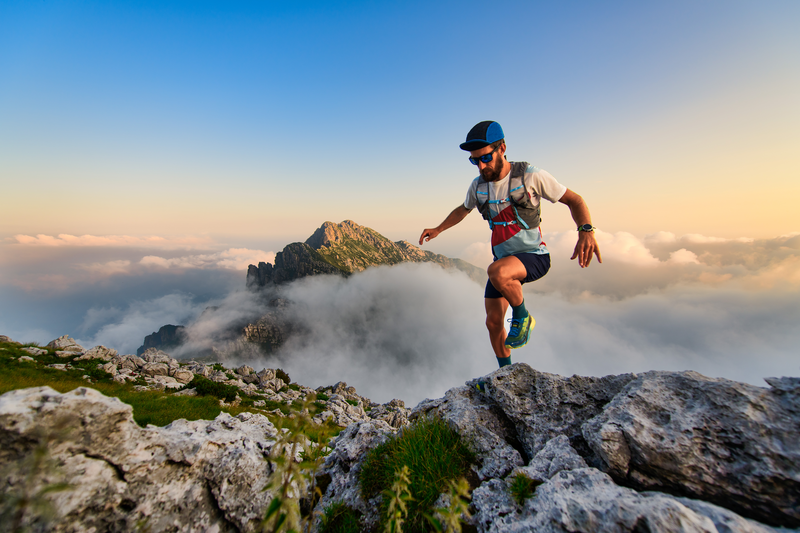
94% of researchers rate our articles as excellent or good
Learn more about the work of our research integrity team to safeguard the quality of each article we publish.
Find out more
ORIGINAL RESEARCH article
Front. Neurol. , 11 May 2021
Sec. Epilepsy
Volume 12 - 2021 | https://doi.org/10.3389/fneur.2021.669517
This article is part of the Research Topic Epilepsy Mortality: Leading Causes of Death, Co-morbidities, Cardiovascular Risk and Prevention View all 11 articles
Rationale: Currently, there is some ambiguity over the role of postictal generalized electro-encephalographic suppression (PGES) as a biomarker in sudden unexpected death in epilepsy (SUDEP). Visual analysis of PGES, known to be subjective, may account for this. In this study, we set out to perform an analysis of PGES presence and duration using a validated signal processing tool, specifically to examine the association between PGES and seizure features previously reported to be associated with visually analyzed PGES.
Methods: This is a prospective, multicenter epilepsy monitoring study of autonomic and breathing biomarkers of SUDEP in adult patients with intractable epilepsy. We studied videoelectroencephalogram (vEEG) recordings of generalized convulsive seizures (GCS) in a cohort of patients in whom respiratory and vEEG recording were carried out during the evaluation in the epilepsy monitoring unit. A validated automated EEG suppression detection tool was used to determine presence and duration of PGES.
Results: We studied 148 GCS in 87 patients. PGES occurred in 106/148 (71.6%) seizures in 70/87 (80.5%) of patients. PGES mean duration was 38.7 ± 23.7 (37; 1–169) seconds. Presence of tonic phase during GCS, including decerebration, decortication and hemi-decerebration, were 8.29 (CI 2.6–26.39, p = 0.0003), 7.17 (CI 1.29–39.76, p = 0.02), and 4.77 (CI 1.25–18.20, p = 0.02) times more likely to have PGES, respectively. In addition, presence of decerebration (p = 0.004) and decortication (p = 0.02), older age (p = 0.009), and hypoxemia duration (p = 0.03) were associated with longer PGES durations.
Conclusions: In this study, we confirmed observations made with visual analysis, that presence of tonic phase during GCS, longer hypoxemia, and older age are reliably associated with PGES. We found that of the different types of tonic phase posturing, decerebration has the strongest association with PGES, followed by decortication, followed by hemi-decerebration. This suggests that these factors are likely indicative of seizure severity and may or may not be associated with SUDEP. An automated signal processing tool enables objective metrics, and may resolve apparent ambiguities in the role of PGES in SUDEP and seizure severity studies.
