- 1Department of Rehabilitation Medicine, Hanchuan People's Hospital, Hanchuan, China
- 2Department of Pharmacy, Hanchuan People's Hospital, Hanchuan, China
Stroke is a major cause of death and disability in adults. Conventional therapy (CT) has limited effectiveness, and therefore, various virtual reality (VR) rehabilitation programs have been designed. However, their efficacy in regaining motor function in patients with subacute stroke is questionable. Therefore, we conducted this meta-analysis to determine the efficacy of VR, compared to CT, in restoring motor function in this patient population. Up to October 10, 2020, nine electronic databases were searched for relevant articles reporting the effectiveness of VR in regaining motor function in patients with subacute stroke. This search was updated on March 7, 2021, with no additional added articles. The control group included CT, physical therapy, occupational therapy, or a combination of them. Effectiveness is defined as the positive change from baseline values to the last follow-up point. The Cochrane's revised risk-of-bias tool was used to determine the quality of included trials. A metaregression analysis was conducted to determine the effect of “time since last stroke” on reported outcomes. Publication bias and sensitivity analyses were also carried out. A total of 19 studies (17 randomized controlled trials, 1 cohort study, and 1 crossover trial) were included in the qualitative analysis, whereas 16 trials were meta-analyzed. A great improvement in motor function was noted in the VR group, when compared to preintervention values [standardized mean difference (SMD) = 1.14; 95% confidence interval (CI) = 0.77–1.52; I2 = 82%; P < 0.001]. When compared to CT, VR resulted in mild improvement in motor function (SMD = 0.47; 95% CI = 0.22–0.72; I2 = 75%; P < 0.001). However, upon trim-and-fill adjustment, this finding was deemed insignificant (SMD = 0.08; 95% CI = −0.16 to 0.33; I2 = 82.6%; P < 0.001). Ten studies had low risk, five had some concerns, three had high risk, and one had a moderate risk of bias. VR programs can be used jointly with CT for the rehabilitation of the motor function of patients with subacute stroke. However, more studies are still warranted to determine the effectiveness of these interventions in retaining the cognitive function and physical performance of such patients.
Introduction
Stroke is a major cause of mortality and acquired disabilities in the adult population (1, 2). Recently, the risk of stroke-related mortality has been greatly reduced because of the improved accessibility to healthcare and stroke management protocols, which include recanalization therapy (3), decompression therapy (4), and stroke unit management (5). However, there is a remarkable increase in the number of neurologically impaired patients with significant disabilities (6). Of whom, only a few may regain some functionality in affected upper limb (UL) or lower limb (LL) (7, 8). This, in turn, will greatly impact the affected individuals' ability to self-care and their engagement in social activities. As most activities of daily living (ADLs) involve the use of ULs and LLs, it is of great importance to improve their functional state in post stroke patients.
In clinical practice, post stroke rehabilitation currently depends mainly on promoting neuroplasticity after brain injury (9, 10). In an attempt to maximize the effect of neuroplasticity, training must be based on learning repetitive, challenging, and motivating, as well as intense tasks (11, 12). In this context, conventional therapies (CTs), in the form of occupational or physical therapy, are commonly used to improve the motor function of affected limbs following brain injury (13–15). However, researchers have been studying other treatment options because conventional rehabilitation programs are often time-consuming and resource-intensive, and their outcomes rely mainly on the ability and prior training of the interventionist. Therefore, virtual reality (VR) has gained attention in the past decades for its potential benefits in promoting motor recovery in stroke survivors. Moreover, it has been reported that repetition, intensity, and dose in CT settings are not sufficient to reach plasticity-based optimal motor recovery (16). The aforementioned limitations drove the introduction of new options of potential benefit in regaining the motor function in affected individuals, such as VR.
VR-based interventions are now used as therapeutic options for promoting neurorehabilitation in patients with stroke, enabling patients to perform their daily activities, which are difficult to be carried out in a rehabilitative facility. Also, compared to the standard CT, VR therapy is designed to offer entertainment and joy, and therefore, it encourages patients to participate more in the rehabilitative program (17, 18). Moreover, VR programs can be carried out in clinical settings and at a low cost, and therefore, many patients can comply with them.
VR training therapy has been increasingly used in order to facilitate motor recovery in stroke survivors, and the majority of published articles investigated patients in the chronic stage. They also have shown that the use of VR is beneficial in this regard. For example, a recent systematic review and meta-analysis concluded that VR therapy could moderately improve the motor function of both ULs and LLs in patients with chronic stroke in comparison with CTs (19). Moreover, compared to CT, VR has been shown to result in a positive impact on balance as well (20).
That being said, the number of studies investigating the effect of VR-based interventions in patients with subacute stroke remains scarce (9, 21–23), and no conclusions have been reached in this regard. This topic is of great interest because (1) the plasticity of the brain remains good during the acute and subacute phase (24, 25) and (2) the effectiveness of rehabilitation therapy–induced neuroplasticity is limited in chronic stroke patients, particularly those who missed the window of opportunity that is present during the subacute phase (when the brain plasticity peaks) (26).
Therefore, we conducted the current investigation to systematically review the available high-quality evidence in the literature regarding the use of VR rehabilitation interventions, compared to CTs, in regaining the functional state of affected limbs among patients with subacute stroke.
Materials and Methods
Search Strategy and Study Selection
The study process was conducted following the accepted methodology recommendations of the PRISMA checklist for systematic review and meta-analysis where registration of the protocol is not mandated (27). An electronic database search was conducted for relevant studies published from inception until October 10, 2020, in nine databases: PubMed, Google Scholar, Scopus, Web of Science, The New York Academy of Medicine, Virtual health library, the System for Information on Gray Literature in Europe, ClinicalTrials.gov, and meta Register of Controlled Trials. Of note, this search was updated on March 7, 2021, to include any recently published relevant articles. However, no additional articles were found eligible.
