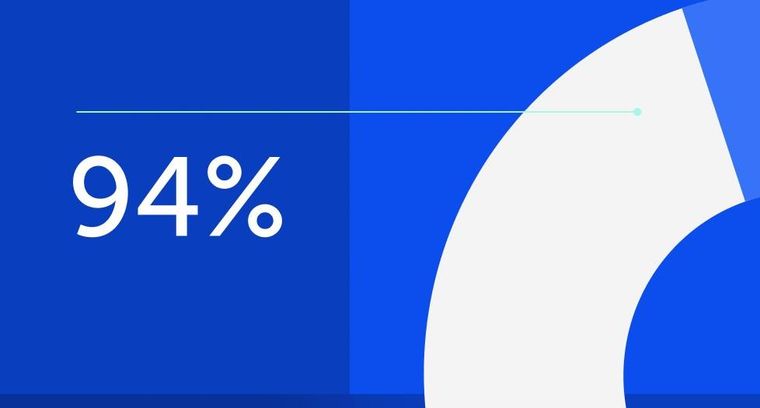
94% of researchers rate our articles as excellent or good
Learn more about the work of our research integrity team to safeguard the quality of each article we publish.
Find out more
ORIGINAL RESEARCH article
Front. Neurol., 28 October 2021
Sec. Neurocritical and Neurohospitalist Care
Volume 12 - 2021 | https://doi.org/10.3389/fneur.2021.732830
Background: Although increasing cerebral perfusion pressure (CPP) is commonly accepted to improve brain tissue oxygen pressure (PbtO2), it remains unclear whether recommended CPP targets (i. e., >60 mmHg) would result in adequate brain oxygenation in brain injured patients. The aim of this study was to identify the target of CPP associated with normal brain oxygenation.
Methods: Prospectively collected data including patients suffering from acute brain injury and monitored with PbtO2, in whom daily CPP challenge using vasopressors was performed. Initial CPP target was >60 mmHg; norepinephrine infusion was modified to have an increase in CPP of at least 10 mmHg at two different steps above the baseline values. Whenever possible, the same CPP challenge was performed for the following days, for a maximum of 5 days. CPP “responders” were patients with a relative increase in PbtO2 from baseline values > 20%.
Results: A total of 53 patients were included. On the first day of assessment, CPP was progressively increased from 73 (70–76) to 83 (80–86), and 92 (90–96) mmHg, which resulted into a significant PbtO2 increase [from 20 (17–23) mmHg to 22 (20–24) mmHg and 24 (22–26) mmHg, respectively; p < 0.001]. Median CPP value corresponding to PbtO2 values > 20 mmHg was 79 (74–87) mmHg, with 2 (4%) patients who never achieved such target. Similar results of CPP targets were observed the following days. A total of 25 (47%) were PbtO2 responders during the CPP challenge on day 1, in particular if low PbtO2 was observed at baseline.
Conclusions: PbtO2 monitoring can be an effective way to individualize CPP values to avoid tissue hypoxia. Low PbtO2 values at baseline can identify the responders to the CPP challenge.
Monitoring of patients with critical illness has expanded significantly over the past several decades. As for hemodynamics, respiratory or renal functions, several studies have underlined the importance of monitoring brain function, not only in patients with a primary brain injury [i.e., traumatic brain injury (TBI), subarachnoid hemorrhage (SAH), post-anoxic brain injury], but also in those with a systemic disease, such as sepsis, acute pancreatitis, or cardiogenic shock, who may present different alterations of cerebral function and potentially may develop secondary brain injuries (1), which can impact on long-term functional and cognitive recovery (2).
In patients suffering from TBI, the combination of intracranial pressure (ICP) and cerebral perfusion pressure (CPP)-guided therapy is recommended for the current management of these patients (3); similarly, ICP and CPP are the cornerstone of management of severe non-traumatic brain injury patients, although the evidence suggesting that such approach might impact on long-term outcomes remain limited (4). Importantly, increased ICP could be a late and insensitive indicator of some secondary brain injuries (5, 6); in particular, the occurrence of tissue hypoxia may occur even in the absence of intracranial hypertension and, when untreated or refractory to therapeutic interventions, is associated with an increased risk of poor neurological outcome in brain injured patients (7, 8). As such, rather than protocolizing therapies to target fixed ICP and CPP values for all patients, independently from the underlying brain injury, comorbid diseases, and the severity of brain edema, the implementation of brain tissue oxygen pressure (PbtO2) monitoring could be helpful to individualize ICP and CPP values based on cerebral oxygenation.
