- 1Department of Otolaryngology-Head and Neck Surgery, Johns Hopkins University School of Medicine, Baltimore, MD, United States
- 2Department of Neurology, Johns Hopkins University School of Medicine, Baltimore, MD, United States
- 3Department of Neurology, Royal Prince Alfred Hospital, Camperdown, NSW, Australia
Objective: Capillaries within the inner ear form a semi-permeable barrier called the blood-labyrinth barrier that is less permeable than capillary barriers elsewhere within the human body. Dysfunction of the blood-labyrinth barrier has been proposed as a mechanism for several audio-vestibular disorders. There has been interest in using magnetic resonance imaging (MRI) with intravenous gadolinium-based contrast agents (GBCA) as a marker for the integrity of the blood labyrinth barrier in research and clinical settings. This scoping review evaluates the evidence for using intravenous gadolinium-enhanced MRI to assess the permeability of the blood-labyrinth barrier in healthy and diseased ears.
Methods: A systematic search was conducted of three databases: PubMed, EMBASE, CINAHL PLUS. Studies were included that used GBCA to study the inner ear and permeability of the blood-labyrinth barrier. Data was collected on MRI protocols used and inner ear enhancement patterns of healthy and diseased ears in both human and animal studies.
Results: The search yielded 14 studies in animals and 53 studies in humans. In healthy animal and human inner ears, contrast-enhanced MRI demonstrated gradual increase in inner ear signal intensity over time that was limited to the perilymph. Signal intensity peaked at 100 min in rodents and 4 h in humans. Compared to controls, patients with idiopathic sudden sensorineural hearing loss and otosclerosis had increased signal intensity both before and shortly after GBCA injection. In patients with Ménière's disease and vestibular schwannoma, studies reported increased signal at 4 h, compared to controls. Quality assessment of included studies determined that all the studies lacked sample size justification and many lacked adequate control groups or blinded assessors of MRI.
Conclusions: The included studies provided convincing evidence that gadolinium crosses the blood-labyrinth barrier in healthy ears and more rapidly in some diseased ears. The timing of increased signal differs by disease. There was a lack of evidence that these findings indicate general permeability of the blood-labyrinth barrier. Future studies with consistent and rigorous methods are needed to investigate the relationship between gadolinium uptake and assessments of inner ear function and to better determine whether signal enhancement indicates permeability for molecules other than gadolinium.
Introduction
Living tissues need a steady supply of nutrients to support metabolism and clear waste. Blood vessels lined by endothelial cells transport these metabolic resources, and capillaries are the site at which nutrients and waste are exchanged. Capillaries have different features depending on the needs of nearby tissues, being more porous, for example in the liver, or more restrictive in the retina (1). In some locations, such as the brain, capillaries form junctions that are so impermeable they create a continuous barrier. In the inner ear, a blood-labyrinth barrier was first proposed to explain differential uptake of intravenously injected compounds between the endolymph and perilymph spaces (2).
Intravenously injected compounds reach the inner ear via the labyrinthine artery, a branch of the anterior inferior cerebellar artery, that subsequently branches into smaller vessels to supply the labyrinth and cochlea (Figure 1A). Capillary networks in the inner ear are clustered around the stria vascularis and spiral ligament in the cochlea, and the sensory epithelia of the vestibular system (3). These capillary networks are—like in the eye and brain—the presumed location of the blood-labyrinth barrier, composed of endothelial cells with tight-junctions surrounded by pericytes and resident macrophages (4) (Figure 1B). These barriers tightly regulate ion composition within the endolymph and perilymph, and are permeable to water, glucose, and small molecules (5, 6). Molecules transit across the barrier via a variety of mechanisms including diffusion, endocytosis, and transcellular protein transport (7) (Figure 1C).
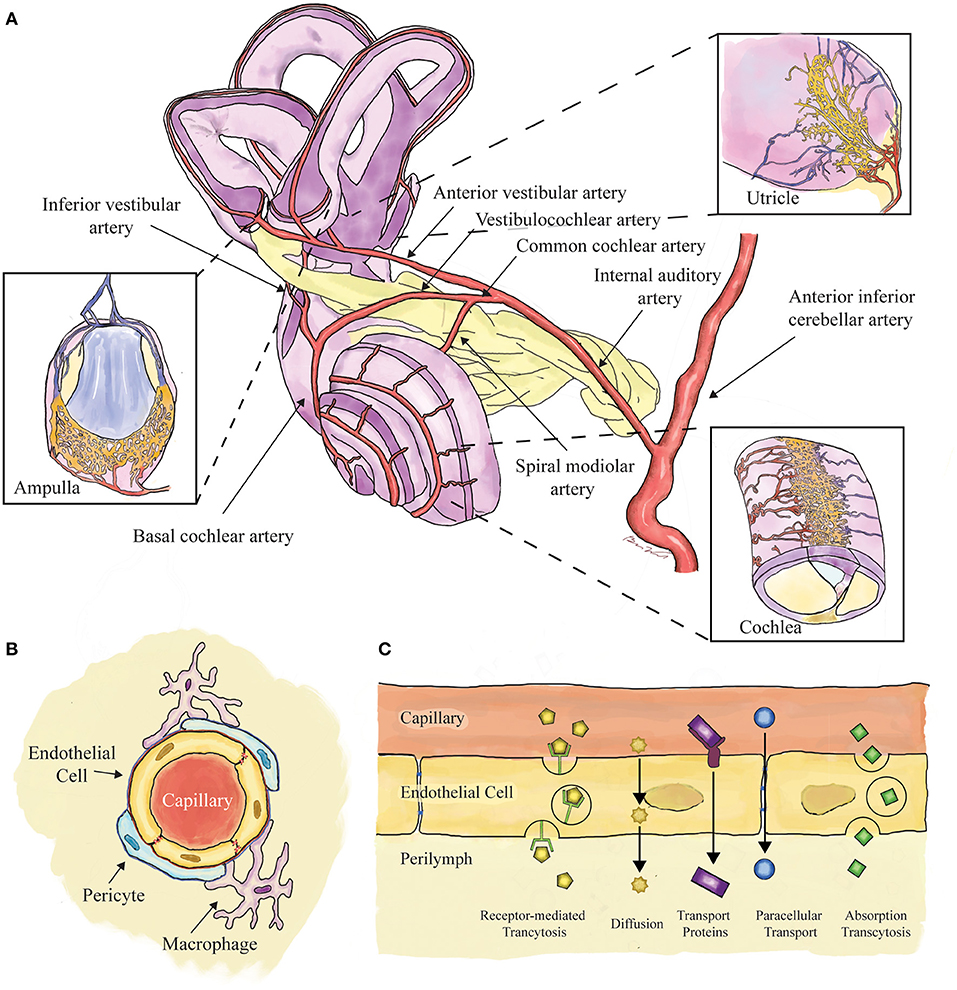
Figure 1. Schematic showing the components of the blood-labyrinth barrier. (A) Blood supply to the labyrinth is shown, with insets showing capillary beds near the sensory epithelia of the ampullae, otoconial organs, and cochlea. (B) Capillaries of the blood-labyrinth barrier include endothelial cells with tight junctions, surrounded by pericytes and resident macrophages that regulate permeability. (C) Examples of hypothetical mechanisms by which molecules can transit across the barrier are shown.