Sudden unexpected death in epilepsy (SUDEP) incidence ranges from 1.1 to 5.9 per 1,000 patient-years in epilepsy clinic populations to 6.3–9.3 per 1,000 patients with intractable epilepsy, posing a significant public health problem (1). Risk factors that increase risk include ≥3 generalized convulsive seizure (GCS) per year, younger age of onset of epilepsy and longer duration of epilepsy (2–4). In a case-control study (5), duration of postictal generalized electroencephalographic suppression (PGES) was significantly longer in patients who later died of SUDEP; duration >50 s was significantly associated with a higher risk of SUDEP (5). All patients in the Mortality in Epilepsy Monitoring Units Study (MORTEMUS) had PGES in the agonal seizure (6). However, a case control study of patients who later died of SUDEP did not find PGES predictive (7). Although several observations have been made on factors associated with PGES, seizure severity, and potential SUDEP risk, almost all of have been made with visual analysis of PGES, which we now know to be subjective and perhaps unreliable. This may explain apparent ambiguity over the role of PGES in SUDEP (8–10). In this study, we set out to perform an analysis of PGES presence and duration using a validated signal processing tool (11), specifically to examine the association between PGES and seizure features previously reported to be associated with visually analyzed PGES.
All patients were prospectively consented participants in the National Institute of Neurological Disorders and Stroke (NINDS) Center for SUDEP Research's (CSR) Autonomic and Imaging Biomarkers of SUDEP multicenter project (U01-NS090407) and its preliminary phase, the Prevention and Risk Identification of SUDEP Mortality (PRISM) project (P20NS076965). We studied video-electroencephalogram (vEEG) recordings of GCS in a cohort of patients who underwent long-term monitoring in the epilepsy monitoring unit (EMU). Generalized convulsive seizures (GCS) included generalized tonic-clonic seizures, focal to bilateral tonic-clonic seizures, and focal-onset motor bilateral clonic seizures (12).
The cohort included a total of 87 patients who had 148 GCS, in whom inductance plethysmography (abdominal and thoracic belts) and vEEG recording were carried out during the evaluation in EMU. EEGs were recorded on Nihon Kohden EEG acquisition software with a 1,000 Hz sampling rate using conventional bipolar and common average referenced 10–20 montages. Chest and abdominal excursions were recorded using inductance plethysmography (Ambu, Ballerup, Denmark). Peripheral capillary oxygen saturation (SpO2) and heart rate (HR) were monitored using pulse oximetry (NellcorOxiMax N-600x, Covidien, Minneapolis, MN, USA). The cardiopulmonary data were time-synchronized with the EEG data.
A validated automated EEG suppression detection tool (11) was used to determine presence and duration of PGES and supplemented by two-experienced clinical epileptologists (SL and NL) who reviewed the EEG records with differences resolved by consensus when the tool gave no solution.
The following data were collected and analyzed: Age on admission and at epilepsy onset, gender, body mass index (BMI), duration of epilepsy, previous epilepsy surgery and number of GCS in the preceding 12 months (classified as ≥3 and <3), seizure semiological presentation and duration, body position at seizure end before nursing intervention (supine, prone, lateral, sitting, or undetermined), state prior to seizure onset (awake/sleep and sleep state [N1, N2, N3, and R]).
Tonic phase was defined as tonicity in both arms, further classified into decerebration (both upper limbs straight symmetric), decortication (both upper limbs flexed symmetrically) or hemi-decerebration (tonic extension of one arm with flexion of contralateral arm without progression to decortication or decerebration). Postictal generalized electroencephalographic suppression (PGES), postictal burst suppression and EEG recovery in the post-convulsive period were identified. The EEG signal-processing algorithm was trained to recognize PGES as in previous studies (5, 13): an immediate postictal (within 30 s after GCS) generalized absence of electroencephalographic activity >10 μV in amplitude, lasting at minimum 1 s. Duration of PGES referred to the time interval from PGES onset to the appearance of burst suppression or continuous slowing. Burst Suppression (BS) was defined as generalized, intermittent slowing during the post-convulsive period, alternating with PGES. Combined PGES and burst suppression made up the EEG recovery duration.
