- 1Department of Internal Medicine II, University Hospital Regensburg, Regensburg, Germany
- 2Department of Nephrology, University Hospital Regensburg, Regensburg, Germany
- 3Department of Genetic Epidemiology, University Hospital Regensburg, Regensburg, Germany
Hypothesis: Positive airway pressure (PAP) is the standard treatment for sleep-disordered breathing (SDB), a prevalent condition in patients with type 2 diabetes mellitus (DM2). Recent studies showed that short-term PAP treatment may cause weight gain. However, long-term data for patients with DM2 are scarce. Therefore, the aim of the present analysis was to assess changes in weight and glycemic control in patients with DM2 and treated vs. untreated SDB.
Methods: The DIAbetes COhoRtE (DIACORE) study is a prospective population-based cohort study in patients with DM2. At baseline, patients of the DIACORE-SDB sub-study were tested for SDB [defined as apnea-hypopnea-index (AHI) ≥ 15/h] using a two-channel ambulatory SDB-monitoring device. In this observational study, PAP treatment was initiated in a subgroup of patients with SDB (SDB PAP) within clinical routine between the baseline and first follow-up visit [median observation period of 2.3 (2.2; 2.4) years], whereas the other patients with SDB did not receive PAP (SDB untreated). At baseline and first follow-up visit, weight and HbA1c were assessed.
Results: Of the 346 patients with SDB [mean age 68 years, 71% male, body-mass index (BMI) 31.9 kg/m2], 17% were in the SDB PAP and 83% in the SDB untreated group. Weight change within the observation period was similar in both groups (−0.2 and −0.9 kg; p = 0.322). The percentage of patients with severe weight gain (≥ 5 kg) within the observation period was significantly higher in the SDB PAP group compared to the SDB untreated group (15.0 vs. 5.6%; p = 0.011). Multivariable regression analysis, accounting for baseline HbA1c, insulin substitution, BMI, waist-to-hip ratio (WHR), physical activity, and AHI, showed that PAP treatment was significantly associated with a weight gain ≥ 5 kg [odds ratio (OR) = 3.497; 95% CI (1.343; 9.106); p = 0.010] and an increase in HbA1c [B = 2.410; 95% CI (0.118; 4.702); p = 0.039].
Conclusion: Median weight change was similar in patients with SDB with and without PAP treatment. However, patients with DM2 and PAP treatment have an increased risk of severe long-term weight gain and an increase in HbA1c.
Clinical Trial registration: DRKS00010498
Introduction
Sleep-disordered breathing (SDB) is a highly prevalent condition in patients with type 2 diabetes mellitus (DM2) (1). The prevalence of at least moderate obstructive sleep apnea [OSA, defined as apnea-hypopnea-index (AHI) ≥ 15/h] is estimated as 25–59% in patients with DM2 (2), compared to a prevalence of 50% in men and 23% in women in the general population (3). OSA is characterized by recurrent pharyngeal collapse, which results in upper airway obstruction and intermittent hypoxia (4). Arousals from sleep restore the patency of the airway but lead to sleep fragmentation (4).
Obesity is one of the most important risk factors for OSA (4). It contributes to the development of OSA because pharyngeal fat deposition reduces upper airway lumen and fat deposition in the thorax and abdomen reduces lung volume (5). Furthermore, elevated levels of leptin and inflammatory cytokines in obese patients impair the respiratory drive and the neuromuscular control of the upper airway (5).
The interaction between OSA and obesity is bidirectional since OSA can facilitate weight gain (6). Many patients with OSA suffer from daytime sleepiness due to sleep fragmentation. Therefore, they may be less active and gain weight if caloric intake is not reduced accordingly (7). Moreover, leptin and ghrelin levels are increased in patients with OSA (8). Leptin is produced by adipocytes and inhibits appetite (9). However, permanently elevated leptin levels in patients with OSA lead to a resistance to the weight-reducing effects of leptin (10). Elevated ghrelin levels in patients with OSA stimulate appetite, which can result in higher food intake followed by weight gain (7). These alterations in appetite-regulating hormones in addition to the activation of the sympathetic nervous system, the stimulation of the hypothalamic-pituitary-adrenal axis, and the increase in systemic inflammation are possible mechanisms for developing insulin resistance and DM2 in patients with OSA (11). Thus, OSA, obesity, and DM2 influence and impair each other (11). This is of particular interest as OSA, obesity, and DM2 are important cardiovascular risk factors (12).
There are some possible therapies of OSA, such as mandibular advancement devices, active position therapy, and upper airway stimulation therapy (13), but positive airway pressure (PAP) is the standard first-line treatment for OSA (14). It delivers compressed air into the upper airway to keep it open (14). Hence, the number of apneas and hypopneas and subjective daytime sleepiness are reduced (14). In meta-analyses, short-term PAP did not improve the glycemic control measured by HbA1c in patients with DM2 (15–19). The effect of PAP on weight was controversial. Some studies reported weight gain (20, 21), while others reported weight loss after initiation of PAP treatment (22). Drager et al. showed in a meta-analysis that PAP treatment promotes a small but significant increase in weight and BMI (23). However, data for patients with DM2 are scarce. The previous meta-analyses included only 128 and 496 patients with DM2 and analyzed only the short-term effect of PAP (treatment duration ≤ 6 months) on BMI (15, 18). To our knowledge, so far there are no studies investigating the long-term weight change following PAP treatment specifically in patients with DM2. Therefore, the aim of the present analysis was to assess long-term changes in weight and glycemic control in patients with DM2 and treated vs. untreated SDB.
Methods
Study Design—DIAbetes COhoRtE and DIACORE-SDB Sub-Study
The analyzed patients were participants of the DIACORE-SDB sub-study. DIACORE (DRKS00010498) is a prospective cohort study with follow-up visits every 2 years in patients with DM2 (24). The baseline study was conducted from 2010 to 2014 and included 3,000 patients recruited in the two study centers Regensburg and Mannheim, as previously described (24, 25). Briefly, written invitations were mailed to all patients with DM2 who were registered with one of five medical insurance companies and two diabetologists in Regensburg (24, 25). Additional invitations were sent to patients with DM2 who had received inpatient treatment at the University Hospital Regensburg's Internal Medicine Departments within 2 years before the mailing (24, 25).
Of the 3,000 patients recruited at baseline, we invited all patients recruited in Regensburg into the DIACORE-SDB sub-study. Total 1,415 patients agreed to participate and were tested with a two-channel ambulatory SDB-monitoring device at the baseline visit (Figure 1). Monitoring for SDB was not performed in the Mannheim study center (25). The patients did not receive any lifestyle recommendations within the study. The protocol, data protection strategy, and study procedures were approved by the Ethics Committees of participating institutions and are in accordance with the Declaration of Helsinki. Patients participated in DIACORE only after providing informed written consent. The study protocol has been previously described (24, 25).
