- Department of Medical Imaging Center, Qinghai University Affiliated Hospital, Xining, China
Objective: Headache and memory impairment are the primary clinical symptoms of chronic mountain sickness (CMS). In this study, we used voxel-based morphometry (VBM) and the amplitude of the low-frequency fluctuation method (ALFF) based on blood oxygen level-dependent functional magnetic resonance imaging (BOLD-fMRI) to identify changes in the brain structure and function caused by CMS.
Materials and Methods: T1W anatomical images and a resting-state functional MRI (fMRI) of the whole brain were performed in 24 patients diagnosed with CMS and 25 normal controls matched for age, sex, years of education, and living altitude. MRI images were acquired, followed by VBM and ALFF data analyses.
Results: Compared with the control group, the CMS group had increased gray matter volume in the left cerebellum crus II area, left inferior temporal gyrus, right middle temporal gyrus, right insula, right caudate nucleus, and bilateral lentiform nucleus along with decreased gray matter volume in the left middle occipital gyrus and left middle temporal gyrus. White matter was decreased in the bilateral middle temporal gyrus and increased in the right Heschl's gyrus. Resting-state fMRI in patients with CMS showed increased spontaneous brain activity in the left supramarginal gyrus, left parahippocampal gyrus, and left middle temporal gyrus along with decreased spontaneous brain activity in the right cerebellum crus I area and right supplementary motor area.
Conclusion: Patients with CMS had differences in gray and white matter volume and abnormal spontaneous brain activity in multiple brain regions compared to the controls. This suggests that long-term chronic hypoxia may induce changes in brain structure and function, resulting in CMS.
Introduction
Chronic mountain sickness (CMS) was initially described by Monge in 1928 and was later replicated by other groups in the early medical literature (1–6). It is a syndrome caused by an inability of individuals to adapt to high altitudes (altitude >2,500 m) and is typically manifested as hyperhemoglobinemia and hypoproteinemia (7). Common symptoms include headache, dizziness, insomnia, fatigue, tinnitus, attention deficit, amnesia, and muscle and joint pain. Some patients may also have cerebral edema, eventually leading to encephalopathy (8). Hypoxia is an important cause of CMS due to low oxygen levels in high-altitude areas. In recent years, with an increase in the plateau population, CMS has received greater attention (9, 10). Most published literature has focused on the epidemiology, pathophysiology, and genetics (11–16) of CMS. In contrast, there is little research on imaging, except for a few studies, such as the one by Bao et al., which evaluated brain damage in patients with CMS. The authors found that multiple structural changes occur in the brains of patients with CMS and are correlated to the severity of the disease and the impairment of cognitive functions (17). Wei et al. suggested that the differences in cortical morphometry in the brains of native Tibetans may reflect adaptations related to high altitudes (18). Furthermore, imaging studies of plateau residents have been limited to normal controls. Structural MRI studies of adolescents migrating from sea level to a high altitude environment demonstrated the following changes: (a) decreased gray matter volume in the right postcentral gyrus, right superior frontal gyrus, bilateral anterior insula, right anterior cingulate cortex, bilateral pre-frontal cortex, left precentral cortex, and right lingual cortex, and (b) increased gray matter volume in the right middle frontal gyrus, right parahippocampal gyrus, right inferior and middle temporal gyri, bilateral inferior ventral pons, and right cerebellum crus I (19, 20). A study on adolescents migrating from a high altitude to sea level environment demonstrated the following changes: (a) increased gray matter volume in the left insula, left inferior parietal gyrus, and right superior parietal gyrus, (b) decreased gray matter volume in the left precentral cortex and multiple sites in the cerebellar cortex (left lobule VIII, bilateral lobule VI, and crus I/II), and (c) decreased white matter volume in the right superior frontal gyrus (21). Studies using functional MRIs (fMRIs) on long-term high-altitude residents have indicated that subjects' brains undergo functional changes (22, 23); the left pyramis, and left and superior temporal gyrus were more activated, and the left middle occipital gyrus was less activated in the high-altitude group than in the control group (22). High-altitude residents also showed a decreased activation in the inferior and middle frontal gyrus, the middle occipital and lingual gyrus, the pyramis of the vermis, and the thalamus (23). Our research aims to study structural and functional changes simultaneously, using voxel-based morphometry (VBM) and the amplitude of low-frequency fluctuation (ALFF) methods, from resting-state fMRI in patients with CMS. The VBM provides a quantitative and comprehensive assessment of anatomical changes in the brain (24). This technique has been widely used in the study of changes in the brain morphology caused by various diseases (25–28). Currently, the methods for analyzing resting-state blood oxygen level-dependent functional magnetic resonance imaging (BOLD-fMRI) data are regional homogeneity (ReHo), ALFF, and independent component analysis (ICA). The ALFF method has been widely used in clinical settings (29–31) to deduce information about the amplitude of spontaneous activity of regional neurons (32, 33). To the best of our knowledge, no studies have used both structural MRI volumetrics and resting-state fMRI to study CMS.
