- 1Department of Psychology, Catholic University of the Sacred Heart, Milan, Italy
- 2Neurological Clinic, Department of Clinical and Experimental Medicine, University of Pisa, Pisa, Italy
- 3Department of Clinical and Experimental Medicine, University of Pisa, Pisa, Italy
- 4Azienda Unità Sanitaria Locale (USL) Toscana Nord Ovest, Pisa, Italy
- 5Psychology Research Laboratory, Istituto Auxologico Italiano IRCCS, Milan, Italy
Alzheimer's disease (AD) and Parkinson's disease (PD) are neurodegenerative disorders characterized by cognitive impairment and functional decline increasing with disease progression. Within non-pharmacological interventions, transcranial direct current stimulation (tDCS) might represent a cost-effective rehabilitation strategy to implement cognitive abilities with positive implications for functional autonomy and quality-of-life of patients. Our systematic review aimed at evaluating the effects of tDCS upon cognition in people suffering from AD and PD. We searched for randomized controlled trials (RCTs) into PubMed, Web of Science, and Cochrane Library. Three review authors extracted data of interest, with neuropsychological tests or experimental cognitive tasks scores as outcome measures. A total of 17 RCTs (10 trials for AD and 7 trials for PD) were included. Compared with sham stimulation, tDCS may improve global cognition and recognition memory in patients with AD and also some executive functions (i.e., divided attention, verbal fluency, and reduction of sensitivity to interference) in patients with PD. Criticism remains about benefits for the other investigated cognitive domains. Despite preliminary emerging evidences, larger RCTs with common neuropsychological measures and long-term follow-ups establishing longevity of the observed effects are necessary for future research in applied psychology field, alongside improved clinical guidelines on the neurodegenerative disorders pertaining electrodes montage, sessions number, duration and intensity of the stimulation, and cognitive battery to be used.
Introduction
Application of Transcranial Direct Current Stimulation (tDCS) in Cognitive Rehabilitation
The tDCS is a neurostimulation method, painless, substantially devoid of the significant side effects, economic, simple to apply and even suitable for a home environment administration under supervision of remote therapist, also in case of the neurological disorders (1–4). In such a technique, a weak current—usually 1/2 mA at constant frequency—is applied to the scalp through one or two stimulation electrodes in targeted brain regions, as single or bilateral configuration modes (5). The current leads to changes in the extracellular milieu that, in turn, affects the resting membrane potential of the neuronal populations in the proximity of electrodes placement (6). However, although stimulation is applied over limited brain areas, the distribution of the current that reaches the cortex depends on intensity, modulation duration, electrodes montage and size, and orientation of the electric field in relation to anatomical features of the cortex (7). While the anodal tDCS increases cortical excitability in the brain region under and around the electrode placement, the cathode tDCS decreases it (8). Short-term effects of the tDCS occur through non-synaptic mechanisms by depolarization of resting membrane potential, while long-term effects likely occur through NMDA-dependent mechanisms and appear to be consistent with synaptic plasticity (9, 10).
Despite potential associated adverse events (e.g., tingling, itching, burning sensation, mild headache, bright flashes of light and skin burn, etc.) (6), tDCS is globally considered as a safe, tolerable and low-cost rehabilitation strategy. Contraindications only pertain to metallic implants in the head/body, craniotomy or history of seizure (11, 12). As a result, tDCS has been applied with promising results to many neurological disorders (13–16) and neuropsychiatric conditions (17–20), resulting in an exponential growth of studies in the last decades.
Cognitive Deficits in Alzheimer's Disease and Parkinson's Disease
Recently, the development of novel non-invasive methods of brain stimulation, such as transcranial magnetic stimulation (TMS) and tDCS has increased the interest in neuromodulatory approaches as potential tools to counteract a progressively more severe cognitive deterioration related to the course of neurodegenerative disorders, such as AD and PD.
On one side, AD is a progressive neurodegenerative disorder and accounts for most of dementia in elderly people, currently affecting 5.8 million people in the US alone (21). This percentage is dramatically estimated to increase, by reaching 65.7 million people affected by AD in 2030 worldwide (22). The number of new cases of AD significantly increases with aging, with an incidence of 76 of every 1,000 people of 85 years and older (21). AD has a devastating effect on patients and their caregivers and determines a tremendous socioeconomic impact on the health system. Usually, cognitive deficits are present in patients prior to the time of AD onset (i.e., mild cognitive impairment due to AD) (23), and typically affect episodic memory and executive functions domains (24). Usually, memory impairment is the earliest representing the core symptom of the disease and functional autonomy of patients decreases with progression of AD, also as a consequence of a wider range of supplementary cognitive deficits (25). Cholinesterase inhibitors are considered as the main pharmacologic treatment for patients with AD although response is quite limited (26–28).
On the other side, PD represents another chronic neurodegenerative disorder leading to a progressive decrement of functional autonomy of the patients. It affects about 1% of people who are aged older than 60 years and reported standardized incidence rates of PD are 8–18 per 100.000 person-years (29). Because of the dopamine reduction in the pars compacta of the substantia nigra, typical motor symptoms are characterized by resting tremor, rigidity, bradykinesia, and postural instability. Patients with PD show additional motor deficits including gait disturbance and motor complications, such as dyskinesia in the course of the disease (30). Despite its nosographic definition remarking motor deficits, PD has been progressively conceived as a “complex brain disease” including non-motor symptoms, such as cognitive disturbances (31, 32). In PD, there is a spectrum of cognitive dysfunction, ranging from mild cognitive impairment (PD-MCI) to PD dementia (PDD). Cognitive impairment is quite common in PD, affecting approximately 30–40% of the patients (33). Cognitive deficits might be present at early stages of the disease and are usually characterized by executive functions and visuospatial deficits (34–36). Neurocognitive deterioration pertaining to the frontal domains and attention system is a consequence of dopamine reduction (i.e., frontal-striatal syndrome). Structural abnormalities of fronto-parietal areas and subcortical regions (37) and temporo-parietal regions (38) implicated in visual stimuli analysis have been observed in patients with PD, too. A particular impairment of implicit motor sequence learning (IMSL) is also displayed by patients with PD, consisting of difficulties in acquisition of multiple single movements to be performed in a sequential order without conscious awareness needed for retrieval (39).
Alternative non-invasive neurostimulatory techniques such as tDCS require urgent development in the next future, both for AD and PD. However, performed investigations on tDCS effects upon cognition in patients with AD and PD to date present some limitations. They did not focus only on randomized controlled trials (RCTs) (22, 40, 41), mixed results from TMS and tDCS (42, 43), encompassed adjunctive cognitive or physical training (22, 40, 44, 45) to tDCS or adopted inclusion criteria for selecting studies encompassing vascular dementia or other neurological disorders, as well as patients with mild cognitive impairment (46).
