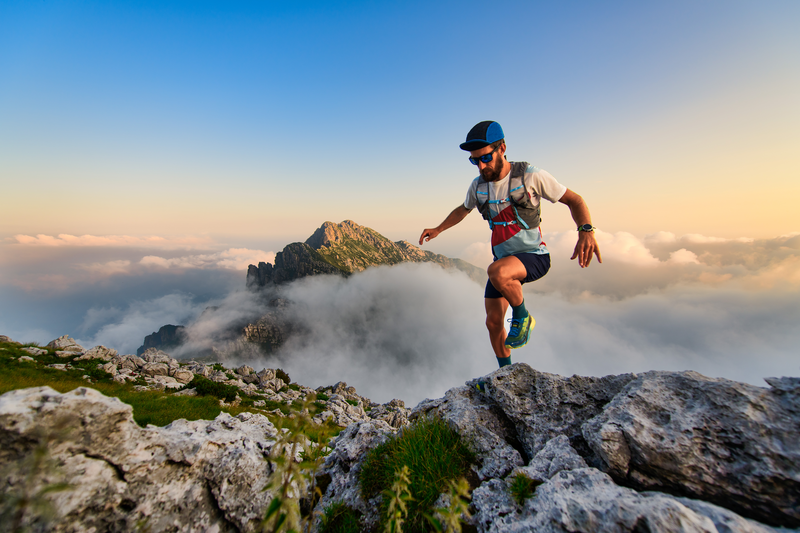
94% of researchers rate our articles as excellent or good
Learn more about the work of our research integrity team to safeguard the quality of each article we publish.
Find out more
MINI REVIEW article
Front. Neurol. , 13 January 2022
Sec. Pediatric Neurology
Volume 12 - 2021 | https://doi.org/10.3389/fneur.2021.806506
This article is part of the Research Topic Genetically Determined Epilepsies: Perspectives in the Era of Precision Medicine View all 19 articles
SNAREs (soluble N-ethylmaleimide sensitive factor attachment protein receptor) are an heterogeneous family of proteins that, together with their key regulators, are implicated in synaptic vesicle exocytosis and synaptic transmission. SNAREs represent the core component of this protein complex. Although the specific mechanisms of the SNARE machinery is still not completely uncovered, studies in recent years have provided a clearer understanding of the interactions regulating the essential fusion machinery for neurotransmitter release. Mutations in genes encoding SNARE proteins or SNARE complex associated proteins have been associated with a variable spectrum of neurological conditions that have been recently defined as “SNAREopathies.” These include neurodevelopmental disorder, autism spectrum disorder (ASD), movement disorders, seizures and epileptiform abnormalities. The SNARE phenotypic spectrum associated with seizures ranges from simple febrile seizures and infantile spasms, to severe early-onset epileptic encephalopathies. Our study aims to review and delineate the epileptic phenotypes associated with dysregulation of synaptic vesicle exocytosis and transmission, focusing on the main proteins of the SNARE core complex (STX1B, VAMP2, SNAP25), tethering complex (STXBP1), and related downstream regulators.
Epilepsy is defined as a large heterogeneous group of diseases in which individuals have an enduring predisposition to seizures (1), characterized by many different seizure types and epilepsy syndromes (2, 3). The term “epileptic encephalopathy” refers to a group of disorders in which unremitting epileptic activity contributes to progressive cerebral dysfunction (4). The epilepsies have a wide range of etiologies, which include genetic, metabolic, immune, and inflammatory factors; acquired or congenital brain abnormalities, infections, trauma or hypoxic-ischemic insults due to brain injuries (3). A genetic cause has been found in more than 50% of epilepsy phenotypes, particularly in developmental epileptic encephalopathies (5, 6). Pathogenic variants in genes encoding several voltage-gated K+, Na+, and Ca2+ channels subunits are the most common genetic cause of epileptic encephalopathies, accounting for a group of diseases defined as “channelopathies” (7). Another class of functionally related proteins have been discovered by James E. Rothman in 1994 and given the name SNARE (soluble N-ethylmaleimide sensitive factor attachment protein receptor) (8). Since then, several mutations in each subunit of the SNARE complex have been associated with an heterogenous group of neurological disorders, together referred to as SNAREopathies. SNAREs are a protein family involved in transport and release mechanisms of synaptic vesicles inside the neuron. They mediate the fusion of membranes by localizing both at the vesicular and target membrane. The core SNARE machinery consists of VAMP2 (synaptobrevin-2), the only vesicular binding SNARE (v-SNARE) of the complex and a combination of target membrane SNARE proteins (t-SNAREs): syntaxin1-A (STX1A) and synaptosomal-associated protein 25 kD (SNAP25) (9). The assembly of the SNARE machinery is carefully arranged by the assembly complex, which is composed of MUNC18-1 and MUNC13-1 and plays an essential role in the fusion of synaptic vesicles (10). Pathogenic biallelic and monoallelic variants disrupting proteins of the SNARE complex and associated regulators are a known cause for neurodevelopmental disorders consisting of an overlapping phenotype of developmental delay (DD), intellectual disability (ID), movement disorders, and epilepsy. The SNARE phenotypic spectrum associated with seizures ranges from simple febrile seizures and infantile spasms, to severe early-onset epileptic encephalopathies. Here we review the epileptic phenotypes associated with dysregulation of synaptic vesicle exocytosis and transmission, focusing on the main proteins of the SNARE core complex (STX1B, VAMP2, SNAP25), assembly complex (STXBP1, UNC13A), and related downstream regulators.
Sintaxin-1B (STX1B) codes for a presynaptic plasma membrane protein that belongs to the syntaxins family and is predominantly expressed in neurons. STX1B is part of the SNARE complex and its main role is to mediate the calcium-dependent synaptic vesicle release (11, 12). The crucial role of STX1B is underlined by studies performed on animal models. In fact, STX1B KO mice presented with impaired brain development, disrupted motor coordination, and only survived the first 14 days. Hippocampal cell cultures viability was lower compared to controls (13). Interestingly, heterozygous STX1B presented with a less severe phenotype (14), while heterozygous zebrafish models showed jerks and paroxysmal movements. Epileptic episodes were observed in approximately 50% of the animals, with increased events associated with higher temperature which is in concordance with the occurrence of febrile seizures (15).
A total of 40 different heterozygous or denovo mutations in STX1B have been described so far. These included 4 missense, 2 indels, 6 nonsense, 7 frameshift, 7 splice variants and 4 large indels (Table 1). Most of these mutations were predicted to cause haploinsufficiency of STX1B, resulting in early termination of the protein. Sixty-two individuals have been described so far and the mainly reported phenotype was epilepsy (Table 2) (15–28). Wolking et al. (23) divides STX1B-associated epileptic phenotypes in four different groups: (1) benign epilepsy syndrome with febrile and afebrile seizures corresponding to “genetic epilepsies with febrile seizures plus,” (2) “genetic generalized epilepsy” phenotype, (3) “developmental and epileptic encephalopathy” syndrome with refractory seizures and moderate to severe developmental deficits, and (4) focal epilepsy phenotype. Seizures have been described in almost all the individuals (61/62, 98%) and, where specified, they were the main symptom at onset in the majority of the individuals (43/48, 90%). Generalized seizures were the most common type of onset, presenting in 53 individuals (53/57, 93%), while focal-onset seizures occurred in 14 individuals (14/39, 36%). Seizure type ranged from tonic-clonic seizures (40/56, 71%) absence seizures (16/47, 34%), tonic or atonic seizures (24/56, 43%) to myoclonic seizures (16/30, 53%). Infantile spasms and status epilepticus were infrequent, both being reported in only two individuals. Out of the 47 electroencephalography recordings available, 42 showed epileptiform or non-epileptiform abnormalities (42/47, 89%).