Materials and Methods
Subjects
After being assessed at our institution between May 2017 and May 2020, 25 patients diagnosed with CMS were selected for this study. One patient was excluded because of excessive head motion during the MRI. The currently used international diagnostic criteria for CMS are the Qinghai criteria (34), which include indicators for hemoglobin and various clinical symptoms. None of the patients received any treatment for CMS before MRI examination. Control subjects (n = 25) with comparable age, educational background, and high-altitude exposure were recruited. Physical examination showed no positive signs of CMS and a normal hemogram and blood pressure in the control subjects. All subjects included in the study were right-handed and had lived in a plateau at altitudes above 3,000 m for at least 5 years. The subjects were native highlanders from the Qinghai Province and had no documented drug abuse, high blood pressure, diabetes, neurological disorders, or history of head injuries such as loss of consciousness and mental illness. Men are reportedly more prone to CMS than women (35–37). To avoid sex-related differences, we recruited only men in our study. The experimental protocol was approved by the ethics committee of our institution. All subjects provided written informed consent before participating in the study.
Equipment and Technical Indicators
The MRI scans were conducted using a Philips Achieva 3.0 TX scanner with an eight-channel coil. Anatomical MRI scans were performed on all subjects, including fluid attenuated inversion recovery (FLAIR), T2-weghted imaging (T2WI), and diffusion-weighted imaging (DWI). Further scans were performed on subjects who had no abnormalities on baseline images that could interfere with quantitative analysis and interpretation of the resting-state fMRI. A turbo field echo (TFE) sequence was used to obtain three-dimensional (3D)-T1 images with the following parameters: TR = 7.5 ms, TE = 3.5 ms, excitation angle: 7°, matrix: 256 × 256, transverse scanning, slice thickness: 2 mm, 176 images. Using the echo-planar imaging (EPI) sequence (TR/TE = 2,500/30 ms, FOV = 224 mm X 224 mm, flip angle = 90), axial slices of 3.5 mm thickness covering the whole brain were applied to each subject to acquire the BOLD fMRI data (scan time: 385 s).
Data Processing
VBM Method
Data were processed using the VBM8 toolbox implemented in SPM8 (Wellcome Department of Imaging Neuroscience, University College London, London, UK). The analysis was performed using MATLAB (MathWorks, Natick, MA, USA). The following steps were included: (1) Each scan was visually examined for scanning artifacts and anatomic abnormalities, (2) The plane of the image was adjusted to the anterior commissure (AC) and posterior commissure (PC) lines on the transverse plane (3). Each reoriented image was segmented into gray matter, white matter, and cerebrospinal fluid. Diffeomorphic anatomical registration through exponentiated lie algebra (DARTEL) was used to achieve a high dimension of registration and standardization, and (4) normalized images were transformed into Montreal Neurological Institute (MNI) space. Gray matter images were then smoothed using a Gaussian kernel of 8 mm full width at half maximum (FWHM).
ALFF Method
The fMRI data were preprocessed using a data processing assistant for resting-state fMRI (DPARSF). The first 10 time points were discarded to stabilize the signals. One patient was excluded due to head motion of >1.5 mm and 1.5 degrees. After processing, the data for each individual were spatially normalized to the MNI space. Images were resampled to 3 mm and smoothed with a kernel of 8 mm FWHM. The REST package (REST, http://resting-fmri. sourceforge.net) was used to calculate the ALFF using a voxel-based approach. The power spectrum was obtained by square-rooted FFT and averaged across 0.01–0.08 Hz at each voxel. The averaged square root was used as the ALFF. To reduce the global effects of variability across the participants, the ALFF of each voxel was divided by the global mean ALFF value obtained previously within the whole-brain mask.
Statistical Analysis
VBM Analysis
A two-sample t-test was conducted between the patients and control groups. A statistical parametric map was generated at |t| > 2.0 1, P < 0.05, cluster > 50.
ALFF Analysis
Two-sample t-test was conducted on the groups. The statistical parametric map was generated at P < 0.05, cluster > 100 (uncorrected).
Results
Demographic and Physiological Information
The demographic and physiological data of the CMS and control groups, including age, body weight, altitude, education, CMS score, and hematological parameters (HGB, RBC), are shown in Table 1.
Detailed Information of CMS Patients
Detailed information of the patients with CMS, including the altitude where they lived (in meters), hematological parameters (HGB, RBC), CMS score, and duration of residence at high altitude, is shown in Table 2.
VBM Result
Compared with the control group, the patients with CMS showed an increase in gray matter volume in the right cerebellum crus II area, left inferior temporal gyrus, right middle temporal gyrus, right insula, right caudate nucleus, and bilateral lentiform nucleus. Volume was decreased in the left middle occipital gyrus and left middle temporal gyrus (Table 3, Figure 1). White matter was decreased in the bilateral middle temporal gyrus and increased in the right Heschl's gyrus (Table 4, Figure 2).