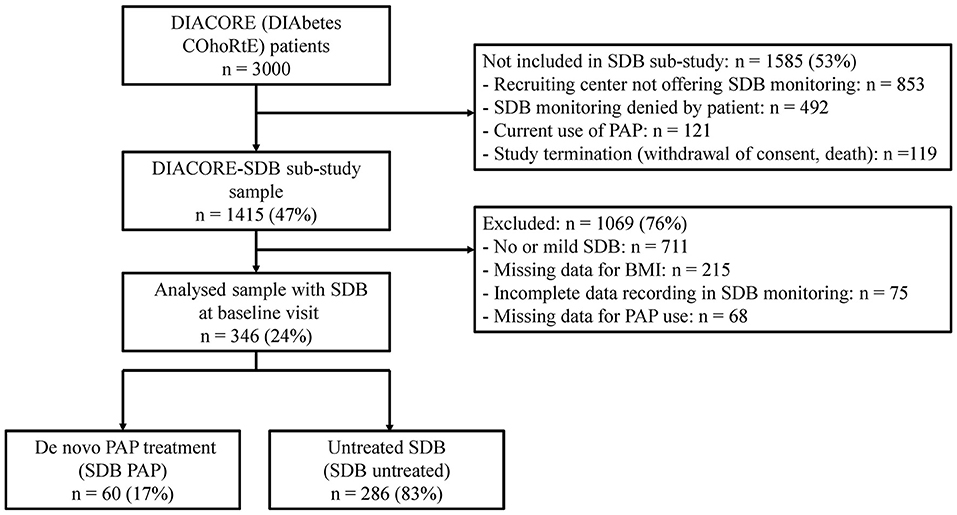
Figure 1. Study flow chart. BMI, body-mass index; PAP, positive airway pressure; SDB, sleep-disordered breathing.
Study Population—DIACORE and DIACORE-SDB Sub-Study
All patients with DM2 living in the region around Regensburg and Speyer were eligible for participating in DIACORE (24). The inclusion criteria were age ≥18 years, self-reported Caucasian ethnicity, and prevalent DM2 (24). Furthermore, patients were only included if they were able to fully understand the study information and provide informed written consent (24).
Exclusion criteria were the following: chronic renal replacement therapy, history of active malignancy within the last 5 years, autoimmune disease potentially affecting kidney function, hemochromatosis, type 1 diabetes, acute infection, fever, pregnancy, and chronic viral hepatitis or HIV infection (24). Patients from Regensburg were included in the DIACORE-SDB sub-study if they consented to perform SDB monitoring. Patients with current use of PAP were excluded (25).
Assessments at Baseline and Follow-Up Visit—DIACORE and DIACORE-SDB Sub-Study
A standardized protocol, such as physical examination, blood sampling, and a standardized online questionnaire, was performed at baseline and first follow-up visit (24). Anthropometric parameters (weight, height, waist circumference, and hip circumference) were measured in light clothing without shoes (24). Whole blood samples were drawn after the patient had rested for at least 15 min (24). Medication, physical activity level, and alcohol and nicotine consumption were determined by a questionnaire (24). High alcohol consumption was defined as drinking alcohol more than two times a week. Nicotine consumption was assessed by questionnaire and dichotomized as current vs. non-current smoking. Patients who have never smoked were included in the non-current smoking group. Low physical activity was defined as exercise less than three times a week (26). Severe weight gain within the observation period was defined as weight gain ≥5 kg or an increase in BMI ≥2 kg/m2 (27). In the DIACORE-SDB sub-study, SDB was assessed at the baseline visit, the initiation of PAP treatment was assessed at the first follow-up visit (Figure 2). In this analysis, the observation period was defined as the time between baseline and first follow-up visit.
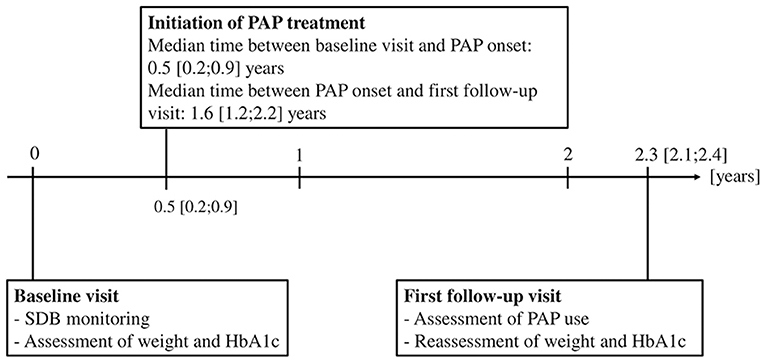
Figure 2. Timeline of initiation of PAP treatment and assessment of clinical data within the median observation period of 2.3 (2.2; 2.4) years. The mean self-reported PAP usage was 6.2 ± 2.4 h/night. Total 87% of the patients in the SDB PAP group used their PAP device ≥4 h/night, 8% did not use their PAP device regularly. HbA1c, hemoglobin A1c; PAP, positive airway pressure; SDB, sleep-disordered breathing.
Assessment of SDB at Baseline Visit—DIACORE-SDB Sub-Study
Nasal flow and pulse oximetry were measured using the portable ApneaLink® device (ResMed, Sydney, Australia) as previously described (25). Trained study personnel instructed participants on how to use the device at home. The use of ApneaLink® for assessing SDB has been validated in several studies (28, 29). By comparing ApneaLink® to polysomnography, the gold standard in assessing SDB, studies have reported a sensitivity of 73–91% and a specificity of 87–95% using an AHI cut-off value of 15/h (29, 30). AHI, oxygen desaturation index, mean oxygen saturation, and minimum oxygen saturation were documented (25). The default settings of the monitoring device were used for the definitions of apnea and hypopnea. Apnea was defined as an 80% decrease in airflow for 10 s, and hypopnea was defined as a decrease in the airflow by 50–80% vs. baseline for 10 s followed by a 4% decrease in oxygen saturation (31). ApneaLink® data were automatically scored but manually checked for accuracy and plausibility. Clinically relevant SDB was defined as AHI ≥ 15/h (32). Due to the missing chest band, a clear differentiation in obstructive and central apnea was not possible.
Additionally, subjective daytime sleepiness was assessed using the self-administered, validated Epworth Sleepiness Scale. Individuals were asked to rate their likelihood of falling asleep in several common situations. Possible scores range from 0 to 24. Excessive daytime sleepiness was defined as a score of ≥11 (33).