For the purposes of conducting this research, we used the definition of the subacute phase of stroke as the period from 7 days to 6 months following the occurrence of stroke, as highlighted in the recent consensus of the Stroke Recovery and Rehabilitation Roundtable Taskforce (28). The search was conducted using the following keywords: (subacute stroke OR stroke) AND (virtual reality) AND (trial OR RCT or random OR randomized) and/or medical subject (MeSH) terms, as appropriate. We further did a manual search of references in our included articles to avoid missing relevant studies (29, 30). The search process was done based on the PICO framework: participants were any patient with subacute stroke (as defined above) affecting the motor function of upper or lower extremities, the interventions were VR-based therapies, the comparison was CTs, and treatment effectiveness was the outcome of interest. “Conventional therapy” could consist of usual CT, physical therapy, occupational therapy or a combination of any of them. “Effectiveness” was defined as the positive change from baseline values to the last follow-up point. The change in motor function was our primary outcome of interest; in the case of multiple scales, effectiveness was measured by the change in the scale that was reported as the primary outcome or most relevant to other studies (to maintain homogeneity). We also aimed to highlight the feasibility of using different VR programs in patients with subacute stroke.
We included all original studies that assessed the effectiveness of different VR programs in subacute stroke patients. However, articles were excluded according to the following exclusion criteria: (1) nonoriginal studies or nonhuman (in vitro or animal) studies; (2) duplicate records, overlapped data, or when data could not be reliably extracted; (3) incomplete reports; and (4) abstract-only articles, reviews, thesis, books, conference papers, or articles without available full texts.
The title and abstract screening were performed by four independent reviewers. Then, three independent reviewers performed a full-text screening to ensure the inclusion of relevant articles in our systematic review. Any disagreement was resolved by discussion and referring to the senior author when necessary.
Data Extraction
Two authors developed the data extraction sheet using the Microsoft Excel software. Data extraction was performed by three independent reviewers using the Excel sheet. The fourth independent reviewer performed data checking to ensure the accuracy of extracted data. All disagreements and discrepancies were resolved by discussion and consultation with the senior author when necessary.
Risk of Bias
Three independent reviewers evaluated the risk of bias of included studies. For randomized controlled trials, we decided to use Cochrane's revised quality assessment tool (ROB-II) (31). For non-randomized studies, the risk of bias in non-randomized studies of interventions (ROBINS-I) was used to assess the quality of included studies (32). Any discrepancy between the reviewers was solved by discussion.
Statistical Analysis
All data were analyzed using the R software, version 4.0.2 (33). The standardized mean difference (SMD) effect size and its variance were calculated using the preintervention and postintervention data for both intervention and control groups (34, 35). Using a “meta” package, changes from baseline for both intervention and control were analyzed to calculate the pooled SMD and the corresponding standard errors (36). The SMD was used because of the difference in measurement methodology among included studies, and it is more generalizable than the mean difference (37).
The related 95% confidence intervals (CIs) of the computed effect size were calculated using a fixed- or random-effects model based on the extent of heterogeneity. To assess heterogeneity, Q statistics and I2 test were used, with I2 value higher than 50% or P < 0.05 considered significant (38). Moreover, we conducted Egger regression test to assess publication bias, which was considered significant when P < 0.10 (39, 40). Whenever publication bias was found, the trim-and-fill method of Duvall and Tweedie was applied to add studies that appeared to be missing (41) to enhance the symmetry. Furthermore, metaregression was used to explore the effect of “time passed since the last stroke” on the VR effectiveness (42). In the case of statistically significant results, a leave-one-out sensitivity analysis was performed by iteratively removing one study at a time to confirm that our findings were not driven by any single study (43).
Results
Search Results
We identified 861 records after excluding 202 duplicates using the Endnote X9 software. Title and abstract screening resulted in 88 records for further full-text screening. No articles were added after performing a manual search. Finally, a total of 19 studies were included in the systematic review, and 16 articles were eligible for meta-analysis (Figure 1).
Study Characteristics
Nineteen studies compared VR vs. controls. According to the countries in which the included trials were conducted, four studies were conducted in Turkey, four in Korea, two in Norway, two in Italy, two in Australia, two in Canada, two in China, and one in Germany. Based on the design of included studies, 17 articles were randomized controlled trials, one was a cohort study, and the last one had a crossover design (Table 1).
Risk of Bias
The overall risk of bias was low in nine studies, whereas five studies had some concerns, and three had a high risk of bias (Figure 2). For non-randomized trials, the risk of bias was moderate in one of them and low in the other (Figure 3).
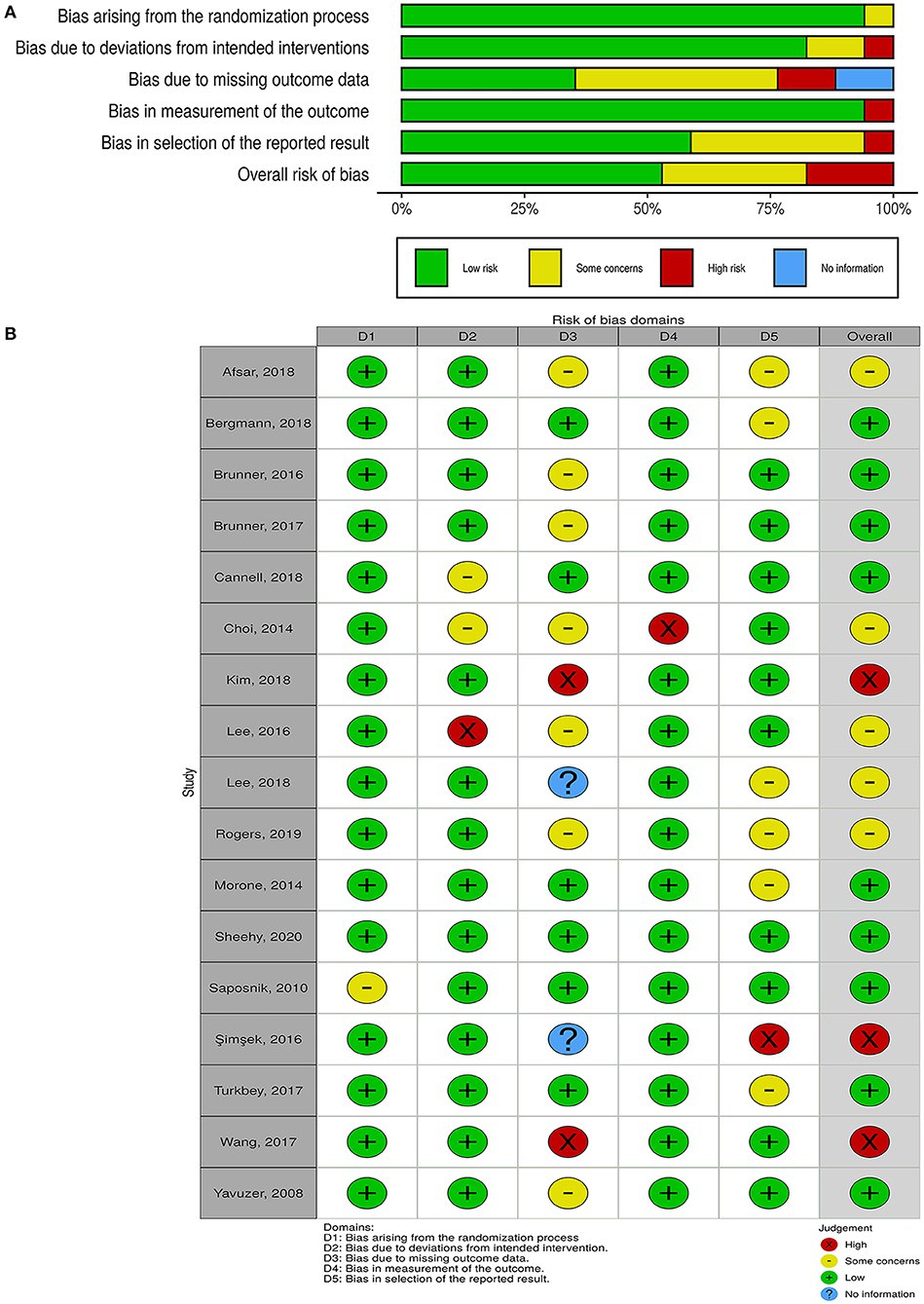
Figure 2. Quality of the included randomized controlled trials. (A) Risk-of-bias graph: review authors' judgments about each risk-of-bias item presented as percentages across all included studies. (B) Risk-of-bias summary: review authors' judgments about each risk-of-bias item for each included study.