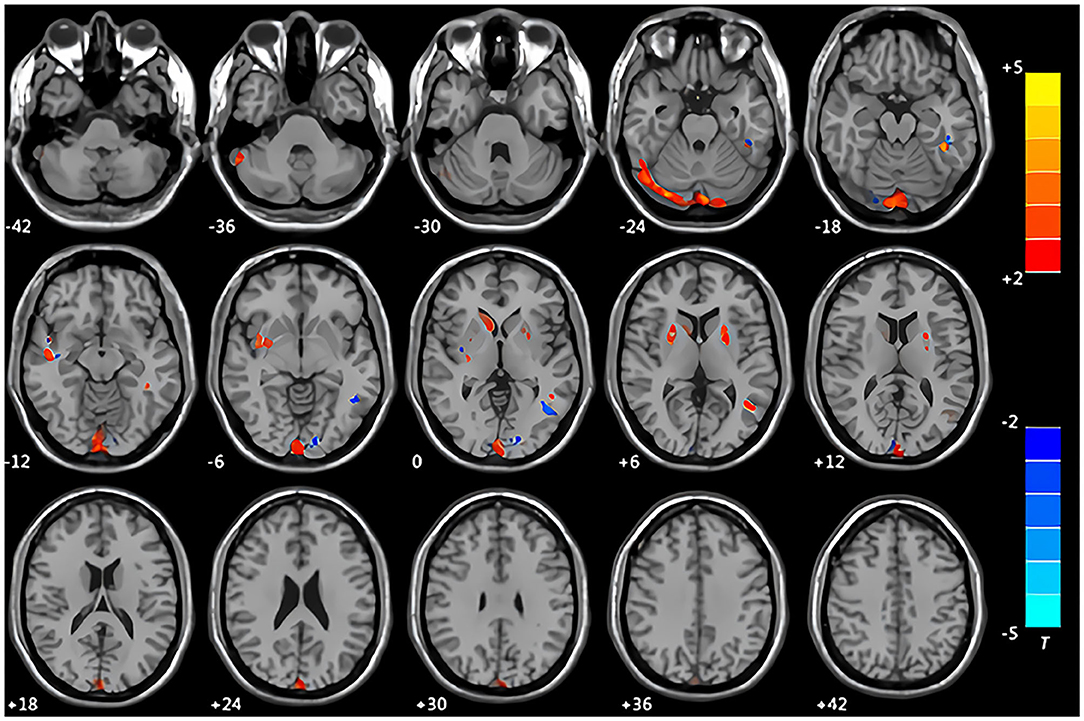
Figure 1. Maps of changed brain regions of gray matter in patients with chronic mountain sickness (CMS) compared with the control group. Areas in red are regions where gray matter volume was significantly increased: left cerebellum crus II area, left inferior temporal gyrus, right insula, right caudate nucleus, bilateral lentiform nucleus. Areas in blue are regions where gray matter volume was significantly decreased: left middle occipital gyrus, left middle temporal gyrus.
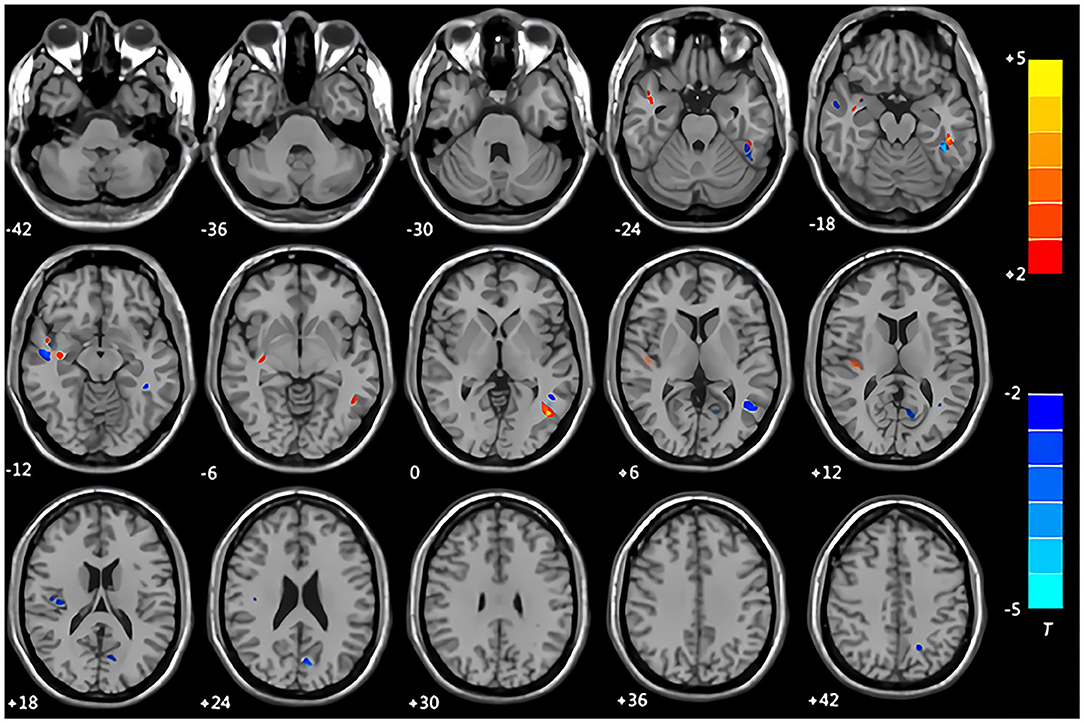
Figure 2. Maps of changed brain regions of white matter in patients with CMS compared with the control group. Areas in blue are regions where white matter volume was significantly decreased in the bilateral middle temporal gyrus. Areas in red are regions where white matter volume was significantly increased in the right Heschl's gyrus.