Global developmental delay was documented in 15 individuals (15/47, 32%), and 23 individuals manifested various degrees of intellectual disability (23/59, 39%). Motor impairment, mainly ataxia, has been reported in 15 individuals (15/48, 31%). Behavioral or movement abnormalities were infrequent, accounting for 10% and 4% of the cases, respectively (5/45 and 2/51, respectively). When available, magnetic resonance imaging (MRI) was unremarkable for the majority of the cases (22/27, 81%).
VAMP2 encodes synaptobrevin-2, a major neuronal v-SNARE protein responsible for fusing synaptic vesicles at mammalian central nerve terminals (29, 30). VAMP2 KO mice presented with abnormal body shape and died shortly after birth, brain abnormalities were not detected (31). Moreover, synaptic vesicle observed under the electron microscope from VAMP2 KO mice presented abnormal morphology and size (32).
De novo mutations in VAMP2 have hitherto been reported in 11 individuals with neurodevelopmental disorder (Table 1) (33–35). VAMP2 has been first described as a causative gene by Salpietro et al. (33), who reported 5 unrelated individuals with de novo heterozygous mutations. Simmons (34) and Sunaga (35) further expanded the cohort with 6 additional unrelated individuals carrying de novo heterozygous mutations in VAMP2. The mutations described so far include 7 missense, 2 indels, 1 nonsense and 1 frameshift. The entire cohort of variants is localized in the highly conserved SNARE motif.
All the affected individuals showed moderate to severe global developmental delay and intellectual disability (11/11; 100%). Behavioral abnormalities, including Autism Spectrum Disorder (ASD) or Rett-like features, were virtually present in all the reported cases (9/9; 100%). Movement abnormalities were present in 6 individuals (6/11, 55%) and included chorea (n = 3), dystonia (n = 1), myoclonic jerks (n = 1), tremor (n = 1) and hyperkinetic movements (n = 1). Even though seizures have only been reported in 6 individuals (6/11, 55%), electroencephalography (EEG) recordings, where available, showed abnormalities in 7 individuals (7/9; 78%). Generalized-onset seizures were the most prevalent type, occuring in 5 individuals (5/6, 83%). Among those, seizure type varied from tonic-clonic (n = 2), tonic/atonic (n = 1) and myoclonic (n = 1). Infantile spasms have been reported in 3 individuals (3/6, 50%), two of them in the West syndrome spectrum. Focal seizures were less frequent, occurring in 3 individuals (3/6; 50%). Two individuals experienced status epilepticus (2/6, 33%). Magnetic resonance imaging was unremarkable for 6 individuals, otherwise MRI findings included corpus callosum thinning or hypoplasia (n = 2), periventricular FLAIR hyperintensities (n = 1) or mild brain atrophy (n = 1) (Table 2). Of the 11 individuals with de novo variants, seven different missense variants were identified, in addition to two single residue deletions and two stop gains. All variants were absent from GnomAD database blablabla to date, it seems that no clear correlation between the type of variant and the phenotype has been identified.
SNAP25 encodes the SNAP-25 protein, a t-snare widely expressed in the brain which is localized both at the presynaptic nerve terminal as well as to neuronal membranes (36). SNAP-25 is distinctive in the SNARE complex as it lacks a transmembrane domain and contains two SNARE motifs separated by a linker region (37). When trying to generate KO SNAP25 mice, it was observed that heterozygous mice did not present any significant differences in comparison to their wilt-type littermates. However, no homozygous SNAP25-/- were generated from the heterozygous crosses. Analysis of the homozygous fetuses revealed smaller size, absence of movements and blotchy appearance likely caused by vascular abnormalities of the skin. Morphology of the brain appeared normal (38). There have been 19 different de novo mutations identified so far in SNAP25 across 23 patients (39). Out of the total number of variants, 14 were missense, 2 nonsense and 2 splice-site (Table 1). The mutations are localized in the SNARE motifs and are predicted to disrupt the SNARE complex, but thorough functional studies are yet to be performed.
All individuals presented with global developmental delay and intellectual disability (23/23, 100%), ranging between profound (4/20; 20%), severe (5/20; 25%), moderate (6/20; 30%), and mild (5/20; 25%). Regression was reported in five individuals (5/17; 29%) with three of them showing signs of regression with the onset of seizures. All individuals showed variable degree of motor delay, with motor impairment being more evident in 12 individuals (12/15, 80%). Seizures have been reported in 17 individuals (17/23, 74%). The age of seizure onset ranged between the 7th day of life to 12 years. In 14 individuals, the onset was before 2 years of age. Most individuals showed a broad spectrum of epileptic spasms, generalized and focal seizures. Generalized-onset or focal to generalized-onset seizures were the most frequently occuring, being reported in 11 individuals (11/17, 65%), while focal seizures were described in 6 individuals (6/17, 35%). Seizure spectrum included tonic-clonic (n = 7), absence (n = 6), tonic or atonic (n = 4), myoclonic (n = 4) seizures and epileptic spasms (n = 5). Status epilepticus was reported in only one individual. EEG abnormal findings, generally multifocal epileptic discharges and generalized spike wave discharges, were documented in 15 out of the 16 available records (15/16, 94%). Less frequently reported features were abnormal movements (5/21, 24%) and behavioral abnormalities (3/18, 17%). MRI was performed on 21 individuals and was unremarkable in 15 individuals (15/21, 71%) (Table 2).
MUNC18-1, also known as Syntaxin-binding protein-1 (STXBP1), belongs to the Sec1/Munc18 (SM) family. MUNC18-1, together with MUNC13-1, plays a crucial role in the SNARE complex assembly. More specifically, MUNC18-1 forms an inactive complex with syntaxin-1 to secure correct positioning of the latter. The formation of this complex is likely to represent the initial event for synaptic vesicle fusion (40).
KO mice have shown severe phenotype, dying immediately after birth and with no neurotransmission activity recorded. Moreover, degeneration was observed from cultured neurons from these mice (41, 42). Mutations in STXBP1 are the most commonly reported in literature, with a large difference in numbers compared to mutations in other SNARE proteins. So far, 163 distinct mutations and 41 large indels have been identified in STXBP1. The majority of this group is composed of 59 missenses, followed by 40 frameshift, 34 splice-site, 28 nonsense and 2 indels (Table 1). When missense variants are compared to all other types of mutations, there is no correlation to presence or absence of epilepsy. Cases reported to date were monoallelic, with the exception of two recently described siblings carrying a biallelic STXBP1 L446F mutation (43).