Assessment of PAP Treatment at First Follow-Up Visit – DIACORE-SDB Sub-Study
Results of the SDB monitoring at the baseline visit were reported to the patient and if requested, to their general practitioner. In the DIACORE-SDB sub-study, no treatment recommendation in respect of SDB was given to the patients. The decision to send the patient to a sleep laboratory for further diagnosis and treatment of SDB was made by the patient and general practitioner.
A newly initiated PAP treatment was assessed by questionnaire at the first follow-up visit (Figure 2). If a patient claimed to have started PAP treatment within the observation period, the start of treatment and the self-reported usage (hours used per night and days used per week) were recorded. The mean self-reported usage per night was calculated using the formula .
Statistical Analysis
Descriptive data were represented as mean ± SD for normally distributed variables and as median with interquartile ranges for non-normally distributed variables. Differences in continuous variables were evaluated with unpaired t-test for normally distributed variables and with Mann-Whitney U-test for non-normally distributed variables. Categorical variables were compared by a chi-square test and baseline and follow-up data by paired t-test.
Linear regression analysis was performed for continuous variables and logistic regression analysis for categorical variables to analyze the association of PAP treatment with change in HbA1c and weight gain ≥5 kg, respectively. Age, sex, HbA1c, oral antidiabetic drugs, insulin substitution, BMI, waist-to-hip ratio (WHR), physical activity, smoking status, alcohol consumption, Epworth Sleepiness Scale, and AHI at baseline were used as covariates. All variables with a p-value <0.2 in univariable regression were included in multivariable regression analysis (34). In addition, a multivariable regression with known potential confounders of change in weight and HbA1c, including baseline HbA1c, insulin substitution, BMI, WHR, physical activity, AHI, and PAP treatment, was performed. Results were shown as beta estimates with 95% CI in linear regression models and odds ratio (OR) with 95% CI in logistic regression models. The p-values < 0.05 were considered statistically significant. Data were analyzed using the SPSS software package (SPSS 25.0, IBM SPSS Statistics, Armonk, NW, USA).
Results
Of the 1,415 patients participating in the DIACORE-SDB sub-study, 346 had clinically relevant SDB (AHI ≥ 15/h) at baseline and were included in the analysis (Figure 1). At baseline, the mean age was 68 years, and 71% were men. The patients were mostly obese (mean BMI 31.9 kg/m2), had an HbA1c of 52 ± 11 mmol/mol (6.9 ± 1.0%), and a median DM2 duration of 11 (6, 17) years (Table 1).
Within the observation period of 2.3 (2.1; 2.4) years, 60 patients (17%) initiated PAP treatment (SDB PAP), while 286 patients (83%) with SDB did not start PAP (SDB untreated, Figure 1). Thus, the cohort of 346 SDB patients was divided into the SDB PAP group and the SDB untreated group. The PAP treatment was initiated at 0.5 (0.2; 0.9) years after the baseline visit, and the median duration between initiation of PAP treatment and the first follow-up visit was 1.6 (1.2; 2.2) years (Figure 2). The percentage of patients who used PAP for at least 1 year was 81%. The mean self-reported PAP usage was 6.2 ± 2.4 h per night and 52 patients (87%) used their PAP device ≥4 h per night.
The patients of the SDB PAP group had a higher WHR at baseline than the patients of the SDB untreated group (p = 0.011), but there was no difference in baseline weight or BMI (Table 1). Furthermore, the patients of the SDB PAP group were younger and had a higher baseline AHI compared to the patients of the untreated SDB group (p = 0.037 and p = 0.001; Table 1).
Weight Change Within the Observation Period
After a median observation period of 2.3 (2.1; 2.4) years, there was a statistically significant decrease in weight and BMI compared to baseline in the SDB untreated group (each p < 0.001), but not in the SDB PAP group (Table 2). However, the changes in weight and BMI within the observation period were similar in the SDB PAP and SDB untreated groups (Table 2; Supplementary Figure 1A). In the SDB PAP group, the percentage of patients with severe weight gain ≥5 kg within the observation period was significantly higher than in the SDB untreated group (p = 0.011, Supplementary Figure 1B). Moreover, the percentage of patients with a relevant increase in BMI (defined as ≥ 2 kg/m2) within the observation period was significantly higher in the SDB PAP group than in the SDB untreated group (p = 0.029; Table 2).
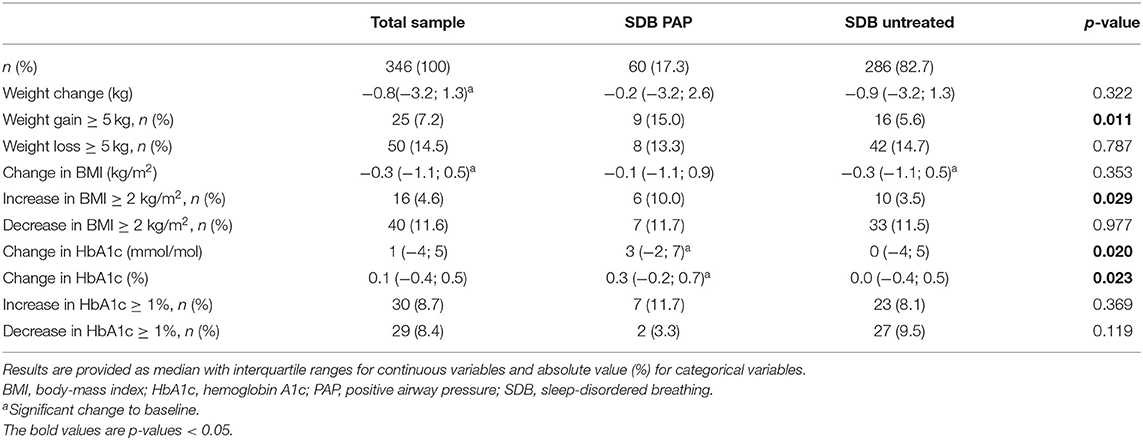
Table 2. Changes in weight and HbA1c of the analyzed 346 subjects within the observation period of 2.3 (2.2;2.4) years.
In the univariable regression analysis, PAP treatment, WHR, and HbA1c at baseline were significantly associated with severe weight gain within the observation period (Table 3). Multivariable regression including PAP treatment, WHR, and HbA1c, at baseline showed that PAP treatment and HbA1c at baseline were associated with an increased OR for a severe weight gain ≥5 kg within the observation period [OR = 3.256; 95% CI (1.293; 8.023); p = 0.012 and OR = 1.036; 95% CI (1.006; 1.066); p = 0.017, respectively; Table 3].