Localization of the putative epileptogenic zone was defined based on clinical history, seizure semiology, neuroimaging, and EEG. High-resolution magnetic resonance imaging (MRI) or computed tomography (CT) results were collected (Table 1). The number of antiepileptic drugs (AED) on admission and changes of AED before the recorded GCS (tapering, withdrawal, increase or no change) were recorded. Early nursing intervention was defined as oxygen administration or oral suctioning applied during the seizure or within 5 s of seizure termination. Respiratory data included peripheral arterial oxygen saturation (SpO2) at preictal baseline, GCS onset, SpO2 nadir, duration of hypoxemia (<90%, from GCS onset to 3 min postictal), and presence of central apnea (pre-GCS or post-GCS) were collected using respiratory inductance plethysmography and finger pulse oximetry. Central apnea was defined as ≥1 missed breaths without any other explanation (i.e., speech, movement, or intervention). In some cases whose respiratory data were missing, review of the video to assess respiratory effort helped in differentiating obstructive from central apnea.
Descriptive statistics were provided for seizure-specific demographic and clinical variables (Table 1). Generalized estimating equation (GEE) method was used to model the presence of PGES or PGES duration on different demographic and clinical variables. Variables with a p < 0.1 were employed in the multivariate models. For the binary variable presence of PGES, a binary distribution and a logit link were specified in GEE and odds ratios, 95% confidence intervals, and p-values were reported (Table 3). For the continuous variable PGES duration, covariate coefficient estimates, standard error, and corresponding p-values from GEE method were provided (Table 4). P < 0.05 in the final models were considered significant. All analyses were performed in SAS 9.4 (Cary, NC).
Data from 148 GCS in 87 patients were collected. These patients were previously reported in a paper studying breathing changes as biomarkers of SUDEP (13). Details of seizure-specific demographics, clinical characteristics and MRI neuroimaging details are presented in Table 1. Seizure-specific electroclinical seizure features and cardiorespiratory data are shown in Table 2.
Mean age on admission was 37.4 ± 14.1 (34; 18–77) years and at epilepsy onset was 19.7 ± 16.06 (16–67) years. Epilepsy duration was 17.5 ± 12.2 (15; 0.08–45) years, with 65% more than 10 years. Mean body mass index was 28.7 ± 6.6 kg/m2 (28.1; 15.1–45.8 kg/m2). Twenty-six patients had <3 GCS in the last year and 52 had ≥3. Frequency of GCS was unknown in nine patients. Sixteen (10.8%) had previous epilepsy surgery. Ninety (60.8%) of the seizures were recorded from 50 patients who had negative neuroimaging (details seen in Table 1).
Seizures were of temporal onset in 37(25%), generalized in 28(18.9%), frontal in 23(15.5%), multifocal in 23(15.5%), lateralized but not localized in 20 (13.5%), bitemporal in 12(8.1%), occipital in one (0.7%), and parietal in one (0.7%). Seizure onset could not be localized or lateralized in the remaining three seizures. Seizure onset was left in 49 (33.1%), right in 33 (22.3%), bilateral in 35 (23.6%), and generalized in 28 (18.9%). The remaining three were undetermined. At the time of the recorded seizure, patients were on three or more AEDs in 23% seizures, on two in 50.7%, on one in 25.7%, and 0.7% on no AEDs. Oral suctioning was used in 62/148 (41.89%) seizures and oxygen administration on 84/148 (56.76%).
Tonic semiology was seen in 108/148 (72.9%) seizures, with decerebration in 66/148 (44.6%), decortication in 29/148 (19.6%), and hemi-decerebration in 13/148 (8.8%) (Table 2) The mean duration of tonic phase was 8.7 (8.50, 1–23) seconds (s). The mean duration of the clonic phase was 34.9 (32–110) seconds and of the entire GCS, 49.22 (48, 5–126) seconds, Table 2.