More than 400 cases have been reported so far (19, 44–103). However, for many individuals, detailed clinical information was not available. Nevertheless, the key clinical findings in STXBP1-spectrum comprised global developmental delay and/or intellectual disability, seizures and variable presence of movement disorder, motor impairment or behavioral abnormalities. The most commonly described clinical feature is global developmental delay, presenting in 95% of the patients for whom information was available (297/313, 95%). The range of intellectual disability may vary: Stamberger et al. (56) reported that more than 80% of the individuals described until then presented with severe to profound intellectual disability.
Seizures were reported in 401 individuals (401/446, ~90%). A wide spectrum of seizure types was described in most individuals. Where specified, epileptic spasms frequently occurred at some stage during the disease course (162/260, ~63%). Other frequent seizure types were generalized-onset seizures (108/159, ~68%) and focal seizures (140/274, ~51%). Almost 90% of seizures occur in early infancy as the first symptom. When performed, EEG was reported abnormal in 71% of the cases (160/226, ~71%).
Other less commonly reported features include motor impairment [143/261 (55%)], movement disorders [130/261 (50%)] and behavioral abnormalities [96/274 (35%)]. In 146 of the 257 individuals for whom brain magnetic resonance imaging (MRI) was available, no abnormalities were documented (146/257, ~57%) (Table 2).
MUNC13-1 is a protein encoded by UNC13A and is highly expressed in the hippocampus, cerebellum, cortex, striatum, and olfactory bulb (104). By binding both to synaptobrevin-2 and syntaxin-1, it aids in the formation of the SNARE complex, thus making synaptobrevin-2 more accessible by the MUNC18-1/syntaxin-1 formation (105).
Studies on MUNC13-1 KO mice have shown absence of evoked and spontaneous excitatory and inhibitory neurotransmitter release and synapses reduction in docked vesicles (106, 107). Until now, only two cases have been reported with mutations in MUNC13-1 (108, 109). A homozygous nonsense mutation (p.Gln102Ter) in UNC13A was identified in a girl with microcephaly, cortical hyperexcitability, fatal myasthenia, global developmental delay and intellectual disability. Seizures were not reported, but EEG showed abnormalities. MRI documented thinning of corpus callosum. Subsequently, a de novo heterozygous missense mutation was identified in a boy with dyskinetic movement disorder, developmental delay, intellectual disability, autism and ADHD, who also experienced febrile seizures.
Epileptic phenotypes have also been associated with mutations in other SNARE-associated proteins, here we review GOSR2, SNAP29, STXBP5L, and CPLX1. The Golgi snap receptor complex member 2 (GOSR2), is part of a complex responsible for docking and fusion of newly synthesized proteins from the endoplasmic reticulum (110). To date, seven different biallelic variants have been reported in GOSR2 (Table 1). All individuals share a similar phenotype with myoclonus epilepsy, ataxia, and usually relatively preserved cognition. SNAP29, acting in the autophagosome-lysosome fusion (111), has been found to harbor 10 distinct biallelic mutations (Table 1). Namely, 5 frameshifts, 2 nonsense and 3 missense (112). Mutations in SNAP29 have been linked to CEDNIK syndrome, whose clinical features include microcephaly, severe neurologic impairment, psychomotor retardation, failure to thrive, and facial dysmorphism, as well as palmoplantar keratoderma and late-onset ichthyosis (113). Global developmental delay and intellectual disability have been reported in all the affected individuals. Seizures are not very common and have been described in 9 of the 25 reported individuals (9/25, ~36%).
The function of STXBP5L is still not clear, but it has been observed it plays a role in the inhibition of the formation of the SNARE complex. Only one homozygous missense variant has been reported so far in two siblings with seizures, global developmental delay and MRI abnormalities (114, 115). Complexin-1 (CPLX1) is a neuronal protein of the SNARE complex, which contributes to vesicle fusing. To date, 2 nonsense and 1 missense biallelic mutations have been reported in the gene. Only five cases have been described so far, all presenting with seizures and global developmental delay (116, 117).
In the previous paragraphs, the epileptic syndromes associated with mutations or variants in the SNARE complex were briefly reviewed. Since the identification of SNARE proteins, many studies have focused on the role of the single molecules within the whole complex. Mutations in each subunit of the complex and in the related upstream and downstream regulators have been identified in a heterogeneous group of disorders, mostly neurological disorders. We focused on the association between the SNARE complex and seizures. Only four proteins involved in the synaptic vesicle fusion have not been linked to epileptic phenotypes: α-synuclein, synaptobrevin-1, and synaptotagmin-1 and -2.
Deficits in the subunits of the core complex (synaptobrevin-2, syntaxin-1B and SNAP-25), Munc18-1 and complexin-1 are mainly associated with an overlapping spectrum of developmental delay, intellectual disability, epilepsy, and movement disorders. Overall, the most reported feature is epilepsy, presenting in almost 90% of the individuals with a SNARE dysfunction. Particularly, mutations in syntaxin-1B are most associated with epileptic phenotypes. The type of seizures may vary from generalized tonic-clonic, myoclonic or absence seizures to focal seizures. Infantile spasms or West syndrome were also reported in association with SNARE dysfunction. The epileptic phenotypes associated with the main vesicle fusion machinery have been characterized in Table 2. Given the significant overlap in seizure semiology, it is not possible to differentiate the genetic cause based on seizure type. Global developmental delay and intellectual disability are also frequent features, presenting in 85% of the individuals. However, dysfunctions in STX1B and GOSR2 are less commonly associated with developmental delay, as most of the affected individuals don't show intellectual impairment. Motor impairment and movement abnormalities, including ataxia, gait abnormalities, tremor, hyperkinetic movements, chorea and myoclonus, are variably present, affecting almost half of the individuals. Behavioral abnormalities, comprising Autism Spectrum Disorder, Rett-like phenotypes and stereotypies, were less commonly documented in the cohort. Brain MRI was performed and reported as normal in more than 50% of the overall cohort. We comprehensively reported the total cohort of mutations identified so far in the genes that form the core SNARE complex and some associated proteins. The zygosity of genes in the core SNARE complex was heterozygous or de novo, indicating the crucial role of these proteins. Studies on animal models have confirmed this, by showing that homozygous KO animal models are either incompatible with life or severely affected, not surviving their first days. Therefore, it is likely that the early onset of the epileptic phenotype might be the consequence of the disruption of the neurotransmitters release machinery. Except for STXBP1, where only one biallelic variant was reported out of the total 209, all other discussed genes presented biallelic and monoallelic variants. In conclusion, we comprehensively described a cohort of more than 600 individuals affected with dysfunction in proteins of the SNARE complex or synaptic vesicle machinery. We illustrated the phenotypic spectrum of the SNARE-associated disorders and focused on the epileptic phenotypes. This review underlines the key role of SNARE proteins in the pathogenicity of epilepsy and the prevalence of this phenotype. Limitations of this study are mainly attributable to its retrospective nature. One important limitation has been the lack of precise information on the main phenotype and on the neuroradiological features, particularly when the mutation was reported in big cohort studies. Clinical features were summarized through percentages, but we cannot exclude the risk of under or overestimation. We reviewed the function of the main SNARE proteins, taking in consideration the consequence of their disruption in animal models. However, to better understand the pathways involved in these disease mechanisms, further functional studies will be required.
EC, CR, VS, and HH: conceptualization, writing—review, and editing. HH: funding acquisition. EC and CR: writing—original draft. All authors contributed to the article and approved the submitted version.