Although higher than recommended CPP targets (i.e., >60 mmHg) have been associated with adequate PbtO2 values in heterogeneous populations of brain injured patients, few prospective studies have evaluated the effects of increasing CPP using vasopressors on PbtO2 in such patients. In one small study (n = 11) on TBI patients, increasing CPP from 70 to 90 mmHg with norepinephrine resulted in a significant increase in PbtO2 (from 17 ± 8 to 22 ± mmHg), despite brain metabolism was only marginally affected (8). In another study including 14 TBI patients, increasing CPP resulted also in a significant increase in PbtO2, although this was measured after a challenge of inspired oxygen at 100% on the ventilator (9). However, all these studies did not specifically investigate the proportion of patients requiring higher than recommended CPP to obtain adequate PbtO2 values, neither whether this CPP target would change along the hospital stay.
The aim of this study was therefore to evaluate which level of CPP corresponds to an adequate PbtO2 in an heterogeneous population of brain injured patients. Secondary aims were to assess: (a) whether this level of CPP change over time and (b) the characteristics of patients requiring higher than recommended CPP targets to have optimal brain oxygenation values.
This was an analysis of prospectively collected data including all adult (>18 years of age) patients with an acute primary brain injury admitted to the ICU of Erasme Hospital, Brussels, Belgium, between January 2016 and December 2020. Eligible patients were those: (a) having a PbtO2 monitoring catheter, which was inserted according to the decision of senior ICU physician and an experienced neurosurgeon; (b) receiving vasopressors to target a CPP of at least 60–70 mmHg; (c) who underwent daily CPP challenge as part of routine patient management (see below). Data for all measurements were recorded into the patient management data system (PDMS, Picis Critical Care Manager; Picis Inc., Wakefield, MA, USA). Exclusion criteria were a malfunctioning PbtO2 catheter, the absence of vasopressor therapy, elevated ICP (>25 mmHg) refractory to different interventions (i.e., sedation, osmotic therapy, and hyperventilation), clinical contraindication to increased CPP (i.e., frequent arrhythmias or acute heart failure). The study was approved by the ethical committee of the Erasme Hospital (Comité d'Ethique Hospitalo-Facultaire Erasme – ULB; P2021/038), which waived the need of informed consent given the observational design of the study analyzing recorded data into the PDMS.
Patients were managed according to local protocols, based on international recommendations (3, 4, 10, 11). A triple lumen bolt allowing the insertion of a PbtO2 probe (IM3.ST_EU; Integra LifeSciences Corporation, Plainsboro, NJ, USA), alone or in association with an eight-contact depth EEG electrode and a microdialysis catheter, was placed in the operating room by a neurosurgeon in patients with TBI, subarachnoid hemorrhage (SAH), or intracranial hemorrhage (ICH), who had indications for ICP monitoring (i.e., abnormal CT-scan findings and a Glasgow Coma Score on admission <9). The bolt was positioned in the normal-appearing brain area of the injured side or, in case of aneurysmal SAH, on either the ipsilateral side of the aneurysm (i.e., anterior circulation) or on the right side (i.e., no aneurysm identified or aneurysm located in the posterior circulation). Other continuously monitored variables included electrocardiogram, mean arterial pressure (MAP), peripheral oxygen saturation, end-tidal carbon dioxide and body temperature (i.e., with urinary or esophageal probes), as a routine approach in all severe brain injured patients.
