- Fundación para la Lucha contra las Enfermedades Neurológicas de la Infancia (FLENI), Buenos Aires, Argentina
Andropause results from the natural decrease in testosterone levels that occurs with age. In contrast to menopause, which is a universal, well-characterized process associated with absolute gonadal failure, andropause ensues after gradual decline of both hypothalamic-pituitary-gonadal axis activity, as well as of testicular function, a process which usually develops over a period of many years. Increasing evidence on greater risk of Multiple sclerosis (MS) associated with lower testosterone levels is being reported. Likewise, epidemiological studies have shown a later age of onset of MS in men, relative to women, which could perhaps respond to the decline in protective testosterone levels. In this review, we will discuss the role of androgens in the development and function of the innate and adaptive immune response, as well as in neuroprotective mechanisms relevant to MS. Testosterone effects observed in different animal models and in epidemiological studies in humans will be discussed, as well as their correlation with physical disability and cognitive function levels. Finally, published and ongoing clinical trials exploring the role of androgens, particularly at key stages of sexual maturation, will be reviewed.
Introduction
Multiple sclerosis (MS) is a demyelinating immune-mediated disease of the central nervous system (CNS) universally more prevalent in women than in men, a phenomenon shared with several other autoimmune diseases. Indeed, different studies have reported greater MS prevalence in females, with the current female/male ratio estimated to be 3:1 (1). Important gender differences in inflammatory activity and progression of disease have also been observed. Male patients not only develop disease later, they experience less relapses. However, they also accumulate disability faster, reach milestones more rapidly, and show poorer recovery after initial exacerbations, compared to females (2). These findings have led to extensive studies on differences between the immune and nervous systems of men and women, in response not only to their specific gonadal hormones, but also to genetic factors, exposure to environmental factors and varying lifestyles (3). It has been hypothesized that the natural age-related decline in testosterone, the main male sex hormone, may play a role in these gender-related differences in MS prevalence and in clinical characteristics.
Several studies have highlighted the modulatory role of female sex hormones on MS disease activity during different stages of reproduction such as puberty, pregnancy and menopause. However, less is known about the impact of reproductive senescence in men. In contrast to menopause, which is a universal, well-characterized process associated with absolute gonadal failure, andropause is characterized by a gradual decline in hypothalamic-pituitary-gonadal axis activity, as well as decreased testicular function, occurring over a period of many years. Concentrations of bioavailable testosterone can decrease by as much as 50% between the ages of 25 and 75 years (4). Given that in men there is no abrupt hormonal cutoff, or clear period of symptomatic change, some authors argue that the term “andropause” or “male menopause” is inappropriate, and that the phenomenon should be called late-onset hypogonadism (LOH) or age-related androgen decline (5). Dehydroepiandrosterone (DHEA), an adrenal precursor to more potent androgens and estrogens, as well as its metabolite DHEA sulfate (DHEA-S) also decline with age, at a rate of 3% per year, 3 times faster than testosterone, falling to one-third of serum concentrations by age 70 (6).
In this review we will discuss the role of androgens in the development and function of the innate and adaptive immune response, as well as neuroprotective mechanisms relevant to MS. Testosterone effects observed in different animal models and epidemiological studies in humans will be analyzed, as well as their correlation with physical disability and cognitive function levels. Finally, we will review published and ongoing clinical trials exploring the role of androgens, particularly at different stages of sexual maturation.