Our systematic review tried to bypass the aforementioned limitations and represents an update systematic review of RCTs evaluating the effects of tDCS upon cognition in AD and PD as a stand-alone technique (i.e., without combined cognitive or physical training) compared with sham (i.e., placebo) stimulation.
Methods
Search Strategy
This update systematic review adheres to the Preferred Reporting Items for Systematic Reviews and Meta-analyses (PRISMA) Statement (47). PubMed, Web of Science, and Cochrane Library databases were systematically screened for RCTs using the following terms: “Alzheimer's Disease” or “Parkinson's disease” and “transcranial direct current stimulation” and “cognition” or “cognitive abilities” or “cognitive deficits” or “cognitive impairment” (only upper time limit: September 31, 2021). Additional titles were added based on the bibliographies of the relevant issues and through the use of hand search of journals and other pertinent resources. Figure 1 shows the PRISMA flowchart.
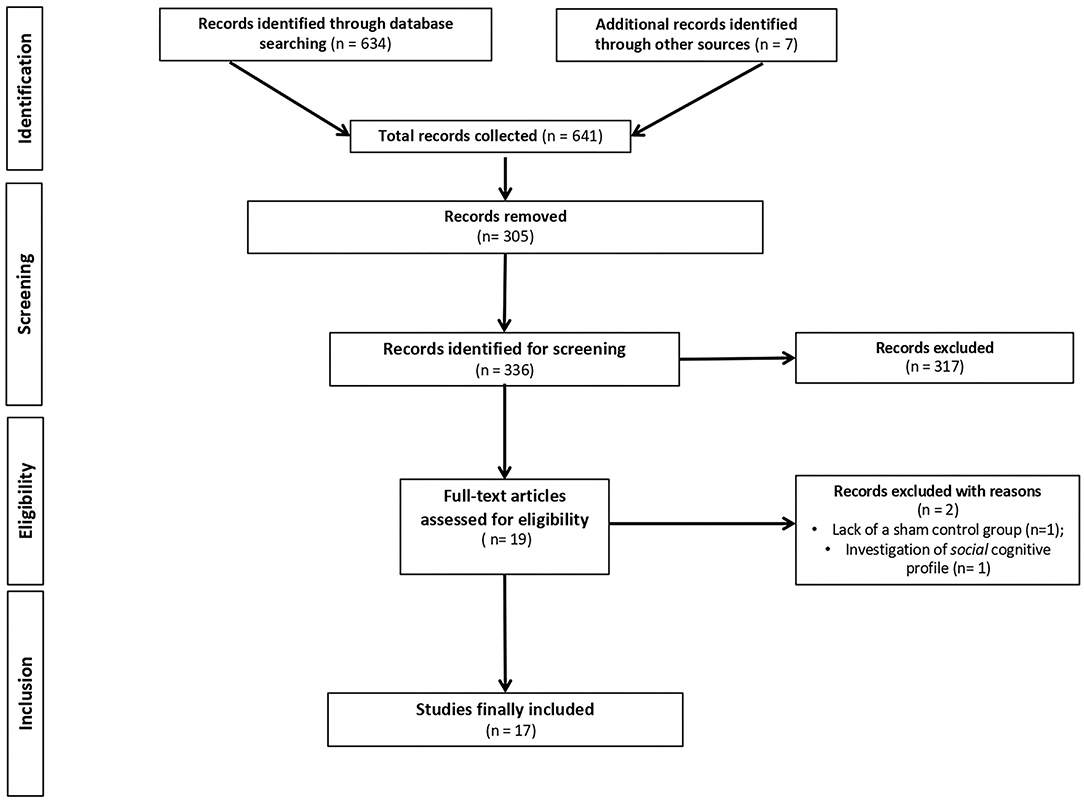
Figure 1. PRISMA (Preferred reporting of systematic reviews and meta-analysis) flowchart of search results.
Study Selection Criteria
Studies from the literature search were selected if they met the following criteria: (1) assessing the effects of tDCS on cognitive functioning; (2) including patients with AD or PD selected by recognized international diagnostic criteria, i.e., NINCDS–ADRDA criteria for AD (48) and UK Brain Bank criteria for PD (49), respectively; (3) RCT as study design; (4) measures of cognition as primary or secondary outcomes; (5) presence of sham (i.e., placebo) stimulation. Exclusion criteria encompassed: (1) multicomponent interventions (e.g., tDCS plus physical or cognitive training) or high-definition tDCS techniques; (2) other noninvasive brain stimulation techniques (e.g., TMS); (3) studies recruiting individuals with neurological disorders different from AD and PD (i.e., other dementia types or vascular dementia, mild cognitive impairment, stroke, multiple sclerosis, traumatic brain injury, focal brain disorders, etc.) or classified as having mild/major neurocognitive disorder and also psychiatric diseases and other relevant medical conditions that might interfere with cognitive functioning; (4) studies recruiting healthy older adults; (5) animal studies; and (6) manuscripts written in other languages than English.
Quality of the Studies and Assessment of Risk of Bias Evaluation
Three independent reviewers (DMC, FC, and RC) first evaluated methodological criteria used by RCTs examining tDCS effects upon cognition in AD (Table 1) and PD (Table 2) patients and then assessed the risk of bias according to the Quality Assessment Tool for Quantitative Studies (63) developed by the Effective Public Health Practice Project (EPHPP) (Tables 3, 4 for AD and PD, respectively). In both the cases, disagreement was discussed until a consensus among reviewers was definitely reached.
Results
Studies Selection, Evaluation, and Report
Initially, 634 records were identified through databases and manual search (Figure 1). After removing duplicates (n = 305), we screened the titles and the abstracts of the remaining records and identified 19 articles for a full-text inspection. Two studies (64, 65) were excluded because of different reasons (see Figure 1). Finally, 17 articles were included in our systematic review, 10 pertaining AD (12, 20, 50–57) and 7 pertaining PD (10, 39, 58–62). The evaluation of methodological criteria used was first shown in Tables 1, 2. The assessment of risk of bias (Tables 3, 4) reported that 15 studies were of moderate quality whereas only 2 studies were of strong quality (12, 53).
Outcomes: tDCS Effects on Cognitive Domains
A summary of the included studies was reported in Tables 5, 6 for patients with AD and for patients with PD, respectively. A total of 9 study designs were parallel ones (12, 20, 53–58, 61) whereas 8 study designs were crossover ones (10, 39, 50–52, 59, 60, 62). More specifically, the washout period of the latter studies performing different tDCS stimulations presented in counterbalanced order across participants, substantially varied from 48 h (10, 51) to 71.1 ± 5.8 days (52). A total of 5 studies (52–54, 58, 59) performed a follow-up, varying from 1 week (53, 54, 59) to 2 months (53), with 2 investigations reporting a prolonged tDCS effect upon cognition, particularly on the visual recognition memory (52) and divided attention (58). Furthermore, Gangemi et al. (56) adopted the longest intervention of stimulation (i.e., 10 days a month for 8 months).
