any product that may be evaluated in this article, or claim that may be made by its manufacturer, is not guaranteed or endorsed by the publisher.
Footnotes
References
1. Mansfield KE, Sim J, Croft P, Jordan KP. Identifying patients with chronic widespread pain in primary care. Pain. (2017) 158:110. doi: 10.1097/j.pain.0000000000000733
2. Wolfe F, Clauw DJ, Fitzcharles MA, Goldenberg DL, Katz RS, Mease P, et al. The American College of Rheumatology preliminary diagnostic criteria for fibromyalgia and measurement of symptom severity. Arth Care Res. (2010) 62:600–10. doi: 10.1002/acr.20140
3. Gran JT. The epidemiology of chronic generalized musculoskeletal pain. Best Pract Res Clin Rheumatol. (2003) 17:547–61. doi: 10.1016/S1521-6942(03)00042-1
4. Turk DC, Wilson HD, Cahana A. Treatment of chronic non-cancer pain. Lancet. (2011) 377:2226–35. doi: 10.1016/S0140-6736(11)60402-9
5. Chou R, Turner JA, Devine EB, Hansen RN, Sullivan SD, Blazina I, et al. The effectiveness and risks of long-term opioid therapy for chronic pain: a systematic review for a national institutes of health pathways to prevention workshop effectiveness and risks of long-term opioid therapy for chronic pain. Ann Intern Med. (2015) 162:276–86. doi: 10.7326/M14-2559
6. National Academies of Sciences, Engineering and Medicine. Pain Management and the Opioid Epidemic: Balancing Societal and Individual Benefits and Risks of Prescription Opioid Use. Washington, DC: National Academies Press. (2017).
7. Farias M, Wikholm C, Delmonte R. What is mindfulness-based therapy good for? Lancet Psychiatry. (2016) 3:1012–3. doi: 10.1016/S2215-0366(16)30211-5
8. Coronado-Montoya S, Levis A, Kwakkenbos L, Steele R, Turner E, Thombs B. Reporting of positive results in randomized controlled trials of mindfulness- based mental health interventions. PLoS ONE. (2016) 11:e0153220. doi: 10.1371/journal.pone.0153220
9. Veehof MM, Oskam MJ, Schreurs KM, Bohlmeijer ET. Acceptance-based interventions for the treatment of chronic pain: a systematic review and meta- analysis. Pain. (2011) 152:533–42. doi: 10.1016/j.pain.2010.11.002
10. Williams AC, Eccleston C, Morley S. Psychological Therapies for the Management Of Chronic pain (excluding headache) in Adults. London: The Cochrane Library. (2012).
11. Hofmann SG, Asmundson GJ. Acceptance and mindfulness-based therapy: new wave or old hat? Clin Psychol Rev. (2008) 28:1–6. doi: 10.1016/j.cpr.2007.09.003
12. Kingsley JD. Autonomic dysfunction in women with fibro- myalgia. Arthritis Res Ther. (2012). 14:103. doi: 10.1186/ar3728
13. Bradley LA. Pathophysiology of fibromyalgia. Am J Med. (2009) 122:S22–30. doi: 10.1016/j.amjmed.2009.09.008
14. Barakat A, Vogelzangs N, Licht CM, Geenen R, MacFarlane GJ, De Geus EJ, et al. Dysregulation of the autonomic nervous system and its association with the presence and intensity of chronic widespread pain. Arthritis Care Res. (2012) 64:1209–16. doi: 10.1002/acr.21669
15. Bruehl S, Olsen RB, Tronstad C, Sevre K, Burns JW, Schirmer H, et al. Chronic pain-related changes in cardiovascular regulation and impact on comorbid hypertension in a general population: the Tromsø study. Pain. (2018) 159:119–27. doi: 10.1097/j.pain.0000000000001070
16. McCraty R, Shaffer F. Heart rate variability: new perspectives on physiological mechanisms, assessment of self-regulatory capacity, and health risk. Glob Adv Health Med. (2015) 4:46–61. doi: 10.7453/gahmj.2014.073
17. Laborde S, Mosley E, Thayer JF. Heart rate variability and cardiac vagal tone in psychophysiological research–recommendations for experiment planning, data analysis, and data reporting. Front Psychol. (2017) 8:213. doi: 10.3389/fpsyg.2017.00213
18. Nahman-Averbuch H, Sprecher E, Jacob G, Yarnitsky D. The relationships between parasympathetic function and pain perception: the role of anxiety. Pain Pract. (2016) 16:1064–72. doi: 10.1111/papr.12407
19. Larkin KT, Tiani AG, Brown LA. Cardiac Vagal Tone and Stress. In: Oxford Research Encyclopedia of Neuroscience. Oxford: Oxford University Press. (2021).