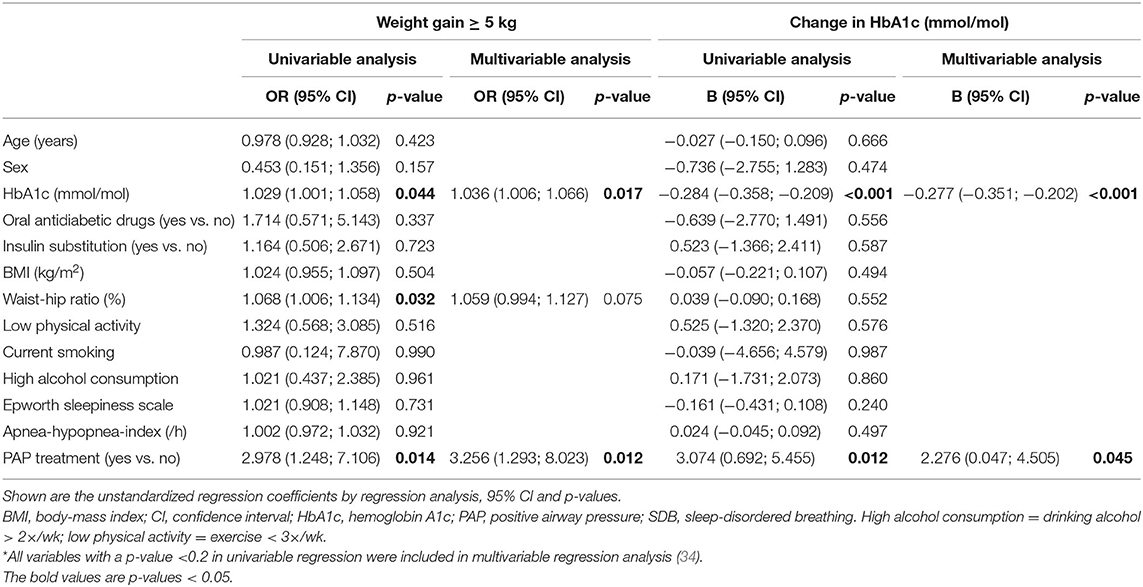
Table 3. Modulators for weight gain ≥5 kg and change in HbA1c within the observation period in 346 patients with type 2 diabetes mellitus*.
In addition, we performed a multivariable regression model, including known potential modulators of weight change, i.e., baseline HbA1c, insulin substitution, BMI, WHR, physical activity, AHI, and PAP treatment (Table 4). Also in this model, PAP treatment and HbA1c were significantly associated with severe weight gain ≥5 kg within the observation period [OR = 3.497; 95% CI (1.343; 9.106); p = 0.010 and OR = 1.036; 95% CI (1.006; 1.068); p = 0.019, respectively; Table 4]. Including current smoking as another confounder into this model did not change the association between PAP treatment and severe weight gain ≥5 kg within the observation period significantly [OR = 3.500; 95% CI (1.337; 9.163); p = 0.011].
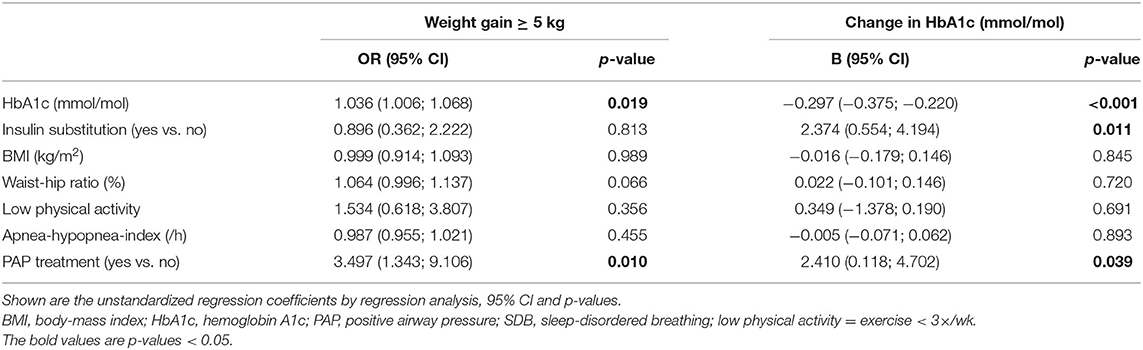
Table 4. Multivariable regression analysis with known potential modulators for weight gain ≥5 kg and change in HbA1c within the observation period in 346 patients with type 2 diabetes mellitus.
In a sub-analysis stratified by sex, the higher percentage of patients with severe weight gain within the observation period in the SDB PAP group remained significant among men (19.6 vs. 6.0% in the untreated SDB group, p = 0.003). In women, there was no significant difference between the SDB PAP and SDB untreated groups (0.0 vs. 4.7%, p = 0.407). The association of severe weight gain with PAP treatment was not significantly different between men and women, accounting for known modulators for weight gain, such as baseline HbA1c, insulin substitution, BMI, WHR, physical activity, and AHI (interaction term, p = 0.999).
Changes in Glycemic Control Within the Observation Period
The increase in HbA1c within the median observation period of 2.3 (2.2; 2.4) years was significantly higher in the SDB PAP group than in the SDB untreated group (Table 2; Supplementary Figure 1C). Moreover, in the SDB PAP group, the HbA1c was increased significantly compared to baseline (p = 0.008). There was no significant difference between the SDB PAP and SDB untreated groups regarding a strong increase (≥1%) or a strong decrease (≥1%) in HbA1c within the observation period (Table 2).
In univariable and multivariable regression analyses, adjusting for PAP treatment and HbA1c, PAP treatment, and HbA1c at baseline were significantly associated with changes in HbA1c (Table 3). PAP treatment was a predictor for an increase in HbA1c within the observation period [B = 2.276; 95% CI (0.047; 4.505); p = 0.045; Table 3].
Additionally, we performed a multivariable regression model, including known potential modulators of change in HbA1c, i.e., baseline HbA1c, insulin substitution, BMI, WHR, physical activity, AHI, and PAP treatment (Table 4). PAP treatment was significantly associated with an increase in HbA1c within the observation period [B = 2.410; 95% CI (0.118; 4.702); p = 0.039; Table 4].
In men, the increase in HbA1c within the observation period was significantly higher in the SDB PAP group compared to the SDB untreated group [3 mmol/mol (0.3%) vs. 0 mmol/mol (0.0%), p = 0.017], whereas in women, there was no difference between the SDB PAP and the SDB untreated groups [2 mmol/mol (0.2%) vs. 0 mmol/mol (0.0%), p = 0.388]. The association of change in HbA1c with PAP treatment was not significantly different between men and women accounting for known modulators for change in HbA1c, such as baseline HbA1c, insulin substitution, BMI, WHR, physical activity, and AHI (interaction term, p = 0.505).