The role of the blood-labyrinth barrier in clinical medicine is receiving increased attention. Studies have shown that several hours following intravenous administration of gadolinium-based contrast agents (GBCA), the perilymphatic space of the inner ear enhances on magnetic resonance imaging (MRI) studies (8). MRI using stronger static magnetic fields and new pulse sequences has improved spatial resolution and takes advantage of this contrast between the endolymph-filled membranous labyrinth and the surrounding perilymphatic space. These MRI techniques have emerged as a useful research tool in the study of Ménière's disease, a disorder in which patients commonly have a swelling of the membranous labyrinth (9). It is presumed that the increased signal intensity reflects GBCA crossing the blood-labyrinth barrier.
More recently, studies have begun exploring the use of GBCA to determine the integrity of the blood-labyrinth barrier in disease. In addition to transmitting small molecules, capillaries also allow the transit of white blood cells into tissues (10). During inflammation, released cytokines can activate capillary endothelial cells, increasing capillary permeability (11). Increased permeability may have deleterious effects on the inner ear (4). An imaging marker of the permeability of the blood-labyrinth barrier could provide diagnostic and prognostic information and aid the development of new therapies for inner ear diseases. The aim of this scoping review was to assess the evidence for the use of GBCA as a marker of permeability of the blood-labyrinth barrier in animals and humans with inner ear disease.
Methods
A scoping review was performed with the aim of synthesizing knowledge regarding the use of MRI to assess the integrity of the blood-labyrinth barrier. A broad search was performed using three databases: PubMed, EMBASE, and CINAHL Plus. This search was performed on 10/5/2020 using controlled vocabulary (e.g., MeSH terms in PubMed) and keywords related to the concepts of the “inner ear,” “contrast-enhanced MRI,” and the “blood-labyrinth barrier” (see Supplementary Material). The search strategy was created with assistance from staff at the Welch Medical Library at Johns Hopkins Medicine.
Two study members (CS and BW) independently evaluated articles and included those that met the following inclusion criteria: aims to assess the blood-labyrinth barrier with MRI, uses a GBCA administered via intravenous injection, includes original data, is not a case report (i.e., must include data from more than one individual), and is written in English language. Additional articles were included via a snowballing approach, where the references in each included article were assessed using the same inclusion criteria.
For both clinical and animal studies we recorded data on enhancement of inner ear structures in diseased and healthy ears, the imaging protocol, MRI static magnetic field strength, the GBCA and dose, and the time from contrast injection to image acquisition.
All co-authors reviewed the included studies and two study members (CS and BW) also evaluated the quality and risk of bias of each clinical research article using a modified version of the National Institutes of Health (NIH) Quality Assessment Tool for Cross-Sectional Studies (12) (Table 1).
Since the goal of this scoping review was to qualitatively describe the current literature and map key concepts in this field, test statistics were not performed.
Results
Literature Search
Our database search yielded 243 citations, 88 from PubMed, 109 from EMBASE, and 46 from CINAHL Plus. After duplicate articles were removed, there were 135 unique search results. After full text review with the application of selection criteria we had 40 included publications. Additional review of the references from the initial included publications yielded an additional 27 citations that met the selection criteria and were included in the final analysis (Figure 2).
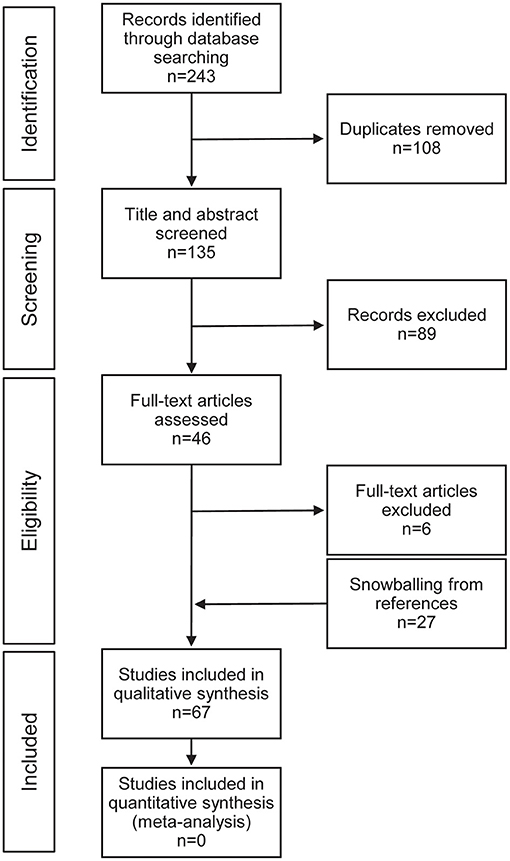
Figure 2. Preferred reporting items for systematic reviews and meta-analyses (PRISMA) flow diagram for study selection.
Animal Studies
There were 14 animal studies in this review that evaluated MRI of healthy and diseased inner ears of guinea pigs and/or mice. Eleven of these studies administered contrast agent in doses of 1.5 mmol/kg and used MRI scanners of magnetic field strengths 4.7 Tesla (T) or greater. Studies in healthy ears of guinea pigs and mice reported increased signal intensity within the perilymph, consistent with GBCA uptake, that increased over time and peaked at 100 min, whereas no enhancement was seen in the endolymph (13–18). Enhancement was first seen in the cochlea with relative greater signal in the scala tympani than the scala vestibuli, followed by the utricle and saccule, and the ampullated ends of the semicircular canals (19, 20). The areas correspond to capillary networks in the inner ear, suggesting entry of GBCA at the blood-labyrinth barrier. Comparison of different GBCA found some variation in signal intensity and uptake kinetics, but intravenous administration of both macrocyclic and linear agents reliably led to enhancement of perilymph (20, 21). In mice, all GBCAs led to enhancement of perilymph with increasing intensity over time from 15–105 min (20). Gadobutrol (Gadovist) was shown to have the most rapid uptake and greatest signal enhancement while gadopentetate dimeglumine (Magnevist) had the slowest and lowest intensity of enhancement (20). In three studies, mechanical trauma to and inflammation of the inner ear were found to increase the rate of signal intensity rise in the inner ear following intravenous GBCA over 15–80 min above the rate in control animals (14, 19, 22).