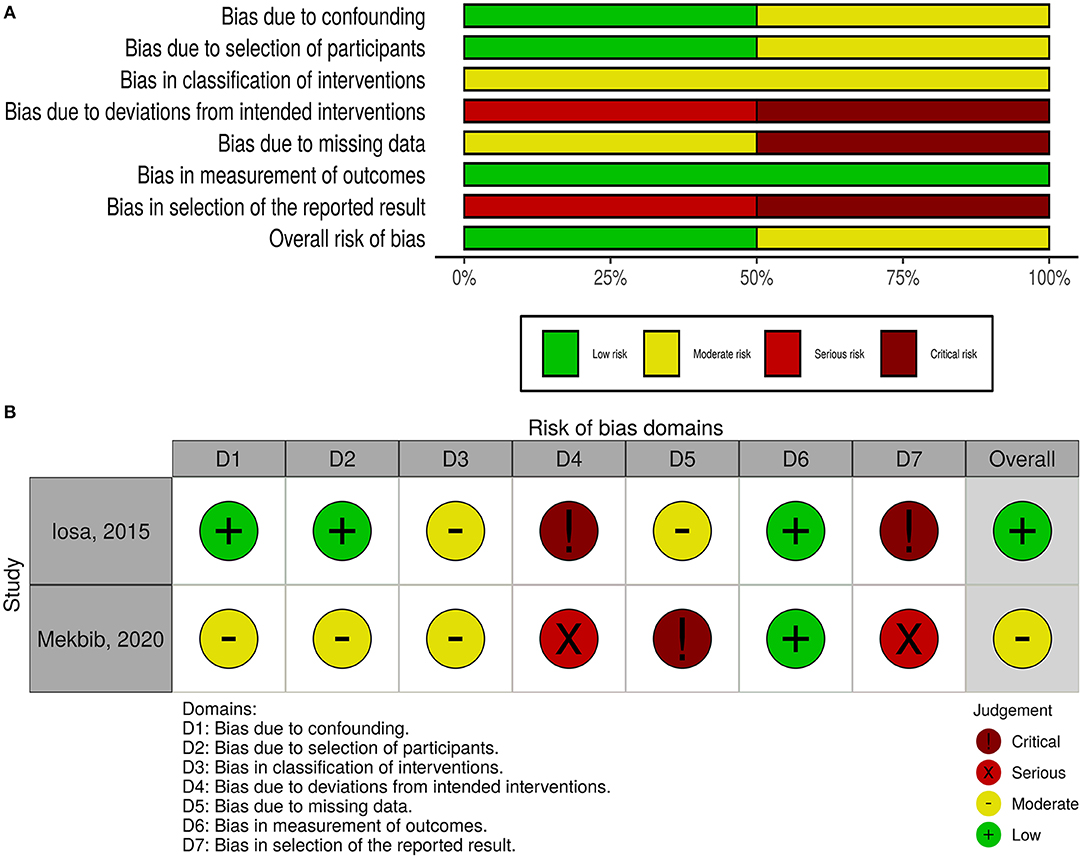
Figure 3. Quality of the included non-randomized controlled trials. (A) Risk-of-bias graph: review authors' judgments about each risk-of-bias item presented as percentages across all included studies. (B) Risk-of-bias summary: review authors' judgments about each risk-of-bias item for each included study.
Effectiveness of VR Therapy
The details of VR-based interventions and the control groups are provided in Table 2. Sixteen studies evaluating the effectiveness of VR therapy (preintervention vs. post intervention) were included in the analysis. There was a significant improvement in motor function score following VR therapy in patients with subacute stroke, compared to their preintervention score (SMD = 1.14; 95% CI = 0.77–1.52; P < 0.001). According to Egger regression test, there was no significant risk of bias among included studies (P = 0.275); however, significant heterogeneity was present (I2 = 82% and P < 0.001) (Figure 4). The contribution of each study to the overall heterogeneity is presented in Supplementary Figure 1. Moreover, the leave-one-out sensitivity analysis did not affect the significance of the overall effect size, indicating that the findings were not driven by any single study Supplementary Figure 2. The meta regression analysis did not show any significant effect of the post stroke duration (days) on the treatment effects (P = 0.230) (Supplementary Figure 3).