Effects of Androgens on Immune Function
Andropause appears to contribute, at least in part, to immunosenescence, and consequently to progression of disability in MS. Immunosenescence is an age-associated decline in function of both the adaptive and innate immune systems. MS patients may experience premature onset of this phenomenon (7). In parallel, specific effects of androgens include a shift from Th1 to Th2 phenotype, based on increased production of IL-5 and IL-10, and decreased pro-inflammatory cytokines including IFN-γ, TNFα, IL-1, IL-6, and IL-17. Testosterone also reduces lymphocyte proliferation and differentiation and may suppress immunoglobulin production (8). Supra-physiological doses of testosterone also inhibit cytotoxic NK cell activity (9, 10). Therefore, androgens should, in principle, be considered anti-inflammatory hormones. In the CNS, dihydrotestosterone inhibits LPS-induced release of proinflammatory factors, including TNF-α, IL-1β, IL-6; iNOS, COX-2, NO, and PGE2 in BV2 cells and primary microglia cells, through suppression of TLR4-mediated NF-κB and MAPK p38 signaling pathways, thus protecting neurons from inflammatory damage induced by activated microglia (11). Similar changes have been observed in experimental in vivo models. In fact, castration of male animals has had detrimental effect on susceptibility to, and severity of EAE (12). MBP-specific T lymphocytes derived from the spleen of male animals during the effector phase of adoptive EAE, produced significantly higher levels of IL-10 (13), and treatment with testosterone was protective, an effect linked to androgen-mediated Th2 bias, as suggested by the IFNγ/IL-10 ratio (12, 13).
Similar to testosterone, DHEA inhibits transcription factor NF-κB, and suppresses secretion of IL-1β, TNF-α, and IFN-γ (14). In animal models, DHEA decreases T cell response and shows anti-inflammatory effect on microglia and astrocytes, ameliorating EAE severity and inflammation (15, 16).
In the thymus, the autoimmune regulator (Aire) gene prevents autoimmunity by promoting self-antigen expression in medullary thymic epithelial cells, such that developing T cells that recognize these self-antigens within the thymus, undergo clonal deletion. Androgens recruit androgen receptors to Aire promoter regions, enhancing Aire transcription. Thus, androgen levels in males may increase Aire expression to a degree that protects against autoimmunity. In line with this, in EAE mice, androgen administration as well as male gender, protected against autoimmunity in an Aire-dependent manner, indicating that control of intrathymic Aire-mediated tolerance mechanisms by androgens, contributes to explain gender-related differences observed in MS (17).
Neuroprotective Mechanisms
Testosterone crosses the blood-brain-barrier, directly influencing neuronal cells (18, 19). The main effects of testosterone on the CNS are summarized in Table 1. Similarly, in humans, DHEA has demonstrated neuroprotective effects, increasing neurite growth, promoting neurogenesis and neuronal survival, influencing apoptosis, and catecholamine synthesis and secretion, as well as exerting antioxidant effects (6).
Recent studies have shown that testosterone may also play an important role in myelination processes. Indeed, after lysolecithin-induced demyelination, testosterone favors astrocyte recruitment and spontaneous oligodendrocyte-mediated remyelination (35). Similarly, castration of male animals results in decrease in myelination in the corpus callosum, both under normal conditions and after long term administration of cuprizone. These processes are reversed following exogenous testosterone administration (36).
In line with these findings, other studies have shown that testosterone, via specific involvement of androgen receptors, induces proliferation and differentiation of oligodendrocyte precursors (OPCs), as well as activation and proliferation of astrocytes and microglial cells (36).
Clinical Findings
Andropause or LOH refers to the gradual and lifelong decline in serum testosterone concentration and testicular function that occurs with aging. Healthy older men will experience approximately 40% reduction in total Leydig cell mass over time. LOH will also likely be influenced by comorbidities associated with aging and the development of chronic illnesses, including obesity, diabetes, cardiovascular disease, and inflammatory disorders, all associated with accelerated aging-related testosterone decline. Presentation of MS symptoms in older patients will be further impacted by these events (37, 38).
Clinical characteristics of andropause include diminished sexual desire and erectile capacity, decreased intellectual activity, fatigue, depression, loss of muscle mass and body hair, anemia, decrease in bone mineral density resulting in osteoporosis, and increased visceral fat and obesity (39). These symptoms may overlap with the effects of aging, worsening the motor disability, fatigue, cognitive decline and psychiatric symptoms caused by MS (19).