Remarkably, participants of AD recruited from the studies were different in terms of global cognition at baseline. A range of Mini Mental State Examination (MMSE) scores was present among studies, with that of (54) (i.e., 15.0 ± 3.1 for the tDCS group and 15.2 ± 2.6 for the sham group) reporting the lowest ones. Such a discrepancy was not revealed for the PD selected studies.
In patients with AD, recognition memory—both verbal and visual one—improved at different current intensities, stimulation duration, and number of sessions (i.e., from 1.5 to 2 mA, from 15 to 30 min, from 3 to 5 sessions, respectively) by tDCS of the temporal cortex (50–52) while a clear-cut effect on the global cognition was obtained after a 2 mA stimulation for 25 of 30 min of the left DLPFC (12), both on the anodal and cathodal modality (53) or anodal stimulation of the frontotemporal cortex (56, 57). Visuoconstructive ability (55) and language abilities (i.e., naming) (12) seemed to ameliorate after daily sessions (2 mA for 20/30 min) of tDCS, too.
In patients with PD, executive efficiency was enhanced either thanks to the stimulation of DLPFC as a single brain area through variable sessions of treatment (i.e., 1–10 sessions) at 1–2 mA of 20-min current stimulation (10, 58, 61) or thanks to the combined stimulation of DLPFC and primary motor cortex (PMC) at 1.5 mA after a 3-session intervention of 30 min (60). Finally, beneficial effects of anodal tDCS over the primary motor cortex were found in relation to IMSL in such patients (39) after 1 week from the intervention (i.e., 2 mA intensity for 20 min per session during the cognitive task). In four cases, no cognitive improvement was revealed after tDCS intervention (20, 54, 59, 62).
Transcranial DCS was well tolerated by the patients even if some side effects were sometimes reported (i.e., tingling, sleepiness, mild headache, neck pain, skin redness, scalp pain, scalp burning, somnolence, and trouble concentrating) [e.g., (53, 54, 58)].
Discussion
This systematic review aimed to provide a comprehensive overview of the current knowledge about the effects of tDCS stimulation upon cognition for patients with AD and PD when compared with sham (placebo) stimulation. Transcranial DC stimulation seems to ameliorate cognitive vitality of patients in relation to global cognition and recognition memory in AD and divided attention, verbal fluency, and reduction of sensitivity to interference in PD, respectively. From a neuropsychological point of view, criticism remains about potential usefulness of tDCS for working memory, processing speed and visual attention, visuospatial abilities and verbal learning performances while initial proof arises about language improvement after tDCS.
Heterogeneity of the patients (i.e., age, disease onset, severity and duration, premorbid level of functioning), tDCS delivery settings (i.e., clinics, hospitals, and home treatment), concomitant pharmacological therapy and concurrent psychopathological symptoms, particularly depression and apathy not routinely evaluated except for some investigations represent confounding variables that make difficult to compare among studies [cf. (66)].
In four cases (20, 54, 59, 62), we reported the lack of results about cognition improvement after tDCS. In our opinion, it should be because of the use of the neuropsychological assessment/experimental tasks adopted for the evaluation of cognitive functions associated to the stimulated brain areas and/or small sample size.
Some researchers (12, 53, 54) stimulated the DLPFC in patients with AD, as a brain area critically associated with working memory and to a repertory of the frontal abilities, including planning, abstract reasoning, mental flexibility, and attentional set shifting. A recent investigation using [11C]-raclopride positron emission tomography demonstrated that tDCS of the DLPFC enhance attention system and executive functioning because of an increased release of dopamine neurotransmitter (67) in healthy males, probably allowing a more accurate performances on cognitive tests requiring an additional recruitment of attentional resources and executive control.
Other researchers (10, 58, 60) investigated the activation of the same brain area in patients with PD with encouraging findings, given that it has been related to executive deficits because of the dopaminergic dysfunction of the fronto-striatal network and to top–down attentional deficits due to alterations of the cholinergic fronto-parietal circuits, commonly reported in these patients (68). Remarkably, the enhancement of locomotor skills of patients with PD may benefit from executive efficiency (69) too. It has also been suggested that a possible beneficial effect of tDCS specific stimulation for patients with PD could be the induction of dopamine release in the caudate nucleus via the glutamatergic corticostriatal pathway, as shown in animal studies (70). Transcranial DC stimulation might also have a neuroprotective role in PD, by reducing the oxidative damage of dopamine neurons and by modulating functional connectivity of the corticostriatal and thalamocortical circuits of the human brain (40).
The selected studies of Boggio et al. (51, 52) and Bystad et al. (20) stimulated the medial temporal cortex in patients with AD, as a brain area critically associated with different memory performances [cf. (71, 72)]. Alterations of the medial temporal lobe which might influence visual recognition memory are well recognized in patients with AD (73, 74). Patients with AD also report a selective hypoactivation of the temporoparietal cortex (TPC) that is normally involved in word recognition memory tasks (75). Transcranial tDCS of the TPC seems to enhance such a memory process (50), too.
Despite the difficulty of drawing definitive conclusions, results from the RCTs globally show a tendency toward positive effects of tDCS for patients with AD and PD, however, it is less clear which stimulation procedure leads to the best results. Evidence-based guidelines developed by Lefaucheur et al. (76) on the therapeutic use of tDCS reported no recommendation for the efficacy of specific tDCS parameters (i.e., electrodes placement, number and timing of sessions and duration, intensity and time of stimulation), by concluding that the optimization of tDCS protocols should be better addressed in the next future to offer a more pronounced therapeutic effect also in case of patients with AD and PD. Recently, given that tDCS has showing promising clinical results, a team of experts in conducting systematic reviews of the clinical trials have concluded that such rehabilitation technique is probably effective in PD, both for motor and cognitive aspects (77).
Some studies have shown that connections between different areas of the AD brain are impaired at specific time points, and that stimulation of other brain areas not primarily associated with commonly impaired cognitive functioning could yield promising results. A fundamental aspect of AD pathophysiology is based on the dysfunction of long-range cortical networks (78). As an illustration, not only the hippocampus and the associative cerebral cortices are involved in memory processes but also the posterior parietal cortex (PPC) exerts a key role for attentional resources in supporting memory processes (79), i.e., notoriously damaged in AD. Recently, a notable investigation has shown that mechanisms of cerebellar-cortical plasticity are impaired in AD (80) too. Given its role in the higher cognitive functions, new potential therapeutic strategies should be also built up in the next future to modulate neural activity in the cerebellum.