Discussion
The present analysis investigated changes in weight and glycemic control in patients with DM2 and treated vs. untreated SDB and resulted in several key findings. First, there was no significant association between PAP treatment and median weight change within the observation period. Second, the percentage of patients with a severe weight gain ≥5 kg within the observation period was significantly higher in patients of the SDB PAP group. The SDB PAP group had a 3.5-fold increased OR for a weight gain ≥5 kg compared to the SDB untreated group. Third, HbA1c was increased significantly in the SDB PAP group compared to the SDB untreated group.
Our finding that PAP treatment was not associated with a significant change in median weight and BMI in patients with DM2 is consistent with the findings of previous meta-analyses (15, 18, 35). Feng et al. included in their analysis 128 patients with DM2 who received PAP treatment for a maximum of 4 months (15), Zhu et al. analyzed 496 patients with DM2 from randomized controlled trials (RCTs) with PAP treatment for 6 months or shorter (18). Both concluded that the effect of short-term PAP treatment on BMI was not significant in patients with DM2 (15, 18).
In contrast to these findings, a large meta-analysis with 3,181 patients from 25 RCTs reported a significant increase in weight and BMI following OSA treatment with PAP (23). With the exception of one study (36), PAP use ranged from 1–6 months with a median duration of 3 months (23). All of the mentioned meta-analyses investigated the short-term effects of PAP on weight and BMI. In contrast, the median duration of PAP use in our analysis was 1.6 years, representing a long-term PAP application.
Ou et al. analyzed the long-term effect of PAP on weight and BMI in a study with 2,843 patients (30% had DM2) and found no difference in weight change between the PAP and the control group after a mean follow-up of 3.8 years (37). A sub-analysis of those patients with DM2 was not performed (37). However, male participants who used PAP ≥4 h per night gained slightly more weight than matched male control subjects without PAP (37).
We did not find an increase in median weight following PAP treatment, such as the meta-analysis of Drager et al. (23). However, we found an increased OR for a severe weight gain ≥5 kg in patients with PAP treatment. Thus, PAP treatment may not result in clinically significant weight gain in all patients, but in some. Supporting this hypothesis, in a large retrospective analysis, the majority of PAP-treated patients with OSA (84%) had no significant change in weight during a 5-year follow-up period (38). However, 10% of the patients gained significant weight during the PAP treatment (38). These weight gainers were the youngest and most obese at baseline and had a higher prevalence of impaired fasting glucose or DM2 (38). Previous studies investigating the long-term effect of PAP on BMI and weight included a proportion of patients with pathological glucose tolerance no >40% (36–39), whereas the present analysis only examined patients with DM2 (Table 5).
In addition to changes in weight, we analyzed changes in glycemic control measured by HbA1c following PAP treatment. In contrast to previous evidence, our analysis showed a significant increase in HbA1c following PAP treatment in patients with DM2. Five meta-analyses have investigated the effect of PAP treatment on HbA1c in patients with DM2, and all of them reported that PAP did not alter HbA1c levels significantly (15–19). However, none of these studies exceeded a follow-up duration of 6 months. This short-term use of PAP might not be long enough to detect changes in HbA1c (18). HbA1c is a measure of glucose levels during the last 2–3 months, which takes time to change (18). A randomized clinical trial investigated the long-term effect of PAP on glycemic control in 888 patients with OSA and stable cardiovascular disease (40). In those with pre-existing DM2 (n = 274, 31%) and prediabetes (n = 452, 51%), there was no significant difference between the PAP and usual care groups in HbA1c during follow-up (median duration 4.3 years) (40). However, when restricted to patients with pre-existing DM2 but not using insulin, a slight but significant increase in HbA1c in PAP users was found (40). Two other studies examining the association between long-term PAP and glycemic control were controversial (41, 42) (Table 5). Therefore, further studies analyzing the long-term effect of PAP on HbA1c in patients with DM2 are warranted.
Some possible mechanisms could explain how PAP treatment could promote weight gain and an increase in HbA1c. PAP may reduce the basal metabolic rate (43). This could lead to a positive energy balance followed by weight gain since patients with OSA do not change their eating behavior and physical activity after PAP treatment (43, 44). Furthermore, PAP treatment is associated with increased levels of growth hormone and insulin-like growth factor 1 (45–47). The restoration of the growth hormone/insulin-like growth factor 1 axis after PAP treatment may lead to changes in body composition and an increase in lean body mass (47). Growth hormone does not only promote protein anabolism and muscle growth but it is also considered diabetogenic, such as insulin resistance and hyperglycemia (48). This could aggravate existing insulin resistance in patients with DM2 and thus impair glycemic control as observed in the present analysis. There were no significant differences between the groups regarding insulin substitution, oral antidiabetic drugs, or physical activity at baseline or follow-up that would explain the increase in HbA1c within the observation period in the SDB PAP group (Supplementary Table 1).
The association between weight gain, cardiovascular disease, and mortality in patients with DM2 is controversial (49, 50). The observed 3 mmol/mol (0.3%) increase in HbA1c in patients with DM2 and PAP treatment could result in an increased risk of all-cause and cardiovascular mortality since in a previous meta-analysis, every 11 mmol/mol (1%) increase in HbA1c was associated with a 15% increase of all-cause mortality and a 25% increase in cardiovascular mortality in patients with DM2 (51).
The strengths of the present analysis are the sample size and the high-resolution phenotyping of a study sample of outpatients with DM2 in a prospective setting. The observation period of 2.3 (2.2; 2.4) years and the median PAP duration of 1.6 years allowed us to investigate the changes in weight and glycemic control following PAP treatment over a long period of time. Furthermore, the compliance to the PAP treatment was high with a mean usage of 6.2 hours per night.
The following limitations warrant discussion: First, due to the observational character of the study, a causal relationship of the findings in this analysis cannot be concluded. Second, due to the simplified SDB monitoring, we cannot distinguish between obstructive and central sleep apnea. However, a large cohort study with a study sample representative of the German population suggested that the vast majority of patients had OSA and only a minority had central sleep apnea (52). Third, all information regarding the use of a PAP device was collected from self-reported data and not verified by data from the PAP device. It was shown that the objectively measured PAP use was approximately 1 h less than the patients have reported (53). Fourth, no data regarding caloric intake, eating behavior, or psychological traits were assessed. Thus, possible confounders for change in weight and HbA1c are missing in the present analysis.
In conclusion, median weight change was similar in patients with SDB with and without PAP treatment. However, patients with DM2 and PAP treatments have an increased risk of severe long-term weight gain and an increase in HbA1c. Further research on the long-term effect of PAP on weight change and glycemic control in patients with DM2 is warranted.
Data Availability Statement
The raw data supporting the conclusions of this article will be made available by the authors, without undue reservation.
Ethics Statement
The studies involving human participants were reviewed and approved by Ethikkommission University Regensburg. The patients/participants provided their written informed consent to participate in this study.