For patients requiring vasopressors, initial CPP and PbtO2 targets are >60 mmHg and >20 mmHg, respectively. Cerebral perfusion pressure (CPP) was calculated as the difference between MAP and ICP; MAP was zeroed at the level of the left atrium (i.e., with patient at 30° recumbent position). After the initial daily assessment of the patient, which can also include the evaluation of pupillary function using an automated pupillometry (NeurOptics NPi-200; Neuroptics, Irvine, CA, USA) to calculate the Neurological Pupil Index (NPi), or brain flow velocities with Transcranial Doppler (TCD) to measure mean flow velocities (mFV) and the pulsatility index (PI) (12), CPP was set around 60–70 mmHg (i.e., if not already within these ranges) and norepinephrine infusion was modified to have an increase in CPP of at least 10 mmHg at two different steps above the baseline values. At each CPP level (i.e., stabilized for at least 5 min), PbtO2, ICP, NPi, mFV, and PI were collected and results used to adjust vasopressor infusion daily in order to define CPP goals according to PbtO2 values (i.e., target ≥ 20 mmHg) and/or guide further interventions. This CPP challenge was part of the routine management of patients, as it had a short duration (i.e., 10–15 min) and was performed by an experienced intensivist (FST), whenever possible. Apart from the CPP intervention, all other relevant physiological variables were kept stable. Also, the same CPP challenge was performed for the following days, always initiating on a CPP around 60–70 mmHg and testing two additional steps. A maximum of 5 days of testing was considered (i.e., either brain oxygenation was normalized and the catheter removed or increased ICP or persistent brain hypoxia would prevent further testing); CPP “responders” were defined as those patients with a relative increase in PbtO2 from baseline values > 20%.
For all patients, demographics, comorbid diseases, reasons for ICU admission as well as ICU length of stay and hospital mortality were collected. The severity of disease scores [i.e., Glasgow Coma Scale on admission, World Federation of Neurological Surgeons (WFNS) score in SAH patients; Marshall (13) and modified Fisher scores (14, 15) for cerebral CT-scan in TBI or SAH patients, respectively; and location and volume of ICH] were collected. The use of different therapies (i.e., mechanical ventilation, sedative, analgesic, vasopressor, inotrope, antiepileptic, barbituric, and/or osmotic drugs), as well as different interventions (i.e., ICP monitoring, hypothermia, hypocapnia, and decompressive craniotomy) was collected. Intracranial hypertension was defined by the observation of at least one ICP value above 20 mmHg for at least 5 min at any time. Brain tissue hypoxia was defined by a PbtO2 below 20 mmHg.
Neurological outcome at hospital discharge was assessed using the Glasgow Outcome Scale (GOS) (15); favorable neurological outcome (FO) was considered as a GOS 4–5, while unfavorable outcome (UO) as GOS 1–3.
The primary outcome of the study was to evaluate which level of CPP corresponds to a PbtO2 ≥ 20 mmHg. Secondary outcomes included: (a) proportion and characteristics of PbtO2 responders; (b) comparison of PbtO2 changes according to the presence of baseline tissue hypoxia; (c) comparison of PbtO2 changes over time; (d) association of PbtO2 changes with NPi, mFV, and/or PI changes; (e) differences in PbtO2 changes during CPP challenges according to neurological outcome.
Data were analyzed using R statistical software version 4.0.3 (R Foundation for Statistical Computing), Prism (GraphPad Software Inc.), and IBM SPSS Statistics for Macintosh 27 (Armonk, NY, USA). Categorical variables were expressed as count (percentage) and continuous variables as mean ± standard deviation (SD) or median (25th−75th percentiles). The Kolmogorov-Smirnov test was used, and histograms and normal-quantile plots were examined to verify the normality of distribution of continuous variables. Differences between groups were assessed using the chi-square test or Fisher's exact test for categorical variables and Student's t-test, or Mann–Whitney U-test for continuous variables, as appropriate. Mixed model procedure with restricted maximum likelihood (REML) estimation and “unstructured” covariance structure was used to examine the differences in PbtO2 changes during CPP challenge over different days of assessment. All tests are two tailed and the statistical significance was set at the 5% level.
Over a total of 162 patients, 109 were excluded (i.e., n = 49, not on vasopressors during the PbtO2 monitoring period; n = 29, refractory intracranial hypertension; n = 31, measurements not performed) and 53 were eventually included into the final analysis. No significant differences were observed between included and excluded patients (Supplementary Table 1), except for a shorter ICU length of stay and higher mortality for the excluded group. Characteristics of the study population are shown in Table 1; the most frequent brain injury was SAH (n = 29, 55%). Hospital mortality occurred in 18 (34%) of patients, while 33 (62%) presented with UO.
Table 1. Characteristics of study population, according to neurological outcome (UO, unfavorable; FO, favorable).