Seventy-two seizures occurred during wakefulness, 73 during sleep, and the remaining three (in one patient) during postictal stupor due to a seizure cluster. Out of 72 seizures that occurred during wakefulness, 53 had PGES. Out of 73 seizures that occurred during sleep, 52 had PGES. The duration of PGES in seizures arising from awake state was 41.52 (2–169) seconds, and 35.83 (1–119) seconds when asleep. Supine posture was seen after 98/148 (66%) seizures with mean PGES duration of 38.89 (2–169). Lateral posture was seen after 41/148 (28%) seizures with mean PGES duration of 31.25 (23–36) seconds. Prone posture was seen in 4/148 (28%) with mean PGES duration of 38.00 (28–42) seconds. Sitting posture was grouped with supine due to the small number (one patient), and undetermined was excluded from analysis (Table 2).
Mean baseline SpO2 was 95.46 ± 2.35 (96; 89–100)%. The SpO2 nadir mean was 59.64 ± 14.10 (60; 22–87)%. In 33% (49/148) seizures, central apnea occurred before GCS (ictal central apnea, ICA) and in 21% (31/148), apnea occurred postictally (post-convulsive central apnea, PCCA) (Table 2).
PGES occurred in 106/148 (71.6%) seizures and 70/87 (80.5%) patients (57 female). Mean duration was 38.7 ± 23.7 (37; 1–169) seconds (s). PGES duration ≥50 s occurred in 18% (19/106). EEG recovery duration was 83.4 + 119.2 (54; 1–1,091) seconds. In univariate analysis, we found that older age was associated with longer PGES duration (p = 0.03) and higher BMI was associated with the presence of PGES (p = 0.04). Gender, age at epilepsy onset, epilepsy duration, previous epilepsy surgery, and the number of GCS within 12 months prior to admission were not associated with PGES. Epileptogenic zone and imaging abnormalities were not associated with occurrence or duration of PGES. During EMU monitoring, most patients had changes in AEDs (tapering: 46.6%, withdrawal: 47.3%).The number of AEDs at admission or changes before recorded GCS were not correlated with PGES presence or duration. Similarly, presence or duration of PGES were not associated with early nursing intervention (oxygen administration or oral suctioning).
In univariate analysis, presence of decerebration (p < 0.01), decortication (p = 0.01), and hemidecerebration (p < 0.0001) during tonic phase were all associated with PGES presence. In addition, decerebration was associated with longer PGES duration (p = 0.009). Duration of GCS, tonic or clonic phase were not associated with PGES incidence (p = 0.39; p = 0.54; p = 0.26, respectively) or PGES duration (p = 0.46; p = 0.37; p = 0.35). Sleep (p = 0.1) and lateral posture (p = 0.04) were inversely correlated to duration of PGES. Severe hypoxemia (SpO2 <75%) was associated with PGES presence (p = 0.007) and duration (p = 0.003); hypoxemia duration in turn was associated with PGES duration (p = 0.03). For central apnea, there was no association with occurrence of ICA or PCCA and PGES.
In multivariate analysis, presence of decerebration, decortication and hemi-decerebration during the tonic phase, were 8.29 (CI 2.6–26.39, p < 0.001), 7.17 (CI 1.29–39.76, p = 0.02), and 4.77 (CI 1.25–18.20, p = 0.02) times more likely to have PGES, respectively, compared to absence of tonic phase (Table 3). In addition, decerebration presence (p = 0.004), decortication presence (p = 0.02), older age (p = 0.009), and hypoxemia duration (p = 0.03) were associated with longer PGES durations. Sleep state (as opposed to awake) was associated with shorter PGES duration (p = 0.001; Tables 3, 4).
Table 3. Multivariate analysis of electroclinical seizure features and cardiorespiratory data with PGES presence.
Table 4. Multivariate analysis of electroclinical seizure features and cardiorespiratory data with PGES duration.
We confirmed, using an objective method for analysis of PGES, that several variables indicated by visual analysis were indeed associated with this phenomenon. PGES is a common phenomenon following GCS, with an incidence of 72%, and has an average duration of 38.7 s. PGES occurrence is associated with presence of a tonic phase during GCS and presence of severe hypoxemia. In addition, longer durations of tonic phase and hypoxemia, older age and postictal supine and sitting position (compared to lateral) are associated with longer PGES duration.