20. Thayer JF, Lane RD. A model of neurovisceral integration in emotion regulation and dysregulation. J Affect Disord. (2000) 61:201–16. doi: 10.1016/S0165-0327(00)00338-4
21. Frøkjaer JB, Bergmann S, Brock C, Madzak A, Farmer AD, Ellrich J, et al. Modulation of vagal tone enhances gastroduodenal motility and reduces somatic pain sensitivity. Neurogastroenterol Motil. (2016) 28:592–8. doi: 10.1111/nmo.12760
22. Juel J, Brock C, Olesen SS, Madzak A, Farmer AD, Aziz Q, et al. Acute physiological and electrical accentuation of vagal tone has no effect on pain or gastrointestinal motility in chronic pancreatitis. J Pain Res. (2017) 10:1347. doi: 10.2147/JPR.S133438
23. Tracy LM, Ioannou L, Baker KS, Gibson SJ, Georgiou-Karistianis N, Giummarra MJ. Meta-analytic evidence for decreased heart rate variability in chronic pain implicating parasympathetic nervous system dysregulation. Pain. (2016) 157:7–29. doi: 10.1097/j.pain.0000000000000360
24. Dang K, Kirk MA, Monette G, Katz J, Ritvo P. Meaning in life and vagally-mediated heart rate variability: evidence of a quadratic relationship at baseline and vagal reactivity differences. Int J Psychophysiol. (2021) 165:101–11. doi: 10.1016/j.ijpsycho.2021.03.001
25. Matuz A, van der Linden D, Kisander Z, Hernádi I, Kázmér K, Csathó Á. Enhanced cardiac vagal tone in mental fatigue: analysis of heart rate variability in Time-on-Task, recovery, and reactivity. PLoS ONE. (2021) 16:e0238670. doi: 10.1371/journal.pone.0238670
26. Howland RH. Vagus nerve stimulation. Curr Behav Neurosci Rep. (2014) 1:64–73. doi: 10.1007/s40473-014-0010-5
27. Vonck K, Raedt R, Naulaerts J, De Vogelaere F, Thiery E, Van Roost D, et al. Vagus nerve stimulation… 25 years later! What do we know about the effects on cognition? Neurosci Biobehav Rev. (2014) 45:63–71. doi: 10.1016/j.neubiorev.2014.05.005
28. Lange G, Janal MN, Maniker A, FitzGibbons J, Fobler M, Cook D, et al. Safety and efficacy of vagus nerve stimulation in fibromyalgia: a phase I/II proof of concept trial. Pain Med. (2011) 12:1406–13. doi: 10.1111/j.1526-4637.2011.01203.x
29. Busch V, Zeman F, Heckel A, Menne F, Ellrich J, Eichhammer P. The effect of transcutaneous vagus nerve stimulation on pain perception–an experimental study. Brain Stimul. (2013) 6:202–9. doi: 10.1016/j.brs.2012.04.006
30. Stancak JA, Kuna M, Dostalek C, Vishnudevananda S. Kapalabhati–yogic cleansing exercise. II. EEG topography analysis. Homeost Health Dis. (1991) 33:182–9.