The authors declare that the research was conducted in the absence of any commercial or financial relationships that could be construed as a potential conflict of interest.
All claims expressed in this article are solely those of the authors and do not necessarily represent those of their affiliated organizations, or those of the publisher, the editors and the reviewers. Any product that may be evaluated in this article, or claim that may be made by its manufacturer, is not guaranteed or endorsed by the publisher.
This research was supported by the Wellcome Trust, Medical Research Council, NIHR BRC and UK Research and Innovation.
The Supplementary Material for this article can be found online at: https://www.frontiersin.org/articles/10.3389/fneur.2021.806506/full#supplementary-material
1. Fisher RS, Acevedo C, Arzimanoglou A, Bogacz A, Cross JH, Elger CE, et al. ILAE Official Report: a practical clinical definition of epilepsy. Epilepsia. (2014) 55:475–82. doi: 10.1111/epi.12550
2. Fisher RS, Cross JH, French JA, Higurashi N, Hirsch E, Jansen FE, et al. Operational classification of seizure types by the International League against epilepsy: position paper of the ILAE Commission for Classification and Terminology. Epilepsia. (2017) 58:522–30. doi: 10.1111/epi.13670
3. Scheffer IE, Berkovic S, Capovilla G, Connolly MB, French J, Guilhoto L, et al. ILAE classification of the epilepsies: position paper of the ILAE Commission for Classification and Terminology. Epilepsia. (2017) 58:512–21. doi: 10.1111/epi.13709
4. Berg AT, Berkovic SF, Brodie MJ, Buchhalter J, Cross JH, van Emde Boas W, et al. Revised terminology and concepts for organization of seizures and epilepsies: report of the ILAE Commission on Classification and Terminology, 2005–2009. Epilepsia. (2010) 51:676–85. doi: 10.1111/j.1528-1167.2010.02522.x
5. Shellhaas RA, Wusthoff CJ, Tsuchida TN, Glass HC, Chu CJ, Massey SL, et al. Profile of neonatal epilepsies. Neurology. (2017) 89:893–9. doi: 10.1212/WNL.0000000000004284
6. Palmer EE, Schofield D, Shrestha R, Kandula T, Macintosh R, Lawson JA, et al. Integrating exome sequencing into a diagnostic pathway for epileptic encephalopathy: Evidence of clinical utility and cost effectiveness. Mol Genet Genomic Med. (2018) 6:186–99. doi: 10.1002/mgg3.355
7. Myers KA, Scheffer IE. Precision medicine approaches for infantile-onset developmental and epileptic encephalopathies. Annu Rev Pharmacol Toxicol. (2021) 62:84449. doi: 10.1146/annurev-pharmtox-052120-084449
8. Rothman JE, Warren G. Implications of the SNARE hypothesis for intracellular membrane topology and dynamics. Curr Biol. (1994) 4:220–33. doi: 10.1016/s0960-9822(00)00051-8
9. Söllner T, Whiteheart SW, Brunner M, Erdjument-Bromage H, Geromanos S, Tempst P, et al. SNAP receptors implicated in vesicle targeting and fusion. Nature. (1993) 362:318–24. doi: 10.1038/362318a0
10. Zhang Y, Hughson FM. Chaperoning SNARE Folding and Assembly. Annu Rev Biochem. (2021) 90:581–603. doi: 10.1146/annurev-biochem-081820-103615
11. Smirnova T, Miniou P, Viegas-Pequignot E, Mallet J. Assignment of the human syntaxin 1B gene (STX) to chromosome 16p11.2 by fluorescence in situ hybridization. Genomics. (1996) 36:551–3. doi: 10.1006/geno.1996.0506
12. Mishima T, Fujiwara T, Kofuji T, Saito A, Terao Y, Akagawa K. Syntaxin 1B regulates synaptic GABA release and extracellular GABA concentration, and is associated with temperature-dependent seizures. J Neurochem. (2021) 156:604–13. doi: 10.1111/jnc.15159
13. Kofuji T, Fujiwara T, Sanada M, Mishima T, Akagawa K. HPC-1/syntaxin 1A and syntaxin 1B play distinct roles in neuronal survival. J Neurochem. (2014) 130:514–25. doi: 10.1111/jnc.12722
14. Wu YJ, Tejero R, Arancillo M, Vardar G, Korotkova T, Kintscher M, et al. Syntaxin 1B is important for mouse postnatal survival and proper synaptic function at the mouse neuromuscular junctions. J Neurophysiol. (2015) 114:2404–17. doi: 10.1152/jn.00577.2015
15. Schubert J, Siekierska A, Langlois M, May P, Huneau C, Becker F, et al. Mutations in STX1B, encoding a presynaptic protein, cause fever-associated epilepsy syndromes. Nat Genet. (2014) 46:1327–32. doi: 10.1038/ng.3130
16. Lerche H, Weber YG, Baier H, Jurkat-Rott K, Kraus de Camargo O, Ludolph AC, et al. Generalized epilepsy with febrile seizures plus: further heterogeneity in a large family. Neurology. (2001) 57:1191–98. doi: 10.1212/WNL.57.7.1191
17. Weber YG, Jacob M, Weber G, Lerche H. A BFIS-like syndrome with late onset and febrile seizures:suggestive linkage to chromosome 16p11.2-16q12.1. Epilepsia. (2008) 49:1959-64. doi: 10.1111/j.1528-1167.2008.01646.x
18. Vlaskamp DRM, Rump P, Callenbach PMC, Vos YJ, Sikkema-Raddatz B, van Ravenswaaij-Arts CMA, et al. Haploinsufficiency of the STX1B gene is associated with myoclonic astatic epilepsy. Eur J Paediatr Neurol. (2016) 20:489–92. doi: 10.1016/j.ejpn.2015.12.014
19. Oates S, Tang S, Rosch R, Lear R, Hughes EF, Williams RE, et al. Incorporating epilepsy genetics into clinical practice: a 360°evaluation. NPJ Genomic Med. (2018) 3:1–11. doi: 10.1038/s41525-018-0052-9
20. Peres J, Antunes F, Zonjy B, Mitchell AL, Lhatoo SD. Sleep-related hypermotor epilepsy and peri-ictal hypotension in a patient with syntaxin-1B mutation. Epileptic Disord. (2018) 20:413–7. doi: 10.1684/epd.2018.0996
21. Borlot F, Almeida BI, Combe SL, Andrade DM, Filloux FM, Myers KA. Clinical utility of multigene panel testing in adults with epilepsy and intellectual disability. Epilepsia. (2019) 60:1661–9. doi: 10.1111/epi.16273
22. Tian Y, Hou C, Wang XY, Yang ZX, Ma YL, Cao BB, et al. A novel inherited STX1B mutation associated with generalized epilepsy with febrile seizures plus: a family analysis and literature review. Chinese J Pediatr. (2019) 57:206–10. doi: 10.3760/cma.j.issn.0578-1310.2019.03.010