On the first day of assessment, baseline PbtO2 and CPP were 20 (16–21) mmHg and 73 (70–76) mmHg, respectively; brain hypoxia at baseline was observed in 26 (49%) of patients (Tables 1, 2). CPP was progressively increased to 83 (80–86) mmHg and 92 (90–96) mmHg, which resulted into a significant PbtO2 increase [to 22 (19–22) mmHg and 24 (20–24) mmHg, respectively; p < 0.001; Table 2]. During CPP increase, ICP also significantly decreased from baseline values. Median CPP value corresponding to PbtO2 values ≥ 20 mmHg on day 1 was 79 (74–87) mmHg, with 2 (4%) patients who never achieved such target; similar CPP values corresponding to PbtO2 values >20 mmHg were observed in traumatic (n = 38) and non-traumatic brain injury [79 (75–86) mmHg vs. 77 (72–88) mmHg; p = 0.52]. CPP challenges were repeated in 34 patients on day 2, 23 on day 3, 11 on day 4, and 7 on day 5; reasons for not performing CPP challenges the following days were mainly increased ICP (n = 4), the need for CPP > 90 mmHg to keep stable cerebral oxygenation (n = 5), or absence of operator and discontinuation of vasopressor therapy in others. Two patients on day 1 and 1 patient on day 3 never achieved the PbtO2 target. Similar values of CPP corresponding to PbtO2 values ≥ 20 mmHg were observed in the following days [80 (73–87) mmHg on day 2; 79 (72–92) mmHg on day 3; 80 (72–89) mmHg on day 4; 80 (68–92) mmHg on day 5; Figure 1]. No significant complications (i.e., increase in ICP, arrhythmias) during CPP challenge were reported.
Table 2. Changes in study variables on the first day of assessment, according to different steps of mean arterial pressure (MAP) and neurological outcome (UO, unfavorable; FO, favorable).
A total of 25 (47%) were PbtO2 responders during the CPP challenge on day 1. PbtO2 responders had similar characteristics to non-responders, including the presence of TBI, with the exception a lower PbtO2 at baseline (Table 3). Changes in PbtO2 on the first day of assessment were significantly higher over time in responders than in non-responders (Table 4; Figure 2).
Table 3. Characteristics of population according to PbtO2 responder or non-responder to CPP challenge.
Table 4. Changes in study variables on the first day of assessment, according to response to CPP challenge and baseline tissue hypoxia.
Figure 2. PbtO2 changes during the CPP challenge in responders and non-responders (left panel), in baseline normal PbtO2 values or tissue hypoxia (middle panel) or according to the neurological outcome (UO, unfavorable; FO, favorable – right panel).
Among patients with baseline tissue hypoxia on the first day of assessment (Supplementary Table 2), changes in PbtO2 were significantly higher in those with tissue hypoxia when compared to others (Table 4; Figure 2). Similar changes in PbtO2 between days 1, 2, and 3 were observed (Wald chi-square 6.42; p = 0.59; Figure 3); data on day 4 and 5 were not included into this analysis because of the high number of missing challenges.
A total of 19 (56%), 10 (42%), 4 (36%), and 3 (43%) were PbtO2 responders during the CPP challenge on days 2, 3, 4, and 5, respectively. A total of 18 (53%), 12 (50%), 4 (36%), and 3 (43%) had baseline brain tissue hypoxia at baseline on days 2, 3, 4, and 5, respectively.
A total of 123 paired assessment of PbtO2 with mFV and PI changes and 59 of PbtO2 with NPi changes were available over the first 5 days of assessment. No correlation of PbtO2 changes were observed with changes in mFV or PI (correlation index of −0.043 and −0.031, with p-values of 0.57 and 0.68, respectively), while a weak correlation between PbtO2 and NPi changes was observed (correlation index of 0.181, p = 0.017).
Differences between patients with UO and FO are shown in Table 1. Before the CPP challenge, baseline characteristics were similar between groups on day 1. Also, changes in PbtO2 over time on day 1 were not statistically significant between groups (Table 2; Figure 2).
In this study, including a heterogeneous population of brain injured patients, increasing CPP resulted in a significant increase of the brain oxygenation in most of patients. The “optimal” CPP, i.e., the CPP value corresponding to the absence of tissue hypoxia, was higher than in recommended targets (i.e., around 80 mmHg). Half of patients showed a significant increase in PbtO2 during the CPP challenge, in particular, if they had lower PbtO2 values at baseline. The effects of CPP on PbtO2 changes were similar in the following days of assessment. Non-invasive neuromonitoring could not adequately predict PbtO2 changes during CPP increase.