Both MS symptoms as well as their severity also appear to differ between males and females. Men present later onset of disease (40, 41) and experience less frequent relapses with poorer recovery (42, 43). They show faster progression (Malik Neurology, 2014), worse outcomes (42) more cerebellar involvement, as well as greater cognitive impairment (42, 44). In line with these clinical findings, MRIs from men with MS show less inflammation (40), more gray matter atrophy (44) and more T1 lesions (45). Intriguingly, these clinical findings are not observed in pediatric MS cases prior to puberty (46), or in women with MS onset during menopause (around 50 years of age on average in Western societies), suggesting a more complex interplay between hormonal mechanisms related to reproductive senescence or aging, and the course of the disease (47). Young MS patients show slower progression of disability than adults or late onset MS patients, but experience relapses more frequently (48, 49). Risk of relapse seems to decrease continuously with time until patients reach the age of 35. In contrast, disability worsening remains stable from childhood to about 32 years of age, and then increases sharply after the age of 45 (50). Typical age of MS onset in men is around 40, coinciding with the physiological age-related decline in androgen levels, suggesting loss of testosterone could contribute to development of MS. Overlap with other phenomena related to aging, such as immunosenescence could also influence patient symptom profiles. Effects of androgen levels and aging in men with MS are summarized in Figure 1.
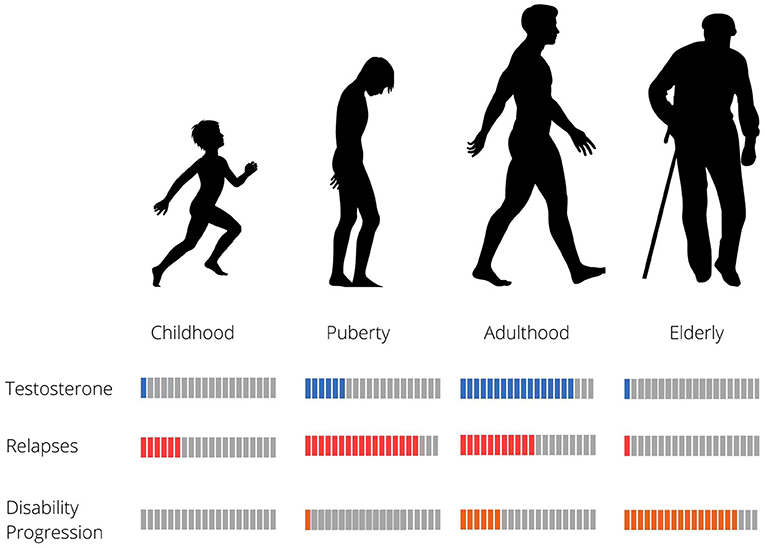
Figure 1. Relapse rate, disability progression and testosterone levels in relation to age in MS males.
Several studies have found lower testosterone levels in men with MS, compared to healthy age-matched subjects (51–53). In a cohort of 96 men with relapsing remitting MS, mean age of 40 years and disease duration <10 years, 39% of patients were hypogonadal with no compensatory rise in luteinizing hormone, suggesting central hypogonadism (54). Interestingly, the authors reported a correlation between low testosterone levels and disability (54). Other studies however, were not able replicate these findings (53).
An analysis of linked national Hospital Episode Statistics from England reported a strong positive association (5-fold elevation) between testicular hypofunction, as a proxy for low testosterone levels, and subsequent risk of MS in males (55).
Recently, increased risk of MS was reported in transgender individuals receiving estrogens and anti-androgens (56), suggesting influence of feminizing hormones, or low testosterone levels on risk of disease, providing further evidence of the importance of sex hormones in MS pathophysiology.
Potential Use of Testosterone for Ms Treatment
Testosterone replacement therapy is commonly indicated in aging and hypogonadal men. Hormonal supplementation induces virilization, improved libido and energy, increased muscle strength and fat-free mass, and strengthens bone density. Its use requires monitoring of prostate-specific antigen, as well as of hematocrit levels.