Human Studies
Our search yielded 53 studies in humans. Of these, 43 were cross-sectional studies and 10 were descriptive case series. Case series were defined as studies that provided only descriptive characteristics of included patients. The case series provided a useful historical framework for subsequent studies but were not included in our analysis (23–32). In healthy human ears, described in two included studies, enhancement of the inner ear peaked at 4 h following intravenous injection of 0.1 and 0.3 mmol/kg GBCA using 3T MRI and 3D fluid-attenuated inversion recovery (FLAIR) pulse sequences (8, 33).
Our quality assessment found flaws in methodological rigor across the included studies (Table 2). Assessment of the included cross-sectional studies with the NIH quality assessment tool found that none (0%) of the studies provided sample size justification, 20 (47%) either did not specify whether the MRI assessment was blinded or had used assessors that were not blinded, and 18 (42%) lacked a control population for comparing abnormal enhancement. Additionally, 35 (81%) did not assess enhancement at multiple time points, and 21 (49%) did not provide data on signal intensity with different levels of contrast exposure (e.g., signal intensity before and after contrast administration).
Contrast-enhanced MRI in patients with idiopathic sudden sensorineural hearing loss (ISSHL) was described in 14 included studies (Table 3). These studies reported increased signal intensity within the affected inner ear on 3D-FLAIR imaging taken before and after contrast administration. However, not all patients demonstrated increased signal intensity at either time point. Two studies noted that hyperintensity of the diseased ears was more prevalent in MRI acquired before contrast was administered, as opposed to images taken shortly after or at 4 h after GBCA injection (44, 54). Others reported an increased prevalence of abnormal enhancement of diseased ears when MRI was performed 4 h after GBCA administration as compared to MRI performed shortly after GBCA administration (58, 64). One study reported a correlation between increased signal in the diseased ear and poorer prognosis, but the association was only observed for pre-contrast imaging (44). Another study described a poorer prognosis when increased signal was observed on MRI performed 4 h after contrast administration (69). A third study reported a correlation between enhancement seen on MRI taken shortly after contrast injection and poorer initial hearing level but did not find a correlation with prognosis for hearing recovery (54).
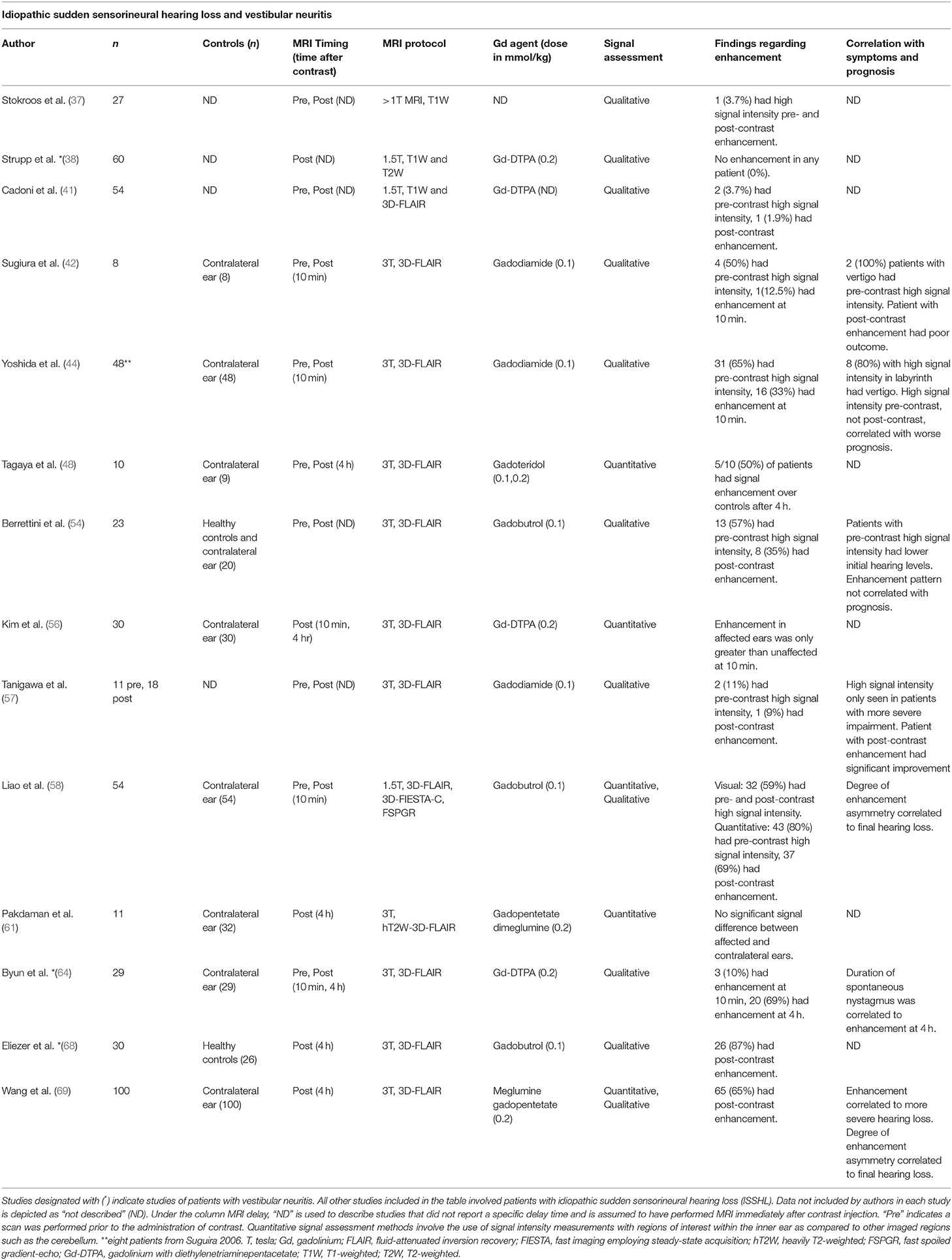
Table 3. Summary of findings in studies of idiopathic sudden sensorineural hearing loss and vestibular neuritis.