The improvement in PbtO2 values during CPP increase using vasopressors has also been reported in previous studies. Johnston et al. reported a significant increase in brain oxygenation when CPP was increased from 70 to 90 mmHg (8); these changes were also associated with an increase in cerebral blood flow and a decrease in oxygen extraction fraction (OER). However, as CPP challenge resulted in a greater proportional increase in PbtO2 than OER, the authors concluded that this intervention could potentially increase the oxygen gradient between the vascular and tissue compartments. In another study, increasing CPP also resulted into a significant increase in PbtO2 (9). Our study included a larger population of patients, included other diseases than TBI, and tested two different CPP targets above the baseline values. Importantly, this CPP challenge should be performed only in those patients with low PbtO2 values at the baseline, in the absence of intracranial hypertension or hypoxemia. Increasing CPP in patients with normal PbtO2 at baseline would result in less significant oxygen improvement and no theoretical effect on tissue metabolism. In one study, Stocchetti et al. also reported that PbtO2 regularly improved after 22 CPP challenges, in particular when low oxygen values were present at the baseline (16). Baseline PbtO2 below 20 mm Hg was often associated with CPP values within normal ranges. Interestingly, increasing CPP with other drugs than norepinephrine, such as dopamine, might result in less predictable CPP increase and less significant PbtO2 increase (17, 18). Moreover, PbtO2 would change in response to CPP challenge if placed into “at-risk” areas, as in our study, while effects might be minimal if the catheter is inserted into normal-appearing parenchyma (17).
It has been suggested that cerebral hemodynamics after an acute brain injury could be assessed using non-invasive monitoring, such as cerebral blood flow velocities or cerebral autoregulation indices. As such, because PbtO2 is dependent on CPP below the lower limit of autoregulation (i.e., the value of CPP corresponding to the direct dependency of cerebral blood flow from the systemic driving pressure) (19), recommended CPP targets can still result in tissue hypoxia in the absence of elevated ICP, as autoregulation might be impaired or the lower limit shifted toward higher CPP thresholds in brain injured patients (20). Individualized CPP values based on optimal autoregulation status has also been suggested in TBI patients (20); however, targeting “safe” CPP values based on the plateau curve of autoregulation could not correspond to adequate brain oxygenation in some patients (21). In one study (19), PbtO2 was pressure dependent when autoregulation was impaired, while it remained within stable values if autoregulation was intact. However, tissue hypoxia could occur even within normal autoregulation indices (19), thus suggesting that only the presence of PbtO2 monitoring could help to optimize CPP and avoid tissue hypoxia. As such, it is not surprising that changes in PbtO2 during the CPP challenge would not correlate with changes in mFV or PI, two parameters derived from the analysis of cerebral blood flow velocities that are commonly used to estimate cerebral hypoperfusion in the presence of intracranial hypertension (22). In SAH patients, no correlation was observed between PbtO2 measurements and, simultaneously, TCD recording (23). In another study including TBI patients, episode of cerebral hypoxia had all mFV <40 cm/s; however, no correlation was observed between PbtO2 and mFV in the whole cohort (24). Unfortunately, we did not specifically assess cerebral autoregulation in our study and could not provide additional data to the relationship between autoregulatory status and brain oxygenation. Similarly, NPi assessment using automated pupillometry could reflect elevated ICP (25); however, as tissue hypoxia may occur even within normal ICP values, the lack of sufficient correlation between PbtO2 and NPi changes was expected.