In a pilot clinical trial with cross-over design, 10 RRMS men aged <65 years, were treated with 100 mg of testosterone (6 months observation followed by 12 months of treatment). Treatment resulted in improvement of cognitive performance, and slowing of brain atrophy, with no significant effect on gadolinium-enhancing lesions (Gd) (57). Subsequent evaluations of this same study have shown testosterone treatment decreased CD4+T cells and IL-2 production, and increased NK cells as well as TGF-β1 secretion (8). Furthermore, voxel-based morphometry of the brain showed not only less global atrophy, but also significant increase in gray matter in a cluster in the right frontal cortex (58).
TOTEM RRMS (NCT 03910738) is a phase 2, multicenter, randomized placebo controlled, double-blind trial carried out in 40 testosterone-deficient men with relapsing-remitting MS, which aims to prevent MS progression. Patients will be randomized into two groups, to receive either testosterone undecanoate or placebo over a period of 66 weeks. All patients will be treated with natalizumab during the trial. The primary outcome is to measure the neuroprotective and remyelinating effects of testosterone using tensor diffusion imaging techniques and thalamic atrophy analyzes. Secondary outcomes include use of conventional MRI sequences as well as clinical parameters to assess cognition, fatigue, quality of life, impact on work activity and anxiety/depression. Recruitment is expected to end around September 2021 (59).
The main clinical trials using testosterone are summarized in Table 2. These trials underline the potential use of testosterone as an immunomodulatory, neuroprotective and remyelinating molecule. Importantly, testosterone did not cause significant side effects in any of these trials, suggesting this treatment could represent a safe adjunctive therapy for MS and other neurodegenerative diseases (19).
Conclusions and Future Perspectives
Age is one of the major determinants of the clinical course of MS. Aging mechanisms will likely affect multiple clinical aspects of the disease, as well as influence underlying pathological mechanisms, immunological changes, and treatment efficacy.
Notably, transition from RRMS to more progressive disease phases will overlap with the naturally occurring age-related decline of androgens in men, and with menopause in women. Clinical findings already suggest specific association between reproductive senescence and MS progression. We have described the influence of testosterone on MS and its potential effects on immunosenescence as well as on neuroprotection. However, further longitudinal studies on disease activity in diverse populations of men with MS are needed. Available information regarding DMTs in older men suffering from andropause and MS is limited, as clinical trials often restrict enrollment to those aged < 55 years. Understanding the complex interplay between reproductive senescence and the course of MS is of critical importance to elucidate mechanisms driving disease progression in older patients, and minimize progression of disability.
Author Contributions
All authors listed have made a substantial, direct and intellectual contribution to the work, and approved it for publication.
Conflict of Interest
The authors declare that the research was conducted in the absence of any commercial or financial relationships that could be construed as a potential conflict of interest.
Publisher's Note
All claims expressed in this article are solely those of the authors and do not necessarily represent those of their affiliated organizations, or those of the publisher, the editors and the reviewers. Any product that may be evaluated in this article, or claim that may be made by its manufacturer, is not guaranteed or endorsed by the publisher.