MRI findings in patients with Ménière's disease were described in 12 studies (Table 4). These studies reported increased enhancement of the affected ear in MRI acquired 4 h after contrast but no abnormal hyperintensity in imaging before contrast or at imaging 10 min after contrast. One study compared imaging taken 10 min and 4 h after contrast injection and reported an increase in signal intensity over controls only at 4 h (52). This observation was supported by one other study; however, this study only included one patient with unilateral definite Ménière's disease, and three with possible Ménière's disease (53). One study reported a correlation between enhancement on imaging 4 h after contrast and both the degree of hearing loss and severity of endolymphatic hydrops (73), while another study found no association between enhancement and hearing loss (50).
Five included studies examined MRI findings in patients with vestibular schwannoma (Table 5). Two studies reported enhancement of inner ear structures 7–10 min after contrast administration (45, 46). This was supported by another study which found decreased signal on 3D-FIESTA shortly after contrast injection which, similar to hyperintensity on 3D-FLAIR, suggests increased protein content in the inner ear (55). Another study reported a greater enhancement 5–8 h after contrast injection as compared to imaging immediately after contrast injection (70). Signal intensity at 5–8 h was correlated with poorer hearing as measured by pure tone thresholds and word recognition scores (70). The other studies with imaging performed shortly after GBCA administration reported no correlation between signal intensity and hearing level or tumor size (45, 46).
Four studies reported on MRI findings in patients with otosclerosis (Table 6). Two described increased signal intensity on MRI before and shortly after contrast administration (59, 63). Two studies reported a correlation between enhancement and disease stage (60, 63), while another study reported no correlation between enhancement and degree of hearing loss (74).
Our review also included 10 studies reporting abnormal inner ear enhancement in patients with sudden facial nerve paralysis (34, 35, 47), DFNA9 (mutation in the COCH gene) (67), Cogan syndrome (25), viral and bacterial labyrinthitis (23, 24, 30), and other inner ear abnormalities (39, 43, 49, 72).
Discussion
Damage to the blood-labyrinth barrier has been implicated in the pathophysiology of inner ear disorders such as Ménière's disease and ISSHL (54, 75). Recently, investigators have begun using intravenous contrast-enhanced MRI to assess the permeability of this barrier as a potential tool in research and for diagnosis. The goal of this review was to evaluate current evidence for the role of gadolinium-based contrast in understanding blood-labyrinth barrier function and its utility in evaluating the integrity of this barrier in disease states of the inner ear.
Studies of both healthy and diseased ears of animals and humans demonstrated enhancement of inner ear structures following the administration of IV-gadolinium. In healthy ears of mice and guinea pigs, enhancement peaked and plateaued at 100 min after contrast administration. Further studies in animal ears affected by mechanical trauma (14) and inflammation (22) demonstrated increased enhancement, suggesting that disease states may increase the inner ear's permeability to gadolinium-based agents, potentially through alterations in the blood-labyrinth barrier. Studies have not sought to identify the mechanism(s) by which gadolinium crosses the blood-labyrinth barrier. Notably, these animal studies used an MRI magnetic field strength of at least 4.7 T, which exceeds the standard used in most human studies (1.5 and 3.0 T). Animals were also administered gadolinium at doses that were up to 15 times greater than the clinical standard of 0.1 mmol/kg for humans. Studies in healthy humans reported reliable gadolinium uptake within the inner ear 4 h after injection with either 0.1 (33) or 0.3 (8) mmol/kg gadolinium using a 3 T MRI scanner.
Pathologies such as ISSHL, Ménière's disease, otosclerosis, and vestibular schwannoma have been shown to alter this baseline GBCA enhancement by increasing the intensity of signal on MRI within inner ear structures. Although abnormal enhancement was well-described by most studies, there were conflicting reports of correlations between this enhancement and clinical characteristics such as prognosis and disease severity.
Although increased enhancement was common in these diseases, there were distinct differences by disease in the time of the observed increased signal. In both ISSHL and otosclerosis, hyperintense inner ear signal was present even before contrast was administered and just minutes after GBCA injection. Meanwhile, enhancement in patients with Ménière's disease or vestibular schwannoma was reported after a delay of four or more hours from contrast administration. Hyperintensity prior to contrast injection in cerebrospinal fluid has been hypothesized to result from increased protein content and could be a hypothesis for the increased pre-contrast signal seen in some patients with ISSHL and otosclerosis (76). Quicker uptake of contrast, or increased baseline signal, could indicate more severe blood-labyrinth barrier damage resulting in greater passage of GBCA and possibly protein into the perilymph. Alternatively, GBCA could have different routes of entry into perilymph, depending on the pathophysiology of the disease. The kinetics of enhancement with gadolinium could be important for evaluating the blood-labyrinth barrier in different disease states. However, due to the current paucity of studies on the time course of enhancement in disease, it is impossible to make a reliable comparison of blood-labyrinth barrier permeability in different disease states. Only two studies with few patients reported on enhancement findings at two time points in patients with Ménière's disease (52). While this early data is promising, studies with images taken at multiple time points are needed to better describe differences in the pattern of enhancement among different inner ear diseases compared to control ears.
Our analysis of the evidence was hampered by study design. Most notably there was a lack of adequate control groups, sample size justifications, and clear patient recruitment details. Compounding these quality issues was the substantial degree of variability in study protocols—particularly regarding the contrast agents used and the methods for determining enhancement. Counter et al. demonstrated that although linear and macrocyclic GBCA agents were useful in enhancing the inner ear structures, there was a distinct heterogenicity in kinetics (20). This makes it difficult to compare studies using different GBCA agents, given the importance of consistent enhancement kinetics when comparing uptake patterns in different disease states.
Additionally, there was no consensus on how to distinguish between normal and abnormal enhancement patterns. In studies that used controls, either from contralateral ears or healthy volunteers, some studies used subjective, visual assessment. Others used more objective measures of signal intensity normalized to signal from regions of the scan that were presumably unaffected by the disease. The consistency of control groups used in studies on systemic diseases or disease that can affect both ears such as Ménière's disease is particularly important. One included study found that asymptomatic ears of patients with Ménière's disease had increased signal intensity on contrast-enhanced MRI compared to controls (61), while another study described contrast enhancement of the asymptomatic ear in two patients with clinically unilateral Ménière's disease (66).