This study has several limitations to acknowledge. First, the small sample size and monocentric and retrospective design might introduce significant selection biases and limit the generalizability of our findings, although characteristics of included and excluded patients were similar. Second, although we suggested the need for higher than recommended CPP targets, MAP transducer was zeroed at the atrium level, while some centers would place the zero-reference point next to cerebral anatomical structures, i.e., the foramen of Monro, which would result in 10–15 mmHg lower CPP, depending on the elevation of the head of the bed (usually 15–30°) (26). Third, many patients had baseline ICP, CPP, and PbtO2 within normal values at baseline, which might not entirely justify a daily “CPP challenge.” However, the duration of CPP challenge was short, being supervised by an experienced intensivist, and was clinically relevant, as conducted in a well-designed approach, and informative for daily patient management. Interestingly, in those patients with normal PbtO2 levels at baseline, lower than recommended CPP targets might have been theoretically used, still maintaining adequate oxygen levels. However, lower CPP values would be outside of routine management in brain injured patients and future studies should prospectively evaluate the safety of such approach. Fourth, the procedure was considered as safe (i.e., no complications were recorded); however, we did not assess changes in cerebral metabolism (i.e., glucose or lactate/pyruvate ratio), which might have also been influenced by changes in brain hemodynamics. Fifth, CPP augmentation was not compared with other interventions able to increase PbtO2 in brain injured patients (i.e., increase in FiO2 and arterial hyperoxia) (27). As such, whether increased CPP rather than, as an example, increased FiO2 would result in similar effects on brain oxygenation or would have a different safety profile in brain injured patients remains unknown. Sixth, we only assessed short-terms effects of increasing CPP, while persistently high CPP values might be associated with an increased risk of acute respiratory failure, at least in TBI patients, and would not result in better neurological outcome than standard targets (28). Seventh, we defined tissue hypoxia as a PbtO2 <20 mmHg; ischemic thresholds for PbtO2 have been tested using the association of this value with patients' outcome; a recent study identified a threshold of 19 mmHg which adequately separated patients with unfavorable and favorable neurological outcome after TBI (29). However, the ischemic threshold might vary among different brain disease and within patients and the presence of concomitant metabolism monitoring (i.e., microdialysis) might be helpful to identify ischemic levels of PbtO2 when they would be associated with low cerebral glucose levels and increased lactate/pyruvate ratio. Eighth, the cerebrovascular pathophysiology among the different types of acute brain injury is different; in particular, SAH patients can develop delayed cerebral ischemia between 4 and 15 days after the injury, whereas cerebral hyperemia and intracranial hypertension may be more common in TBI patients. As such, our findings are also relevant to the very early phase after the injury and in the absence of uncontrolled intracranial hypertension. Finally, we provided different descriptive analyses without multivariable assessment, as the number of events was limited; however, multiple statistical comparisons exposes to the inflation of false positive tests within dependent datasets.
In this heterogeneous population of acute brain injured patients, PbtO2 monitoring suggested the need for higher than recommended CPP targets to avoid tissue hypoxia. A higher response in brain oxygenation to the CPP challenge was observed in those patients with low PbtO2 values at baseline. The effects of CPP increase over brain oxygenation are consistent over time.
The raw data supporting the conclusions of this article will be made available by the authors, without undue reservation.
The studies involving human participants were reviewed and approved by Ethical Committee of the Erasme Hospital. Written informed consent for participation was not required for this study in accordance with the national legislation and the institutional requirements.
FST, SS, and MK conceived the study protocol. AQ and FST performed the transcranial Doppler. MK, EG, and LP collected the data. HN, LP, and MK analyzed the data. FST, MK, JC, and OD drafted the present manuscript. SS, EG, LP, and AQ critically revised the manuscript. All authors read and approved the final manuscript.
FST is the Scientific Advisor for Neuroptics Inc. and received lecture fees from Integra LifeSciences.
The remaining authors declare that the research was conducted in the absence of any commercial or financial relationships that could be construed as a potential conflict of interest.
All claims expressed in this article are solely those of the authors and do not necessarily represent those of their affiliated organizations, or those of the publisher, the editors and the reviewers. Any product that may be evaluated in this article, or claim that may be made by its manufacturer, is not guaranteed or endorsed by the publisher.