References
1. Koch-Henriksen N, Sørensen PS. The changing demographic pattern of multiple sclerosis epidemiology. Lancet Neurol. (2010) 9:520–32. doi: 10.1016/S1474-4422(10)70064-8
2. Ribbons KA, McElduff P, Boz C, Trojano M, Izquierdo G, Duquette P, et al. Male sex is independently associated with faster disability accumulation in relapse-onset MS but not in primary progressive MS. PLoS ONE. (2015) 10:e0122686. doi: 10.1371/journal.pone.0122686
3. Greer J, Mccombe P. Role of gender in multiple sclerosis: clinical effects and potential molecular mechanisms. J Neuroimmunol. (2011) 234:7–18. doi: 10.1016/j.jneuroim.2011.03.003
4. Gould DC, Jacobs HS, Petty R. The male menopause—does it exist? Foragainst. BMJ. (2000) 320:858–61. doi: 10.1136/bmj.320.7238.858
5. Saad F, Gooren L. Late onset hypogonadism of men is not equivalent to the menopause. Maturitas. (2014) 79. doi: 10.1016/j.maturitas.2014.06.016
6. Maninger N, Wolkowitz OM, Reus VI, Epel ES, Mellon SH. Neurobiological and neuropsychiatric effects of dehydroepiandrosterone (DHEA) and DHEA sulfate (DHEAS). Front Neuroendocrinol. (2009) 30:65. doi: 10.1016/j.yfrne.2008.11.002
7. Bolton C, Smith P. The influence and impact of ageing and immunosenescence (ISC) on adaptive immunity during multiple sclerosis (MS) and the animal counterpart experimental autoimmune encephalomyelitis (EAE). Ageing Res Rev. (2017) 41:64–81. doi: 10.1016/j.arr.2017.10.005
8. Gold SM, Chalifoux S, Giesser BS, Voskuhl RR. Immune modulation and increased neurotrophic factor production in multiple sclerosis patients treated with testosterone. J Neuroinflammation. (2008) 5:32. doi: 10.1186/1742-2094-5-32
9. Gold SM, Voskuhl RR. Estrogen and testosterone therapies in multiple sclerosis. Prog Brain Res. (2009) 175:239–51. doi: 10.1016/S0079-6123(09)17516-7
10. Chitnis T. The role of testosterone in MS risk and course. Mult Scler. (2018) 24:36–41. doi: 10.1177/1352458517737395
11. Yang L, Zhou R, Tong Y, Chen P, Shen Y, Miao S, et al. Neuroprotection by dihydrotestosterone in LPS-induced neuroinflammation. Neurobiol Dis. (2020) 140:104814. doi: 10.1016/j.nbd.2020.104814
12. Bebo BF, Schuster JC, Vandenbark AA, Offner H. Gender differences in experimental autoimmune encephalomyelitis develop during the induction of the immune response to encephalitogenic peptides. J Neurosci Res. (1998) 52:420–6. doi: 10.1002/(SICI)1097-4547(19980515)52:4andlt
13. Dalal M, Kim S, Voskuhl RR. Testosterone therapy ameliorates experimental autoimmune encephalomyelitis and induces a T helper 2 bias in the autoantigen-specific T lymphocyte response. J Immunol. (1997) 159, 3–6.
14. Prall S, Muehlenbein M. DHEA modulates immune function: a review of evidence. Vitam Horm. (2018) 108:125–44. doi: 10.1016/bs.vh.2018.01.023
15. Yilmaz C, Karali K, Fodelianaki G, Gravanis A, Chavakis T, Charalampopoulos I, et al. Neurosteroids as regulators of neuroinflammation. Front Neuroendocrinol. (2019) 55:100788. doi: 10.1016/j.yfrne.2019.100788
16. Boghozian R, Mckenzie B, Saito L, Mehta N, Branton W, Lu J-Q, et al. Suppressed oligodendrocyte steroidogenesis in multiple sclerosis: implications for regulation of neuroinflammation. Glia. (2017) 65:1590–606. doi: 10.1002/glia.23179
17. Zhu M-L, Bakhru P, Conley B, Nelson JS, Free M, Martin A, et al. Sex bias in CNS autoimmune disease mediated by androgen control of autoimmune regulator. Nat Commun. (2016) 7:11350. doi: 10.1038/ncomms11350