Author Contributions
LS, SS, and MA were involved in the conception, hypotheses delineation, and design of the present sub-study, the analysis and interpretation of such information, writing the article, and in its revision prior to submission. CB, IH, and BJ are the principal investigators of the DIACORE study and were involved in the design of this sub-study, the acquisition and interpretation of the data, and the critical revision of the article prior to submission. SS is the guarantor of this work and, as such, had full access to all data of the study and takes responsibility for the integrity of the data and the accuracy of the data analysis. All authors contributed to the article and approved the submitted version.
Funding
The DIACORE study was funded by the KfH-Stiftung Präventivmedizin e.V. CB received funding from the KfH-Stiftung Präventivmedizin e.V., the Else Kröner-Fresenius-Stiftung, and the Doktor Robert Pfleger-Stiftung. The DIACORE-SDB sub-study was funded by ResMed (Martinsried, Germany). The authors declare that this study received funding from KfH-Stiftung Präventivmedizin e.V., ResMed, and the Doktor Robert Pfleger-Stiftung. The funders were not involved in the study design, collection, analysis, interpretation of data, the writing of this article or the decision to submit it for publication.
Conflict of Interest
MA received research grants from ResMed, the ResMed Foundation, Philips Respironics, and the Else Kröner-Fresenius-Foundation (2018_A159) as well as lecture and consulting fees from ResMed, Philips Respironics, Boehringer-Ingelheim, NRI, Novartis and Bresotec.
The remaining authors declare that the research was conducted in the absence of any commercial or financial relationships that could be construed as a potential conflict of interest.
Publisher's Note
All claims expressed in this article are solely those of the authors and do not necessarily represent those of their affiliated organizations, or those of the publisher, the editors and the reviewers. Any product that may be evaluated in this article, or claim that may be made by its manufacturer, is not guaranteed or endorsed by the publisher.
Acknowledgments
We thank all participating patients of the DIACORE study. We thank the physicians and health insurance companies supporting the DIACORE study: Axel Andreae, Gerhard Haas, Sabine Haas, Jochen Manz, Johann Nusser, Günther Kreisel, Gerhard Bawidamann, Frederik Mader, Susanne Kißkalt, Johann Hartl, Thomas Segiet, Christiane Gleixner, Christian Scholz, Monika Schober (Chief of Supply Management, Allgemeine Ortskrankenkasse Bayern), Cornelia Heinrich (Communication Manager, Allgemeine Ortskrankenkasse Bayern), Thomas Bohnhoff (Disease Management, Techniker Krankenkasse), Thomas Heilmann (Head of Disease Management, Techniker Krankenkasse), Stefan Stern (Consulting Physician, Allgemeine Ortskrankenkasse Bayern), Andreas Utz (Head of Department, Allgemeine Ortskrankenkasse Bayern), Georg Zellner (Chief of Supply Management, Deutsche Angestellten Krankenkasse), Werner Ettl (Barmer-GEK), Thomas Buck (Barmer-GEK), Rainer Bleek (IKK classic), and Ulrich Blaudzun (IKK classic). We further thank the study nurses for their expert work in performing the study visits: Simone Neumeier, Sarah Hufnagel, Isabell Haller, Petra Jackermeier, Sabrina Obermüller, Christiane Ried, Ulrike Hanauer, Bärbel Sendtner, Natalia Riewe-Kerow, Konstantin Dumann, and Britta Hörmann (PhD-Students) for their expert work in conducting the study visits.
Supplementary Material
The Supplementary Material for this article can be found online at: https://www.frontiersin.org/articles/10.3389/fneur.2021.745049/full#supplementary-material
Abbreviations
AHI, apnea-hypopnea-index; BMI, body-mass index; DIACORE, DIAbetes CohORtE; DM2, type 2 diabetes mellitus; HbA1c, hemoglobin A1c; OSA, obstructive sleep apnea; PAP, positive airway pressure; SDB, sleep-disordered breathing; WHR, waist-to-hip ratio.
References
1. Kroner T, Arzt M, Rheinberger M, Gorski M, Heid IM, Böger CA, et al. Sex differences in the prevalence and modulators of sleep-disordered breathing in outpatients with type 2 diabetes. J Diabetes Res. (2018) 2018:7617524. doi: 10.1155/2018/7617524
2. Reutrakul S, Mokhlesi B. Obstructive sleep apnea and diabetes: a state of the art review. Chest. (2017) 152:1070–86. doi: 10.1016/j.chest.2017.05.009
3. Heinzer R, Vat S, Marques-Vidal P, Marti-Soler H, Andries D, Tobback N, et al. Prevalence of sleep-disordered breathing in the general population: the HypnoLaus study. Lancet Respir Med. (2015) 3:310–8. doi: 10.1016/S2213-2600(15)00043-0
4. Malhotra A, White DP. Obstructive sleep apnoea. Lancet. (2002) 360:237–45. doi: 10.1016/S0140-6736(02)09464-3
5. Ong CW, O'Driscoll DM, Truby H, Naughton MT, Hamilton GS. The reciprocal interaction between obesity and obstructive sleep apnoea. Sleep Med Rev. (2013) 17:123–31. doi: 10.1016/j.smrv.2012.05.002
6. Phillips BG, Hisel TM, Kato M, Pesek CA, Dyken ME, Narkiewicz K, et al. Recent weight gain in patients with newly diagnosed obstructive sleep apnea. J Hypertens. (1999) 17:1297–300. doi: 10.1097/00004872-199917090-00009
7. Pillar G, Shehadeh N. Abdominal fat and sleep apnea: the chicken or the egg? Diabetes Care. (2008) 31(Suppl. 2):S303–9. doi: 10.2337/dc08-s272
8. Harsch IA, Konturek PC, Koebnick C, Kuehnlein PP, Fuchs FS, Pour Schahin S, et al. Leptin and ghrelin levels in patients with obstructive sleep apnoea: effect of CPAP treatment. Eur Respir J. (2003) 22:251–7. doi: 10.1183/09031936.03.00010103
9. Gale SM, Castracane VD, Mantzoros CS. Energy homeostasis, obesity and eating disorders: recent advances in endocrinology. J Nutr. (2004) 134:295–8. doi: 10.1093/jn/134.2.295