The goal of this scoping review was to evaluate the usefulness of IV-gadolinium MRI in assessing blood-labyrinth barrier permeability in healthy and diseased ears. Following intravenous administration of GBCA, GBCA enters the inner ear in healthy ears after a delay, presumably through the blood-labyrinth barrier. Despite these observations in healthy animals and humans, we found no conclusive evidence to support the assumption that gadolinium can be used to directly measure the health of the blood-labyrinth barrier. Our review also found a lack of studies that could correlate abnormal enhancement of the inner ear with a more general breakdown of the blood labyrinth barrier. While there is compelling evidence demonstrating increased GBCA uptake in diseased ears, future work must be done to clarify if any connection exists between abnormal enhancement and breakdown of the blood-labyrinth barrier. Furthermore, different disease states may affect the blood-labyrinth barrier by different mechanisms. There is a gap in the current literature regarding the mechanisms of GBCA uptake into the perilymph, the time course of uptake in diseased human ears, and the natural course of GBCA efflux from the perilymph. Rigorous future studies with adequate controls, clear patient recruitment methods, and objective measures of enhancement patterns are needed to determine the utility of contrast-enhanced MRI in assessing the integrity of the blood-labyrinth barrier.
Data Availability Statement
The original contributions presented in the study are included in the article/Supplementary Material, further inquiries can be directed to the corresponding author.
Author Contributions
BW and CS conceived of the concept of the article and the study design, applied the article quality assessment, and performed the initial draft of the manuscript. BW designed Figure 1. CS performed the initial search, designed Figure 2, and constructed the tables. All authors reviewed the included articles. All authors reviewed and approved the final submitted version of the manuscript.
Funding
BW is supported by clinician-scientist awards from the American Otological Society and Johns Hopkins University School of Medicine, as well as K23DC018302. NA is supported by T32DC000027. JP is supported by a Junior Fellowship from the Garnett Passe and Rodney Williams Memorial Foundation and by philanthropy received from the Cinquegrana, Lott, and Schwerin families through Prof. David Zee at Johns Hopkins University.
Conflict of Interest
The authors declare that the research was conducted in the absence of any commercial or financial relationships that could be construed as a potential conflict of interest.
Acknowledgments
The authors would like to acknowledge the help of Rachael Lebo at the Welch Medical Library who assisted CS with the development of the initial search.
Supplementary Material
The Supplementary Material for this article can be found online at: https://www.frontiersin.org/articles/10.3389/fneur.2021.662264/full#supplementary-material
References
1. Pournaras CJ, Rungger-Brändle E, Riva CE, Hardarson SH, Stefansson E. Regulation of retinal blood flow in health and disease. Prog Retin Eye Res. (2008) 27:284–330. doi: 10.1016/j.preteyeres.2008.02.002
2. Misrahy GA, Spradley JF, Beran AV, Garwood VP. Permeability of cochlear partitions: comparison with blood-brain barrier. Acta Otolaryngol. (1960) 52:525–34. doi: 10.3109/00016486009123183
3. Mazzoni A. The vascular anatomy of the vestibular labyrinth in man. Acta Oto-Laryngol Suppl. (1990) 472:1–83. doi: 10.3109/00016489009121137
4. Zhang W, Dai M, Fridberger A, Hassan A, Degagne J, Neng L, et al. Perivascular-resident macrophage-like melanocytes in the inner ear are essential for the integrity of the intrastrial fluid-blood barrier. Proc Natl Acad Sci USA. (2012) 109:10388–93. doi: 10.1073/pnas.1205210109
5. Juhn SK, Rybak LP, Prado S. Nature of blood-labyrinth barrier in experimental conditions. Ann Otol Rhinol Laryngol. (1981) 90(2 Pt 1):135–41. doi: 10.1177/000348948109000208
6. Juhn SK, Rybak LP, Fowlks WL. Transport characteristics of the blood–perilymph barrier. Am J Otolaryngol. (1982) 3:392–6. doi: 10.1016/S0196-0709(82)80016-1
7. Nyberg S, Abbott NJ, Shi X, Steyger PS, Dabdoub A. Delivery of therapeutics to the inner ear: the challenge of the blood-labyrinth barrier. Sci Transl Med. (2019) 11:eaao0935. doi: 10.1126/scitranslmed.aao0935
8. Carfrae MJ, Holtzman A, Eames F, Parnes SM, Lupinetti A. 3 Tesla delayed contrast magnetic resonance imaging evaluation of Meniere's disease. Laryngoscope. (2008) 118:501–5. doi: 10.1097/MLG.0b013e31815c1a61
9. Rauch SD, Merchant SN, Thedinger BA. Meniere's syndrome and endolymphatic hydrops. Double-blind temporal bone study. Ann Otol Rhinol Laryngol. (1989) 98:873–83. doi: 10.1177/000348948909801108
10. Hirose K, Discolo CM, Keasler JR, Ransohoff R. Mononuclear phagocytes migrate into the murine cochlea after acoustic trauma. J Comp Neurol. (2005) 489:180–94. doi: 10.1002/cne.20619
11. Ichimiya I, Yoshida K, Hirano T, Suzuki M, Mogi G. Significance of spiral ligament fibrocytes with cochlear inflammation. Int J Pediatr Otorhinolaryngol. (2000) 56:45–51. doi: 10.1016/S0165-5876(00)00408-0
12. Study Quality Assessment Tools | NHLBI, NIH. Available online at: http://www.nhlbi.nih.gov/health-topics/study-quality-assessment-tools (accessed January 19, 2021).