31. Paccione CE, Diep LM, Stubhaug A, Jacobsen HB. Motivational nondirective resonance breathing vs. transcutaneous vagus nerve stimulation in the treatment of fibromyalgia: study protocol for a randomized controlled trial. Trials. (2020) 21:1–23. doi: 10.1186/s13063-020-04703-6
32. Paccione CE, Jacobsen HB. Motivational non-directive resonance breathing as a treatment for chronic widespread pain. Front Psychol. (2019) 10:1207. doi: 10.3389/fpsyg.2019.01207
33. Xu J, Vik A, Groote IR, Lagopoulos J, Holen A, Ellingsen Ø, et al. Nondirective meditation activates default mode network and areas associated with memory retrieval and emotional processing. Front Hum Neurosci. (2014) 8:86. doi: 10.3389/fnhum.2014.00086
34. Elstad M. Respiratory variations in pulmonary and systemic blood flow in healthy humans. Acta Physiol. (2012) 205:341–8. doi: 10.1111/j.1748-1716.2012.02419.x
35. Moore AW, Gruber T, Derose J, Malinowski P. Regular, brief mindfulness meditation practice improves electrophysiological markers of attentional control. Front Hum Neurosci. (2012) 6:18. doi: 10.3389/fnhum.2012.00018
37. Kreuzer PM, Landgrebe M, Husser O, Resch M, Schecklmann M, Geisreiter F, et al. Transcutaneous vagus nerve stimulation: retrospective assessment of cardiac safety in a pilot study. Front Psychiatry. (2012) 3:70. doi: 10.3389/fpsyt.2012.00070
38. Dietrich S, Smith J, Scherzinger C, Hofmann-Preiß K, Eisenkolb A, Ringler R. A novel transcutaneous vagus nerve stimulation leads to brainstem and cerebral activations measured by functional MRI. Biomed Eng. (2008) 53:104–11. doi: 10.1515/BMT.2008.022
39. Jongkees BJ, Immink MA, Finisguerra A, Colzato LS. Transcutaneous vagus nerve stimulation (tVNS) enhances response selection during sequential action. Front Psychol. (2018) 9:1159. doi: 10.3389/fpsyg.2018.01159
40. Peuker ET, Filler TJ. The nerve supply of the human auricle. Clin Anat. (2002) 15:35–7. doi: 10.1002/ca.1089
41. Kraus T, Hosl K, Kiess O, Schanze A, Kornhuber J, Forster C. BOLD fMRI deactivation of limbic and temporal brain structures and mood enhancing effect by transcutaneous vagus nerve stimulation. J Neural Transm. (2007) 114:1485–93. doi: 10.1007/s00702-007-0755-z
42. Altini M, Van Hoof C, Amft O. Relation between estimated cardiorespiratory fitness and running performance in free-living: an analysis of HRV4Training data. In: Biomedical & Health Informatics (BHI), IEEE EMBS International Conference on 2017 Feb 16 (249-252). Orlando, FL: IEEE. (2017).
43. Williams S, Booton T, Watson M, Rowland D, Altini M. Heart rate variability is a moderating factor in the workload-injury relationship of competitive crossfitTM athletes. J Sports Sci Med. (2017) 16:443.
44. Altini M, Amft O. HRV4Training: large-scale longitudinal training load analysis in unconstrained free-living settings using a smartphone application. In Engineering in Medicine and Biology Society (EMBC). In: IEEE 38th Annual International Conference of the 2016 Aug 16 (2610±2613). IEEE. doi: 10.1109/EMBC.2016.7591265
45. Plews DJ, Scott B, Altini M, Wood M, Kilding AE, Laursen PB. Comparison of heart-rate-variability recording with smartphone photoplethysmography, Polar H7 chest strap, and electrocardiography. Int J Sports Physiol Perform. (2017) 12:1324–8. doi: 10.1123/ijspp.2016-0668
46. Bolanos M, Nazeran H, Haltiwanger E. Comparison of heart rate variability signal features derived from electrocardiography and photoplethysmography in healthy individuals. In Engineering in Medicine and Biology Society. EMBS'06.In: 28th Annual International Conference of the IEEE 2006 Aug 30 (4289-4294). IEEE. (2006).