18. Collongues N, Patte-Mensah C, Seze J, Mensah-Nyagan A-G, Derfuss T. Testosterone and estrogen in multiple sclerosis: from pathophysiology to therapeutics. Expert Rev Neurother. (2018) 18:515–22. doi: 10.1080/14737175.2018.1481390
19. Bianchi V, Rizzi L, Bresciani E, Omeljaniuk R, Torsello A. Androgen therapy in neurodegenerative diseases. J Endocr Soc. (2020) 4:bvaa120. doi: 10.1210/jendso/bvaa120
20. Meydan S, Kus I, Tas U, Ogeturk M, Sancakdar E, Dabak D, et al. Effects of testosterone on orchiectomy-induced oxidative damage in the rat hippocampus. J Chem Neuroanat. (2010) 40:281–5. doi: 10.1016/j.jchemneu.2010.07.006
21. Son S, Lee J, Kim H-G, Kim D, Ahn Y-C, Son C. Testosterone depletion increases the susceptibility of brain tissue to oxidative damage in a restraint stress mouse model. J Neurochem. (2015) 136:106–17. doi: 10.1111/jnc.13371
22. Ramsden M, Shin T, Pike C. Androgens modulate neuronal vulnerability to kainate lesion. Neuroscience. (2003) 122:573–8. doi: 10.1016/j.neuroscience.2003.08.048
23. Marinis Ed, Fiocchetti M, Acconcia F, Ascenzi P, Marino M. Neuroglobin upregulation induced by 17β-estradiol sequesters cytocrome c in the mitochondria preventing H2O2-induced apoptosis of neuroblastoma cells. Cell Death Dis. (2013) 4:e508. doi: 10.1038/cddis.2013.30
24. Gürer B, Kertmen H, Kasim E, Yilmaz ER, Kanat BH, Sargon MF, et al. Neuroprotective effects of testosterone on ischemia/reperfusion injury of the rabbit spinal cord. Injury. (2015) 46:240–8. doi: 10.1016/j.injury.2014.11.002
25. Toro-Urrego N, Garcia-Segura L, Echeverria V, Barreto G. Testosterone protects mitochondrial function and regulates neuroglobin expression in astrocytic cells exposed to glucose deprivation. Front Aging Neurosci. (2016) 8:152. doi: 10.3389/fnagi.2016.00152
26. Lustig RH. Sex hormone modulation of neural development in vitro. Horm Behav. (1994) 28:383–95. doi: 10.1006/hbeh.1994.1035
27. Fanaei H, Karimian SM, Sadeghipour HR, Hassanzade G, Kasaeian A, Attari F, et al. Testosterone enhances functional recovery after stroke through promotion of antioxidant defenses, BDNF levels and neurogenesis in male rats. Brain Res. (2014) 1558:74–83. doi: 10.1016/j.brainres.2014.02.028
28. Spritzer MD, Roy EA. Testosterone and adult neurogenesis. Biomolecules. (2020) 10:225. doi: 10.3390/biom10020225
29. Matsumoto A. Hormonally induced neuronal plasticity in the adult motoneurons. Brain Res Bull. (1997) 44:539–47. doi: 10.1016/S0361-9230(97)00240-2
30. Leranth C, Petnehazy O, MacLusky NJ. Gonadal hormones affect spine synaptic density in the CA1 hippocampal subfield of male rats. J Neurosci. (2003) 23:1588–92. doi: 10.1523/JNEUROSCI.23-05-01588.2003
31. Beyer C, Hutchison J. Androgens stimulate the morphological maturation of embryonic hypothalamic aromatase-immunoreactive neurons in the mouse. Brain Res Dev Brain Res. (1997) 98:74–81. doi: 10.1016/S0165-3806(96)00170-8
32. Barreto G, Veiga S, Azcoitia I, Garcia-Segura LM, Garcia-Ovejero D. Testosterone decreases reactive astroglia and reactive microglia after brain injury in male rats: role of its metabolites, oestradiol and dihydrotestosterone. Eur J Neurosci. (2007) 25:3039–46. doi: 10.1111/j.1460-9568.2007.05563.x
33. Zárate S, Stevnsner T, Gredilla R. Role of estrogen and other sex hormones in brain aging. Neuroprotection and DNA repair. Front Aging Neurosci. (2017) 9:430. doi: 10.3389/fnagi.2017.00430