13. Counter SA, Bjelke B, Klason T, Chen Z, Borg E. Magnetic resonance imaging of the cochlea, spiral ganglia and eighth nerve of the guinea pig. Neuroreport. (1999) 10:473–9. doi: 10.1097/00001756-199902250-00006
14. Counter SA, Bjelke B, Borg E, Klason T, Chen Z, Duan ML. Magnetic resonance imaging of the membranous labyrinth during in vivo gadolinium (Gd-DTPA-BMA) uptake in the normal and lesioned cochlea. Neuroreport. (2000) 11:3979–83. doi: 10.1097/00001756-200012180-00015
15. Zou J, Pyykko I, Bretlau P, Klason T, Bjelke B. In vivo visualization of endolymphatic hydrops in guinea pigs: magnetic resonance imaging evaluation at 4.7 tesla. Ann Otol Rhinol Laryngol. (2003) 112:1059–65. doi: 10.1177/000348940311201212
16. Zou J, Pyykkö I, Counter SA, Klason T, Bretlau P, Bjelke B. In vivo observation of dynamic perilymph formation using 4.7 t MRI with gadolinium as a tracer. Acta Otolaryngol (Stockh). (2003) 123:910. doi: 10.1080/00016480310000548
17. Zou J, Zhang W, Poe D, Zhang Y, Ramadan UA, Pyykko I. Differential passage of gadolinium through the mouse inner ear barriers evaluated with 4.7T MRI. Hear Res. (2010) 259:36–43. doi: 10.1016/j.heares.2009.09.015
18. Counter SA, Nikkhou S, Brene S, Damberg P, Sierakowiak A, Klason T, et al. MRI evidence of endolymphatic impermeability to the gadolinium molecule in the in vivo mouse inner ear at 9.4 tesla. Open Neuroimaging J. (2013) 7:27–31. doi: 10.2174/1874440001307010027
19. Counter SA, Zou J, Bjelke B, Klason T. 3D MRI of the in vivo vestibulo-cochlea labyrinth during Gd-DTPA-BMA uptake. Neuroreport. (2003) 14:1707–12. doi: 10.1097/00001756-200309150-00010
20. Counter SA, Nikkhou-Aski S, Damberg P, Berglin CE, Laurell G. Ultra-high-field (9.4 T) MRI analysis of contrast agent transport across the blood-perilymph barrier and intrastrial fluid-blood barrier in the mouse inner ear. Otol Neurotol. (2017) 38:1052–9. doi: 10.1097/MAO.0000000000001458
21. Videhult Pierre P, Rasmussen JE, Nikkhou Aski S, Damberg P, Laurell G. High-dose furosemide enhances the magnetic resonance signal of systemic gadolinium in the mammalian cochlea. Otol Neurotol. (2020) 41:545–53. doi: 10.1097/MAO.0000000000002571
22. Floc'h JL, Tan W, Telang RS, Vlajkovic SM, Nuttall A, Rooney WD, et al. Markers of cochlear inflammation using MRI. J Magn Reson Imaging JMRI. (2014) 39:150–61. doi: 10.1002/jmri.24144
23. Seltzer S, Mark AS. Contrast enhancement of the labyrinth on MR scans in patients with sudden hearing loss and vertigo: evidence of labyrinthine disease. AJNR Am J Neuroradiol. (1991) 12:13–6.
24. Mark AS, Seltzer S, Nelson-Drake J, Chapman JC, Fitzgerald DC, Gulya AJ. Labyrinthine enhancement on gadolinium-enhanced magnetic resonance imaging in sudden deafness and vertigo: correlation with audiologic and electronystagmographic studies. Ann Otol Rhinol Laryngol. (1992) 101:459–64. doi: 10.1177/000348949210100601
25. Majoor M.H.J.M., Albers F.W.J., Casselman J.W. Clinical relevance of magnetic resonance imaging and computed tomography in Cogan's syndrome. Acta Otolaryngol (Stockh). (1993) 113:625–31. doi: 10.3109/00016489309135875
26. Albers FW, Demuynck KM, Casselman JW. Three-dimensional magnetic resonance imaging of the inner ear in idiopathic sudden sensorineural hearing loss. ORL J Oto-Rhino-Laryngol Its Relat Spec. (1994) 56:1–4. doi: 10.1159/000276599
27. Sone M, Mizuno T, Naganawa S, Nakashima T. Imaging analysis in cases with inflammation-induced sensorineural hearing loss. Acta Otolaryngol. (2009) 129:239–43. doi: 10.1080/00016480802226163
28. Peng R, Chow D, De Seta D, Lalwani AK. Intensity of gadolinium enhancement on MRI is useful in differentiation of intracochlear inflammation from tumor. Otol Neurotol. (2014) 35:905–10. doi: 10.1097/MAO.0000000000000300
29. Mark AS, Fitzgerald D. Segmental enhancement of the cochlea on contrast-enhanced MR: correlation with the frequency of hearing loss and possible sign of perilymphatic fistula and autoimmune labyrinthitis. AJNR Am J Neuroradiol. (1993) 14:991–6.
30. Fitzgerald DC, Mark AS. Viral cochleitis with gadolinium enhancement of the cochlea on magnetic resonance imaging scan. Otolaryngol–Head Neck Surg. (1999) 121:130–2. doi: 10.1016/S0194-5998(99)70141-5
31. Wilson DF, Talbot JM, Hodgson RS. Magnetic resonance imaging-enhancing lesions of the labyrinth and facial nerve. Clinical correlation. Arch Otolaryngol Head Neck Surg. (1994) 120:560–4. doi: 10.1001/archotol.1994.01880290070012
32. Hegarty JL, Patel S, Fischbein N, Jackler RK, Lalwani AK. The value of enhanced magnetic resonance imaging in the evaluation of endocochlear disease. Laryngoscope. (2002) 112:8–17. doi: 10.1097/00005537-200201000-00002
33. Naganawa S, Komada T, Fukatsu H, Ishigaki T, Takizawa O. Observation of contrast enhancement in the cochlear fluid space of healthy subjects using a 3D-FLAIR sequence at 3 Tesla. Eur Radiol. (2006) 16:733–7. doi: 10.1007/s00330-005-0046-8
34. Sartoretti-Schefer S, Wichmann W, Valavanis A. Idiopathic, herpetic, and HIV-associated facial nerve palsies: abnormal MR enhancement patterns. AJNR Am J Neuroradiol. (1994) 15:479–85.
35. Sartoretti-Schefer S, Scherler M, Wichmann W, Valavanis A. Contrast-enhanced MR of the facial nerve in patients with posttraumatic peripheral facial nerve palsy. AJNR Am J Neuroradiol. (1997) 18:1115–25.
36. Fitzgerald DC, Mark AS. Sudden hearing loss: frequency of abnormal findings on contrast-enhanced MR studies. AJNR Am J Neuroradiol. (1998) 19:1433–6.