23. Wolking S, May P, Mei D, Møller RS, Balestrini S, Helbig KL, et al. Clinical spectrum of STX1B-related epileptic disorders. Neurology. (2019) 92: e1238–49. doi: 10.1212/WNL.0000000000007089
24. Krenn M, Wagner M, Hotzy C, Graf E, Weber S, Brunet T, et al. Diagnostic exome sequencing in non-acquired focal epilepsies highlights a major role of GATOR1 complex genes. J Med Genet. (2020) 57:624–33. doi: 10.1136/jmedgenet-2019-106658
25. Tang S, Addis L, Smith A, Topp SD, Pendziwiat M, Mei D, et al. Phenotypic and genetic spectrum of epilepsy with myoclonic atonic seizures. Epilepsia. (2020) 61:995–1007. doi: 10.1111/epi.16508
26. Burghardt K, Baba N, Schreyer I, Graneß I, Hübner CA. STX1B-related epilepsy in a 24-month-old female infant. Epilepsy Behav. Rep. (2021) 15:10039. doi: 10.1016/j.ebr.2020.100391
27. Krenn M, Schloegl M, Pataraia E, Gelpi E, Schröder S, Rauscher C, et al. Delineation of epileptic and neurodevelopmental phenotypes associated with variants in STX1B. Seizure. (2021) 87:25–9. doi: 10.1016/j.seizure.2021.02.027
28. Liu Y-H, Cheng Y-T, Tsai M-H, Chou I-J, Hung P-C, Hsieh M-Y, et al. Genetics and clinical correlation of Dravet syndrome and its mimics—experience of a tertiary center in Taiwan. Pediatr Neonatol. (2021) 62:550–8. doi: 10.1016/j.pedneo.2021.05.022
29. Rothman JE, Söllner TH. Throttles and dampers: controlling the engine of membrane fusion. Science. (1997) 276:1212–3. doi: 10.1126/science.276.5316.1212
30. Weber T, Zemelman BV, McNew JA, Westermann B, Gmachl M, Parlati F, et al. SNAREpins: minimal machinery for membrane fusion. Cell. (1998) 92:759–72. doi: 10.1016/s0092-8674(00)81404-x
31. Schoch S, Deák F, Königstorfer A, Mozhayeva M, Sara Y, Südhof TC, et al. SNARE function analyzed in synaptobrevin/VAMP knockout mice. Science. (2001) 294:1117–22. doi: 10.1126/science.1064335
32. Deák F, Schoch S, Liu X, Südhof TC, Kavalali ET. Synaptobrevin is essential for fast synaptic-vesicle endocytosis. Nat Cell Biol. (2004) 6:1102–8. doi: 10.1038/ncb1185
33. Salpietro V, Malintan NT, Llano-Rivas I, Spaeth CG, Efthymiou S, Striano P, et al. Mutations in the neuronal vesicular SNARE VAMP2 affect synaptic membrane fusion and impair human neurodevelopment. Am J Hum Genet. (2019) 104:721–30. doi: 10.1016/j.ajhg.2019.02.016
34. Simmons RL, Li H, Alten B, Santos MS, Jiang R, Paul B, et al. Overcoming presynaptic effects of VAMP2 mutations with 4-aminopyridine treatment. Hum Mutat. (2020) 41:1999–2011. doi: 10.1002/humu.24109
35. Sunaga Y, Muramatsu K, Kosaki K, Sugai K, Mizuno T, Kouno M, et al. Variant in the neuronal vesicular SNARE VAMP2 (synaptobrevin-2): first report in Japan. Brain Dev. (2020) 42:529–33. doi: 10.1016/j.braindev.2020.04.001
36. Chilcote TJ, Galli T, Mundigl O, Edelmann L, McPherson PS, Takei K, et al. Cellubrevin and synaptobrevins: similar subcellular localization and biochemical properties in PC12 cells. J Cell Biol. (1995) 129:219–31. doi: 10.1083/jcb.129.1.219
37. Veit M, Söllner TH, Rothman JE. Multiple palmitoylation of synaptotagmin and the t-SNARE SNAP-25. FEBS Lett. (1996) 385:119–23. doi: 10.1016/0014-5793(96)00362-6
38. Washbourne P, Thompson PM, Carta M, Costa ET, Mathews JR, Lopez-Benditó G, et al. Genetic ablation of the t-SNARE SNAP-25 distinguishes mechanisms of neuroexocytosis. Nat Neurosci. (2002) 5:19–26. doi: 10.1038/nn783
39. Klöckner C, Sticht H, Zacher P, Popp B, Babcock HE, Bakker DP, et al. De novo variants in SNAP25 cause an early-onset developmental and epileptic encephalopathy. Genet Med. (2021) 23:653–60. doi: 10.1038/s41436-020-01020-w
40. Dulubova I, Sugita S, Hill S, Hosaka M, Fernandez I, Südhof TC, et al. A conformational switch in syntaxin during exocytosis: role of munc18. EMBO J. (1999) 18:4372–82. doi: 10.1093/emboj/18.16.4372
41. Verhage M, Maia AS, Plomp JJ, Brussaard AB, Heeroma JH, Vermeer H, et al. Synaptic assembly of the brain in the absence of neurotransmitter secretion. Science. (2000) 287:864–9. doi: 10.1126/science.287.5454.864
42. Heeroma JH, Roelandse M, Wierda K, van Aerde KI, Toonen RF, Hensbroek RA, et al. Trophic support delays but does not prevent cell-intrinsic degeneration of neurons deficient for munc18-1. Eur J Neurosci. (2004) 20:623–34. doi: 10.1111/j.1460-9568.2004.03503.x
43. Lammertse HCA, Van Berkel AA, Iacomino M, Toonen RF, Striano P, Gambardella A, et al. Homozygous STXBP1 variant causes encephalopathy and gain-of-function in synaptic transmission. Brain. (2020) 143:441–51. doi: 10.1093/brain/awz391
44. Ehret JK, Engels H, Cremer K, Becker J, Zimmermann JP, Wohlleber E, et al. Microdeletions in 9q33.3-q34.11 in five patients with intellectual disability, microcephaly, and seizures of incomplete penetrance: Is STXBP1 not the only causative gene? Mol Cytogenet. (2015) 8:1–14. doi: 10.1186/s13039-015-0178-8
45. Di Meglio C, Lesca G, Villeneuve N, Lacoste C, Abidi A, Cacciagli P, et al. Epileptic patients with de novo STXBP1 mutations: key clinical features based on 24 cases. Epilepsia. (2015) 56:1931–40. doi: 10.1111/epi.13214
46. Allen NM, Conroy J, Shahwan A, Lynch B, Correa RG, Pena SDJ, et al. Unexplained early onset epileptic encephalopathy: exome screening and phenotype expansion. Epilepsia. (2016) 57:e12–7. doi: 10.1111/epi.13250
47. Gburek-Augustat J, Beck-Woedl S, Tzschach A, Bauer P, Schoening M, Riess A. Epilepsy is not a mandatory feature of STXBP1 associated ataxia-tremor-retardation syndrome. Eur J Paediatr Neurol. (2016) 20:661–5. doi: 10.1016/j.ejpn.2016.04.005
48. Guacci A, Chetta M, Rizzo F, Marchese G, Filippo MR, De Giurato G, et al. Phenytoin neurotoxicity in a child carrying new STXBP1 and CYP2C9 gene mutations. Seizure. (2016) 34:26–8. doi: 10.1016/j.seizure.2015.11.004