The Supplementary Material for this article can be found online at: https://www.frontiersin.org/articles/10.3389/fneur.2021.732830/full#supplementary-material
1. Helbok R, Schiefecker AJ, Beer R, Dietmann A, Antunes AP, Sohm F, et al. Early brain injury after aneurysmal subarachnoid hemorrhage: a multimodal neuromonitoring study. Crit Care. (2015) 19:75. doi: 10.1186/s13054-015-0809-9
2. Maldonado JR. Acute brain failure: pathophysiology, diagnosis, management, and sequelae of delirium. Crit Care Clin. (2017) 33:461–519. doi: 10.1016/j.ccc.2017.03.013
3. Carney N, Totten AM, O'Reilly C, Ullman JS, Hawryluk GWJ, Bell MJ, et al. Guidelines for the management of severe traumatic brain injury, fourth edition. Neurosurgery. (2017) 80:6–15. doi: 10.1227/NEU.0000000000001432
4. Le Roux P, Menon DK, Citerio G, Vespa P, Bader MK, Brophy GM, et al. Consensus summary statement of the International multidisciplinary consensus conference on multimodality monitoring in neurocritical care : a statement for healthcare professionals from the neurocritical care society and the European Society of Intensive Care Medicine. Intensive Care Med. (2014) 40:1189–209. doi: 10.1007/s00134-014-3369-6
5. Eriksson EA, Barletta JF, Figueroa BE, Bonnell BW, Vanderkolk WE, McAllen KJ, et al. Cerebral perfusion pressure and intracranial pressure are not surrogates for brain tissue oxygenation in traumatic brain injury. Clin Neurophysiol. (2012) 123:1255–60. doi: 10.1016/j.clinph.2011.08.035
6. Fiore M, Bogossian E, Creteur J, Oddo M, Taccone FS. Role of brain tissue oxygenation (PbtO2) in the management of subarachnoid haemorrhage: a scoping review protocol. BMJ Open. (2020) 10:e035521. doi: 10.1136/bmjopen-2019-035521
7. Kirkman MA, Smith M. Brain oxygenation monitoring. Anesthesiol Clin. (2016) 34:537–56. doi: 10.1016/j.anclin.2016.04.007
8. Johnston AJ, Steiner LA, Coles JP, Chatfield DA, Fryer TD, Smielewski P, et al. Effect of cerebral perfusion pressure augmentation on regional oxygenation and metabolism after head injury. Crit Care Med. (2005) 33:189–95. doi: 10.1097/01.CCM.0000149837.09225.BD
9. Rosenthal G, Hemphill JC, Sorani M, Martin C, Morabito D, Obrist WD, et al. Brain tissue oxygen tension is more indicative of oxygen diffusion than oxygen delivery and metabolism in patients with traumatic brain injury. Crit Care Med. (2008) 36:1917–24. doi: 10.1097/CCM.0b013e3181743d77
10. Connolly ES, Rabinstein AA, Carhuapoma JR, Derdeyn CP, Dion J, Higashida RT, et al. Guidelines for the management of aneurysmal subarachnoid hemorrhage: a guideline for healthcare professionals from the American Heart Association/american Stroke Association. Stroke. (2012) 43:1711–37. doi: 10.1161/STR.0b013e3182587839
11. Morgenstern LB, Hemphill JC, Anderson C, Becker K, Broderick JP, Connolly ES, et al. Guidelines for the management of spontaneous intracerebral hemorrhage: a guideline for healthcare professionals from the American Heart Association/American Stroke Association. Stroke. (2010) 41:2108–29. doi: 10.1161/STR.0b013e3181ec611b
12. Purkayastha S, Sorond F. Transcranial Doppler ultrasound: technique and application. Semin Neurol. (2012) 32:411–20. doi: 10.1055/s-0032-1331812
13. Marshall LF, Marshall SB, Klauber MR, Van Berkum Clark M, Eisenberg H, Jane JA, et al. The diagnosis of head injury requires a classification based on computed axial tomography. J Neurotrauma. (1992) 9 (Suppl. 1):S287–92.
14. Rosen DS, Macdonald RL. Subarachnoid hemorrhage grading scales: a systematic review. Neurocrit Care. (2005) 2:110–8. doi: 10.1385/NCC:2:2:110
15. Jennett B, Bond M. Assessment of outcome after severe brain damage. Lancet Lond Engl. (1975) 1:480–4. doi: 10.1016/S0140-6736(75)92830-5
16. Stocchetti N, Chieregato A, De Marchi M, Croci M, Benti R, Grimoldi N. High cerebral perfusion pressure improves low values of local brain tissue O2 tension (PtiO2) in focal lesions. Acta Neurochir Suppl. (1998) 71:162–5. doi: 10.1007/978-3-7091-6475-4_47
17. Kiening KL, Härtl R, Unterberg AW, Schneider GH, Bardt T, Lanksch WR. Brain tissue pO2-monitoring in comatose patients: implications for therapy. Neurol Res. (1997) 19:233–40. doi: 10.1080/01616412.1997.11740805