47. Stolarz K, Staessen JA, Kuznetsova T, Tikhonoff V, Babeanu S, Casiglia E, et al. Host and environmental determinants of heart rate and heart rate variability in four European populations. J Hypertens. (2003) 21:525–35. doi: 10.1097/00004872-200303000-00018
48. Chan HL, Lin MA, Chao PK, Lin CH. Correlates of the shift in heart rate variability with postures and walking by time–frequency analysis. Comput Methods Prog Biomed. (2007) 86:124–30. doi: 10.1016/j.cmpb.2007.02.003
49. Quintana DS, Alvares GA, Heathers JA. Guidelines for reporting articles on psychiatry and heart rate variability (GRAPH): recommendations to advance research communication. Transl Psychiatry. (2016) 6:e803. doi: 10.1038/tp.2016.73
50. Vlemincx E, Van Diest I, Van den Bergh O. A sigh following sustained attention and mental stress: effects on respiratory variability. Physiol Behav. (2012) 107:1–6. doi: 10.1016/j.physbeh.2012.05.013
51. Quintana DS, Heathers JA. Considerations in the assessment of heart rate variability in biobehavioral research. Front Psychol. (2014) 5:805. doi: 10.3389/fpsyg.2014.00805
52. Wolfe F, Clauw DJ, Fitzcharles MA, Goldenberg DL, Häuser W, Katz RL, et al. Revisions to the 2010/2011 fibromyalgia diagnostic criteria. In: Seminars in arthritis and rheumatism 2016 Dec 1. Philadelphia, PA: WB Saunders. (2016). p. 319–29.
54. Simmons JP, Nelson LD, Simonsohn U. False-positive psychology undisclosed flexibility in data collection and analysis allows presenting anything as significant. Psychol Sci. (2011) 22:1359–66. doi: 10.1177/0956797611417632
55. Quintana DS. Statistical considerations for reporting and planning heart rate variability case-control studies. Psychophysiology. (2017) 54:344–9. doi: 10.1111/psyp.12798
58. Shaffer F, McCraty R, Zerr CL. A healthy heart is not a metronome: an integrative review of the heart's anatomy and heart rate variability. Front Psychol. (2014) 5:1040. doi: 10.3389/fpsyg.2014.01040
59. Marmerstein JT, McCallum GA, Durand DM. Direct measurement of vagal tone in rats does not show correlation to HRV. Sci Rep. (2021) 11:1–2. doi: 10.1038/s41598-020-79808-8
60. Verkuil B, Langås JR, Korbee N, Burger AM. Auricular stimulation of the vagus nerve does not increase cardiac baroreflex sensitivity: a randomized placebo-controlled trial. PsyarXiv [Preprint] (2019). doi: 10.31234/osf.io/kzvt4
61. Wolf V, Kühnel A, Teckentrup V, Koenig J, Kroemer NB. Does transcutaneous auricular vagus nerve stimulation affect vagally mediated heart rate variability? A living and interactive Bayesian meta-analysis. Psychophysiology. (2021) 58:e13933. doi: 10.1111/psyp.13933
62. Atreja A, Bellam N, Levy SR. Strategies to enhance patient adherence: making it simple. Medscape Gen Med. (2005) 7:4.