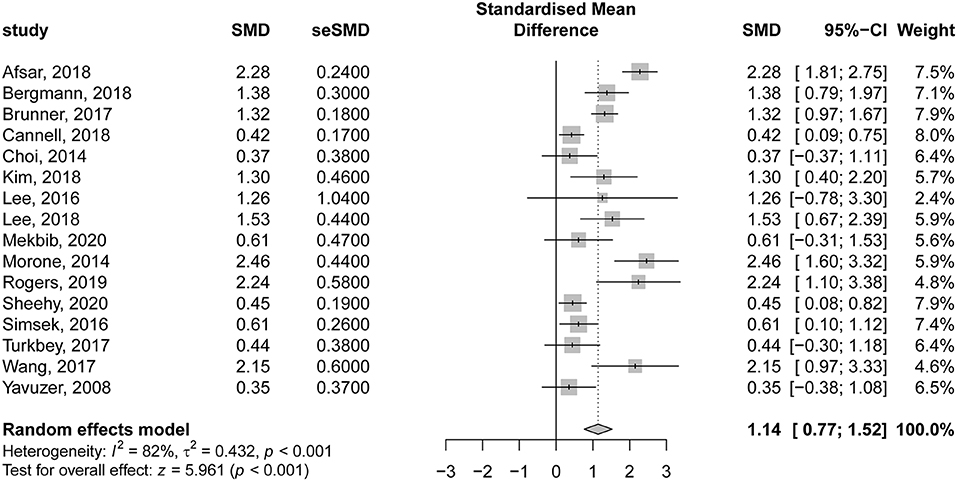
Figure 4. Forest plot for the effectiveness of virtual reality–based rehabilitation of motor function in subacute stroke patients (preintervention vs. postintervention).
In the same context, 15 studies evaluating the comparative effectiveness of VR therapy and CTs were included in the analysis. There was a significant improvement in motor function following VR therapy in patients with subacute stroke, compared to those undergoing CT (SMD = 0.47; 95% CI = 0.22–0.72; P < 0.001). Nevertheless, there was significant heterogeneity among the included studies (I2 = 75% and P < 0.001) (Figure 5). The contribution of each study to the overall heterogeneity is presented in Supplementary Figure 4. The leave-one-out sensitivity analysis did not affect the significance of the overall effects indicating that the findings were not driven by a single study (Supplementary Figure 5).
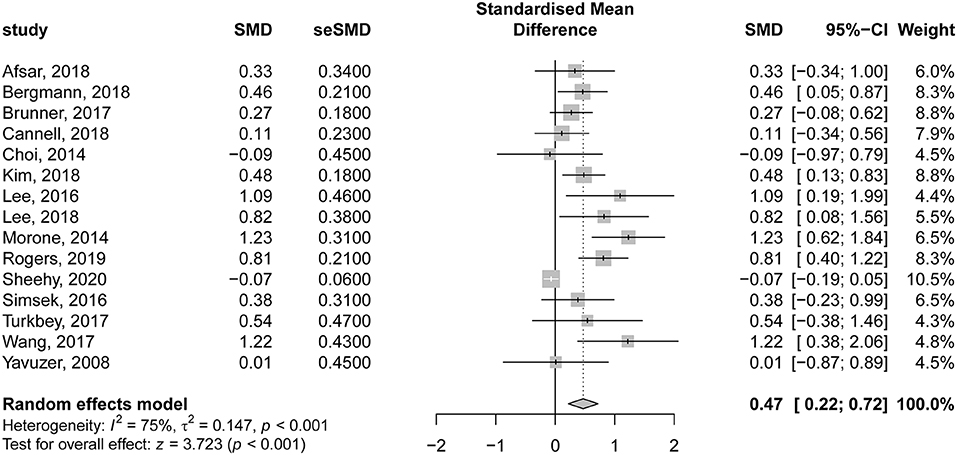
Figure 5. Forest plot for the comparative effectiveness of virtual reality–based rehabilitation of motor function vs. conventional therapy in subacute stroke patients.
Noteworthy, according to Egger regression test, there was a significant risk of bias among the included studies (P = 0.001). Therefore, we used the trim-and-fill method to “trim” the studies that caused the asymmetry of the funnel plot so that the overall effect estimate would be minimally affected by publication bias and then to “fill” imputed missing studies in the plot according to the bias-corrected overall effect estimate. Unfortunately, upon using this method, the overall effect estimate was deemed insignificant (SMD = 0.08; 95% CI = −0.16–0.33; P = 0.507), with a higher heterogeneity (I2 = 82.6% and P < 0.001) (Figure 6). This indicates that VR therapy is not associated with significant improvement in motor function as compared with CT. The meta regression analysis did not show any significant effect of the post stroke duration (days) on the treatment effects (P = 0.413) (Supplementary Figure 6).
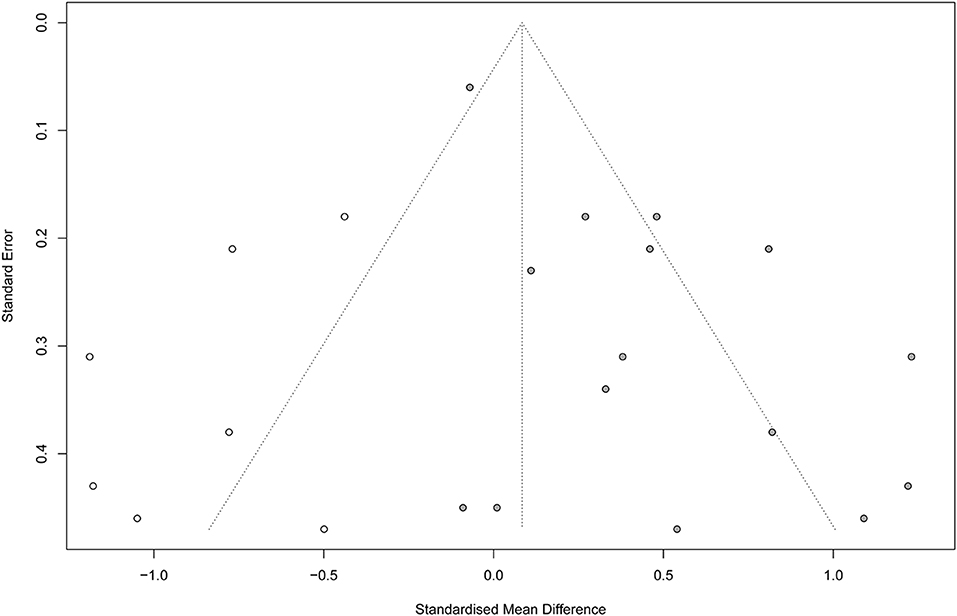
Figure 6. Funnel plot with trim-and-fill method. Number of studies combined: k = 22 (with seven added studies).