37. Stokroos RJ, Albers FW, Krikke AP, Casselman JW. Magnetic resonance imaging of the inner ear in patients with idiopathic sudden sensorineural hearing loss. Eur Arch Oto-Rhino-Laryngol. (1998) 255:433–6. doi: 10.1007/s004050050093
38. Strupp M, Jager L, Muller-Lisse U, Arbusow V, Reiser M, Brandt T. High resolution Gd-DTPA MR imaging of the inner ear in 60 patients with idiopathic vestibular neuritis: no evidence for contrast enhancement of the labyrinth or vestibular nerve. J Vestib Res Equilib Orientat. (1998) 8:427–33. doi: 10.3233/VES-1998-8603
39. Schick B, Brors D, Koch O, Schafers M, Kahle G. Magnetic resonance imaging in patients with sudden hearing loss, tinnitus and vertigo. Otol Neurotol. (2001) 22:808–12. doi: 10.1097/00129492-200111000-00016
40. Naganawa S, Koshikawa T, Nakamura T, Fukatsu H, Ishigaki T, Aoki I. High-resolution T1-weighted 3D real IR imaging of the temporal bone using triple-dose contrast material. Eur Radiol. (2003) 13:2650–8. doi: 10.1007/s00330-003-1922-8
41. Cadoni G, Cianfoni A, Agostino S, Scipione S, Tartaglione T, Galli J, et al. Magnetic resonance imaging findings in sudden sensorineural hearing loss. J Otolaryngol. (2006) 35:310–6. doi: 10.2310/7070.2006.0066
42. Sugiura M, Naganawa S, Teranishi M, Nakashima T. Three-dimensional fluid-attenuated inversion recovery magnetic resonance imaging findings in patients with sudden sensorineural hearing loss. Laryngoscope. (2006) 116:1451–4. doi: 10.1097/01.mlg.0000228005.78187.23
43. Sone M, Mizuno T, Sugiura M, Naganawa S, Nakashima T. Three-dimensional fluid-attenuated inversion recovery magnetic resonance imaging investigation of inner ear disturbances in cases of middle ear cholesteatoma with labyrinthine fistula. Otol Neurotol. (2007) 28:1029–33. doi: 10.1097/MAO.0b013e3181587d95
44. Yoshida T, Sugiura M, Naganawa S, Teranishi M, Nakata S, Nakashima T. Three-dimensional fluid-attenuated inversion recovery magnetic resonance imaging findings and prognosis in sudden sensorineural hearing loss. Laryngoscope. (2008) 118:1433–7. doi: 10.1097/MLG.0b013e318172ef85
45. Yamazaki M, Naganawa S, Kawai H, Nihashi T, Fukatsu H, Nakashima T. Increased signal intensity of the cochlea on pre- and post-contrast enhanced 3D-FLAIR in patients with vestibular schwannoma. Neuroradiology. (2009) 51:855–63. doi: 10.1007/s00234-009-0588-6
46. Lee IH, Kim H-J, Chung WH, Kim E, Moon JW, Kim ST, et al. Signal intensity change of the labyrinth in patients with surgically confirmed or radiologically diagnosed vestibular schwannoma on isotropic 3D fluid-attenuated inversion recovery MR imaging at 3 T. Eur Radiol. (2010) 20:949–57. doi: 10.1007/s00330-009-1626-9
47. Nakata S, Mizuno T, Naganawa S, Sugiura M, Yoshida T, Teranishi M, et al. 3D-FLAIR MRI in facial nerve paralysis with and without audio-vestibular disorder. Acta Otolaryngol (Stockh). (2010) 130:632–6. doi: 10.3109/00016480903338123
48. Tagaya M, Teranishi M, Naganawa S, Iwata T, Yoshida T, Otake H, et al. 3 Tesla magnetic resonance imaging obtained 4 hours after intravenous gadolinium injection in patients with sudden deafness. Acta Otolaryngol (Stockh). (2010) 130:665–9. doi: 10.3109/00016480903384176
49. Tanigawa T, Tanaka H, Sato T, Nakao Y, Katahira N, Tsuchiya Y, et al. 3D-FLAIR MRI findings in patients with low-tone sudden deafness. Acta Otolaryngol (Stockh). (2010) 130:1324–8. doi: 10.3109/00016489.2010.496461
50. Suzuki H, Teranishi M, Sone M, Yamazaki M, Naganawa S, Nakashima T. Contrast enhancement of the inner ear after intravenous administration of a standard or double dose of gadolinium contrast agents. Acta Otolaryngol (Stockh). (2011) 131:1025–31. doi: 10.3109/00016489.2011.598552
51. Tagaya M, Yamazaki M, Teranishi M, Naganawa S, Yoshida T, Otake H, et al. Endolymphatic hydrops and blood-labyrinth barrier in Meniere's disease. Acta Otolaryngol (Stockh). (2011) 131:474–9. doi: 10.3109/00016489.2010.534114
52. Naganawa S, Yamazaki M, Kawai H, Bokura K, Sone M, Nakashima T. Visualization of endolymphatic hydrops in Meniere's disease after single- dose intravenous gadolinium-based contrast medium: timing of optimal enhancement. Magn Reson Med Sci. (2012) 11:43–51. doi: 10.2463/mrms.11.43
53. Sano R, Teranishi M, Yamazaki M, Isoda H, Naganawa S, Sone M, et al. Contrast enhancement of the inner ear in magnetic resonance images taken at 10 minutes or 4 hours after intravenous gadolinium injection. Acta Otolaryngol (Stockh). (2012) 132:241–6. doi: 10.3109/00016489.2011.639085
54. Berrettini S, Seccia V, Fortunato S, Forli F, Bruschini L, Piaggi P, et al. Analysis of the 3-dimensional fluid-attenuated inversion-recovery (3D-FLAIR) sequence in idiopathic sudden sensorineural hearing loss. JAMA Otolaryngol– Head Neck Surg. (2013) 139:456–64. doi: 10.1001/jamaoto.2013.2659
55. Ishikawa K, Haneda J, Okamoto K. Decreased vestibular signal intensity on 3D-FIESTA in vestibular schwannomas differentiating from meningiomas. Neuroradiology. (2013) 55:261–70. doi: 10.1007/s00234-012-1100-2
56. Kim TY, Park DW, Lee YJ, Lee JY, Lee SH, Chung JH, et al. Comparison of inner ear contrast enhancement among patients with unilateral inner ear symptoms in mr images obtained 10 minutes and 4 hours after gadolinium injection. AJNR Am J Neuroradiol. (2015) 36:2367–72. doi: 10.3174/ajnr.A4439
57. Tanigawa T, Shibata R, Tanaka H, Gosho M, Katahira N, Horibe Y, et al. Usefulness of three-dimensional fluid-attenuated inversion recovery magnetic resonance imaging to detect inner-ear abnormalities in patients with sudden sensorineural hearing loss. J Laryngol Otol. (2015) 129:11–5. doi: 10.1017/S0022215114003028