Focus on the neurophysiological aspects of other neurostimulation techniques, such as TMS/transcranial alternating current stimulation (tACS) and EEG activity, may offer supplementary information able to deeply investigate brain circuitry modulation. For example, mechanisms of cortical plasticity have been investigated in patients with AD by TMS protocols, such as theta brust stimulation (TBS) showing a clear impairment of long-term potentiation (LTP) cortical-like plasticity and a relative sparing of long-term depression (LTD) mechanisms in AD (78). There is also evidence that spike-timing-dependent plasticity (STDP) is compromised in AD, as revealed by studies adopting paired associates stimulation (PAS) protocols (81). Similarly, some interesting studies using cerebellar continuous TBS have reported promising results that may help identifying specific neurophysiological phenotypes as that shown by a group of patients clinically diagnosed as PD with normal dopaminergic functional imaging defined as SWEED (Scans Without Evidence of Dopaminergic Deficit). Patients with SWEED present with a mild impairment in cerebello-thalamo-cortical circuit and this neurophysiological phenotype differs from the that observed in PD and dystonic patients, suggesting a distinct involvement of this pathway in the pathophysiology of disorders (82).
These observations could lead researcher to implement neurostimulation techniques exploring different sites of stimulation or to even consider multisites techniques that could give more insight on the correct parameters to be used. A recent area of interest is represented by an implementation of tDCS technique, namely, high-definition tDCS (i.e., HD-tDCS), a novel approach that uses smaller electrodes whose configuration can be optimized for targeting specific brain regions (83). Such a technique offers some advantages then conventional method, as follows: (i) it can stimulate more precisely a target cortical region; (ii) aftereffects last at least 30 min longer than those obtained with conventional tDCS; (iii) it potentially reduces the likelihood of side effects; (iv) it determines less discomfort and improves applicability in the elderly (84).
Given the relatively minor neurodegenerative changes, tDCS appears to be more promising in early phases of the disease, i.e., MCI due to AD and in the Parkinson's disease mild cognitive impairment (PD-MCI), as confirmed by preliminary investigations also when it is delivered alongside cognitive or physical training (85–88). Accordingly, it has already been documented how tDCS stimulation is less effective in patients with AD in the advanced stages of the disease (43, 89). Moreover, tDCS can modulate brain activity in a manner similar to TMS with the advantages of being easily applied and substantially safe. Our review confirms that tDCS is well-tolerated by the patients with slight side effects not frequently reported (i.e., tingling, sleepiness, mild headache, neck pain, skin redness, scalp pain, scalp burning, somnolence, and trouble concentrating). Transcranial DC stimulation is also reliably blinded by placebo (i.e., sham stimulation) in the clinical settings.
Despite emerging evidences, the larger RCTs are welcome in the next future for replicating preliminary results on patients with AD and PD and for measuring the effects on different outcomes beside cognition (i.e., psychopathological dimensions such as depression and/or apathy, health-related quality-of-life, personal and instrumental autonomy and also motor functioning for patients with PD) allowing researchers to depict a more comprehensive analysis of tDCS potential.
Cortical plasticity and connectivity result to be impaired in the neurodegenerative conditions and neurophysiological findings could provide more robust evidence about the implementation of tDCS protocols for these diseases. The optimization of tDCS protocols should also start from early response of the patient to the treatment. Future tDCS studies would also take advantage of computational models to ensure a calibration of the stimulation technique on specificity of the patient (90). Finally, tDCS should be widely delivered to patients with neurological disorders as an at-home rehabilitation strategy under supervision of therapists, in order to improve personalized medicine purposes [cf. (91–93)].
Data Availability Statement
The original contributions presented in the study are included in the article/supplementary material, further inquiries can be directed to the corresponding author.
Author Contributions
DMC made the most substantial contribution in data conception, knowledge synthesis, acquisition, analysis, interpretation of data, and as well as manuscript writing. FC and RC were involved in checking for quality of the studies and risk of bias evaluation. UB and GC revised the paper for intellectual content. All the authors approved the final version of the manuscript.
Conflict of Interest
The authors declare that the research was conducted in the absence of any commercial or financial relationships that could be construed as a potential conflict of interest.
Publisher's Note
All claims expressed in this article are solely those of the authors and do not necessarily represent those of their affiliated organizations, or those of the publisher, the editors and the reviewers. Any product that may be evaluated in this article, or claim that may be made by its manufacturer, is not guaranteed or endorsed by the publisher.
References
1. Dobbs B, Pawlak N, Biagioni M, Agarwal S, Shaw M, Pilloni G, et al. Generalizing remotely supervised transcranial direct current stimulation (tDCS): feasibility and benefit in Parkinson's disease. J Neuroeng Rehabil. (2018) 7:114. doi: 10.1186/s12984-018-0457-9
2. Agarwal S, Pawlak N, Cucca A, Sharma K, Dobbs B, Shawm M, et al. Remotely-supervised transcranial direct current stimulation paired with cognitive training in Parkinson's disease: an open-label study. J ClinNeurosci. (2018) 57:51–7. doi: 10.1016/j.jocn.2018.08.037