Discussion
The field of neurorehabilitation after stroke, especially during the subacute phase, is still evolving. The current paradigms of neurorehabilitation strategies in order to enhance the motor function of affected extremities are focused on high-intensity, task-specific interventions with the main character of repetition (58, 59). The repetition of such programs or tasks potentiates the acquisition of new along with the retrieval of previous motor functions of affected limbs, leading to long-term potentiation. VR game–based programs are a group of interventions that are directed to incorporate affected populations in a computer-simulated environment while giving them almost real-time feedback on their performance. According to previous evidence (59, 60), the degree to which VR programs can aid or facilitate current CT programs is still to be determined.
We conducted this meta-analysis to determine the comparative effectiveness of VR-based systems in the rehabilitation of the motor function of affected limbs in patients with subacute stroke. We aimed to investigate the effect of VR on restoring the motor function of both UL and LL; however, this was not possible because the majority of included studies investigated the effect of VR on the motor function of the upper limbs, whereas only two studies recruited patients with hemiparesis with no description of which limb was investigated. Therefore, we reported the change in the motor function of affected limbs, with no discrimination, following VR-based therapy as compared to CT. The CT group included various programs: physical therapy, occupational therapy, functional electrical stimulation, or a combination of them.
In our study, we noted that VR interventions resulted in a great improvement in motor function of affected limbs in patients with subacute stroke compared to preintervention values (SMD = 1.14; 95% CI = 0.77–1.52). However, we detected significant considerable heterogeneity, which on further analysis revealed that the study of Afsar et al. (45) was the major contributor to the resultant heterogeneity. This could be related to their heterogeneous population compared to other studies. They included patients with mild to moderate upper limb motor deficits, and they were further subdivided into two groups (VR vs. conventional). Furthermore, patients in both groups received CT. Also, patients in the VR group received mild training of 30 min per day 5 days a week for 4 weeks using the Xbox Kinect gaming console in addition to CT of 60 min five times a week for 4 weeks. The aforementioned factors could contribute to the observed heterogeneity. Furthermore, the sensitivity analysis revealed that the effect size “great improvement” was not driven by a single study. The variable “time since last stroke” had no significant effect on the outcomes.
Upon comparing VR programs to CT, our analysis revealed a significant mild improvement (0.5 ≤ SMD ≤ 0.8) in the motor function of the affected limb in favor of VR-based rehabilitation programs. We observed considerable heterogeneity, and the study of (61) was the major contributor. This could be explained by the fact that both groups (VR and control group) in the previous study had VR training of the upper limb in addition to their assigned interventions. Surprisingly, following the trim-and-fill adjustment of risk of bias, the observed results were deemed insignificant (SMD = 0.08; 95% CI = −0.16 to 0.33), indicating no significant difference between both interventions on restoring the motor function of affected limbs. Our findings are comparable to the systematic review and meta-analysis of Laver et al. (62), who assessed the efficacy of VR in improving upper limb motor function in patients with stroke. A significant but mild improvement (SMD = 0.28) in motor function was noted in favor of VR-based interventions. Noteworthy, only 2 of the 12 analyzed studies recruited patients with subacute stroke (9, 12). Therefore, our study provides stronger evidence in regard to patients with subacute stroke, indicating that VR does not result in significant improvement in motor function. In 2019, an umbrella review of meta-analyses was conducted to determine the impact of various neurorehabilitation interventions on changes in ADLs in patients with subacute stroke (63). A total of 55 meta-analyses were investigated, reporting 21 subacute rehabilitation interventions. Of investigated interventions, VR was reported by two meta-analyses (62, 64) and resulted in mild to moderate improvement of ADLs in the subacute phase of stroke rehabilitation. However, the authors highlighted that the lack of high-quality evidence in analyzed meta-analyses highlights the need for more research. In our review, the majority of studied interventions were designed mainly to improve motor function rather than other outcomes, including cognitive function, activity performance, ADLs, or feasibility. As for rehabilitation of motor function, it was reported that constraint-induced motor therapy is considered, by far, the most promising intervention in patients with stroke, in general, based on the findings of a recent systematic review (59).
The feasibility of VR-based rehabilitative interventions was assessed in three trials. In the crossover trial of Iosa et al. (57), four elderly patients with subacute stroke were allocated to receive six sessions of 30 min of leap motion controller–based intervention in addition to CT. Participation in these sessions was excellent in three patients and very good in the remaining patient. This highlights the feasibility of this intervention for neurorehabilitation in this patient group due to the easiness of its use without the need for the subject to stand alone. However, because of the limited number of included participants, these conclusions still need confirmation by larger, well-conducted trials. In the pilot, randomized controlled trial of (12), patients were allocated to receive Nintendo Wii gaming (nine patients) or recreational therapy in the form of playing cards, bingo, or Jenga (eight patients). Feasibility was reflected by the total time of intervention receipt. The mean total time of VR was comparable to that of recreational therapy (388 vs. 364 min; P = 0.75). Meanwhile, Brunner et al. (56) recorded 50 videos of patients with subacute stroke who were allocated to receive either VR or CT, and the authors reported higher feasibility in the VR group, with higher mean time of active practice (77.6 min) compared to the CT group (67.3 min). Of note, the fact that patients knew they were being recorded affects the validity and interpretation of this finding. Therefore, despite the promising feasibility of VR-based interventions for the neurorehabilitation in patients with subacute stroke, more robust trials of larger sample sizes are still warranted to reach a more definitive conclusion.
In view of the paucity of well-designed randomized controlled trials, the limited funding for stroke rehabilitation programs and research, and the limitations of CT, VR interventions can be used adjunctly with CT for the following reasons: (1) VR-based interventions are accessible for all patients with subacute stroke, (2) the low cost of VR programs, and (3) it requires no special resources or assistance. Therefore, we hypothesize that VR interventions might potentiate motor rehabilitation in subacute stroke patients when added to CT. However, this finding remains inconclusive for the following reasons.
First, a wide variety of VR programs were reported. Each of these programs included different activities aiming to improve the function of certain parts of the affected limb. For example, the VR program in the study of (45) included the active abduction, adduction, flexion, and extension of the shoulder in addition to flexion and extension of the elbow. Meanwhile, Choi et al. (47) focused on regaining the motor function of affected ULs by promoting certain activities in their VR program, including extension and internal and external rotation of the shoulder; flexion and extension of the elbow; and pronation and supination of the forearm. On the other hand, another trial focused on regaining more delicate motor functions, and therefore the VR programs were more focused on improving the delicate movements of the fingers (promoting the development of pinching and grasping), digits (improving their dexterity and coordination), and hand (enhancing flexion and extension) (51). Other VR programs were primarily used to regain arm motions, such as reaching, wrist extension, and hand grasping and releasing (22).
