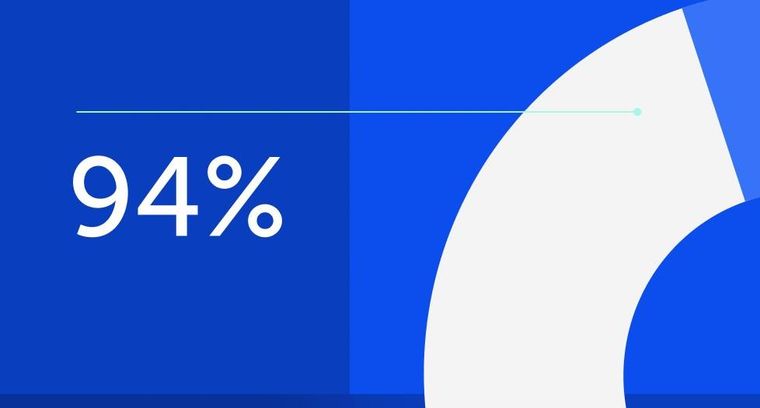
94% of researchers rate our articles as excellent or good
Learn more about the work of our research integrity team to safeguard the quality of each article we publish.
Find out more
PERSPECTIVE article
Front. Neurol., 12 July 2021
Sec. Neurotrauma
Volume 12 - 2021 | https://doi.org/10.3389/fneur.2021.685313
Traumatic brain injury is a rapidly increasing source of morbidity and mortality across the world. As such, the evaluation and management of traumatic brain injuries ranging from mild to severe are under active investigation. Over the last two decades, quantitative pupillometry has been increasingly found to be useful in both the immediate evaluation and ongoing management of traumatic brain injured patients. Given these findings and the portability and ease of use of modern pupillometers, further adoption and deployment of quantitative pupillometers into the preclinical and hospital settings of both resource rich and medically austere environments.
Traumatic brain injury (TBI) represents one of the world's most prevalent causes of morbidity and mortality. With recent estimates placing its worldwide annual incidence at 64–74 million cases per year, it far exceeds rates of other diseases such as tuberculosis (8.8 million/year) and stroke (16.9 million/year) (1). Of these, resource-poor and medically austere areas are the most affected with low-middle income countries being responsible for three times the number of TBI cases as high income countries (1). Within the Department of Defense (DoD) alone, there have been 413,858 TBIs since 2000 (2).
While TBI management in resource-wealthy areas is driven by imaging, intracranial monitoring, and neurological intensive care units (NICU), management of these injuries in both resource-poor areas and in the non/pre-hospital setting has significant limitations when compared to such an expensive paradigm. Mild TBI (mTBI), for example, is often diagnosed and followed through serial examinations, subjective complaints, and tools such as the standard assessment of concussion (SAC) (3) and military acute concussion evaluation 2 (MACE2) (4). Meanwhile, initial management for moderate/severe TBI (mod/sTBI) is often driven by a TBI severity estimate in the form of the patient's Glasgow coma scale (GCS) score despite known limitations such as a lack of long-term prognostic accuracy (5–7). Furthermore, definitive care may require lengthy and costly medical transportation that not only limits accessibility to care, but also makes the decision of who to transport all the more critical.
Quantitative pupillometry (QP) is rapidly emerging as an effective, affordable, easy-to-use, and reliable tool to diagnose and follow patients with TBIs. The purpose of this Perspective is to advocate for the expanded use of QP in the evaluation and management of patients with TBIs. Deployment of these devices and the accurate use and interpretation of the data they provide offers the potential to improve clinical outcomes in TBI patients at both resource-rich settings such as well-developed intensive care units and resource limited areas such as the preclinical and military forward deployed settings.
The pupillary light reflex consists of a complex relationship between photoreceptor activity levels and the pupillary radial and sphincter muscles which is mediated by both cranial nerves II and III. Visible light enters the eye through the iris and strikes the retina where it is converted to an electrical impulse by the rod and cone cells. This information is then refined by bipolar cells and transmitted through the layers of the retina until it leaves the retina as the axons of the ganglion cells which, together, form the optic nerve. The optic nerve proceeds posteriorly through the optic chiasm where the nasal retinal fibers decussate before the information continues posteriorly as the optic tracts. En route to the primary visual cortex, each optic tract gives off a pupillary fiber that travels to the pretectal nucleus of the midbrain. The pretectal nucleus then sends fibers which synapse bilaterally with a preganglionic parasympathetic nucleus called the Edinger-Westphal nucleus. The pretectal nerve then alters the baseline level of activity of the nucleus such that increasing levels of optic tract activity (representing increasing levels of light striking the retina) results in increased activity in the Edinger-Westphal nucleus (8).