49. Helbig KL, Farwell Hagman KD, Shinde DN, Mroske C, Powis Z, Li S, et al. Diagnostic exome sequencing provides a molecular diagnosis for a significant proportion of patients with epilepsy. Genet Med. (2016) 18:898–905. doi: 10.1038/gim.2015.186
50. Li D, Bhoj E, McCormick E, Wang F, Snyder J, Wang T, et al. Early infantile epileptic encephalopathy in an STXBP1 patient with lactic acidemia and normal mitochondrial respiratory chain function. Case Rep Genet. (2016) 2016:1–5. doi: 10.1155/2016/4140780
51. Li T, Cheng M, Wang J, Hong S, Li M, Liao S, et al. De novo mutations of STXBP1 in Chinese children with early onset epileptic encephalopathy. Genes Brain Behav. (2018) 17:e12492. doi: 10.1111/gbb.12492
52. Li Y, Jiang L, Wang L, Wang C, Liu C, Guo A, et al. p.His16Arg of STXBP1 (MUNC18-1) Associated with syntaxin 3B causes autosomal dominant congenital nystagmus. Front Cell Dev Biol. (2020) 8:5917781. doi: 10.3389/fcell.2020.591781
53. Lopes F, Barbosa M, Ameur A, Soares G, De SJ, Dias AI, et al. Identification of novel genetic causes of Rett syndrome-like phenotypes. J Med Genet. (2016) 53:190–9. doi: 10.1136/jmedgenet-2015
54. Ortega-Moreno L, Giráldez BG, Verdú A, García-Campos O, Sánchez-Martín G, Serratosa JM, et al. Novel mutation in STXBP1 gene in a patient with non-lesional Ohtahara syndrome. Neurology. (2016) 31:523–527. doi: 10.1016/j.nrleng.2014.10.004
55. Ortega-Moreno L, Giráldez BG, Soto-Insuga V, Pozo RL, Del Rodrigo-Moreno M, Alarcón-Morcillo C, et al. Molecular diagnosis of patients with epilepsy and developmental delay using a customized panel of epilepsy genes. PLoS ONE. (2017) 12:188978. doi: 10.1371/journal.pone.0188978
56. Stamberger H, Nikanorova M, Willemsen MH, Accorsi P, Angriman M, Baier H, et al. STXBP1 encephalopathy A neurodevelopmental disorder including epilepsy (2016). Available online at: http://exac.broadinstitute.org/ (accessed October 2021).
57. Trump N, McTague A, Brittain H, Papandreou A, Meyer E, Ngoh A, et al. Improving diagnosis and broadening the phenotypes in early-onset seizure and severe developmental delay disorders through gene panel analysis. J Med Genet. (2016) 53:310–7. doi: 10.1136/jmedgenet-2015-103263
58. Wang T, Guo H, Xiong B, Stessman HAF, Wu H, Coe BP, et al. De novo genic mutations among a Chinese autism spectrum disorder cohort. Nat Commun. (2016) 7:1–10. doi: 10.1038/ncomms13316
59. Wang J, Jiang L, Cheng M. A girl with protein-losing enteropathy during a ketogenic diet: a case report. BMC Pediatr. (2020) 20:1–4. doi: 10.1186/s12887-020-1991-8
60. Gokben S, Onay H, Yilmaz S, Atik T, Serdaroglu G, Tekin H, et al. Targeted next generation sequencing: the diagnostic value in early-onset epileptic encephalopathy. Acta Neurol Belg. (2017) 117:131–8. doi: 10.1007/s13760-016-0709-z
61. Arafat A, Jing P, Ma Y, Pu M, Nan G, Fang H, et al. Unexplained early infantile epileptic encephalopathy in Han Chinese Children: next-generation sequencing and phenotype enriching. Sci Rep. (2017) 7:1–10. doi: 10.1038/srep46227
62. Olson HE, Kelly M, LaCoursiere CM, Pinsky R, Tambunan D, Shain C, et al. Genetics and genotype–phenotype correlations in early onset epileptic encephalopathy with burst suppression. Ann Neurol. (2017) 81:419–29. doi: 10.1002/ana.24883
63. Parrini E, Marini C, Mei D, Galuppi A, Cellini E, Pucatti D, et al. Diagnostic targeted resequencing in 349 patients with drug-resistant pediatric epilepsies identifies causative mutations in 30 different genes. Hum Mutat. (2017) 38:216–25. doi: 10.1002/humu.23149
64. Shimojima K, Okamoto N, Goel H, Ondo Y, Yamamoto T. Familial 9q33q34 microduplication in siblings with developmental disorders and macrocephaly. Eur J Med Genet. (2017) 60:650–4. doi: 10.1016/j.ejmg.2017.08.017
65. Suri M, Evers JMG, Laskowski RA, O'Brien S, Baker K, Clayton-Smith J, et al. Protein structure and phenotypic analysis of pathogenic and population missense variants in STXBP1. Mol Genet Genomic Med. (2017) 5:495–507. doi: 10.1002/mgg3.304
66. Uddin M, Woodbury-Smith M, Chan A, Brunga L, Lamoureux S, Pellecchia G, et al. Germline and somatic mutations in STXBP1 with diverse neurodevelopmental phenotypes. Neurol Genet. (2017) 3:199. doi: 10.1212/NXG.0000000000000199
67. Weng Y, Du X, Bin R, Yu S, Xia Z, Zheng G, et al. Genetic variants identified from epilepsy of unknown etiology in chinese children by targeted exome sequencing. Sci Rep. (2017) 7:1–11. doi: 10.1038/srep40319
68. Butler KM, da Silva C, Alexander JJ, Hegde M, Escayg A. Diagnostic yield from 339 epilepsy patients screened on a clinical gene panel. Pediatr Neurol. (2017) 77:61–6. doi: 10.1016/j.pediatrneurol.2017.09.003
69. Álvarez Bravo G, Yusta Izquierdo A. The adult motor phenotype of Dravet syndrome is associated with mutation of the STXBP1 gene and responds well to cannabidiol treatment. Seizure. (2018) 60:68–70. doi: 10.1016/j.seizure.2018.06.010
70. Ko A, Youn SE, Kim SH, Lee JS, Kim S, Choi JR, et al. Targeted gene panel and genotype-phenotype correlation in children with developmental and epileptic encephalopathy. Epilepsy Res. (2018) 141:48–55. doi: 10.1016/j.eplepsyres.2018.02.003
71. Lindy AS, Stosser MB, Butler E, Downtain-Pickersgill C, Shanmugham A, Retterer K, et al. Diagnostic outcomes for genetic testing of 70 genes in 8565 patients with epilepsy and neurodevelopmental disorders. Epilepsia. (2018) 59:1062–71. doi: 10.1111/epi.14074
72. Liu S, Wang L, Cai XT, Zhou H, Yu D, Wang Z. Therapeutic benefits of ACTH and levetiracetam in STXBP1 encephalopathy with a de novo mutation. Med. (2018) 97:10663. doi: 10.1097/MD.0000000000010663
73. Liu J, Tong L, Song S, Niu Y, Li J, Wu X, et al. Novel and de novo mutations in pediatric refractory epilepsy. Mol Brain. (2018) 11:1–18. doi: 10.1186/s13041-018-0392-5
74. Liu L, Liu F, Wang Q, Xie H, Li Z, Lu Q, et al. Confirming the contribution and genetic spectrum of de novo mutation in infantile spasms: evidence from a Chinese cohort. Mol Genet Genomic Med. (2021) 9:1689. doi: 10.1002/mgg3.1689