18. Steiner LA, Johnston AJ, Czosnyka M, Chatfield DA, Salvador R, Coles JP, et al. Direct comparison of cerebrovascular effects of norepinephrine and dopamine in head-injured patients. Crit Care Med. (2004) 32:1049–54. doi: 10.1097/01.CCM.0000120054.32845.A6
19. Jaeger M, Dengl M, Meixensberger J, Schuhmann MU. Effects of cerebrovascular pressure reactivity-guided optimization of cerebral perfusion pressure on brain tissue oxygenation after traumatic brain injury. Crit Care Med. (2010) 38:1343–7. doi: 10.1097/CCM.0b013e3181d45530
20. Donnelly J, Czosnyka M, Adams H, Robba C, Steiner LA, Cardim D, et al. Individualizing thresholds of cerebral perfusion pressure using estimated limits of autoregulation. Crit Care Med. (2017) 45:1464–71. doi: 10.1097/CCM.0000000000002575
21. Radolovich DK, Czosnyka M, Timofeev I, Lavinio A, Hutchinson P, Gupta A, et al. Reactivity of brain tissue oxygen to change in cerebral perfusion pressure in head injured patients. Neurocrit Care. (2009) 10:274–9. doi: 10.1007/s12028-009-9190-3
22. Robba C, Taccone FS. How I use transcranial Doppler. Crit Care Lond Engl. (2019) 23:420. doi: 10.1186/s13054-019-2700-6
23. Craven CL, Sae-Huang M, Hoskote C, Watkins LD, Reddy U, Toma AK. Relationship between brain tissue oxygen tension and transcranial Doppler ultrasonography. World Neurosurg. (2021) 149:e942–6. doi: 10.1016/j.wneu.2021.01.070
24. Sokoloff C, Williamson D, Serri K, Albert M, Odier C, Charbonney E, et al. Clinical usefulness of transcranial doppler as a screening tool for early cerebral hypoxic episodes in patients with moderate and severe traumatic brain injury. Neurocrit Care. (2020) 32:486–91. doi: 10.1007/s12028-019-00763-y
25. Robba C, Pozzebon S, Moro B, Vincent JL, Creteur J, Taccone FS. Multimodal non-invasive assessment of intracranial hypertension: an observational study. Crit Care Lond Engl. (2020) 24:379. doi: 10.1186/s13054-020-03105-z
26. Reinstrup P, Unnerbäck M, Marklund N, Schalen W, Arrocha JC, Bloomfield EL, et al. Best zero level for external ICP transducer. Acta Neurochir. (2019) 161:635–42. doi: 10.1007/s00701-019-03856-x
27. Dellazizzo L, Demers SP, Charbonney E, Williams V, Serri K, Albert M, et al. Minimal PaO2 threshold after traumatic brain injury and clinical utility of a novel brain oxygenation ratio. J Neurosurg. (2018) 1–9. [Epub ahead of print].
28. Contant CF, Valadka AB, Gopinath SP, Hannay HJ, Robertson CS. Adult respiratory distress syndrome: a complication of induced hypertension after severe head injury. J Neurosurg. (2001) 95:560–8. doi: 10.3171/jns.2001.95.4.0560
Keywords: brain injury, brain oxygenation, optimal perfusion, individualized therapy, traumatic brain injury, intracranial hemorrhage, subarachnoid hemorrhage
Citation: Kovacs M, Peluso L, Njimi H, De Witte O, Gouvêa Bogossian E, Quispe Cornejo A, Creteur J, Schuind S and Taccone FS (2021) Optimal Cerebral Perfusion Pressure Guided by Brain Oxygen Pressure Measurement. Front. Neurol. 12:732830. doi: 10.3389/fneur.2021.732830
Received: 29 June 2021; Accepted: 29 September 2021;
Published: 28 October 2021.
Edited by:
Anders Lewén, Uppsala University, SwedenReviewed by:
Teodor Mikael Svedung Wettervik, Uppsala University, SwedenCopyright © 2021 Kovacs, Peluso, Njimi, De Witte, Gouvêa Bogossian, Quispe Cornejo, Creteur, Schuind and Taccone. This is an open-access article distributed under the terms of the Creative Commons Attribution License (CC BY). The use, distribution or reproduction in other forums is permitted, provided the original author(s) and the copyright owner(s) are credited and that the original publication in this journal is cited, in accordance with accepted academic practice. No use, distribution or reproduction is permitted which does not comply with these terms.
*Correspondence: Lorenzo Peluso, bG9yZW56b3BlbHVzbzgwQGdtYWlsLmNvbQ==
Disclaimer: All claims expressed in this article are solely those of the authors and do not necessarily represent those of their affiliated organizations, or those of the publisher, the editors and the reviewers. Any product that may be evaluated in this article or claim that may be made by its manufacturer is not guaranteed or endorsed by the publisher.
Research integrity at Frontiers
Learn more about the work of our research integrity team to safeguard the quality of each article we publish.