3. Charvet L, Shaw M, Dobbs B, Frontario A, Sherman K, Bikson M. Remotely supervised transcranial direct current stimulation increases the benefit of at-home cognitive training in multiple sclerosis. Neuromodulation. (2018) 21:383–9. doi: 10.1111/ner.12583
4. Pilloni G, Shaw M, Feinberg C, Clayton A, Palmeri M, Datta A, et al. Long term at-home treatment with transcranial direct current stimulation (tDCS) improves symptoms of cerebellar ataxia: a case report. J Neuroeng Rehabil. (2019) 16:41. doi: 10.1186/s12984-019-0514-z
5. Cerasa A, Gioia MC, Salsone M, Donzuso G, Chiriaco C, Realmuto S. Neurofunctional correlates of attention rehabilitation in Parkinson's disease: an explorative study. J Neurol Sci. (2014) 35:1173–80. doi: 10.1007/s10072-014-1666-z
6. Gonsalvez I, Baror R, Fried P, Santarnecch E, Pascual-Leone A. Therapeutic noninvasive brain stimulation in Alzheimer's disease. Curr Alzheimer Res. (2017) 14:362–76. doi: 10.2174/1567205013666160930113907
7. Mahdavi S, Towhidkhah F, Anzheimer's Disease Neuroimaging Initiative. Computational human head models of tDCS: influence of brain atrophy on current density distribution. Brain Stimul. (2018) 11:104–7. doi: 10.1016/j.brs.2017.09.013
8. Cholerton BA, Zabetian CP, Wan JY, Montine TJ, Quinn JF, Mata IF, et al. Evaluation of cognitive impairment subtypes in Parkinson's disease. Mov Disord. (2014) 29:756–64. doi: 10.1002/mds.25875
9. Nitsche MA, Paulus W. Excitability changes induced in the human motor cortex by weak transcranial direct current stimulation. J Physiol. (2000) 527:633–9. doi: 10.1111/j.1469-7793.2000.t01-1-00633.x
10. Boggio PS, Ferrucci R, Rigonatti SP, Covre P, Nitsche M, Pascual-Leone A. Effects of transcranial direct current stimulation on working memory in patients with Parkinson's disease. J Neurol Sci. (2006) 249:31–8. doi: 10.1016/j.jns.2006.05.062
11. Zhao H, Qiao L, Fan D, Zhang S, Turel O, Li Y, et al. Modulation of brain activity with noninvasive transcranial direct current stimulation (tDCS): clinical applications and safety concerns. Front Psychol. (2017) 10:685. doi: 10.3389/fpsyg.2017.00685
12. Im JJ, Jeong H, Bikson M. Effects of a 6-month at-home transcranial direct current stimulation on cognition and cerebral glucose metabolism in Alzheimer's disease. Brain Stimul. (2019) 12:1222–8. doi: 10.1016/j.brs.2019.06.003
13. Roncero C, Kniefel H, Service E, Thiel A, Probst S, Chertkow H. Inferior parietal transcranial direct current stimulation with training improves cognition in anomic Alzheimer's disease and frontotemporal dementia. Alzheimers Dement. (2017) 3:247–53. doi: 10.1016/j.trci.2017.03.003
14. Gramegna LL, Evangelisti S, Testa C, Baiardi S, Mitolo M, Capellari S, et al. Cognitive rehabilitation and transcranial direct current stimulation in a patient with posterior cortical atrophy: an fMRI study. Am J Case Rep. (2018) 21:729–33. doi: 10.12659/AJCR.909167
15. Gschwind M, Seeck M. Transcranial direct-current stimulation as treatment in epilepsy. Expert Rev Neurother. (2016) 16:1427–41. doi: 10.1080/14737175.2016.1209410
16. Elsner B, Kwakkel G, Kugler J, Mehrholz J. Transcranial direct current stimulation (tDCS) for improving capacity in activities and arm function after stroke: a network meta-analysis of randomised controlled trials. J Neuroeng Rehabil. (2017) 14:95. doi: 10.1186/s12984-017-0301-7
17. Demitras-Tatlidede A, Vahabzadeh-Hagh AM, Pascual-Leone A. Can non-invasive brain stimulation enhance cognition in neuropsychiatric disorders? Neuropharmacol. (2013) 64:566–78. doi: 10.1016/j.neuropharm.2012.06.020
18. Elder GJ, Taylor JP. Transcranial magnetic stimulation and transcranial direct current stimulation: treatments for cognitive and neuropsychiatric symptoms in the neurodegenerative dementias? Alzheimers Res Ther. (2014) 6:74. doi: 10.1186/s13195-014-0074-1
19. Elder GJ, Firbank MJ, Kumar H, Chatterjee P, Chakraborty T, Dutt A, et al. Effects of transcranial direct current stimulation upon attention and visuoperceptual function in Lewy body dementia: a preliminary study. Int Psychogeriatr. (2016) 28:341–7. doi: 10.1017/S1041610215001180
20. Bystad M, Grønli O, Rasmussen ID, Gundersen N, Nordvang L, Wang-Iversen H, et al. Transcranial direct current stimulation as a memory enhancer in patients with Alzheimer's disease: a randomized, placebo-controlled trial. Alzheimers Res Ther. (2016) 8:13. doi: 10.1186/s13195-016-0180-3
21. Alzheimer's Association. Alzheimer's disease facts and figures. Alzheimers Dement. (2019) 15:321–87. doi: 10.1016/j.jalz.2019.01.010
22. Cappon D, Jahanshahi M, Bisiacchi P. Value and efficacy of transcranial direct current stimulation in cognitive rehabilitation: a critical review since 2000. Front Neurosci. (2016) 18:157. doi: 10.3389/fnins.2016.00157
23. Albert MS, DeKosky ST, Dickson D, Dubois B, Feldman HH, Fox NC, et al. The diagnosis of mild cognitive impairment due to Alzheimer's disease: recommendations from the national institute on aging-alzheimer's association workgroups on diagnostic guidelines for Alzheimer's disease. Alzheimers Dement. (2011) 7:270–9. doi: 10.1016/j.jalz.2011.03.008
24. Baudic S, Barba GD, Thibaudet MC, Smagghe A, Remy P, Traykov L. Executive function deficits in early Alzheimer's disease and their relations with episodic memory. Arch Clin Neuropsychol. (2006) 21:15–21. doi: 10.1016/j.acn.2005.07.002
25. Quaglino V, Gounden Y, Lacot E. Talk the talk and walk the walk. evaluation of autonomy in aging and Alzheimer disease by simulating instrumental activities of daily living: the S-IADL. PeerJ4 (2016) 2016:e2351. doi: 10.7717/peerj.2351
26. Cammisuli DM, Danti S, Bosinelli F, Cipriani G. Non-pharmacological interventions for people with Alzheimer's disease: a critical review of the scientific literature from the last ten years. Eur Geriatr Med. (2016) 7:57–64. doi: 10.1016/j.eurger.2016.01.002