34. Ziehn MO, Avedisian AA, Dervin SM, Umeda EA, O'Dell TJ, Voskuhl RR. Therapeutic testosterone administration preserves excitatory synaptic transmission in the hippocampus during autoimmune demyelinating disease. J Neurosci. (2012) 32:12312–24. doi: 10.1523/JNEUROSCI.2796-12.2012
35. Bielecki B, Mattern C, Ghoumari AM, Javaid S, Smietanka K, Ghanem CA, et al. Unexpected central role of the androgen receptor in the spontaneous regeneration of myelin. Proc Natl Acad Sci USA. (2016) 113:14829–34. doi: 10.1073/pnas.1614826113
36. Hussain R, Ghoumari AM, Bielecki B, Steibel J, Boehm N, Liere P, et al. The neural androgen receptor: a therapeutic target for myelin repair in chronic demyelination. Brain. (2013) 136:132–46. doi: 10.1093/brain/aws284
37. Wu F, Tajar A, Pye S, Silman A, Finn J, O'Neill T, et al. Hypothalamic-pituitary-testicular axis disruptions in older men are differentially linked to age and modifiable risk factors: the european male aging study. J Clin Endocrinol Metab. (2008) 93:2737–45. doi: 10.1210/jc.2007-1972
38. Swee DS, Gan EH. Late-onset hypogonadism as primary testicular failure. Front Endocrinol. (2019) 10:372. doi: 10.3389/fendo.2019.00372
39. Jaschke N, Wang A, Hofbauer LC, Rauner M, Rachner TD. Late-onset hypogonadism: clinical evidence, biological aspects and evolutionary considerations. Ageing Res Rev. (2021) 67:1568–637. doi: 10.1016/j.arr.2021.101301
40. Pozzilli C, Tomassini V, Marinelli F, Paolillo A, Gasperini C, Bastianello S. “Gender gap” in multiple sclerosis: magnetic resonance imaging evidence. Eur J Neurol. (2003) 10:95–7. doi: 10.1046/j.1468-1331.2003.00519.x
41. Confavreux C, Vukusic S. Age at disability milestones in multiple sclerosis. Brain. (2006) 129:595–605. doi: 10.1093/brain/awh714
42. Kalincik T, Vivek V, Jokubaitis V, Lechner-Scott J, Trojano M, Izquierdo G, et al. Sex as a determinant of relapse incidence and progressive course of multiple sclerosis. Brain. (2013) 136:3609–17. doi: 10.1093/brain/awt281
43. Malik MT, Healy BC, Benson LA, Kivisakk P, Musallam A, Weiner HL, et al. Factors associated with recovery from acute optic neuritis in patients with multiple sclerosis. Neurology. (2014) 82:2173–9. doi: 10.1212/WNL.0000000000000524
44. Schoonheim MM, Popescu V, Rueda Lopes FC, Wiebenga OT, Vrenken H, Douw L, et al. Subcortical atrophy and cognition: Sex effects in multiple sclerosis. Neurology. (2012) 79:1754–61. doi: 10.1212/WNL.0b013e3182703f46
45. Antulov R, Weinstock-Guttman B, Cox JL, Hussein S, Durfee J, Caiola C, et al. Gender-related differences in MS: a study of conventional and nonconventional MRI measures. Mult Scler. (2009) 15:345–54. doi: 10.1177/1352458508099479
46. Chitnis T. Role of puberty in multiple sclerosis risk and course. Clin Immunol. (2013) 149:192–200. doi: 10.1016/j.clim.2013.03.014
47. Bove R. Autoimmune diseases and reproductive aging. Clin immunol. (2013) 149:251–64. doi: 10.1016/j.clim.2013.02.010
48. Renoux C, Vukusic S, Mikaeloff Y, Edan G, Clanet M, Dubois B, et al. Natural history of multiple sclerosis with childhood onset. N Engl J Med. (2007) 356:2603–13. doi: 10.1056/NEJMoa067597
49. Benson LA, Healy BC, Gorman MP, Baruch NF, Gholipour T, Musallam A, et al. Elevated relapse rates in pediatric compared to adult MS persist for at least 6 years. Mult Scler Relat Disord. (2014) 3:186–93. doi: 10.1016/j.msard.2013.06.004