The output of the Edinger-Westphal nucleus is what ultimately controls the size of the pupil during the pupillary light reflex. These preganglionic parasympathetic fibers exit the midbrain and travel along bilateral cranial nerve III's to reach the ciliary ganglion. This bilateral output from each Edinger-Westphal nucleus is what is responsible for consensual pupillary constriction after a unilateral light stimulus is applied. After synapsing on the ganglion, postganglionic parasympathetic short ciliary nerve fibers then innervate the iris sphincter muscles and lead to pupillary constriction (8). Of note, further complexity and variability in the pupillary light reflex is introduced by the contraction strength of the antagonistic radial muscle and the impact that a variety of circulating hormones, medications, and/or pathophysiological changes can have on the functionality of the involved neurons and muscles.
Quantitative pupillometers are small, portable devices which utilize a combination of infrared and visible light to capture this variability in the pupillary light reflexes. At the outset of each measurement, the device measures the pupil at rest with infrared light which produces no pupillary response, and then measures the pupil throughout the entire pupillary light reflex to a flash of visible light. These devices measure pupil baseline size, constriction latency, constriction velocity, constriction percentage, minimum size, and dilation velocity (9). These variables can then be combined into the neurological pupil index (NPi), which is a NeuroOptics proprietary algorithm found in their pupillometers designed to provide a simple numerical understanding of pupillary function (0–5 with scores < 3 considered abnormal) (10).
In addition to data collection, modern pupillometers have also been designed to store the data, produce graphical representations over a period of time, and transmit the data to the electronic medical record making tracking a patient's pupillary responses and NPi's over time easy and useful. Furthermore, the devices themselves are portable and easy-to-use making them well-adapted for distribution to both neurological intensive care units and the forwardly deployed locations of military healthcare and global first responders where these injuries occur.
Of the predicted 64–74 million TBIs per year, it is estimated that ~81%, or 52–60 million, are mTBIs (1). Populations at some of the highest levels of risk for mTBIs include youth competitive athletes and military personnel. Unfortunately, mTBI screening among these groups often relies at least in part on self-reported symptoms. This introduces a lack of sensitivity due to inherent survey issues, inter-rater variability, and even a lack of patient honesty with regard to symptoms (11, 12). In youth sports alone, it is estimated there are ~500,000–1.2 million untreated concussions annually (13). The lack of detection of these concussions becomes increasingly more important as both pre-clinical and clinical data continue to aggregate demonstrating that repeated concussions are associated with neuroinflammation, cortical loss, and behavioral changes (14–16).
Here, QP represents a potential mechanism for both diagnosing a mTBI and following patients as they recover. Research in adolescent athletes has demonstrated changes in pupillometry following concussive events. One such study utilizing football helmet accelerometers to accurately define mTBIs found that immediately following mTBIs, QP demonstrated alterations in the pupillary light reflex from baseline testing. Specifically, the affected athletes were noted to have decreased pupillary constriction, a reduction in both constriction and dilation velocity, and a trend toward a decreased NPi (with the lack of significance likely limited by the sample size of 13). Comparatively, QP of the same athletes immediately following a game without a mTBI demonstrated only a statistically significant increase in constriction velocity (17). Taken together, these results demonstrate both a rapid, objective manner in which to diagnose acute mTBIs that appears to not be confounded by the altered sympathetic tone patients may be experiencing at the time.
These alterations in the pupillary light reflex have also been demonstrated in the subacute mTBI period. High school athletes recruited from high school concussion programs within 1 month of injury demonstrated a larger pupillary maximum and minimum diameter and a greater percent constriction when compared to controls (18). Within military personnel who were 2–6 weeks from a diagnosed mTBI, QP demonstrated longer constriction latency and a slower constriction and dilation velocity when compared to patients without a mTBI (19).
While each of these studies demonstrate a different group of changes following a mTBI, the presence of these alterations clearly allows for mTBI detection and monitoring through recovery. Further research will be needed to ensure not only that there is an accurate standard from which to compare, but also to better delineate which aspects of the pupillary light reflex and which timepoints during recovery from a mTBI should be evaluated. Nevertheless, the present data suggests that mTBI detection and monitoring would benefit from increased utilization of QP.