27. Renn BN, Asghar-Ali AA, Thielke S, Catic A, Martini SR, Mitchell BG. A systematic review of practice guidelines and recommendations for discontinuation of cholinesterase inhibitors in dementia. Am J Geriatr Psychiatry. (2018) 26:134–47. doi: 10.1016/j.jagp.2017.09.027
28. Han JY, Besser LM, Xiong C. Cholinesterase inhibitors may not benefit mild cognitive impairment and mild Alzheimer disease dementia. Alzheimer Dis Assoc Disord. (2019) 33:87. doi: 10.1097/WAD.0000000000000291
29. De Lau LML, Breteler MMB. Epidemiology of Parkinson's disease. Lancet Neurol. (2006) 5:525–35. doi: 10.1016/S1474-4422(06)70471-9
30. Moustafa AA, Chakravarthy S, Phillips JR. Motor symptoms in Parkinson's disease: a unified framework. Neurosci Biobehav Rev. (2016) 68:727–40. doi: 10.1016/j.neubiorev.2016.07.010
31. Emre M, Aarsland D, Brown R, Burn DJ, Duyckaerts C, Mizuno Y, et al. Clinical diagnostic criteria for dementia associated with Parkinson's disease. Mov Disord. (2007) 22:1689–707. doi: 10.1002/mds.21507
32. Ceravolo R, Rossi C, Kleferle L, Bonuccelli U. Nonmotor symptoms in Parkinson's disease: the dark side of the moon. Future Neurol. (2010) 5:851–71. doi: 10.2217/fnl.10.69
33. Wojatala J, Heber IA, Neuser P, Heller J, Kalbe E, Rehberg SP, et al. Cognitive decline in Parkinson's disease: the impact of then motor phenotype on cognition. J Neurol Neurosurg Psychiatry. (2019) 90:171–9. doi: 10.1136/jnnp-2018-319008
34. Poletti M, Frosini D, Ceravolo R, Bonuccelli U. Mild cognitive impairment in De Novo Parkinson's disease according to movement disorder guidelines. Mov Disord. (2012) 27:1706. doi: 10.1002/mds.25120
35. Hoogland J, van Wanrooij LL, Boel JA, Goldman JG, Stebbins GT, Dalrymple-Alford JC, et al. Detecting mild cognitive deficits in Parkinson's disease: comparison of neuropsychological tests. Mov Disord. (2018) 33:1750–9. doi: 10.1002/mds.110
36. Cammisuli DM, Crowe S. Spatial disorientation and executive dysfunction in elderly nondemented patients with Parkinson's disease. Neuropsychiatr Dis Treat. (2018) 14:2531. doi: 10.2147/NDT.S173820
37. Caproni S, Muti M, Di Rienzo A, Principi M, Caputo N, Calabresi P, et al. Subclinical visuospatial impairment in Parkinson's disease: the role of basal ganglia and limbic system. Front Neurol. (2014) 5:152. doi: 10.3389/fneur.2014.00152
38. Garcia-Diaz AI, Segura B, Baggio HC, Marti MJ, Valldeoriola F, Compta Y, et al. Structural brain correlations of visuospatial and visuoperceptual tests in Parkinson's disease. JINS. (2018) 24:22–44. doi: 10.1017/S1355617717000583
39. Firouzi M, Van Herk K, Kerckhofs E, Swinnen E, Baeken C, Van Overwalle F, et al. Transcranial direct-current stimulation enhances implicit motor sequence learning in persons with Parkinson's disease with mild cognitive impairment. J Neuropsychol. (2021) 15:363–78. doi: 10.1111/jnp.12231
40. Broeder S, Nackaerts E, Heremans E, Vervoort G, Meesen R, Verheyden A, et al. Transcranial direct current stimulation in Parkinson's disease: neurophysiological mechanisms and behavioural effects. Neurosci Biobehav Rev. (2015) 57:105–17. doi: 10.1016/j.neubiorev.2015.08.010
41. Lawrence BJ, Gasson N, Bucks RS, Troeung L, Loftus AM. Cognitive training and non-invasive brain stimulation for cognition in Parkinson's disease: a meta-analysis. Neurorehab Neural Repair. (2017) 31:597–608. doi: 10.1177/1545968317712468
42. Hsu W-Y, Ku Y, Zanto TP, Gazzaley A. Effects of noninvasive brain stimulation on cognitive function in healthy aging and Alzheimer's disease: a systematic review and meta-analysis. Neurobiol Aging. (2015) 36:2348–59. doi: 10.1016/j.neurobiolaging.2015.04.016
43. Freitas C, Madragón-Llorca H, Pascual-Leone A. Noninvasive brain stimulation in Alzheimer's disease: systematic review and perspective for the future. Exp Gerontol. (2011) 46:611–27. doi: 10.1016/j.exger.2011.04.001
44. Mayo-Yáñez M, Corrás T, Méndez-Iglesias R. Transcranial direct current stimulation for treatment of Alzheimer's disease: a systematic review of randomized clinical trials. Cur Psych Rev. (2018) 14:211–4. doi: 10.2174/1573400514666181010153514
45. Beretta VS, Conceição NR, Nóbrega-Sousa P, Orcioli-Silva D, Fonseca Dantas LKB, Bucken Gobbi LT, et al. Transcranial direct current stimulation combined with physical or cognitive training in people with Parkinson's disease: a systematic review. J Neuroeng Rehabilitation. (2020) 17:74. doi: 10.1186/s12984-020-00701-6
46. Inagawa T, Narita Z, Sugawara N, Maruo K, Stickley A, Yokoi Y, et al. A Meta-analysis of the effect of multisession transcranial direct current stimulation on cognition in dementia and mild cognitive impairment. Clin EEG Neurosci. (2019) 50:273–82. doi: 10.1177/1550059418800889
47. Moher D, Liberati A, Tetzlaff J, Altman DGT. group preferred reporting items for systematic reviews and meta-analyses: the PRISMA statement. PLoS Med. (2009) 6:e1000097. doi: 10.1371/journal.pmed.1000097
48. McKhann G, Drachman D, Folstein M, Katzman R, Price D, Stadlan EM. Clinical diagnosis of Alzheimer's disease: report of the NINCDS-ADRDA work group under the auspices of department of health and human services task force on Alzheimer's disease. Neurology. (1984) 34:939–44. doi: 10.1212/WNL.34.7.939
49. Hughes AJ, Daniel SE, Kilford L, Leeas AJ. Accuracy of clinical diagnosis of idiopathic Parkinson's disease: a clinico-pathological study of 100 cases.J Neurol Neurosurg Psychiatry. (1992) 55:181–4. doi: 10.1136/jnnp.55.3.181
50. Ferrucci R, Mameli F, Guidi I, Mrakic-Sposta S, Vergari M, Marceglia SEEA, et al. Transcranial direct current stimulation improves recognition memory in Alzheimer disease. Neurology. (2008) 71:493–8. doi: 10.1212/01.wnl.0000317060.43722.a3
51. Boggio PS, Khoury LP, Martins DCS, Martins OE, Macedo EC, Fregni F, et al. Temporal cortex direct current stimulation enhances performance on a visual recognition memory task in Alzheimer disease. J Neurol Neurosurg Psychiatry. (2009) 80:444–7. doi: 10.1136/jnnp.2007.141853