ALFF Result
Compared with the control group, the patients with CMS in the resting state showed increased spontaneous brain activity in the left supramarginal gyrus, left parahippocampal gyrus, and left middle temporal gyrus along with decreased spontaneous brain activity in the right cerebellum crus I area and right supplementary motor area (Table 5, Figure 3).
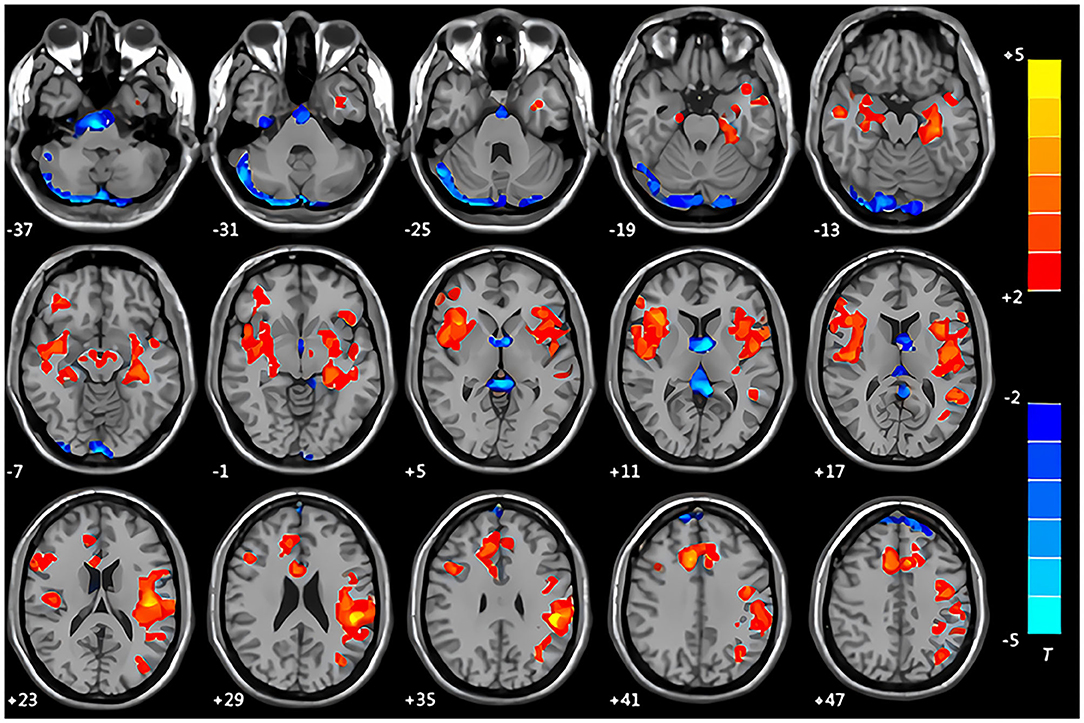
Figure 3. Maps of the amplitude of the low-frequency fluctuation (ALFF) changes in patients with CMS compared with the control group. Areas in red are regions where ALFF value was significantly increased: left supramarginal gyrus, left parahippocampal gyrus, left middle temporal gyrus. Areas in blue are regions where ALFF value was significantly decreased: right cerebellum crus I area, right supplementary motor area.
Discussion
Physiological and Biochemical Effects of Hypoxia on Human Brain Tissue
The human cerebral cortex has been shown to have features of neuroplasticity with a structure and function that can be modified and adapted to different stimuli (38). Certain physical and environmental states can lead to alterations in respiratory and circulatory function, hemoglobin concentration, and arterial oxygen saturation with subsequent changes in cerebral blood flow, thereby leading to cumulative changes in brain structure (39). The brain responds to hypoxia by regulating the changes in the cardiovascular and respiratory systems; therefore, the physiological response to hypoxia may lead to structural changes in various brain regions (20). The microstructure of gray matter is a complex mixture of nerve cells, fibers, neuroglial cells, and vessels. An increase in gray matter volume may be associated with neural cell hyperplasia, increased synapses, or changes in the cerebral vasculature (40). Under hypoxic conditions, however, the decrease in gray matter volume may be associated with the byproducts of metabolism and increased glutamate release from nerve cells (41). The new cortex can regenerate, which can be induced by hypoxia and cerebral ischemia, stimulating the proliferation of microglia and macrophages (42, 43).