While significantly less ubiquitous than mTBI, mod/sTBI represents no less of a medical challenge. With a median hospitalization cost of $55,267 per case and an estimated 5.5 million cases of mod/sTBI annually, ensuring optimal outcomes for invested resources represents a high value decision point (1, 20). Within the military, this decision is even more important as forward-deployed settings are not research rich and accurate medical triage can become crucial in maximizing medical benefit through ensuring limited waste during medically taxing mass casualty events. Research within the last two decades has demonstrated that QP offers benefit to many aspects of the care for a mod/sTBI patient.
The classical neurological trauma examination includes two parts: the GCS score and the pupillary examination. While a patient's GCS score can be replicated with reasonable consistency, multiple reports have demonstrated that the same cannot be said for manual evaluation of the neurologically injured patient's pupillary reactivity. For example, a recent study demonstrated a 22% error rate amongst nurses in detecting anisocoria when compared to QP (9). Another study comparing nursing staff in a neurological intensive care unit to QP, found that the nursing staff had a 50% error rate in correctly diagnosing anisocoria and a 20% error rate in determining pupillary reactivity. In patients with pupils <2 mm in diameter, as may be the case following drug intoxication or medication administration for intubation and stabilization, the error rate increased to 39% (21). Despite this increase in nursing/provider error rate, multiple studies have demonstrated that QP is still able to detect the presence of pupillary constriction in spite of multiple medications including paralytics, opioids, and benzodiazepines (22). In these situations, QP removes this potential for error and instead provides a rapid and reliable physical examination finding.
Such speed and accuracy becomes increasingly important when discussing surgical decision making for the mod/sTBI patient, especially for those worse off. While the GCS's ease of use contributes to its wide use in trauma evaluations, limitations regarding its ability to prognosticate, and therefore drive treatment decisions, have been raised (23, 24). An increasing number of studies have demonstrated the prognostic importance of pupillary reactivity in delineating patients with a GCS score of three (5–7). In these studies specifically, stratification of patients with a GCS of three by pupillary light examination resulted in an identifiable difference in outcome. Patients who had at least one reactive pupil had a mortality rate of 40–50% compared to the 100% mortality rate of those who had bilaterally fixed and dilated pupils (5, 7). Indeed, the addition of the pupillary examination to the GCS score has been found to be significantly more predictive of mortality than GCS alone (6). This utility for QP, as an intervention adjudicator, was recently evaluated in a pilot study of neurotrauma patients. The study demonstrated that all patients who underwent monitor placement in accordance with the 4th edition Brain Trauma Foundation guidelines but had an NPi of >3 had the monitor removed in <24 h (25). Further study will be required, but the ability to easily obtain such highly accurate pupillary information could help identify and expedite those who can benefit from neurosurgical intervention.
QP has also demonstrated utility in the management of mod/sTBI patients within the critical care unit. The management of the mod/sTBI patient is often dictated by frequent neurological evaluations and intermittent head CTs in response to neurological changes. QP has been demonstrated to detect episodes of increased intracranial pressure, specifically uncal herniation, prior to the onset of clinical symptoms (26, 27). While not perfect due to findings such as the Kernohan's notch phenomenon, the abnormalities detected by QP are also predictive of the sidedness of the lesion (26, 28). In addition to accelerating detection of neurological decompensation, QP has also been utilized to help guide the use of hypertonic therapy. Two studies recently demonstrated that abnormal NPi's in patients with increased intracranial pressure could be reduced or normalized with hypertonic therapy thereby providing a non-invasive way to monitor effect of hypertonics (29, 30).
TBI represents a rapidly increasing pathology on a global scale. As both mTBIs and mod/sTBIs continue to increase in prevalence and we understand more regarding the implications of these injuries, identification and management in both the immediate and post-injury setting will become more important. Over the last two decades numerous studies have demonstrated the potential for QP to assist in the detection and management of TBI across its spectrum of severity and the longitude of its course. This utility coupled with the ease of QP and the durability of today's pupillometers make QP a resource that is easy to expand across countries of varying income levels and practice areas of varying levels of austerity.
The original contributions presented in the study are included in the article/supplementary material, further inquiries can be directed to the corresponding author/s.
JB, MS, and BD developed the idea for and organized the manuscript. JB, MS, MM, GM, BC, and BD wrote, revised, and critically evaluated the manuscript. All authors contributed to the article and approved the submitted version.
The views expressed in this article are those of the author and do not reflect the official policy of the Department of Army/Navy/Air Force, Department of Defense, or U.S. Government.
The authors declare that the research was conducted in the absence of any commercial or financial relationships that could be construed as a potential conflict of interest.
1. Dewan MC, Rattani B, Gupta S, Baticulon RE, Hung YC, Punchak M, et al. Estimating the global incidence of traumatic brain injury. J Neurosurg. (2019) 130:1080–97. doi: 10.3171/2017.10.JNS17352
3. McCrea M. Standardized mental status testing on the sideline after sport-related concussion. J Athletic Train. (2001) 36:274–9.