75. Liu X, Shen Q, Zheng G, Guo H, Lu X, Wang X, et al. Gene and phenotype expansion of unexplained early infantile epileptic encephalopathy. Front Neurol. (2021) 12:633637. doi: 10.3389/fneur.2021.633637
76. Aravindhan A, Shah K, Pak J, Veerapandiyan A. Early-onset epileptic encephalopathy with myoclonic seizures related to 9q33.3-q34.11 deletion involving STXBP1 and SPTAN1 genes. Epileptic Disord. (2018) 20:214–218. doi: 10.1684/epd.2018.0969
77. Miao P, Feng J, Guo Y, Wang J, Xu X, Wang Y, et al. Genotype and phenotype analysis using an epilepsy-associated gene panel in Chinese pediatric epilepsy patients. Clin Genet. (2018) 94:512–20. doi: 10.1111/cge.13441
78. Yuge K, Iwama K, Yonee C, Matsufuji M, Sano N, Saikusa T, et al. A novel STXBP1 mutation causes typical Rett syndrome in a Japanese girl. Brain Dev. (2018) 40:493–7. doi: 10.1016/j.braindev.2018.02.002
79. Zhou P, He N, Zhang JW, Lin ZJ, Wang J, Yan LM, et al. Novel mutations and phenotypes of epilepsy-associated genes in epileptic encephalopathies. Genes Brain Behav. (2018) 17:e12456. doi: 10.1111/gbb.12456
80. Muir AM, Myers CT, Nguyen NT, Saykally J, Craiu D, De Jonghe P, et al. Genetic heterogeneity in infantile spasms. Epilepsy Res. (2019) 156:106181. doi: 10.1016/j.eplepsyres.2019.106181
81. O'Brien S, Ng-Cordell E, Astle DE, Scerif G, Baker K. STXBP1-associated neurodevelopmental disorder: a comparative study of behavioral characteristics. J Neurodev Disord. (2019) 11:1–11. doi: 10.1186/s11689-019-9278-9
82. Rezazadeh A, Uddin M, Snead OC, Lira V, Silberberg A, Weiss S, et al. STXBP1 encephalopathy is associated with awake bruxism. Epilepsy Behav. (2019) 92:121–4. doi: 10.1016/j.yebeh.2018.12.018
83. Schönewolf-Greulich B, Bisgaard AM, Møller RS, Dunø M, Brøndum-Nielsen K, Kaur S, et al. Clinician's guide to genes associated with Rett-like phenotypes—investigation of a Danish cohort and review of the literature. Clin. Genet. (2019) 95:221–230. doi: 10.1111/cge.13153
84. Valence S, Cochet E, Rougeot C, Garel C, Chantot-Bastaraud S, Lainey E, et al. Exome sequencing in congenital ataxia identifies two new candidate genes and highlights a pathophysiological link between some congenital ataxias and early infantile epileptic encephalopathies. Genet Med. (2019) 21:553–63. doi: 10.1038/s41436-018-0089-2
85. Vidal S, Brandi N, Pacheco P, Maynou J, Fernandez G, Xiol C, et al. The most recurrent monogenic disorders that overlap with the phenotype of Rett syndrome. Eur J Paediatr Neurol. (2019) 23:609–20. doi: 10.1016/j.ejpn.2019.04.006
86. Yamamoto T, Imaizumi T, Yamamoto-Shimojima K, Lu Y, Yanagishita T, Shimada S, et al. Genomic backgrounds of Japanese patients with undiagnosed neurodevelopmental disorders. Brain Dev. (2019) 41:776–82. doi: 10.1016/j.braindev.2019.05.007
87. Zevenbergen C, Groeneweg S, Swagemakers SMA, De Jong A, Medici-Van Den Herik E, Rispens M, et al. Functional analysis of genetic variation in the SECIS element of thyroid hormone activating type 2 deiodinase. J Clin Endocrinol Metab. (2019) 104:1369–77. doi: 10.1210/jc.2018-01605
88. Chen X, Jin J, Wang Q, Xue H, Zhang N, Du Y, et al. A de novo pathogenic CSNK1E mutation identified by exome sequencing in family trios with epileptic encephalopathy. Hum Mutat. (2019) 40:281–7. doi: 10.1002/humu.23690
89. Cogliati F, Giorgini V, Masciadri M, Bonati MT, Marchi M, Cracco I, et al. Pathogenic variants in STXBP1 and in genes for GABAa receptor subunities cause atypical rett/rett-like phenotypes. Int J Mol Sci. (2019) 20:3621. doi: 10.3390/ijms20153621
90. Johannesen KM, Nikanorova N, Marjanovic D, Pavbro A, Larsen LHG, Rubboli G, et al. Utility of genetic testing for therapeutic decision-making in adults with epilepsy. Epilepsia. (2020) 61:1234–9. doi: 10.1111/epi.16533
91. Lee S, Kim SH, Kim B, Lee ST, Choi JR, Kim HD, et al. enetic diagnosis and clinical characteristics by etiological classification in early-onset epileptic encephalopathy with burst suppression pattern. Epilepsy Res. (2020) 163:106323. doi: 10.1016/j.eplepsyres.2020.106323
92. Lee J, Lee C, Ki CS, Lee J. Determining the best candidates for next-generation sequencing-based gene panel for evaluation of early-onset epilepsy. Mol Genet Genomic Med. (2020) 8:1376. doi: 10.1002/mgg3.1376
93. Lin L, Zhang Y, Pan H, Wang J, Qi Y, Ma Y. Clinical and genetic characteristics and prenatal diagnosis of patients presented GDD/ID with rare monogenic causes. Orphanet J Rare Dis. (2020) 15:1–15. doi: 10.1186/s13023-020-01599-y
94. Mitta N, Menon RN, McTague A, Radhakrishnan A, Sundaram S, Cherian A, et al. Genotype-phenotype correlates of infantile-onset developmental and epileptic encephalopathy syndromes in South India: a single centre experience. Epilepsy Res. (2020) 166:106398. doi: 10.1016/j.eplepsyres.2020.106398
95. Murillo E. Characteristics of people with the STXBP1 syndrome in Spain: Implications for diagnosis. An Pediatr. (2020) 92:71–8. doi: 10.1016/j.anpedi.2019.04.008
96. Banne E, Falik-Zaccai T, Brielle E, Kalfon L, Ladany H, Klinger D, et al. De novo STXBP1 mutation in a child with developmental delay and spasticity reveals a major structural alteration in the interface with syntaxin 1A. Am J Med Genet Part B Neuropsychiatr Genet. (2020) 183:412–22. doi: 10.1002/ajmg.b.32816
97. Ünalp A, Gazeteci Tekin H, Karaoglu P, Akişin Z. Benefits of ketogenic diet in a pediatric patient with Ehlers-Danlos syndrome and STXBP1-related epileptic encephalopathy. Int J Neurosci. (2020) 2020:1–3. doi: 10.1080/00207454.2020.1858825
98. Loussouarn A, Doummar D, Beaugendre Y, Bienvenu T, Charles P, Depienne C, et al. Tremor-like subcortical myoclonus in STXBP1 encephalopathy. Eur J Paediatr Neurol. (2021) 34:62–6. doi: 10.1016/j.ejpn.2021.06.005