The Implications of Changes in Gray Matter
In this study, we investigated the brain structure and functional changes in patients with CMS using VBM and resting-state fMRI. Our results demonstrate that the patients with CMS had increased gray matter in the right cerebellum crus II area, left inferior temporal gyrus, right insula, right caudate nucleus, and bilateral lentiform nucleus along with decreased volume in the left middle occipital gyrus and left middle temporal gyrus. These findings were similar to the results of a study by Zhang et al., which found increased gray matter in the right cerebellum crus II area and right middle temporal gyrus. The insular cortex has been shown to be associated with the control of cardiovascular disease, while the frontal island plays an important role in dyspnea, which is a symptom demonstrated by most patients with CMS (44–47). Several studies have shown that the frontal island is necessary to maintain homeostasis in high-altitude environments. There is a strong correlation between the right frontal insula gray matter and aerobic capacity and significant activation of the insula with dyspnea on fMRI (48–50). In our study, the CMS group showed increased gray matter volume in the insula, which could explain the dyspnea symptoms in patients with CMS. Other brain functional studies on hypoxic patients suffering from a chronic obstructive pulmonary disease (COPD) or obstructive sleep apnea (OSA) also revealed changes in gray matter volume, similar to the findings of our study (51–53).
The Implications of Changes in White Matter
Our results demonstrated decreased white matter volume in the bilateral middle temporal gyrus. There was a decrease in gray matter volume in the left middle temporal gyrus. These findings suggest that the middle temporal gyrus may be more sensitive to long-term hypoxia, as seen in patients with CMS. In this study, changes in gray matter were greater than those in white matter, indicating that gray matter was more sensitive to hypoxia in patients with CMS than white matter (38).
The Implications of Changes in ALFF
The resting-state analysis of patients with CMS showed increased spontaneous brain activity in the left supramarginal gyrus, right central sulcus cover, left parahippocampal gyrus, and left middle temporal gyrus, while the right cerebellar amygdala and right supplementary motor area showed decreased spontaneous brain activity. Hypoxia has been shown to increase nerve cell activity and volume in the hippocampus, which suppresses the normal physiological response to hyperventilation, thereby aggravating the hypoxic state (47, 54–57). One study showed that adolescents migrating from sea level to high-altitude environments had increased gray matter in the hippocampus (19). Our results, using resting-state fMRI, showed increased spontaneous brain activity in the hippocampus of patients with CMS, inducing memory decline (19). Recent research has shown that the cerebellum is also involved in cognition, language, and emotion regulation (58, 59). Our study demonstrated increased ALFF activity and altered gray matter volume in the right cerebellum crus I area, which may be associated with changes in cognitive function, emotional disorder, and irritability in patients with CMS. Future studies with neuropsychological testing in our patient cohorts may be helpful to correlate the posterior fossa findings with altered resting-state fMRI and volumetrics compared to controls in terms of the neuropsychological changes described above.
Our study has multiple limitations. First, we investigated a small sample size of patients with CMS, and this may have skewed the results. Second, we did not perform neuropsychological tests in our patient cohorts to correlate our imaging findings with clinical manifestations. Third, white matter tract integrity may also be affected by hypoxia in patients with CMS. Future studies can also study white matter integrity using DTI tractography in patients with CMS.
In conclusion, the purpose of this study was to investigate changes in the brain structure using volumetrics and brain function using resting-state fMRI in patients with CMS due to long-term hypoxia. The results demonstrated altered gray matter volume, white matter volume, and brain function in patients with CMS compared to the controls, which were most likely related to long-term hypoxia. Therefore, this study shows the effects of long-term hypoxia on brain tissue from the perspective of imaging using volumetrics and resting-state fMRI.
Data Availability Statement
The original contributions presented in the study are included in the article/supplementary material, further inquiries can be directed to the corresponding author.
Ethics Statement
The studies involving human participants were reviewed and approved by Ethics Committee of Qinghai University Affiliated Hospital. The patients/participants provided their written informed consent to participate in this study.
Author Contributions
HB, FW, and XH: conception and design. HB: administrative support and final approval of manuscript. FW and XH: provision of study materials or patients, collection and assembly of data, data analysis, and interpretation. HB and XH: funding acquisition. XH and DK: software and validation. XH and HB: supervision. XH: visualization. XH and FW: manuscript writing. All authors contributed to the article and approved the submitted version.
Funding
This study was funded by the Science and Technology Project of Qinghai Province (No. 2017-SF-158).
Conflict of Interest
The authors declare that the research was conducted in the absence of any commercial or financial relationships that could be construed as a potential conflict of interest.
Publisher's Note
All claims expressed in this article are solely those of the authors and do not necessarily represent those of their affiliated organizations, or those of the publisher, the editors and the reviewers. Any product that may be evaluated in this article, or claim that may be made by its manufacturer, is not guaranteed or endorsed by the publisher.
References
2. Wu TY, Zhang Q, Chen QH. Twenty-six cases of chronic mountain sickness. Natl Med J China. (1987) 67:167–8.
3. Pei SX, Chen XJ, Si Ren BZ, Liu YH, Cheng XS, Harris EM, et al. Chronic mountain sickness in Tibet. Q J Med. (1989) 71:555–74.