4. Khokhar B, Jorgensen-Wagers K, Marion D, Kiser S. Military acute concussion evaluation: a report on clinical usability, utility, and user's perceived confidence. J Neurotrauma. (2021) 38:210–17. doi: 10.1089/neu.2020.7176
5. Tien HC, Cunha JRF, Wu SN, Chughtai T, Tremblay LN, Brenneman FD, et al. Do trauma patients with a glasgow coma scale score of 3 and bilateral fixed and dilated pupils have any chance of survival? J Trauma. (2006) 60:274–8. doi: 10.1097/01.ta.0000197177.13379.f4
6. Hoffman M, Lefering R, Rueger JM, Kolb JP, Izbicki JR, Ruecker AH, et al. Pupil evaluation in addition to glasgow coma scale components in prediction of traumatic brain injury and mortality. Br J Surg. (2012) 99:122–30. doi: 10.1002/bjs.7707
7. Nijboer JMM, Naalt JD, Duis HJ. Patients beyond salvation? Various categories of trauma patients with a minimal glasgow coma score. Injury. (2010) 41:52–7. doi: 10.1016/j.injury.2009.05.030
8. Larson MD, Behrends M. Portable infrared pupillometry: a review. Anesth Analg. (2015) 120:1242–53. doi: 10.1213/ANE.0000000000000314
9. Taylor WR, Chen JW, Meltzer H, Gennarelli TA, Kelbch C, Knowlton S, et al. Quantitative pupillometry, a new technology: normative data and preliminary observations in patients with acute head injury. J Neurosurg. (2003) 98:205–13. doi: 10.3171/jns.2003.98.1.0205
10. Chen JW, Gombart ZJ, Rogers S, Gardiner SK, Cecil S, Bullock RM. Pupillary reactivity as an early indicator of increased intracranial pressure: the introduction of the neurological pupil index. Surg Neurol Int. (2011) 2:82–8. doi: 10.4103/2152-7806.82248
11. Kroshus E, Garnett B, Hawrilenko M, Baugh CM, Calzo JP. Concussion under-reporting and pressure from coaches, teammates, fans, and parents. Soc Sci Med. (2015) 134:66–75. doi: 10.1016/j.socscimed.2015.04.011
12. Register-Mihalik JK, Cameron KL, Kay MC, Kerr ZY, Peck KY, Houston MN, et al. Determinants of intention to disclose concussion symptoms in a population of U.S. Military Cadets. J Sci Med Sport. (2019) 22:509–15. doi: 10.1016/j.jsams.2018.11.003
13. Bryan MA, Rowhani-Rahbar A, Comstock RD, Rivara F. Sports- and recreation-related concussions in US youth. Pediatrics. (2016) 138:e20154635. doi: 10.1542/peds.2015-4635
14. Shultz SR, Bao F, Omana V, Chiu C, Brown A, Cain DP. Repeated mild lateral fluid percussion brain injury in the rat causes cumulative long-term behavioral impairments, neuroinflammation, and cortical loss in an animal model of repeated concussion. J Neurotrauma. (2012) 29:281–94. doi: 10.1089/neu.2011.2123
15. Pearce AJ, Rist B, Fraser CL, Cohen A, Maller JJ. Neurophysiological and cognitive impairment following repeated sports concussion injuries in retired professional rugby league players. Brain Injury. (2018) 32:498–505. doi: 10.1080/02699052.2018.1430376
16. Oyegbile TO, Dougherty A, Tanveer S, Zecavati N, Delasobera BE. High sleep disturbance and longer concussion duration in repeat concussions. Behav Sleep Med. (2020) 18:241–8. doi: 10.1080/15402002.2019.1578223
17. Joseph JR, Swallow JS, Willsey K, Almeida AA, Lorincz MT, Fraumann RK, et al. Pupillary changes after clinically asymptomatic high-acceleration head impacts in high school football athletes. J Neurosurg. (2019) 133:1886–91. doi: 10.3171/2019.7.JNS191272
18. Master CL, Podolak OE, Ciuffreda KJ, Metzger KB, Joshi NR, McDonald CC, et al. Utility of pupillary light reflex metrics as a physiologic biomarker for adolescent sport-related concussion. JAMA Ophthalamol. (2020) 138:1135–41. doi: 10.1001/jamaophthalmol.2020.3466
19. Capó-Aponte JE, Urosevich TG, Walsh DV, Temme LA, Tarbett AK. Pupillary light reflex as an objective biomarker for early identification of blast-induced mTBI. J Spine. (2013) 4:s4–s9. doi: 10.4172/2165-7939.S4-004
20. Dijck JTJM, Dijkman MD, Ophuis RH, Ruiter GCWd, Peul WC, Polinder S. In-hospital costs after severe traumatic brain injury: a systematic review and quality assessment. PLoS ONE. (2019) 14:e0216743. doi: 10.1371/journal.pone.0216743