58. Liao W-H, Wu H-M, Wu H-Y, Tu T-Y, Shiao A-S, Castillo M, et al. Revisiting the relationship of three-dimensional fluid attenuation inversion recovery imaging and hearing outcomes in adults with idiopathic unilateral sudden sensorineural hearing loss. Eur J Radiol. (2016) 85:2188–94. doi: 10.1016/j.ejrad.2016.10.005
59. Lombardo F, Cori S, Aghakhanyan G, Montanaro D, Marchi D, Frijia F, et al. 3D-Flair sequence at 3T in cochlear otosclerosis. Eur Radiol. (2016) 26:3744–51. doi: 10.1007/s00330-015-4170-9
60. Naganawa S, Kawai H, Taoka T, Suzuki K, Iwano S, Satake H, et al. Cochlear lymph fluid signal increase in patients with otosclerosis after intravenous administration of gadodiamide. Magn Reson Med Sci. (2016) 15:308–15. doi: 10.2463/mrms.mp.2015-0121
61. Pakdaman MN, Ishiyama G, Ishiyama A, Peng KA, Kim HJ, Pope WB, et al. Blood-labyrinth barrier permeability in meniere disease and idiopathic sudden sensorineural hearing loss: findings on delayed postcontrast 3D-FLAIR MRI. AJNR Am J Neuroradiol. (2016) 37:1903–8. doi: 10.3174/ajnr.A4822
62. Attye A, Eliezer M, Galloux A, Pietras J, Tropres I, Schmerber S, et al. Endolymphatic hydrops imaging: differential diagnosis in patients with Meniere disease symptoms. Diagn Interv Imaging. (2017) 98:699–706. doi: 10.1016/j.diii.2017.06.002
63. Berrettini S, Lombardo F, Bruschini L, Ciabotti A, Raffaello C, Sara DC, et al. 3D fluid attenuated inversion recovery (FLAIR) magnetic resonance imaging at different stages of otosclerosis. Eur Arch Oto-Rhino-Laryngol. (2018) 275:2643–52. doi: 10.1007/s00405-018-5093-2
64. Byun H, Chung JH, Lee SH, Park CW, Park DW, Kim TY. Clinical value of 4-hour delayed gadolinium-Enhanced 3D FLAIR MR Images in Acute Vestibular Neuritis. Laryngoscope. (2018) 128:1946–51. doi: 10.1002/lary.27084
65. Eliezer M, Poillon G, Gillibert A, Horion J, Cruypeninck Y, Gerardin E, et al. Comparison of enhancement of the vestibular perilymph between gadoterate meglumine and gadobutrol at 3-Tesla in Meniere's disease. Diagn Interv Imaging. (2018) 99:271–7. doi: 10.1016/j.diii.2018.01.002
66. Bernaerts A, Vanspauwen R, Blaivie C, van Dinther J, Zarowski A, Wuyts FL, et al. The value of four stage vestibular hydrops grading and asymmetric perilymphatic enhancement in the diagnosis of Menière's disease on MRI. Neuroradiology. (2019) 61:421–9. doi: 10.1007/s00234-019-02155-7
67. Conte G, Lo Russo F, Caschera L, Zanetti D, Castorina P, Sina C, et al. Audiovestibular Phenotypes and Advanced Magnetic Resonance Imaging Features of Cochlin Gene Mutation Carriers. Audiol Neurootol. (2019) 24:166–73. doi: 10.1159/000501292
68. Eliezer M, Maquet C, Horion J, Gillibert A, Toupet M, Bolognini B, et al. Detection of intralabyrinthine abnormalities using post-contrast delayed 3D-FLAIR MRI sequences in patients with acute vestibular syndrome. Eur Radiol. (2019) 29:2760–9. doi: 10.1007/s00330-018-5825-0
69. Wang J, Ren T, Sun W, Liang Q, Wang W. Post-contrast 3D-FLAIR in idiopathic sudden sensorineural hearing loss. Eur Arch Oto-Rhino-Laryngol. (2019) 276:1291–9. doi: 10.1007/s00405-019-05285-z
70. Bowen AJ, Carlson ML, Lane JI. Inner Ear Enhancement With Delayed 3D-FLAIR MRI Imaging in Vestibular Schwannoma. Otol Neurotol. (2020) 41:1274–9. doi: 10.1097/MAO.0000000000002768
71. Eliezer M, Hautefort C, Van Nechel C, Duquesne U, Guichard J-P, Herman P, et al. Electrophysiological and inner ear MRI findings in patients with bilateral vestibulopathy. Eur Arch Oto-Rhino-Laryngol. (2020) 277:1305–14. doi: 10.1007/s00405-020-05829-8
72. Eliezer M, Poillon G, Levy D, Guichard J-P, Toupet M, Houdart E, et al. Clinical and radiological characteristics of patients with collapse or fistula of the saccule as evaluated by inner ear MRI. Acta Otolaryngol (Stockh). (2020) 140:1–8. doi: 10.1080/00016489.2020.1713396
73. Kahn L, Hautefort C, Guichard J-P, Toupet M, Jourdaine C, Vitaux H, et al. Relationship between video head impulse test, ocular and cervical vestibular evoked myogenic potentials, and compartmental magnetic resonance imaging classification in meniere's disease. Laryngoscope. (2020) 130:E444–52. doi: 10.1002/lary.28362
74. Laine J, Hautefort C, Attye A, Guichard J-P, Herman P, Houdart E, et al. MRI evaluation of the endolymphatic space in otosclerosis and correlation with clinical findings. Diagn Interv Imaging. (2020) 101:537–45. doi: 10.1016/j.diii.2020.03.009
75. Ishiyama G, Lopez IA, Ishiyama P, Vinters HV, Ishiyama A. The blood labyrinthine barrier in the human normal and Meniere's disease macula utricle. Sci Rep. (2017) 7:253. doi: 10.1038/s41598-017-00330-5
Keywords: otosclerosis, Ménière's disease, inner ear, blood-labyrinth barrier, gadolinium, MRI
Citation: Song CI, Pogson JM, Andresen NS and Ward BK (2021) MRI With Gadolinium as a Measure of Blood-Labyrinth Barrier Integrity in Patients With Inner Ear Symptoms: A Scoping Review. Front. Neurol. 12:662264. doi: 10.3389/fneur.2021.662264
Received: 31 January 2021; Accepted: 16 April 2021;
Published: 20 May 2021.
Edited by:
Stefan K. Plontke, Martin Luther University of Halle-Wittenberg, GermanyReviewed by:
Jeremy Hornibrook, University of Canterbury, New ZealandAndreas Deistung, Universitätsklinikum Halle, Germany
Copyright © 2021 Song, Pogson, Andresen and Ward. This is an open-access article distributed under the terms of the Creative Commons Attribution License (CC BY). The use, distribution or reproduction in other forums is permitted, provided the original author(s) and the copyright owner(s) are credited and that the original publication in this journal is cited, in accordance with accepted academic practice. No use, distribution or reproduction is permitted which does not comply with these terms.
*Correspondence: Bryan K. Ward, YndhcmQxNUBqaC5lZHU=