4. Peñaloza D, Sime F. Chronic cor pulmonale due to loss of altitude acclimatization (chronic mountain sickness). Am J Med. (1971) 50:728–43. doi: 10.1016/0002-9343(71)90181-1
5. Guan W, Ga Q, Li R, Bai ZZ, Wuren T, Wang J, et al. Sleep disturbances in long-term immigrants with chronic mountain sickness: a comparison with healthy immigrants at high altitude. Respir Physiol Neurobiol. (2015) 206:4–10. doi: 10.1016/j.resp.2014.11.007
6. Rexhaj E, Rimoldi SF, Pratali L, Brenner R, Andries D, Soria R, et al. Sleep-disordered breathing and vascular function in patients with chronic mountain sickness and healthy high-altitude dwellers. Chest. (2016) 149:991–8. doi: 10.1378/chest.15-1450
7. Julian CG, Vargas E, Gonzales M, Dávila RD, Ladenburger A, Reardon L, et al. Sleep-disordered breathing and oxidative stress in preclinical chronic mountain sickness (excessive erythrocytosis). Respir Physiol Neurobiol. (2013) 186:188–96. doi: 10.1016/j.resp.2013.01.016
8. Bao H, Wang D, Zhao X, Wu Y, Yin G, Meng L, et al. Cerebral edema in chronic mountain sickness: a new finding. Sci Rep. (2017) 7:43224. doi: 10.1038/srep43224
10. Wu T, Kayser B. High altitude adaptation in Tibetans. High Alt Med Biol. (2006) 7:193–208. doi: 10.1089/ham.2006.7.193
11. Villafuerte FC. New genetic and physiological factors for excessive erythrocytosis and chronic mountain sickness. J Appl Physiol. (2015) 119:1481–6. doi: 10.1152/japplphysiol.00271.2015
12. Ronen R, Zhou D, Bafna V, Haddad GG. The genetic basis of chronic mountain sickness. Physiology. (2014) 29:403–12. doi: 10.1152/physiol.00008.2014
13. Hsieh MM, Callacondo D, Rojas-Camayo J, Quesada-Olarte J, Wang X, Uchida N, et al. SENP1, but not fetal hemoglobin, differentiates Andean highlanders with chronic mountain sickness from healthy individuals among andean highlanders. Exp Hematol. (2016) 44:483–90.e2. doi: 10.1016/j.exphem.2016.02.010
14. Maimaitiyimin D, Aikemu A, Kamilijiang M, Salamu A, Zhang X. Effects and mechanisms of acetyl-L-cysteine in rats with chronic mountain sickness with H1-NMR metabolomics methods. Med Sci Monit. (2014) 20:767–73. doi: 10.12659/MSM.890244
15. Negi PC, Asotra S, V RK, Marwah R, Kandoria A, Ganju NK, et al. Epidemiological study of chronic mountain sickness in natives of Spiti Valley in the Greater Himalayas. High Alt Med Biol. (2013) 14:220–9. doi: 10.1089/ham.2012.1127
16. Penaloza D, Arias-Stella J. The heart and pulmonary circulation at high altitudes: healthy highlanders and chronic mountain sickness. Circulation. (2007) 115:1132–46. doi: 10.1161/CIRCULATIONAHA.106.624544
17. Bao H, Li R, He M, Kang D, Zhao L DTI. Study on brain structure and cognitive function in patients with chronic mountain sickness. Sci Rep. (2019) 9:19334. doi: 10.1038/s41598-019-55498-9
18. Wei W, Wang X, Gong Q, Fan M, Zhang J. Cortical thickness of native tibetans in the qinghai- tibetan plateau. AJNR Am J Neuroradiol. (2017) 38:553–60. doi: 10.3174/ajnr.A5050
19. Zhang J, Zhang H, Li J, Chen J, Han Q, Lin J, et al. Adaptive modulation of adult brain gray and white matter to high altitude: structural MRI studies. PLoS ONE. (2013) 8:e68621. doi: 10.1371/journal.pone.0068621
20. Zhang J, Yan X, Shi J, Gong Q, Weng X, Liu Y. Structural modifications of the brain in acclimatization to high-altitude. PLoS ONE. (2010) 5:e11449. doi: 10.1371/journal.pone.0011449
21. Zhang J, Zhang H, Chen J, Fan M, Gong Q. Structural modulation of brain development by oxygen: evidence on adolescents migrating from high altitude to sea level environment. PLoS ONE. (2013) 8:e67803. doi: 10.1371/journal.pone.0067803
22. Yan X, Zhang J, Gong Q, Weng X. Adaptive influence of long term high altitude residence on spatial working memory: an fMRI study. Brain Cogn. (2011) 77:53–9. doi: 10.1016/j.bandc.2011.06.002
23. Yan X, Zhang J, Gong Q, Weng X. Prolonged high-altitude residence impacts verbal working memory: an fMRI study. Exp Brain Res. (2011) 208:437–45. doi: 10.1007/s00221-010-2494-x
24. Ashburner J, Friston KJ. Voxel-based morphometry–the methods. Neuroimage. (2000) 11:805–21. doi: 10.1006/nimg.2000.0582
25. Hribar M, Suput D, Carvalho AA, Battelino S, Vovk A. Structural alterations of brain grey and white matter in early deaf adults. Hear Res. (2014) 318:1–10. doi: 10.1016/j.heares.2014.09.008