21. Couret D, Boumaza D, Grisotto C, Triglia T, Pellegrini L, Ocquidant P, et al. Reliability of standard pupillometry practice in neurocritical care: an observational, double-blinded study. Critical Care. (2016) 20:99–107. doi: 10.1186/s13054-016-1239-z
22. Bower MM, Sweidan AJ, Xu JC, Stern-Neze S, Yu W, Groysman LI. Quantitative pupillometry in the intensive care unit. J Intens Care Med. (2019) 36:383–91. doi: 10.1177/0885066619881124
23. Chaudhuri K, Malham GM, Rosenfeld JV. Survival of trauma patients with coma and bilateral fixed dilated pupils. Injury. (2009) 40:28–32. doi: 10.1016/j.injury.2008.09.004
24. Mauritz W, Leitgeb J, Wilbacher I, Majdan M, Janciak I, Brazinova A, et al. Outcome of brain trauma patients who have a glasgow coma scale score of 3 and bilateral fixed and dilated pupils in the field. Eur J Emerg Med. (2009) 16:153–8. doi: 10.1097/MEJ.0b013e32832a0864
25. El Ahmadieh TY, Bedros N, Stutzman SE, Nyancho D, Venkatachalam AM, MacAllister M, et al. Automated pupillometry as a triage and assessment tool in patients with traumatic brain injury. World Neurosurg. (2020) 145:e163–9. doi: 10.1016/j.wneu.2020.09.152
26. Fischer VE, Boulter JH, Bell RS, Ikeda DS. Paradoxical contralateral herniation detected by pupillometry in acute syndrome of the trephined. Military Med. (2020) 185:532–6. doi: 10.1093/milmed/usz409
27. Papangelou A, Zink EK, Chang WW, Frattalone A, Gergen D, Gottschalk A, et al. Automated pupillometry and detection of clinical transtentorial brain herniation: a case series. Military Med. (2018) 183:e113–21. doi: 10.1093/milmed/usx018
28. Chesnut RM, Gautille T, Blunt BA, Klauber MR, Marshall LE. The localizing value of asymmetry in pupillary size in severe head injury: relation to lesion type and location. Neurosurgery. (1994) 34:840–5. doi: 10.1097/00006123-199405000-00008
29. Ong C, Hutch M, Barra M, Kim A, Zafar S, Smirnakis S. Efects of osmotic therapy on pupil reactivity: quantifcation using pupillometry in critically ill neurologic patients. Neurocrit Care. (2019) 30:307–15. doi: 10.1007/s12028-018-0620-y
Keywords: quantitative pupillometry, automated infrared pupillometry, pupillary examination, traumatic brain injury, coma, neurocritical care
Citation: Boulter JH, Shields MM, Meister MR, Murtha G, Curry BP and Dengler BA (2021) The Expanding Role of Quantitative Pupillometry in the Evaluation and Management of Traumatic Brain Injury. Front. Neurol. 12:685313. doi: 10.3389/fneur.2021.685313
Received: 24 March 2021; Accepted: 18 June 2021;
Published: 12 July 2021.
Edited by:
Elham Rostami, Uppsala University Hospital, SwedenReviewed by:
Anders Lewén, Uppsala University, SwedenCopyright © 2021 Boulter, Shields, Meister, Murtha, Curry and Dengler. This is an open-access article distributed under the terms of the Creative Commons Attribution License (CC BY). The use, distribution or reproduction in other forums is permitted, provided the original author(s) and the copyright owner(s) are credited and that the original publication in this journal is cited, in accordance with accepted academic practice. No use, distribution or reproduction is permitted which does not comply with these terms.
*Correspondence: Jason H. Boulter, amFzb24uaC5ib3VsdGVyLm1pbEBtYWlsLm1pbA==
Disclaimer: All claims expressed in this article are solely those of the authors and do not necessarily represent those of their affiliated organizations, or those of the publisher, the editors and the reviewers. Any product that may be evaluated in this article or claim that may be made by its manufacturer is not guaranteed or endorsed by the publisher.
Research integrity at Frontiers
Learn more about the work of our research integrity team to safeguard the quality of each article we publish.