Another point worth mentioning is the differences in VR programs among included trials. Typically, VR interventions include a head-mounted display, which allows users to experience 3D content (either videos or games) in an immersive virtual environment. In the case of VR interventions in stroke patients with affected ULs, leap motions are usually used in order to track the user's movements. Three of the included trials in our review used this method in their treatment protocol to regain the motor function of affected limbs in patients with subacute stroke (44, 51, 57). However, the remaining studies used the terms “virtual reality intervention” and “game-based intervention” interchangeably. For instance, their treatment protocols incorporated the use of different gaming-based engines or systems, such as the Xbox Kinect system (45, 52), Unity 3D game engine (22), Nintendo Wii Sports Resort package (12, 21, 23, 47, 53), the YouGrabber system (56), Jintronix Rehabilitation system (49), and PlayStation console (50).
Of note, the intensity of the VR intervention was widely variable among included studies. For example, in the study of Brunner et al. (56), a total of 62 patients with subacute stroke underwent VR therapy using the YouGrabber gaming system. The VR therapy lasted for 1 h each and was performed four to five times a week for 12 consecutive weeks. On the other hand, the intensity of the VR intervention was low in the study of (55), where patients with subacute stroke underwent video game–based VR therapy sessions that lasted 20 min each. These sessions were repeated three times per week for a total of 4 consecutive weeks. Therefore, we could not determine the effect of VR intensity on our outcome of interest.
The aforementioned points reflect the heterogeneity of studied populations at baseline, which could also explain the reason for encountering the highly significant considerable heterogeneity in our analyses.
Although our study is the first study to investigate the efficacy of VR interventions in restoring the motor function of patients with subacute stroke, several limitations were encountered. First, most included studies compared VR programs in addition to CT compared to CT alone, which subsequently accounted for more rehabilitation time in the experimental group. This could potentially account for a bias in favor of VR therapy because both the intensity and frequency of rehabilitation, per se, are known to be directly correlated with beneficial functional outcomes. Second, the number of participants in included studies was small, ranging from 4 to 120 patients, and thus, the generalizability of our findings could be affected. Third, eight randomized controlled trials had some concerns and a high risk of bias. Based on these limitations, we recommend conducting more robust, well-designed trials of larger sample sizes and longer follow-up periods to reach more definitive conclusions. Fourth, outcomes were assessed directly after the application of the VR interventions with a short follow-up period, ranging from 2 to 12 weeks (44, 56). Therefore, we are not confident that the improvement in motor function following the implementation of VR protocols is long-lasting. Eventually, our systematic review was conducted mainly to determine the effect of VR intervention on the motor function of patients with subacute stroke. Only two articles reported outcomes related to the cognitive function of subacute stroke patients following VR therapy; however, these studies used different measurement tools with different cutoff values for defining cognitive impairment (47, 54). Therefore, it was inapplicable to conduct a meta-analysis on this outcome, and thus, more research is needed to determine the impact of these VR interventions on other outcomes, such as cognitive function, ADLs, and quality of life.
VR provides a great improvement in motor function in patients with subacute stroke, compared to the preintervention state. However, when compared to CT, mild to no significant improvement in motor function was noted. Therefore, VR can be used as an adjuvant to CT in restoring the motor function of affected limbs. That being said, more studies are still warranted to reach a more definitive conclusion and to investigate the effect of VR on the cognitive function and physical performance of affected patients.
Data Availability Statement
The original contributions presented in the study are included in the article/Supplementary Material, further inquiries can be directed to the corresponding authors.
Author Contributions
Q-cP and LY wrote the manuscript. Q-cP, LY, and YC collected and analyzed the data. All authors contributed to the article and approved the submitted version.
Conflict of Interest
The authors declare that the research was conducted in the absence of any commercial or financial relationships that could be construed as a potential conflict of interest.
Supplementary Material
The Supplementary Material for this article can be found online at: https://www.frontiersin.org/articles/10.3389/fneur.2021.639535/full#supplementary-material
Supplementary Figure 1. Baujat plot showing the contribution of each study into the overall heterogeneity.
Supplementary Figure 2. Leave-one-out sensitivity analysis for the effectiveness of virtual reality–based rehabilitation (preintervention vs. postintervention).
Supplementary Figure 3. Metaregression of the poststroke duration and virtual reality–based rehabilitation effectiveness (preintervention vs. postintervention).
Supplementary Figure 4. Baujat plot showing the contribution of each study into the overall heterogeneity.
Supplementary Figure 5. Leave-one-out sensitivity analysis for the effectiveness of virtual reality–based rehabilitation (VR vs. conventional therapy).
Supplementary Figure 6. Metaregression of the poststroke duration and virtual reality–based rehabilitation effectiveness (VR vs. conventional therapy).