99. Arisaka A, Nakashima M, Kumada S, Inoue K, Nishida H, Mashimo H, et al. Association of early-onset epileptic encephalopathy with involuntary movements—case series and literature review. Epilepsy Behav Reports. (2021) 15:1–3. doi: 10.1016/j.ebr.2020.100417
100. Sharkov A, Dulac O, Gataullina S. STXBP1 germline mutation and focal cortical dysplasia. Epileptic Disord. (2021) 23:143–7. doi: 10.1684/epd.2021.1245
101. Suo G, Cao X, Zheng Y, Li H, Zhang Q, Tang J, et al. A de novo nonsense mutation of STXBP1 causes early-onset epileptic encephalopathy. Epilepsy Behav. (2021) 123:108245. doi: 10.1016/j.yebeh.2021.108245
102. Zaganas I, Vorgia P, Spilioti M, Mathioudakis L, Raissaki M, Ilia S, et al. Genetic cause of epilepsy in a Greek cohort of children and young adults with heterogeneous epilepsy syndromes. Epilepsy Behav Rep. (2021) 16:100477. doi: 10.1016/j.ebr.2021.100477
103. Zhang Y, Wang R, Liu Z, Jiang S, Du L, Qiu K, et al. Distinct genetic patterns of shared and unique genes across four neurodevelopmental disorders. Am J Med Genet Part B Neuropsychiatr Genet. (2021) 186:3–15. doi: 10.1002/ajmg.b.32821
104. Augustin I, Rosenmund C, Südhof TC, Brose N. Munc13-1 is essential for fusion competence of glutamatergic synaptic vesicles. Nature. (1999) 400:457–61. doi: 10.1038/22768
105. Wang S, Li Y, Gong J, Ye S, Yang X, Zhang R, et al. Munc18 and Munc13 serve as a functional template to orchestrate neuronal SNARE complex assembly. Nat Commun. (2019) 10:69. doi: 10.1038/s41467-018-08028-6
106. Varoqueaux F, Sigler A, Rhee JS, Brose N, Enk C, Reim K, et al. Total arrest of spontaneous and evoked synaptic transmission but normal synaptogenesis in the absence of Munc13-mediated vesicle priming. Proc Natl Acad Sci U S A. (2002) 99:9037–42. doi: 10.1073/pnas.122623799
107. Gracheva EO, Maryon EB, Berthelot-Grosjean M, Richmond JE. Differential Regulation of Synaptic Vesicle Tethering and Docking by UNC-18 and TOM-1. Front Synaptic Neurosci. (2010) 2:141. doi: 10.3389/fnsyn.2010.00141
108. Engel AG, Selcen D, Shen X-M, Milone M, Harper CM. Loss of MUNC13-1 function causes microcephaly, cortical hyperexcitability, and fatal myasthenia. Neurol Genet. (2016) 2:e105. doi: 10.1212/NXG.0000000000000105
109. Lipstein N, Verhoeven-Duif NM, Michelassi FE, Calloway N, van Hasselt PM, Pienkowska K, et al. Synaptic UNC13A protein variant causes increased neurotransmission and dyskinetic movement disorder. J Clin Invest. (2017) 127:1005–018. doi: 10.1172/JCI90259
110. Stemmerik MG, Borch JS, Dunø M, Krag T, Vissing J. Myopathy can be a key phenotype of membrin (GOSR2) deficiency. Hum Mutat. (2021) 42:1101–6. doi: 10.1002/humu.24247
111. Tian X, Teng J, Chen J. New insights regarding SNARE proteins in autophagosome-lysosome fusion. Autophagy. (2021) 17:2680–8. doi: 10.1080/15548627.2020.1823124
112. Morelli E, Speranza EA, Pellegrino E, Beznoussenko GV, Carminati F, Garré M, et al. Activity of the SNARE protein SNAP29 at the endoplasmic reticulum and golgi apparatus. Front Cell Dev Biol. (2021) 9:637565. doi: 10.3389/fcell.2021.637565
113. Mah-Som AY, Skrypnyk C, Guerin A, Seroor Jadah RH, Vardhan VN, McKinstry RC, et al. New cohort of patients with CEDNIK syndrome expands the phenotypic and genotypic spectra. Neurol Genet. (2021) 7:e553. doi: 10.1212/NXG.0000000000000553
114. Geerts CJ, Plomp JJ, Koopmans B, Loos M, van der Pijl EM, van der Valk MA, et al. Tomosyn-2 is required for normal motor performance in mice and sustains neurotransmission at motor endplates. Brain Struct Funct. (2015) 220:1971–82. doi: 10.1007/s00429-014-0766-0
115. Kumar R, Corbett MA, Smith NJ, Jolly LA, Tan C, Keating DJ, et al. Homozygous mutation of STXBP5L explains an autosomal recessive infantile-onset neurodegenerative disorder. Hum Mol Genet. (2015) 24:2000–10. doi: 10.1093/hmg/ddu614
116. Karaca E, Harel T, Pehlivan D, Jhangiani SN, Gambin T, Coban Akdemir Z, et al. Genes that affect brain structure and function identified by rare variant analyses of mendelian neurologic disease. Neuron. (2015) 88:386. doi: 10.1016/j.neuron.2015.09.048
Keywords: SNARE (soluble N-ethylmaleimide-sensitive fusion protein attachment protein receptor), epilepsy, seizures, mutations, vesicle fusion, epileptic encephalopathies
Citation: Cali E, Rocca C, Salpietro V and Houlden H (2022) Epileptic Phenotypes Associated With SNAREs and Related Synaptic Vesicle Exocytosis Machinery. Front. Neurol. 12:806506. doi: 10.3389/fneur.2021.806506
Received: 31 October 2021; Accepted: 16 November 2021;
Published: 13 January 2022.
Edited by:
Francesco Nicita, Bambino Gesù Children Hospital (IRCCS), ItalyReviewed by:
Bruria Ben-Zeev, Sheba Medical Center, IsraelCopyright © 2022 Cali, Rocca, Salpietro and Houlden. This is an open-access article distributed under the terms of the Creative Commons Attribution License (CC BY). The use, distribution or reproduction in other forums is permitted, provided the original author(s) and the copyright owner(s) are credited and that the original publication in this journal is cited, in accordance with accepted academic practice. No use, distribution or reproduction is permitted which does not comply with these terms.
*Correspondence: Vincenzo Salpietro, di5zYWxwaWV0cm9AdWNsLmFjLnVr
†These authors have contributed equally to this work
Disclaimer: All claims expressed in this article are solely those of the authors and do not necessarily represent those of their affiliated organizations, or those of the publisher, the editors and the reviewers. Any product that may be evaluated in this article or claim that may be made by its manufacturer is not guaranteed or endorsed by the publisher.
Research integrity at Frontiers
Learn more about the work of our research integrity team to safeguard the quality of each article we publish.