Reports of PGES incidences are variable, ranging from 29 to 74% after GCS (5, 7–10, 14–17). Variability may be due to observer subjectivity, heterogeneous patient populations, and different criteria for diagnosis (7, 18). Differences in visual analysis may be significant; we now know this to be subjective and perhaps unreliable. Our results (72%) indicate PGES to be reliably common. Average PGES duration in our study was 38.7 s. This is roughly concordant with previous studies where PGES duration ranged from 33 to 53 s (8, 10, 17), with a median of 28 s (8). Only 18% of GCS had PGES duration longer than >50 s, suggesting prolonged obtundation is usually infrequent. Prolonged PGES has been suggested as a SUDEP risk factor (5) and associated with more severe postictal coma (17), although other studies have not confirmed this relationship (7, 8). One study found that patients who died of SUDEP had significantly shorter PGES duration compared to living controls (19). In our prospective cohort, two near SUDEP cases occurred (13), with PGES lasting 169 and 75 s, respectively, although no conclusions can be drawn from this observation alone. Analysis of the overall CSR study (of which this report is a part) is currently under way.
We found that presence of tonic phase is associated with both appearance and longer duration of PGES confirming studies based on visual analysis on tonic phase presence (9, 10, 16, 17, 20) and tonic phase duration (9). Different types of tonic phase posturing had varying impact, such that decerebration had the strongest association, followed by decortication, followed by hemi-decerebration. We found that lateral posture (compared to supine) at seizure end is inversely correlated with PGES duration, suggesting briefer obtundation. Although SUDEP cases are more likely to be found in a prone position (21, 22), we could not assess possible associations due to the limited number of prone individuals [4/148 (2.7%)] in our cohort. Sleep state at seizure onset was inversely associated with PGES duration. This is in contrast to other studies that have noted direct association between sleep and increased PGES (8, 10, 23), although others have not (19, 20, 24). Deep sleep before seizure onset, including REM (R) and N3 sleep only occurred in one patient each, and hence the relationship between sleep state and PGES is unresolved here.
In our study, we found that older age at admission was correlated with longer PGES. This is consistent with previous studies (25, 26), but not with others (8–10). We did not find any association between temporal lobe seizure onsets and occurrence of PGES, concordant with some studies (8, 10), but not with others (7, 17) Consistent with all previous studies, we found no association between PGES and GCS frequency, total duration of GCS, gender, epilepsy duration or presence of MRI abnormalities (8–10, 15, 24, 27). AED reduction has been said to increase risk of PGES five-fold (8). Our results suggest that AED reduction did not influence PGES, consistent with a previous study (16), although this lack or relationship may reflect a very small number who did not have medications decreased. Early periictal nursing intervention (14) and O2 administration (10) are reportedly been associated with reduced PGES duration. Other studies found intervention, including early O2 mask administration to have no effect (19, 20, 28), consistent with our current findings. However, hypoxemia duration was associated with PGES duration, reflecting the finding that O2 administration was local EMU protocol driven (regardless of hypoxemia extent) rather than governed by the extent of hypoxemia encountered. This interpretation is in line with ictally triggered severe hypoxemia and hypercapnia previously observed with PGES (14, 15, 29, 30).
One of the main limitations of our study is that the results are largely confirmatory of prior results achieved by visual analysis, and lacks of novel findings. However, the reproducibility of the results may be an indicator that the automated PGES tool is reliable for research studies in order to eliminate the inter-rater variability in PGES assessment in a time-sparing manner.
In this study, with a custom signal-processing tool for PGES analysis, we confirmed observations made with visual analysis, that presence of a tonic phase during GCS, longer hypoxemia, older age and postictal supine or sitting position (compared to lateral) are reliably associated with PGES. The findings suggest that these factors are likely indicative of seizure severity and may or may not be associated with SUDEP. We found that of the different types of tonic phase posturing, decerebration has the strongest association with PGES, followed by decortication, followed by hemi-decerebration. Part of the variability in the literature could result from heterogeneous patient populations, different research methodologies, anti-epileptic medications, or seizure frequency. However, use of visual analysis of PGES detection and quantification, likely plays a part. An automated signal-processing tool enables objective metrics, and may resolve apparent ambiguities in the role of PGES in SUDEP and seizure severity studies.