63. Shaffer F, Meehan ZM, Zerr CL. A critical review of ultra-short-term heart rate variability norms research. Front Neurosci. (2020) 14:594880. doi: 10.3389/fnins.2020.594880
64. Forte G, Troisi G, Pazzaglia M, Pascalis VD, Casagrande M. Heart rate variability and pain: a systematic review. Brain Sci. (2022) 12:153. doi: 10.3390/brainsci12020153
65. Laborde S, Mosley E, Mertgen A. Vagal tank theory: the three rs of cardiac vagal control functioning–resting, reactivity, and recovery. Front Neurosci. (2018) 12:458. doi: 10.3389/fnins.2018.00458
66. Steiner JL, Bigatti SM, Slaven JE, Ang DC. The complex relationship between pain intensity and physical functioning in fibromyalgia: the mediating role of depression. J Appl Biobehav Res. (2017) 22: e12079. doi: 10.1111/jabr.12079
67. Yakunina N, Kim SS, Nam EC. Optimization of transcutaneous vagus nerve stimulation using functional MRI. Neuromodulation. (2017) 20:290–300. doi: 10.1111/ner.12541
68. Breit S, Kupferberg A, Rogler G, Hasler G. Vagus nerve as modulator of the brain–gut axis in psychiatric and inflammatory disorders. Front Psychiatry. (2018) 9:44. doi: 10.3389/fpsyt.2018.00044
69. Mason H, Vandoni M, Debarbieri G, Codrons E, Ugargol V, Bernardi L. Cardiovascular and respiratory effect of yogic slow breathing in the yoga beginner: what is the best approach? Evid Based Complement Altern Med. (2013) 2013:743504. doi: 10.1155/2013/743504
70. Diaphragmatic breathing. Mosby's Medical Dictionary. 8th ed: Elsevier. (2009). Available online at: https://medical-dictionary.thefreedictionary.com/ diaphragmatic+breathing (accessed May 5, 2022).
71. Kapitza KP, Passie T, Bernateck M, Karst M. First non-contingent respiratory biofeedback placebo versus contingent biofeedback in patients with chronic low back pain: a randomized, controlled, double-blind trial. Appl Psychophysiol Biofeedback. (2010) 35:207–17. doi: 10.1007/s10484-010-9130-1
72. Barolin GS. Das respiratorische Feedback (RFB)—Basis und Praxis [The respiratory feedback (RFB)—Basics and practice]. In:Barolin GS, editor. Das Respiratorische Feedback nach Leuner [Leuner's respiratory feedback]. Berlin: Verlag fu r Wissenschaft und Bildung. (2001). pp. 9–31.
73. Jung F, Klapsing-Hessenbruch A. Eine vergleichende Studie der therapeutischen Ergebnisse zwischen Respiratori- schem Feedback (RFB) und einer Placebo-Behandlung [A comparison study of therapeutic results between respiratory feedback (RFB) and placebo treatment]. Zeitschrift fu r Psycho-somatische Medizin und Psychoanalyse. (1978) 24:36–55.
74. Locklin JK, Yanof J, Luk A, Varro Z, Patriciu A, Wood BJ. Respiratory biofeedback during CT-guided proce- dures. J Vasc Interv Radiol. (2007) 18:749–55. doi: 10.1016/j.jvir.2007.03.010
75. Pastor MC, Mene ndez FJ, Sanz MT, Abad EV. The influence of respiration on biofeedback techniques. Appl Psychophysiol Biofeedback. (2008) 33:49–54. doi: 10.1007/s10484-007-9048-4
76. Reiner R. Integrating a portable biofeedback device into clinical practice for patients with anxiety disorders: results of a pilot study. Appl Psychophysiol Biofeedback. (2008) 33:55–61. doi: 10.1007/s10484-007-9046-6
77. Pinna G, Maestri R, Torunski A, Danilowicz-Szymanowicz L, Szwoch M, La Rovere M, et al. Heart rate variability measures: a fresh look at reliability. Clin Sci. (2007) 113:131–40. doi: 10.1042/CS20070055
78. Nussinovitch U, Elishkevitz KP, Nussinovitch M, Segev S, Volovitz B, Nussinovitch N. Reliability of ultra-short ECG indices for heart rate variability. Ann Noninvasive Electrocardiol. (2011) 16:117–22. doi: 10.1111/j.1542-474X.2011.00417.x