26. Sarma MK, Nagarajan R, Keller MA, Kumar R, Nielsen-Saines K, Michalik DE, et al. Regional brain gray and white matter changes in perinatally HIV-infected adolescents. NeuroImage Clinical. (2014) 4:29–34. doi: 10.1016/j.nicl.2013.10.012
27. Shigemoto Y, Matsuda H, Kamiya K, Maikusa N, Nakata Y, Ito K, et al. In vivo evaluation of gray and white matter volume loss in the parkinsonian variant of multiple system atrophy using SPM8 plus DARTEL for VBM. NeuroImage Clinical. (2013) 2:491–6. doi: 10.1016/j.nicl.2013.03.017
28. Colloby SJ, O'Brien JT, Taylor JP. Patterns of cerebellar volume loss in dementia with lewy bodies and Alzheimer's? disease: A VBM-DARTEL study. Psychiatry Res. (2014) 223:187–91. doi: 10.1016/j.pscychresns.2014.06.006
29. Li HJ, Dai XJ, Gong HH, Nie X, Zhang W, Peng DC. Aberrant spontaneous low-frequency brain activity in male patients with severe obstructive sleep apnea revealed by resting-state functional MRI. Neuropsychiatr Dis Treat. (2015) 11:207–14. doi: 10.2147/NDT.S73730
30. Xi Q, Zhao X, Wang P, Guo Q, Jiang H, Cao X, et al. Spontaneous brain activity in mild cognitive impairment revealed by amplitude of low-frequency fluctuation analysis: a resting-state fMRI study. Radiol Med. (2012) 117:865–71. doi: 10.1007/s11547-011-0780-8
31. Yan C, Liu D, He Y, Zou Q, Zhu C, Zuo X, et al. Spontaneous brain activity in the default mode network is sensitive to different resting-state conditions with limited cognitive load. PLoS ONE. (2009) 4:e5743. doi: 10.1371/journal.pone.0005743
32. Zang YF, He Y, Zhu CZ, Cao QJ, Sui MQ, Liang M, et al. Altered baseline brain activity in children with ADHD revealed by resting-state functional MRI. Brain Dev. (2007) 29:83–91. doi: 10.1016/j.braindev.2006.07.002
33. Yang H, Long XY, Yang Y, Yan H, Zhu CZ, Zhou XP, et al. Amplitude of low frequency fluctuation within visual areas revealed by resting-state functional MRI. Neuroimage. (2007) 36:144–52. doi: 10.1016/j.neuroimage.2007.01.054
34. León-Velarde F, Maggiorini M, Reeves JT, Aldashev A, Asmus I, Bernardi L, et al. Consensus statement on chronic and subacute high altitude diseases. High Alt Med Biol. (2005) 6:147–57. doi: 10.1089/ham.2005.6.147
35. Draganski B, Gaser C, Busch V, Schuierer G, Bogdahn U, May A. Neuroplasticity: changes in grey matter induced by training. Nature. (2004) 427:311–2. doi: 10.1038/427311a
36. León-Velarde F, Arregui A, Vargas M, Huicho L, Acosta R. Chronic mountain sickness and chronic lower respiratory tract disorders. Chest. (1994) 106:151–5. doi: 10.1378/chest.106.1.151
37. Zubieta-Castillo G Sr, Zubieta-Calleja GR Jr, Zubieta-Calleja L. Chronic mountain sickness: the reaction of physical disorders to chronic hypoxia. J Physiol Pharmacol. (2006) 57(Suppl. 4):431–42.
38. Beall CM, Goldstein MC. Hemoglobin concentration of pastoral nomads permanently resident at 4,850-5,450 meters in Tibet. Am J Phys Anthropol. (1987) 73:433–8. doi: 10.1002/ajpa.1330730404
39. Iwasaki K, Zhang R, Zuckerman JH, Ogawa Y, Hansen LH, Levine BD. Impaired dynamic cerebral autoregulation at extreme high altitude even after acclimatization. J Cereb Blood Flow Metab. (2011) 31:283–92. doi: 10.1038/jcbfm.2010.88
40. Zatorre RJ, Fields RD, Johansen-Berg H. Plasticity in gray and white: neuroimaging changes in brain structure during learning. Nat Neurosci. (2012) 15:528–36. doi: 10.1038/nn.3045
41. Virués-Ortega J, Buela-Casal G, Garrido E, Alcázar B. Neuropsychological functioning associated with high-altitude exposure. Neuropsychol Rev. (2004) 14:197–224. doi: 10.1007/s11065-004-8159-4
42. Zhu C, Qiu L, Wang X, Xu F, Nilsson M, Cooper-Kuhn C, et al. Age-dependent regenerative responses in the striatum and cortex after hypoxia-ischemia. J Cereb Blood Flow Metab. (2009) 29:342–54. doi: 10.1038/jcbfm.2008.124
43. Pforte C, Henrich-Noack P, Baldauf K, Reymann KG. Increase in proliferation and gliogenesis but decrease of early neurogenesis in the rat forebrain shortly after transient global ischemia. Neuroscience. (2005) 136:1133–46. doi: 10.1016/j.neuroscience.2005.08.043
44. Verberne AJ, Owens NC. Cortical modulation of the cardiovascular system. Prog Neurobiol. (1998) 54:149–68. doi: 10.1016/S0301-0082(97)00056-7
45. Green AL, Paterson DJ. Identification of neurocircuitry controlling cardiovascular function in humans using functional neurosurgery: implications for exercise control. Exp Physiol. (2008) 93:1022–8. doi: 10.1113/expphysiol.2007.039461