The raw data supporting the conclusions of this article will be made available by the authors, without undue reservation.
The studies involving human participants were reviewed and approved by the studies involving human participants were reviewed and approved by Case Western Reserve University and University Hospitals, as part of NIH/NINDS Center for SUDEP Research study (U01 NS090405, U01 NS090406, and U01 NS090407). Written informed consent to participate in this study was provided by the participants' legal guardian/next of kin. The patients/participants provided their written informed consent to participate in this study.
XZ and LV were major role in data acquisition, data analysis and interpretation, and drafted manuscript for intellectual content. NL designed and conceptualized the study, interpretation of the data, and drafted manuscript for intellectual content. SL designed and conceptualized the study, and revised the manuscript for intellectual content. RS, DF, MN, BG, SS, RH, BD, LB, OD, GR, and G-QZ revised the manuscript for intellectual content. JoH, JaH, MR, NH, CS, and LA were involved in the data acquisition and management. LZ was involved in the statistical analysis. All authors contributed to the article and approved the submitted version.
This study was funded by the Center for SUDEP Research: NIH/NINDS U01-NS090405, U01 NS090406, and U01 NS090407.
OD receives grant support from NINDS, NIMH, MURI, CDC, and NSF. He has equity and/or compensation from the following companies: Tilray, Receptor Life Sciences, Qstate Biosciences, Tevard Biosciences, Script Biosciences, Regel Biosciences, Empatica, Papa & Barkley, Rettco, Silver Spike, and California Cannabis Enterprises (CCE).
The remaining authors declare that the research was conducted in the absence of any commercial or financial relationships that could be construed as a potential conflict of interest.
1. Tomson T, Nashef L, Ryvlin P. Sudden unexpected death in epilepsy: current knowledge and future directions. Lancet Neurol. (2008) 7:1021–31. doi: 10.1016/S1474-4422(08)70202-3
2. Hesdorffer DC, Tomson T, Benn E, Sander JW, Nilsson L, Langan Y, et al. Do antiepileptic drugs or generalized tonic-clonic seizure frequency increase SUDEP risk? A combined analysis. Epilepsia. (2012) 53:249–52. doi: 10.1111/j.1528-1167.2011.03354.x
3. Holst AG, Winkel BG, Risgaard B, Nielsen JB, Rasmussen PV, Haunso S, et al. Epilepsy and risk of death and sudden unexpected death in the young: a nationwide study. Epilepsia. (2013) 54:1613–20. doi: 10.1111/epi.12328
4. Sveinsson O, Andersson T, Carlsson S, Tomson T. The incidence of SUDEP: A nationwide population-based cohort study. Neurology. (2017) 89:170–7. doi: 10.1212/WNL.0000000000004094
5. Lhatoo SD, Faulkner HJ, Dembny K, Trippick K, Johnson C, Bird JM. An electroclinical case-control study of sudden unexpected death in epilepsy. Ann Neurol. (2010) 68:787–96. doi: 10.1002/ana.22101
6. Ryvlin P, Nashef L, Lhatoo S, Bateman L, Bird J, Bleasel A, et al. Incidence and mechanisms of cardiorespiratory arrests in epilepsy monitoring units (MORTEMUS): a retrospective study. Lancet Neurol. (2013) 12:966–77. doi: 10.1016/s1474-4422(13)70214-x
7. Surges R, Strzelczyk A, Scott C, Walker M, Sander JJE. Postictal generalized electroencephalographic suppression is associated with generalized seizures. Epilepsy Behav. (2011) 21:271–4. doi: 10.1016/j.yebeh.2011.04.008