52. Boggio PS, Ferrucci R, Mameli F, Martins D, Martins O, Vergari M, et al. Prolonged visual memory enhancement after direct current stimulation in Alzheimer's disease. Brain Stimul. (2012) 5:223–30. doi: 10.1016/j.brs.2011.06.006
53. Khedr EM, Garnal NL, El-Fetoh NA, Khalifa H, Ahmed EM, Ali AM, et al. A double-blind randomized clinical trial on the efficacy of cortical direct current stimulation for the treatment of Alzheimer's disease. Front Aging Neurosci. (2014) 9:275. doi: 10.3389/fnagi.2014.00275
54. Suemoto CK, Apolinario D, Nakamura-Palacios EM, Lopes L, Leite REP, Sales MC. Effects of a non-focal plasticity protocol on apathy in moderate Alzheimer's disease: a randomized, double-blind, sham-controlled trial. Brain Stimul. (2014) 7:308–13. doi: 10.1016/j.brs.2013.10.003
55. Khedr EM, Salama RH, Hameed MA, Elfetoh NA, Seif P. Therapeutic role of transcranial direct current stimulation in Alzheimer disease patients: double-blind, placebo-controlled clinical trial. Neurorehabil Neural Repair. (2019) 33:384–94. doi: 10.1177/1545968319840285
56. Gangemi A, Colombo B, Fabio RA. Effects of short-and long-term neurostimulation (tDCS) on Alzheimer's disease patients: two randomized studies. Aging Clin Exp Res. (2021) 33:383–90. doi: 10.1007/s40520-020-01546-8
57. Gangemi A, Fabio RA. Transcranial direct current stimulation for Alzheimer's disease. Asian J Gerontol Geriatr. (2020) 15:5–9. doi: 10.12809/ajgg-2019-344-oa
58. Doruk D, Gray Z, Bravo GL, Pascual-Leone A, Fregni F. Effects of tDCS on executive function in Parkinson's disease. Neurosci Lett. (2014) 582:27–31. doi: 10.1016/j.neulet.2014.08.043
59. Ferrucci R, Cortese F, Bianchi M, Pittera D, Turrone R, Bocci T, et al. Cerebellar and motor cortical transcranial stimulation decrease levodopa-induced dyskinesias in Parkinson's disease. Cerebellum. (2016) 15:43–7. doi: 10.1007/s12311-015-0737-x
60. Dagan M, Herman T, Harrison R, Zhou J, Giladi N, Ruffini G. Multitarget transcranial direct current stimulation for freezing of gait in Parkinson's disease. Mov Disord. (2018) 33:642–6. doi: 10.1002/mds.27300
61. Bueno MA, Netoa LI, doN Terraa MB, Barbozaa NM, Okanob AH, Smailia SM, et al. Effectiveness of acute transcranial direct current stimulation on non-motor and motor symptoms in Parkinson's disease. Neurosci Lett. (2019) 696:46–51. doi: 10.1016/j.neulet.2018.12.017
62. Lau C-I, Liu M-N, Chang K–C, Chang A, Bai C–H, Tseng C-S. Effect of single-session transcranial direct current stimulation on cognition in Parkinson's disease. CNS Neurosci Ther. (2019) 25:1237–43. doi: 10.1111/cns.13210
63. Thomas BH, Ciliska D, Dobbins M, Micucci S. A process for systematically reviewing the literature: providing the research evidence for public health nursing interventions. Worldviews Evid Based Nurs. (2004) 1:176–84. doi: 10.1111/j.1524-475X.2004.04006.x
64. Pereira JB, Junqué C, Bartrés-Faz D, Martí MJ, Sala-Llonch R, Compta Y, et al. Modulation of verbal fluency networks by transcranial direct current stimulation (tDCS) in Parkinson's disease. Brain Stimul. (2013) 6:16–24. doi: 10.1016/j.brs.2012.01.006
65. Adenzato M, Manenti R, Enrici I, Gobbi E, Brambilla M, Alberici A, et al. Transcranial direct current stimulation enhances theory of mind in Parkinson's disease patients with mild cognitive impairment: a randomized, double-blind, sham-controlled study. Transl Neurodegener. (2019) 8:1–13. doi: 10.1186/s40035-018-0141-9
66. Poletti M, Bonuccelli U. Acute and chronic cognitive effects of levodopa and dopamine agonists on patients with Parkinson's disease. Ther Adv Psychopharmacol. (2013) 3:101–13. doi: 10.1177/2045125312470130
67. Fukai M, Bunai T, Hirosawa T, Kikuchi M, Ito S, Minabe Y, et al. Endogenous dopamine release under transcranial direct-current stimulation governs enhanced attention: a study with positron emission tomography. Transl Psychiatry. (2019) 9:115. doi: 10.1038/s41398-019-0443-4
68. Gratwicke J, Jahanshahi M, Foltynie T. Parkinson's disease dementia: a neural networks perspective. Brain. (2015). 138:1454–76. doi: 10.1093/brain/awv104
69. Manenti R, Brambilla M, Rosini S, Orizio I, Ferrari C, Borroni B, et al. Time up and go task performance improves after transcranial direct current stimulation in patients affected by Parkinson's disease. Neurosci Lett. (2014) 19:74–7. doi: 10.1016/j.neulet.2014.07.052
70. Tanaka T, Takano S, Tanaka S, Hironaka N, Kobayashi K, Hanakawa T, et al. Transcranial direct current stimulation increases extracellular dopamine levels in the rat striatum. Front Syst Neurosci. (2013) 7:6. doi: 10.3389/fnsys.2013.00006
71. Fregni F, Boggio PS, Nitsche M, Bermpohl F, Antal A, Feredoes E, et al. Anodal transcranial direct current stimulation of prefrontal cortex enhances working memory. Exp Brain Res. (2005) 166:23–30. doi: 10.1007/s00221-005-2334-6
72. Blumenfeld RS, Ranganath C. Prefrontal cortex and long-term memory encoding: an integrative review of findings from neuropsychology and neuroimaging. Neuroscientist. (2007) 13:280–91. doi: 10.1177/1073858407299290
73. Dickerson BC, Sperling RA. Functional abnormalities of the medial temporal lobe memory system in mild cognitive impairment and Alzheimer's disease: insights from functional MRI studies. Neuropsychologia. (2008) 46:1624–35. doi: 10.1016/j.neuropsychologia.2007.11.030
74. Pasquini L, Farzaneh R, Somayeh M-B, La Joie R, Zarei M, Sorg C. Medial temporal lobe disconnection and hyperexcitability across Alzheimer's disease stage. JADR. (2019) 3:103–12. doi: 10.3233/ADR-190121
75. Kim JJ, Andersen NC, O'Leary DS, Wiser AK, Ponto LB, Watkins GL, et al. Direct comparison of the neural substrate of recognition memory for words and face. Brain. (1999) 122:1069–83. doi: 10.1093/brain/122.6.1069
76. Lefaucheur JP, Antal A, Ayache SS, Benninger DH, Brunelin J, Cogiamanian F, et al. Evidence-based guidelines on the therapeutic use of transcranial direct current stimulation (tDCS). Clin Neurophysiol. (2017) 128:58–92. doi: 10.1016/j.clinph.2016.10.087