References
1. Feigin VL, Forouzanfar MH, Krishnamurthi R, Mensah GA, Connor M, Bennett DA, et al. Global and regional burden of stroke during 1990-2010: findings from the global burden of disease study 2010. Lancet. (2014) 383:245–54. doi: 10.1016/S0140-6736(13)61953-4
2. Mozaffarian D, Benjamin EJ, Go AS, Arnett DK, Blaha MJ, Cushman M, et al. Heart disease and stroke statistics-2016 update: a report from the american heart association. Circulation. (2016) 133:e38–360. doi: 10.1161/CIR.0000000000000350
3. Pikija S, Magdic J, Sztriha LK, Killer-Oberpfalzer M, Bubel N, Lukic A, et al. Endovascular therapy for tandem occlusion in acute ischemic stroke: intravenous thrombolysis improves outcomes. J Clin Med. (2019) 8:228. doi: 10.3390/jcm8020228
4. Slezins J, Keris V, Bricis R, Millers A, Valeinis E, Stukens J, et al. Preliminary results of randomized controlled study on decompressive craniectomy in treatment of malignant middle cerebral artery stroke. Medicina. (2012) 48:76. doi: 10.3390/medicina48100076
5. Jung S, Stapf C, Arnold M. Stroke unit management and revascularisation in acute ischemic stroke. Eur Neurol. (2015) 73:98–105. doi: 10.1159/000365210
6. Baumann M, Lurbe-Puerto K, Alzahouri K, Aïach P. Increased residual disability among poststroke survivors and the repercussions for the lives of informal caregivers. Topics Stroke Rehabil. (2011) 18:162–71. doi: 10.1310/tsr1802-162
7. Choo PL, Gallagher HL, Morris J, Pomeroy VM, Van Wijck F. Correlations between arm motor behavior and brain function following bilateral arm training after stroke: a systematic review. Brain Behav. (2015) 5:e00411. doi: 10.1002/brb3.411
8. Veerbeek JM, Winters C, Van Wegen EEH, Kwakkel G. Is the proportional recovery rule applicable to the lower limb after a first-ever ischemic stroke? PLoS ONE. (2018) 13:e0189279. doi: 10.1371/journal.pone.0189279
9. da Silva Cameirão M, Bermudez i Badia S, Duarte E, Verschure PF. Virtual reality based rehabilitation speeds up functional recovery of the upper extremities after stroke: a randomized controlled pilot study in the acute phase of stroke using the rehabilitation gaming system. Restor Neurol Neurosci. (2011) 29:287–98. doi: 10.3233/RNN-2011-0599
10. Saposnik G, Cohen LG, Mamdani M, Pooyania S, Ploughman M, Cheung D, et al. Efficacy and safety of non-immersive virtual reality exercising in stroke rehabilitation (EVREST): a randomised, multicentre, single-blind, controlled trial. Lancet Neurol. (2016) 1019–27. doi: 10.1016/S1474-4422(16)30121-1
11. Sampaio-Baptista C, Sanders ZB, Johansen-Berg H. Structural plasticity in adulthood with motor learning and stroke rehabilitation. Annu Rev Neurosci. (2018) 41:25–40. doi: 10.1146/annurev-neuro-080317-062015
12. Saposnik G, Teasell R, Mamdani M, Hall J, Mcilroy W, Cheung D, et al. Effectiveness of virtual reality using wii gaming technology in stroke rehabilitation: a pilot randomized clinical trial and proof of principle. Stroke. (2010) 41:1477–84. doi: 10.1161/STROKEAHA.110.584979
13. Steultjens EM, Dekker J, Bouter LM, Van de Nes JC, Cup EH, Van den Ende CH, et al. Occupational therapy for stroke patients: a systematic review. Stroke. (2003) 34:676–87. doi: 10.1161/01.STR.0000057576.77308.30
14. Jarvis K, Reid G, Edelstyn N, Hunter S. Development of the occupational therapy stroke arm and hand record: an upper limb treatment schedule. Bri J Occup Therapy. (2014) 77:126–33. doi: 10.4276/030802214X13941036266469
15. Ackerley SJ, Byblow WD, Barber PA, Macdonald H, Mcintyre-Robinson A, Stinear CM. Primed physical therapy enhances recovery of upper limb function in chronic stroke patients. Neuro Neural Repair. (2016) 30:339–48. doi: 10.1177/1545968315595285
16. Saposni G, Levin M. Virtual reality in stroke rehabilitation: a meta-analysis and implications for clinicians. Stroke. (2011) 1380–6. doi: 10.1161/STROKEAHA.110.605451
17. Fluet GG, Deutsch JE. Virtual reality for sensorimotor rehabilitation post-stroke: the promise and current state of the field. Curr Phys Med Rehabil Rep. (2013) 1:9–20. doi: 10.1007/s40141-013-0005-2
18. Thomson K, Pollock A, Bugge C, Brady MC. Commercial gaming devices for stroke upper limb rehabilitation: a survey of current practice. Disabil Rehabil Assist Technol. (2016) 11:454–61. doi: 10.3109/17483107.2015.1005031
19. Lee HS, Park YJ, Park SW. The effects of virtual reality training on function in chronic stroke patients: a systematic review and meta-analysis. Biomed Res Int. (2019) 2019:7595639. doi: 10.1155/2019/7595639
20. Iruthayarajah J, Mcintyre A, Cotoi A, Macaluso S, Teasell R. The use of virtual reality for balance among individuals with chronic stroke: a systematic review and meta-analysis. Top Stroke Rehabil. (2017) 24:68–79. doi: 10.1080/10749357.2016.1192361
21. Lee MM, Shin DC, Song CH. Canoe game-based virtual reality training to improve trunk postural stability, balance, and upper limb motor function in subacute stroke patients: a randomized controlled pilot study. J Phys Ther Sci. (2016) 28:2019–24. doi: 10.1589/jpts.28.2019
22. Kim WS, Cho S, Park SH, Lee JY, Kwon S, Paik NJ. A low cost kinect-based virtual rehabilitation system for inpatient rehabilitation of the upper limb in patients with subacute stroke: A randomized, double-blind, sham-controlled pilot trial. Medicine. (2018) 97:e11173. doi: 10.1097/MD.0000000000011173
23. Lee MM, Lee KJ, Song CH. Game-based virtual reality canoe paddling training to improve postural balance and upper extremity function: a preliminary randomized controlled study of 30 patients with subacute stroke. Med Sci Monit. (2018) 24:2590–8. doi: 10.12659/MSM.906451
24. Hara Y. Brain plasticity and rehabilitation in stroke patients. J Nippon Med Sch. (2015) 82:4–13. doi: 10.1272/jnms.82.4
25. Su F, Xu W. Enhancing brain plasticity to promote stroke recovery. Front Neurol. (2020) 11:554089. doi: 10.3389/fneur.2020.554089
26. Chen R, Cohen LG, Hallett M. Nervous system reorganization following injury. Neuroscience. (2002) 111:761–73. doi: 10.1016/S0306-4522(02)00025-8
27. Liberati A, Altman DG, Tetzlaff J, Mulrow C, Gøtzsche PC, Ioannidis JP, et al. The PRISMA statement for reporting systematic reviews and meta-analyses of studies that evaluate health care interventions: explanation and elaboration. PLoS Med. (2009) 6:e1000100. doi: 10.1371/journal.pmed.1000100
28. Bernhardt J, Hayward KS, Kwakkel G, Ward NS, Wolf SL, Borschmann K, et al. Agreed definitions and a shared vision for new standards in stroke recovery research: the stroke recovery and rehabilitation roundtable taskforce. Int J Stroke. (2017) 12:444–50. doi: 10.1177/1747493017711816
29. Ghozy S, Nam NH, Radwan I, Karimzadeh S, Tieu TM, Hashan MR, et al. Therapeutic efficacy of hepatitis B virus vaccine in treatment of chronic HBV infections: a systematic review and meta-analysis. Rev Med Virol. (2020) 30:e2089. doi: 10.1002/rmv.2089
30. Vassar M, Atakpo P, Kash MJ. Manual search approaches used by systematic reviewers in dermatology. J Med Library Assoc. (2016) 104:302. doi: 10.3163/1536-5050.104.4.009
31. Sterne JC, Savović J, Page MJ, Elbers RG, Blencowe NS, Boutron I, et al. RoB 2: a revised tool for assessing risk of bias in randomised trials. BMJ. (2019) 366:l4898. doi: 10.1136/bmj.l4898
32. Sterne JA, Hernán MA, Reeves BC, Savović J, Berkman ND, Viswanathan M, et al. ROBINS-I: a tool for assessing risk of bias in non-randomised studies of interventions. BMJ. (2016) 355:i4919. doi: 10.1136/bmj.i4919
33. Team RC. R: A Language and Environment for Statistical Computing. Vienna: R Foundation for Statistical Computing (2017).