8. Lamberts RJ, Gaitatzis A, Sander JW, Elger CE, Surges R, Thijs RD. Postictal generalized EEG suppression: an inconsistent finding in people with multiple seizures. Neurology. (2013) 81:1252–6. doi: 10.1212/WNL.0b013e3182a6cbeb
9. Tao JX, Yung I, Lee A, Rose S, Jacobsen J, Ebersole JS. Tonic phase of a generalized convulsive seizure is an independent predictor of postictal generalized EEG suppression. Epilepsia. (2013) 54:858–65. doi: 10.1111/epi.12094
10. Alexandre V, Mercedes B, Valton L, Maillard L, Bartolomei F, Szurhaj W, et al. Risk factors of postictal generalized EEG suppression in generalized convulsive seizures. Neurology. (2015) 85:1598–603. doi: 10.1212/WNL.0000000000001949
11. Theeranaew W, McDonald J, Zonjy B, Kaffashi F, Moseley BD, Friedman D, et al. Automated detection of postictal generalized EEG suppression. IEEE Trans Biomed Eng. (2018) 65:371–7. doi: 10.1109/TBME.2017.2771468
12. Fisher RS, Cross JH, French JA, Higurashi N, Hirsch E, Jansen FE, et al. Operational classification of seizure types by the international league against epilepsy: position paper of the ILAE commission for classification and terminology. Epilepsia. (2017) 58:522–30. doi: 10.1111/epi.13670
13. Vilella L, Lacuey N, Hampson JP, Rani MRS, Sainju RK, Friedman D, et al. Postconvulsive central apnea as a biomarker for sudden unexpected death in epilepsy (SUDEP). Neurology. (2019) 92:e171–82. doi: 10.1212/wnl.0000000000006785
14. Seyal M, Bateman L, Li CJE. Impact of periictal interventions on respiratory dysfunction, postictal EEG suppression, and postictal immobility. Epilepsia. (2013) 54:377–82. doi: 10.1111/j.1528-1167.2012.03691.x
15. Seyal M, Hardin K, Bateman LJE. Postictal generalized EEG suppression is linked to seizure-associated respiratory dysfunction but not postictal apnea. Epilepsia. (2012) 53:825–31. doi: 10.1111/j.1528-1167.2012.03443.x
16. Freitas J, Kaur G, Fernandez G, Tatsuoka C, Kaffashi F, Loparo K, et al. Age-specific periictal electroclinical features of generalized tonic-clonic seizures and potential risk of sudden unexpected death in epilepsy (SUDEP). Epilepsy Behav. (2013) 29:289–94. doi: 10.1016/j.yebeh.2013.08.010
17. Xu J, Jin B, Yan J, Wang J, Hu J, Wang Z, et al. Postictal generalized EEG suppression after generalized convulsive seizures: a double-edged sword. Clin Neurophysiol. (2016) 127:2078–84. doi: 10.1016/j.clinph.2015.10.064
18. Tilz C, Stefan H, Hopfengaertner R, Kerling F, Genow A, Wang-Tilz Y. Influence of levetiracetame on ictal and postictal EEG in patients with partial seizures. Eur J Neurol. (2006) 13:1352–8. doi: 10.1111/j.1468-1331.2006.01516.x
19. Kang JY, Rabiei AH, Myint L, Nei M. Equivocal significance of post-ictal generalized EEG suppression as a marker of SUDEP risk. Seizure. (2017) 48:28–32. doi: 10.1016/j.seizure.2017.03.017
20. Asadollahi M, Noorbakhsh M, Simani L, Ramezani M, Gharagozli K. Two predictors of postictal generalized EEG suppression: tonic phase duration and postictal immobility period. Seizure. (2018) 61:135–8. doi: 10.1016/j.seizure.2018.08.009
21. Lamberts R, Thijs R, Laffan A, Langan Y, Sander JJE. Sudden unexpected death in epilepsy: people with nocturnal seizures may be at highest risk. Epilepsia. (2012) 53:253–7. doi: 10.1111/j.1528-1167.2011.03360.x
22. Zhuo L, Zhang Y, Zielke H, Levine B, Zhang X, Chang L, et al. Sudden unexpected death in epilepsy: Evaluation of forensic autopsy cases. Forensic Sci Int. (2012) 223:171–5. doi: 10.1016/j.forsciint.2012.08.024