10. Phillips BG, Kato M, Narkiewicz K, Choe I, Somers VK. Increases in leptin levels, sympathetic drive, and weight gain in obstructive sleep apnea. Am J Physiol Heart Circ Physiol. (2000) 279:H234–7. doi: 10.1152/ajpheart.2000.279.1.H234
11. Barone MTU, Menna-Barreto L. Diabetes and sleep: a complex cause-and-effect relationship. Diabetes Res Clin Pract. (2011) 91:129–37. doi: 10.1016/j.diabres.2010.07.011
12. Lechner K, Schacky C von, McKenzie AL, Worm N, Nixdorff U, Lechner B, et al. Lifestyle factors and high-risk atherosclerosis: pathways and mechanisms beyond traditional risk factors. Eur J Prev Cardiol. (2020) 27:394–406. doi: 10.1177/2047487319869400
13. Arzt M. Update – schlafbezogene Atmungsstörungen. Somnologie. (2020) 24:28–38. doi: 10.1007/s11818-020-00239-8
14. Qaseem A, Holty J-EC, Owens DK, Dallas P, Starkey M, Shekelle P. Management of obstructive sleep apnea in adults: a clinical practice guideline from the American College of Physicians. Ann Intern Med. (2013) 159:471–83. doi: 10.7326/0003-4819-159-7-201310010-00704
15. Feng Y, Zhang Z, Dong Z-Z. Effects of continuous positive airway pressure therapy on glycaemic control, insulin sensitivity and body mass index in patients with obstructive sleep apnoea and type 2 diabetes: a systematic review and meta-analysis. NPJ Prim Care Respir Med. (2015) 25:15005. doi: 10.1038/npjpcrm.2015.5
16. Chen L, Pei J-H, Chen H-M. Effects of continuous positive airway pressure treatment on glycaemic control and insulin sensitivity in patients with obstructive sleep apnoea and type 2 diabetes: a meta-analysis. Arch Med Sci. (2014) 10:637–42. doi: 10.5114/aoms.2014.44854
17. Labarca G, Reyes T, Jorquera J, Dreyse J, Drake L. CPAP in patients with obstructive sleep apnea and type 2 diabetes mellitus: systematic review and meta-analysis. Clin Respir J. (2018) 12:2361–8. doi: 10.1111/crj.12915
18. Zhu B, Ma C, Chaiard J, Shi C. Effect of continuous positive airway pressure on glucose metabolism in adults with type 2 diabetes: a systematic review and meta-analysis of randomized controlled trials. Sleep Breath. (2018) 22:287–95. doi: 10.1007/s11325-017-1554-x
19. Iftikhar IH, Blankfield RP. Effect of continuous positive airway pressure on hemoglobin A(1c) in patients with obstructive sleep apnea: a systematic review and meta-analysis. Lung. (2012) 190:605–11. doi: 10.1007/s00408-012-9404-x
20. Quan SF, Budhiraja R, Clarke DP, Goodwin JL, Gottlieb DJ, Nichols DA, et al. Impact of treatment with continuous positive airway pressure (CPAP) on weight in obstructive sleep apnea. J Clin Sleep Med. (2013) 9:989–93. doi: 10.5664/jcsm.3064
21. Redenius R, Murphy C, O'Neill E, Al-Hamwi M, Zallek SN. Does CPAP lead to change in BMI? J Clin Sleep Med. (2008) 04:205–9. doi: 10.5664/jcsm.27181
22. Loube DI, Loube AA, Erman MK. Continuous positive airway pressure treatment results in weight less in obese and overweight patients with obstructive sleep apnea. J Am Dietetic Assoc. (1997) 97:896–7. doi: 10.1016/S0002-8223(97)00220-4
23. Drager LF, Brunoni AR, Jenner R, Lorenzi-Filho G, Benseñor IM, Lotufo PA. Effects of CPAP on body weight in patients with obstructive sleep apnoea: a meta-analysis of randomised trials. Thorax. (2015) 70:258–64. doi: 10.1136/thoraxjnl-2014-205361
24. Dörhöfer L, Lammert A, Krane V, Gorski M, Banas B, Wanner C, et al. Study design of DIACORE (DIAbetes COhoRtE) - a cohort study of patients with diabetes mellitus type 2. BMC Med Genet. (2013) 14:25. doi: 10.1186/1471-2350-14-25
25. Stadler S, Zimmermann T, Franke F, Rheinberger M, Heid IM, Böger CA, et al. Association of sleep-disordered breathing with diabetes-associated kidney disease. Ann Med. (2017) 49:487–95. doi: 10.1080/07853890.2017.1306100
26. Stadler S, Jalili S, Schreib A, Jung B, Zeman F, Böger CA, et al. Association of sleep-disordered breathing with severe chronic vascular disease in patients with type 2 diabetes. Sleep Med. (2018) 48:53–60. doi: 10.1016/j.sleep.2018.05.001
27. Fernandez-Mendoza J, Vgontzas AN, Kritikou I, Calhoun SL, Liao D, Bixler EO. Natural history of excessive daytime sleepiness: role of obesity, weight loss, depression, and sleep propensity. Sleep. (2015) 38:351–60. doi: 10.5665/sleep.4488
28. Chen H, Lowe AA, Bai Y, Hamilton P, Fleetham JA, Almeida FR. Evaluation of a portable recording device (ApneaLink) for case selection of obstructive sleep apnea. Sleep Breath. (2009) 13:213–9. doi: 10.1007/s11325-008-0232-4
29. Arzt M, Woehrle H, Oldenburg O, Graml A, Suling A, Erdmann E, et al. Prevalence and predictors of sleep-disordered breathing in patients with stable chronic heart failure: The SchlaHF Registry. JACC Heart Fail. (2016) 4:116–25. doi: 10.1016/j.jchf.2015.09.014
30. ERMAN MK, Stewart D, Einhorn D, Gordon N, Casal E. Validation of the ApneaLink™ for the screening of sleep apnea: a novel and simple single-channel recording device. J Clin Sleep Med. (2007) 3:387–92. doi: 10.5664/jcsm.26861
31. Rodenbeck A. Manual der American Academy of Sleep Medicine. Somnologie. (2013) 17:122–30. doi: 10.1007/s11818-013-0611-3
32. Mayer G, Fietze I, Fischer J, Penzel T, Riemann D, Rodenbeck A. S3-Leitlinie. Somnologie. (2009) 13(S1):1–160. doi: 10.1007/s11818-009-0430-8
33. Johns MW. A new method for measuring daytime sleepiness: the Epworth sleepiness scale. Sleep. (1991) 14:540–5. doi: 10.1093/sleep/14.6.540
34. Maldonado G, Greenland S. Simulation study of confounder-selection strategies. Am J Epidemiol. (1993) 138:923–36. doi: 10.1093/oxfordjournals.aje.a116813
35. Yang D, Liu Z, Yang H, Luo Q. Effects of continuous positive airway pressure on glycemic control and insulin resistance in patients with obstructive sleep apnea: a meta-analysis. Sleep Breath. (2013) 17:33–8. doi: 10.1007/s11325-012-0680-8