46. Wager TD, Waugh CE, Lindquist M, Noll DC, Fredrickson BL, Taylor SF. Brain mediators of cardiovascular responses to social threat: part I: reciprocal dorsal and ventral sub-regions of the medial prefrontal cortex and heart-rate reactivity. Neuroimage. (2009) 47:821–35. doi: 10.1016/j.neuroimage.2009.05.043
47. Davenport PW, Vovk A. Cortical and subcortical central neural pathways in respiratory sensations. Respir Physiol Neurobiol. (2009) 167:72–86. doi: 10.1016/j.resp.2008.10.001
48. Peters J, Dauvermann M, Mette C, Platen P, Franke J, Hinrichs T, et al. Voxel-based morphometry reveals an association between aerobic capacity and grey matter density in the right anterior insula. Neuroscience. (2009) 163:1102–8. doi: 10.1016/j.neuroscience.2009.07.030
49. Frisancho AR, Martinez C, Velasquez T, Sanchez J, Montoye H. Influence of developmental adaptation on aerobic capacity at high altitude. J Appl Physiol. (1973) 34:176–80. doi: 10.1152/jappl.1973.34.2.176
50. von Leupoldt A, Dahme B. Cortical substrates for the perception of dyspnea. Chest. (2005) 128:345–54. doi: 10.1378/chest.128.1.345
51. Zhang H, Wang X, Lin J, Sun Y, Huang Y, Yang T, et al. Grey and white matter abnormalities in chronic obstructive pulmonary disease: a case-control study. BMJ Open. (2012) 2:e000844. doi: 10.1136/bmjopen-2012-000844
52. Macey PM, Kumar R, Woo MA, Valladares EM, Yan-Go FL, Harper RM. Brain structural changes in obstructive sleep apnea. Sleep. (2008) 31:967–77.
53. O'Donoghue FJ, Briellmann RS, Rochford PD, Abbott DF, Pell GS, Chan CH, et al. Cerebral structural changes in severe obstructive sleep apnea. Am J Respir Crit Care Med. (2005) 171:1185–90. doi: 10.1164/rccm.200406-738OC
54. Gozal D, Omidvar O, Kirlew KA, Hathout GM, Hamilton R, Lufkin RB, et al. Identification of human brain regions underlying responses to resistive inspiratory loading with functional magnetic resonance imaging. Proc Natl Acad Sci U S A. (1995) 92:6607–11. doi: 10.1073/pnas.92.14.6607
55. Harper RM, Gozal D, Bandler R, Spriggs D, Lee J, Alger J. Regional brain activation in humans during respiratory and blood pressure challenges. Clin Exp Pharmacol Physiol. (1998) 25:483–6. doi: 10.1111/j.1440-1681.1998.tb02240.x
56. Harper RM, Macey PM, Henderson LA, Woo MA, Macey KE, Frysinger RC, et al. fMRI responses to cold pressor challenges in control and obstructive sleep apnea subjects. J Appl Physiol. (2003) 94:1583–95. doi: 10.1152/japplphysiol.00881.2002
57. Macey KE, Macey PM, Woo MA, Harper RK, Alger JR, Keens TG, et al. fMRI signal changes in response to forced expiratory loading in congenital central hypoventilation syndrome. J Appl Physiol. (2004) 97:1897–907. doi: 10.1152/japplphysiol.00359.2004
58. Timmann D, Drepper J, Frings M, Maschke M, Richter S, Gerwig M, et al. The human cerebellum contributes to motor, emotional and cognitive associative learning. A review. Cortex. (2010) 46:845–57. doi: 10.1016/j.cortex.2009.06.009
Keywords: chronic mountain sickness, brain, hypoxia, voxel-based morphometry (VBM), amplitude of low frequency fluctuation (ALFF), functional MRI
Citation: Bao H, He X, Wang F and Kang D (2022) Study of Brain Structure and Function in Chronic Mountain Sickness Based on fMRI. Front. Neurol. 12:763835. doi: 10.3389/fneur.2021.763835
Received: 27 August 2021; Accepted: 08 December 2021;
Published: 07 January 2022.
Edited by:
Peter Herman, Yale University, United StatesReviewed by:
Ivan V. Brak, State Scientific Research Institute of Physiology and Basic Medicine, RussiaHua Guo, Tsinghua University, China
Copyright © 2022 Bao, He, Wang and Kang. This is an open-access article distributed under the terms of the Creative Commons Attribution License (CC BY). The use, distribution or reproduction in other forums is permitted, provided the original author(s) and the copyright owner(s) are credited and that the original publication in this journal is cited, in accordance with accepted academic practice. No use, distribution or reproduction is permitted which does not comply with these terms.
*Correspondence: Haihua Bao, YmFvaGVsZW4yJiN4MDAwNDA7c2luYS5jb20=
†These authors have contributed equally to this work and share first authorship