79. Seppälä S, Laitinen T, Tarvainen MP, Tompuri T, Veijalainen A, Savonen K, et al. Normal values for heart rate variability parameters in children 6-8 years of age: the PANIC Study. Clin Physiol Funct Imaging. (2014) 34:290–6. doi: 10.1111/cpf.12096
80. Plews DJ, Laursen PB, Kilding AE, Buchheit M. Heart rate variability in elite triathletes, is variation in variability the key to effective training? A case comparison. Eur J Appl Physiol. (2012) 112:3729–41. doi: 10.1007/s00421-012-2354-4
81. Malik M. Heart rate variability: Standards of measurement, physiological interpretation, and clinical use: Task force of the European Society of Cardiology and the North American Society for Pacing and Electrophysiology. Ann Noninvasive Electrocardiol. (1996) 1:151–81. doi: 10.1111/j.1542-474X.1996.tb00275.x
82. Shaffer F, Ginsberg JP. An overview of heart rate variability metrics and norms. Front Public Health. (2017) 258. doi: 10.3389/fpubh.2017.00258
83. Downey LV, Zun LS. The effects of deep breathing training on pain management in the emergency department. South Med J. (2009) 102:688–92. doi: 10.1097/SMJ.0b013e3181a93fc5
84. Mohammed AR, Mohammed NS. Effect of breathing exercise on respiratory efficiency and pain intensity among children receiving chemotherapy. J Educ Pract. (2014) 5:18–32.
85. Miller KM. Deep breathing relaxation. AORN J. (1987) 45:484–8. doi: 10.1016/S0001-2092(07)68361-6
86. Lymeus F, Lindberg P, Hartig T. A natural meditation setting improves compliance with mindfulness training. J Environ Psychol. (2019) 64:98–106. doi: 10.1016/j.jenvp.2019.05.008
87. Zeng X, Chio F, Oei T, Leung F, Liu X. A systematic review of associations between amount of meditation practice and outcomes in interventions using the four immeasurables meditations. Front Psychol. (2017) 8:141. doi: 10.3389/fpsyg.2017.00141
88. Cohn M, Fredrickson B. In search of durable positive psychology interventions: predictors and consequences of long-term positive behavior change. J Posit Psychol. (2010) 5:355–66. doi: 10.1080/17439760.2010.508883
89. Barbanti P, Grazzi L, Egeo G, Padovan AM, Liebler E, Bussone G. Non-invasive vagus nerve stimulation for acute treatment of high-frequency and chronic migraine: an open-label study. J Headache Pain. (2015) 16:61. doi: 10.1186/s10194-015-0542-4
Keywords: meditation, diaphragmatic breathing, vagus nerve, vagus nerve stimulation (VNS) therapy, chronic pain and fibromyalgia, heart rate variability (HRV), pain intensity
Citation: Paccione CE, Stubhaug A, Diep LM, Rosseland LA and Jacobsen HB (2022) Meditative-based diaphragmatic breathing vs. vagus nerve stimulation in the treatment of fibromyalgia—A randomized controlled trial: Body vs. machine. Front. Neurol. 13:1030927. doi: 10.3389/fneur.2022.1030927
Received: 31 August 2022; Accepted: 13 October 2022;
Published: 03 November 2022.
Edited by:
Florian Chouchou, Université de La Réunion, FranceReviewed by:
Chantal Verkindt, Université de La Réunion, FranceDavid Hupin, Karolinska Institutet (KI), Sweden
Copyright © 2022 Paccione, Stubhaug, Diep, Rosseland and Jacobsen. This is an open-access article distributed under the terms of the Creative Commons Attribution License (CC BY). The use, distribution or reproduction in other forums is permitted, provided the original author(s) and the copyright owner(s) are credited and that the original publication in this journal is cited, in accordance with accepted academic practice. No use, distribution or reproduction is permitted which does not comply with these terms.
*Correspondence: Charles Ethan Paccione, Q2hhcmxlcy5FdGhhbi5QYWNjaW9uZUBDb2x1bWJpYS5lZHU=