36. Barbé F, Durán-Cantolla J, Sánchez-de-la-Torre M, Martínez-Alonso M, Carmona C, Barceló A, et al. Effect of continuous positive airway pressure on the incidence of hypertension and cardiovascular events in nonsleepy patients with obstructive sleep apnea: a randomized controlled trial. JAMA. (2012) 307:2161–8. doi: 10.1001/jama.2012.4366
37. Ou Q, Chen B, Loffler KA, Luo Y, Zhang X, Chen R, et al. The effects of long-term CPAP on weight change in patients with comorbid OSA and cardiovascular disease: data from the SAVE trial. Chest. (2019) 155:720–9. doi: 10.1016/j.chest.2018.08.1082
38. Myllylä M, Kurki S, Anttalainen U, Saaresranta T, Laitinen T. High adherence to CPAP treatment does not prevent the continuation of weight gain among severely obese OSAS patients. J Clin Sleep Med. (2016) 12:519–28. doi: 10.5664/jcsm.5680
39. Basoglu OK, Zou D, Tasbakan MS, Hedner J, Ryan S, Verbraecken J, et al. Change in weight and central obesity by positive airway pressure treatment in obstructive sleep apnea patients: longitudinal data from the ESADA cohort. J Sleep Res. (2018) 27:e12705. doi: 10.1111/jsr.12705
40. Loffler KA, Heeley E, Freed R, Meng R, Bittencourt LR, Gonzaga Carvalho CC, et al. Continuous positive airway pressure treatment, glycemia, and diabetes risk in obstructive sleep apnea and comorbid cardiovascular disease. Diabetes Care. (2020) 43:1859–67. doi: 10.2337/dc19-2006
41. Guest JF, Panca M, Sladkevicius E, Taheri S, Stradling J. Clinical outcomes and cost-effectiveness of continuous positive airway pressure to manage obstructive sleep apnea in patients with type 2 diabetes in the U.K. Diabetes Care. (2014) 37:1263–71. doi: 10.2337/dc13-2539
42. Malik JA, Masoodi SR, Shoib S. Obstructive sleep apnea in Type 2 diabetes and impact of continuous positive airway pressure therapy on glycemic control. Indian J Endocrinol Metab. (2017) 21:106–12. doi: 10.4103/2230-8210.196005
43. Tachikawa R, Ikeda K, Minami T, Matsumoto T, Hamada S, Murase K, et al. Changes in energy metabolism after continuous positive airway pressure for obstructive sleep apnea. Am J Respir Crit Care Med. (2016) 194:729–38. doi: 10.1164/rccm.201511-2314OC
44. Batool-Anwar S, Goodwin JL, Drescher AA, Baldwin CM, Simon RD, Smith TW, et al. Impact of CPAP on activity patterns and diet in patients with obstructive sleep apnea (OSA). J Clin Sleep Med. (2014) 10:465–72. doi: 10.5664/jcsm.3686
45. Hoyos CM, Killick R, Keenan DM, Baxter RC, Veldhuis JD, Liu PY. Continuous positive airway pressure increases pulsatile growth hormone secretion and circulating insulin-like growth factor-1 in a time-dependent manner in men with obstructive sleep apnea: a randomized sham-controlled study. Sleep. (2014) 37:733–41. doi: 10.5665/sleep.3580
46. Chen L-D, Lin L, Huang J-F, Chen X, Xu Q-Z, Liu J-N. Effect of continuous positive airway pressure on insulin growth factor-1 in patients with obstructive sleep apnea: a meta-analysis. Growth Horm IGF Res. (2015) 25:75–9. doi: 10.1016/j.ghir.2014.12.006
47. Münzer T, Hegglin A, Stannek T, Schoch OD, Korte W, Büche D, et al. Effects of long-term continuous positive airway pressure on body composition and IGF1. Eur J Endocrinol. (2010) 162:695–704. doi: 10.1530/EJE-09-0919
48. Sperling MA. Traditional and novel aspects of the metabolic actions of growth hormone. Growth Horm IGF Res. (2016) 28:69–75. doi: 10.1016/j.ghir.2015.06.005
49. Doehner W, Gerstein HC, Ried J, Jung H, Asbrand C, Hess S, et al. Obesity and weight loss are inversely related to mortality and cardiovascular outcome in prediabetes and type 2 diabetes: data from the ORIGIN trial. Eur Heart J. (2020) 41:2668–77. doi: 10.1093/eurheartj/ehaa293
50. Lee AK, Woodward M, Wang D, Ohkuma T, Warren B, Sharrett AR, et al. The risks of cardiovascular disease and mortality following weight change in adults with diabetes: results from ADVANCE. J Clin Endocrinol Metab. (2020) 105:152–62. doi: 10.1210/clinem/dgz045
51. Zhang Y, Hu G, Yuan Z, Chen L. Glycosylated hemoglobin in relationship to cardiovascular outcomes and death in patients with type 2 diabetes: a systematic review and meta-analysis. PLoS ONE. (2012) 7:e42551. doi: 10.1371/journal.pone.0042551
52. Woehrle H, Schoebel C, Oldenburg O, Young P, Fietze I, Ficker JH, et al. Low long-term mortality in patients with sleep apnoea and positive airway pressure therapy: analysis of a large German healthcare database. Somnologie. (2020) 24:1–8. doi: 10.1007/s11818-020-00259-4
Keywords: glycemic control (HbA1c), positive airway pressure (PAP), sleep-disordered breathing, weight, type 2 diabetes mellitus
Citation: Schaller L, Arzt M, Jung B, Böger CA, Heid IM and Stadler S (2021) Long-Term Weight Change and Glycemic Control in Patients With Type 2 Diabetes Mellitus and Treated vs. Untreated Sleep-Disordered Breathing—Analysis From the DIAbetes COhoRtE. Front. Neurol. 12:745049. doi: 10.3389/fneur.2021.745049
Received: 21 July 2021; Accepted: 27 October 2021;
Published: 02 December 2021.
Edited by:
Melissa Lipford, Mayo Clinic, United StatesReviewed by:
Lyudmila S. Korostovtseva, Almazov National Medical Research Centre, RussiaIrma Rukhadze, Albany Medical College, United States
Copyright © 2021 Schaller, Arzt, Jung, Böger, Heid and Stadler. This is an open-access article distributed under the terms of the Creative Commons Attribution License (CC BY). The use, distribution or reproduction in other forums is permitted, provided the original author(s) and the copyright owner(s) are credited and that the original publication in this journal is cited, in accordance with accepted academic practice. No use, distribution or reproduction is permitted which does not comply with these terms.
*Correspondence: Stefan Stadler, c3RlZmFuLnN0YWRsZXImI3gwMDA0MDt1a3IuZGU=
†These authors have contributed equally to this work