77. Fregni F, El-Hagrassy MM, Pacheco-Barrios K, Carvalho S, Leite J, Simis M, et al. Evidence-based guidelines and secondary meta-analysis for the use of transcranial direct current stimulation in neurological and psychiatric disorders. Int J Neuropsychopharmacol. (2021) 24:256–313. doi: 10.1093/ijnp/pyaa051
78. Di Lorenzo F, Ponzo V, Motta C, Bonnì S, Picazio S, Caltagirone C, et al. Impaired spike timing dependent cortico-cortical plasticity in Alzheimer's disease patients. J Alzheimers Dis. (2018) 66:983–91. doi: 10.3233/JAD-180503
79. Cabeza R, Ciaramelli E, Olson IR, Moscovitch M. The parietal cortex and episodic memory: an attentional account. Nat Rev Neurosci. (2008) 9:613–25. doi: 10.1038/nrn2459
80. Di Lorenzo F, Bonnì S, Picazio S, Motta C, Caltagirone C, Martorana A, et al. of cerebellar theta burst stimulation on contralateral motor cortex excitability in patients with Alzheimer's disease. Brain Topogr. (2020) 33:613–7. doi: 10.1007/s10548-020-00781-6
81. Terranova C, SantAngelo A, Morgante F, Rizzo V, Allegra R, Arena MG, et al. Impairment of sensory-motor plasticity in mild Alzheimer's disease. Brain Stimul. (2013) 6:62–6. doi: 10.1016/j.brs.2012.01.010
82. Schirinzi T, Lorenzo DF, Ponzo V, Palmieri MG, Bentivoglio AR, Schillaci O, et al. Mild cerebello-thalamo-cortical impairment in patients with normal dopaminergic scans (SWEDD). Parkinsonism Relat Disord. (2016) 28:23–8. doi: 10.1016/j.parkreldis.2016.03.023
83. Park CH, Chang WH, Park JY, Shin YI, Kim ST, Kim YH. Transcranial direct current stimulation increases resting state interhemispheric connectivity. Neurosci Lett. (2013) 539:7–10. doi: 10.1016/j.neulet.2013.01.047
84. He F, Li Y, Li C, Fan L, Liu T, Wang J. Repeated anodal high-definition transcranial direct current stimulation over the left dorsolateral prefrontal cortex in mild cognitive impairment patients increased regional homogeneity in multiple brain regions. PLoS ONE. (2021) 16:e0256100. doi: 10.1371/journal.pone.0256100
85. Meinzer M, Lindenberg R, Phan MT. Transcranial direct current stimulation in mild cognitive impairment: Behavioral effects and neural mechanisms. Alzheimers Dement. (2015) 11:1032–40. doi: 10.1016/j.jalz.2014.07.159
86. Yun K, Song JU, Chung YA. Changes in cerebral glucose metabolism after 3 weeks of noninvasive electrical stimulation of mild cognitive impairment patients. Alzheimers Res Ther. (2016) 8:49. doi: 10.1186/s13195-016-0218-6
87. Biundo R, Weis L, Fiorenzato E, Gentile G, Giglio M, Schifano R, et al. Double-blind randomized trial of t-DCS versus sham in Parkinson patients with mild cognitive impairment receiving cognitive training. Brain Stimul. (2015) 8:1223–5. doi: 10.1016/j.brs.2015.07.043
88. Lawrence BJ, Gasson N, Johnson AR, Booth L, Loftus AM. Cognitive training and transcranial direct current stimulation for mild cognitive impairment in Parkinson's disease: a randomized controlled trial. Parkinsons Dis. (2018) 2018:4318475. doi: 10.1155/2018/4318475
89. Nardone R, Bergmann J, Christova M, Caleri F, Tezzon F, Ladurner G, et al. Effect of transcranial brain stimulation for the treatment of Alzheimer disease: a review. Int J Alzheimers Dis. (2012) 2012:687909. doi: 10.1155/2012/687909
90. Rasmussen ID, Boayuea MR, Mittnera M, Bystada M, Grønlib OK, Vangbergc TR, et al. High-definition transcranial direct current stimulation improves delayed memory in Alzheimer's disease patients: a pilot study using computational modeling to optimize electrode position. J Alzheimers Dis. (2021) 83:753–69. doi: 10.3233/JAD-210378
91. Gulley E, Verghese J, Blumen HM, Ayers E, Wang C, Portenoy RK, et al. Neurostimulation for cognitive enhancement in Alzheimer's disease (the NICE-AD study): a randomized clinical trial. Neurodegenr Dis Manag. (2021) 11:277–88. doi: 10.2217/nmt-2020-0061
92. Qiu Y, Jacobs DM, Messer K, Salmon DP, Feldman HH. Cognitive heterogeneity in probable Alzheimer disease: clinical and neuropathologic features. Neurology. (2019) 93:e778–90. doi: 10.1212/WNL.0000000000007967
93. Park J, Oh Y, Chung K, Kim KJ, Kim CO, Park JY. Effect of home-based transcranial direct current stimulation (tDCS) on cognitive function in patients with mild cognitive impairment: a study protocol for a randomized, double-blind, cross-over study. Trails. (2019) 20:278. doi: 10.1186/s13063-019-3360-1
Keywords: transcranial direct current stimulation, cognition, rehabilitation, randomized controlled trials, Alzheimer's disease, Parkinson's disease
Citation: Cammisuli DM, Cignoni F, Ceravolo R, Bonuccelli U and Castelnuovo G (2022) Transcranial Direct Current Stimulation (tDCS) as a Useful Rehabilitation Strategy to Improve Cognition in Patients With Alzheimer's Disease and Parkinson's Disease: An Updated Systematic Review of Randomized Controlled Trials. Front. Neurol. 12:798191. doi: 10.3389/fneur.2021.798191
Received: 19 October 2021; Accepted: 30 December 2021;
Published: 02 February 2022.
Edited by:
Giovanni Morone, Santa Lucia Foundation (IRCCS), ItalyReviewed by:
Francesco Di Lorenzo, Santa Lucia Foundation (IRCCS), ItalyAlessio Baricich, Università degli Studi del Piemonte Orientale, Italy
Copyright © 2022 Cammisuli, Cignoni, Ceravolo, Bonuccelli and Castelnuovo. This is an open-access article distributed under the terms of the Creative Commons Attribution License (CC BY). The use, distribution or reproduction in other forums is permitted, provided the original author(s) and the copyright owner(s) are credited and that the original publication in this journal is cited, in accordance with accepted academic practice. No use, distribution or reproduction is permitted which does not comply with these terms.
*Correspondence: Gianluca Castelnuovo, Z2lhbmx1Y2EuY2FzdGVsbnVvdm8mI3gwMDA0MDt1bmljYXR0Lml0; Z2lhbmx1Y2EuY2FzdGVsbnVvdm8mI3gwMDA0MDthdXhvbG9naWNvLml0