23. Lamberts R, Laranjo S, Kalitzin S, Velis D, Rocha I, Sander J, et al. Postictal generalized EEG suppression is not associated with periictal cardiac autonomic instability in people with convulsive seizures. Epilepsia. (2013) 54:523–9. doi: 10.1111/epi.12021
24. Lee A, Wu S, Zhou X, Liebenthal J, Rose S, Tao JX. Periictal autonomic dysfunction and generalized postictal EEG suppression in convulsive seizures arising from sleep and wakefulness. Epilepsy Behav. (2013) 28:439–43. doi: 10.1016/j.yebeh.2013.06.010
25. Pavlova M, Singh K, Abdennadher M, Katz E, Dworetzky B, White DP, et al. Comparison of cardiorespiratory and EEG abnormalities with seizures in adults and children. Epilepsy Behav. (2013) 29:537–41. doi: 10.1016/j.yebeh.2013.09.026
26. Sarkis RA, Thome-Souza S, Poh MZ, Llewellyn N, Klehm J, Madsen JR, et al. Autonomic changes following generalized tonic clonic seizures: an analysis of adult and pediatric patients with epilepsy. Epilepsy Res. (2015) 115:113–8. doi: 10.1016/j.eplepsyres.2015.06.005
27. Moseley B, DeGiorgio CM. The SUDEP risk inventory: association with postictal generalized EEG suppression. Epilepsy Res. (2015) 117:82–4. doi: 10.1016/j.eplepsyres.2015.09.006
28. Wu S, Issa N, Rose S, Ali A, Tao JX. Impact of periictal nurse interventions on postictal generalized EEG suppression in generalized convulsive seizures. Epilepsy Behav. (2016) 58:22–5. doi: 10.1016/j.yebeh.2016.02.025
29. Moseley B, So E, Wirrell E, Nelson C, Lee R, Mandrekar J, et al. Characteristics of postictal generalized EEG suppression in children. Epilepsy Res. (2013) 106:123–7. doi: 10.1016/j.eplepsyres.2013.05.007
Keywords: PGES, generalized convulsive seizure, epilepsy, SUDEP, mortality, post-ictal generalized EEG suppression, sudden unexpected death in epilepsy
Citation: Zhao X, Vilella L, Zhu L, Rani MRS, Hampson JP, Hampson J, Hupp NJ, Sainju RK, Friedman D, Nei M, Scott C, Allen L, Gehlbach BK, Schuele S, Harper RM, Diehl B, Bateman LM, Devinsky O, Richerson GB, Zhang G-Q, Lhatoo SD and Lacuey N (2021) Automated Analysis of Risk Factors for Postictal Generalized EEG Suppression. Front. Neurol. 12:669517. doi: 10.3389/fneur.2021.669517
Received: 19 February 2021; Accepted: 13 April 2021;
Published: 11 May 2021.
Edited by:
Brian Darryl Moseley, University of Cincinnati, United StatesReviewed by:
Elena Gardella, University of Southern Denmark, DenmarkCopyright © 2021 Zhao, Vilella, Zhu, Rani, Hampson, Hampson, Hupp, Sainju, Friedman, Nei, Scott, Allen, Gehlbach, Schuele, Harper, Diehl, Bateman, Devinsky, Richerson, Zhang, Lhatoo and Lacuey. This is an open-access article distributed under the terms of the Creative Commons Attribution License (CC BY). The use, distribution or reproduction in other forums is permitted, provided the original author(s) and the copyright owner(s) are credited and that the original publication in this journal is cited, in accordance with accepted academic practice. No use, distribution or reproduction is permitted which does not comply with these terms.
*Correspondence: Laura Vilella, bHZpbGVsbGFiZXJ0cmFuQGdtYWlsLmNvbQ==
†These authors share first authorship
Disclaimer: All claims expressed in this article are solely those of the authors and do not necessarily represent those of their affiliated organizations, or those of the publisher, the editors and the reviewers. Any product that may be evaluated in this article or claim that may be made by its manufacturer is not guaranteed or endorsed by the publisher.
Research integrity at Frontiers
Learn more about the work of our research integrity team to safeguard the quality of each article we publish.