50. Wyl V, Décard BF, Benkert P, Lorscheider J, Hänni P, Lienert C, et al. Influence of age at disease onset on future relapses and disability progression in patients with multiple sclerosis on immunomodulatory treatment. Eur J Neurol. (2020) 27:1066–75. doi: 10.1111/ene.14191
51. Wei T, Lightman SL. The neuroendocrine axis in patients with multiple sclerosis. Brain. (1997) 120:1067–76. doi: 10.1093/brain/120.6.1067
52. Safarinejad MR. Evaluation of Endocrine Profile, Hypothalamic–Pituitary–Testis Axis and Semen Quality in Multiple Sclerosis. J Neuroendocrinol. (2008) 20:1368–75. doi: 10.1111/j.1365-2826.2008.01791.x
53. D'Amico E, Zanghì A, Burgio G, Chisari CG, Condorelli RA, la Vignera S, et al. Gonadal steroids and sperm quality in a cohort of relapsing remitting multiple sclerosis: a case-control study. Front Neurol. (2020) 11:756. doi: 10.3389/fneur.2020.00756
54. Bove R, Chitnis T. The role of gender and sex hormones in determining the onset and outcome of multiple sclerosis. Mult Scler J. (2014) 20:520–6. doi: 10.1177/1352458513519181
55. Pakpoor J, Goldacre R, Schmierer K, Giovannoni G, Goldacre MJ. Testicular hypofunction and multiple sclerosis risk: a record-linkage study. Ann Neurol. (2014) 76:625–8. doi: 10.1002/ana.24250
56. Pakpoor J, Wotton CJ, Schmierer K, Giovannoni G, Goldacre MJ. Gender identity disorders and multiple sclerosis risk: a national record-linkage study. Mult Scler. (2016) 22:1759–62. doi: 10.1177/1352458515627205
57. Sicotte NL, Giesser BS, Tandon V, Klutch R, Steiner B, Drain AE, et al. Testosterone treatment in multiple sclerosis. Arch Neurol. (2007) 64:683. doi: 10.1001/archneur.64.5.683
58. Kurth F, Luders E, Sicotte NL, Gaser C, Giesser BS, Ronald S, et al. NeuroImage : clinical Neuroprotective effects of testosterone treatment in men with multiple sclerosis. Elsevier. (2014) 4:454–60. doi: 10.1016/j.nicl.2014.03.001
59. Metzger-Peter K, Kremer LD, Edan G, Loureiro De Sousa P, Lamy J, Bagnard D, et al. The TOTEM RRMS (Testosterone Treatment on neuroprotection and Myelin Repair in Relapsing Remitting Multiple Sclerosis) trial: study protocol for a randomized, double-blind, placebo-controlled trial. Trials. (2020) 21:591. doi: 10.1186/s13063-020-04517-6
Keywords: andropause, testosterone, aging, sex hormones, multiple sclerosis
Citation: Ysrraelit MC and Correale J (2021) Impact of Andropause on Multiple Sclerosis. Front. Neurol. 12:766308. doi: 10.3389/fneur.2021.766308
Received: 28 August 2021; Accepted: 18 October 2021;
Published: 05 November 2021.
Edited by:
Hans-Peter Hartung, Heinrich Heine University of Düsseldorf, GermanyReviewed by:
Jefferson Becker, Pontifical Catholic University of Rio Grande Do Sul, BrazilJorge Tolivia, University of Oviedo, Spain
Copyright © 2021 Ysrraelit and Correale. This is an open-access article distributed under the terms of the Creative Commons Attribution License (CC BY). The use, distribution or reproduction in other forums is permitted, provided the original author(s) and the copyright owner(s) are credited and that the original publication in this journal is cited, in accordance with accepted academic practice. No use, distribution or reproduction is permitted which does not comply with these terms.
*Correspondence: Maria C. Ysrraelit, mcysrraelit@fleni.org.ar
†These authors have contributed equally to this work