34. Huedo-Medina T, Johnson B. Estimating the standardized mean difference effect size and its variance from different data sources: A spreadsheet. Storrs: Authors (2011).
35. Hedges LV, Pustejovsky JE, Shadish WR. A standardized mean difference effect size for multiple baseline designs across individuals. Res Synth Meth. (2013) 4:324–41. doi: 10.1002/jrsm.1086
37. Takeshima N, Sozu T, Tajika A, Ogawa Y, Hayasaka Y, Furukawa TA. Which is more generalizable, powerful and interpretable in meta-analyses, mean difference or standardized mean difference? BMC Med Res Methodol. (2014) 14:30. doi: 10.1186/1471-2288-14-30
38. Higgins JP, Green S. Cochrane Handbook for Systematic Reviews of Interventions. Identifying and measuring heterogeneity (2011).
39. Egger M, Smith GD, Schneider M, Minder C. Bias in meta-analysis detected by a simple, graphical test. BMJ. (1997) 315:629–34. doi: 10.1136/bmj.315.7109.629
40. Peters JL, Sutton AJ, Jones DR, Abrams KR, Rushton L. Comparison of two methods to detect publication bias in meta-analysis. JAMA. (2006) 295:676–80. doi: 10.1001/jama.295.6.676
41. Duval S, Tweedie R. Trim and fill: a simple funnel-plot–based method of testing and adjusting for publication bias in meta-analysis. Biometrics. (2000) 56:455–63. doi: 10.1111/j.0006-341X.2000.00455.x
42. El-Qushayri AE, Khalaf KM, Dahy A, Mahmoud AR, Benmelouka AY, Ghozy S, et al. Fournierand#x2019;s gangrene mortality: A 17-year systematic review and meta-analysis. Int J Inf Dis. (2020) 92:218–25. doi: 10.1016/j.ijid.2019.12.030
43. Abdellatif M, Ghozy S, Kamel MG, Elawady SS, Ghorab MME, Attia AW, et al. Association between exposure to macrolides and the development of infantile hypertrophic pyloric stenosis: a systematic review and meta-analysis. Eur J Pediatr. (2019) 178:301–14. doi: 10.1007/s00431-018-3287-7
44. Mekbib DB, Zhao Z, Wang J, Xu B, Zhang L, Cheng R, et al. Proactive motor functional recovery following immersive virtual reality-based limb mirroring therapy in patients with subacute stroke. Neurotherapeutics. (2020) 17:1919–30. doi: 10.1007/s13311-020-00882-x
45. Afsar SI, Mirzayev I, Umit Yemisci O, Cosar Saracgil SN. Virtual reality in upper extremity rehabilitation of stroke patients: a randomized controlled trial. J Stroke Cerebrov Dis. (2018) 27:3473–8. doi: 10.1016/j.jstrokecerebrovasdis.2018.08.007
46. Bergmann J, Krewer C, Bauer P, Koenig A, Riener R, Müller F. Virtual reality to augment robot-assisted gait training in non-ambulatory patients with a subacute stroke: a pilot randomized controlled trial. Eur J Phys Rehabil Med. (2018) 54:397–407. doi: 10.23736/s1973-9087.17.04735-9
47. Choi JH, Han EY, Kim BR, Kim SM, Im SH, Lee SY, et al. Effectiveness of commercial gaming-based virtual reality movement therapy on functional recovery of upper extremity in subacute stroke patients. Ann Rehabil Med. (2014) 38:485–93. doi: 10.5535/arm.2014.38.4.485
48. Brunner I, Skouen JS, Hofstad H, Aßmus J, Becker F, Sanders AM, et al. Virtual reality training for upper extremity in subacute stroke (VIRTUES): a multicenter RCT. Neurology. (2017) 89:2413–21. doi: 10.1212/wnl.0000000000004744
49. Cannell J, Jovic E, Rathjen A, Lane K, Tyson AM, Callisaya ML, et al. The efficacy of interactive, motion capture-based rehabilitation on functional outcomes in an inpatient stroke population: a randomized controlled trial. Clin Rehabil. (2018) 32:191–200. doi: 10.1177/0269215517720790
50. Yavuzer G, Senel A, Atay MB, Stam HJ. “Playstation eyetoy games” improve upper extremity-related motor functioning in subacute stroke: a randomized controlled clinical trial. Eur J Phys Rehabil Med. (2008) 44:237–44.
51. Wang ZR, Wang P, Xing L, Mei LP, Zhao J, Zhang T. Leap Motion-based virtual reality training for improving motor functional recovery of upper limbs and neural reorganization in subacute stroke patients. Neural Regen Res. (2017) 12:1823–31. doi: 10.4103/1673-5374.219043
52. Türkbey TA, Kutlay S, Gök H. Clinical feasibility of Xbox KinectTM training for stroke rehabilitation: a single-blind randomized controlled pilot study. J Rehabil Med. (2017) 49:22–9. doi: 10.2340/16501977-2183
53. Simşek TT, Çekok K. The effects of Nintendo Wii(TM)-based balance and upper extremity training on activities of daily living and quality of life in patients with sub-acute stroke: a randomized controlled study. Int J Neurosci. (2016) 126:1061–70. doi: 10.3109/00207454.2015.1115993
54. Rogers JM, Duckworth J, Middleton S, Steenbergen B, Wilson PH. Elements virtual rehabilitation improves motor, cognitive, and functional outcomes in adult stroke: evidence from a randomized controlled pilot study. J Neuroeng Rehabil. (2019) 16:56. doi: 10.1186/s12984-019-0531-y
55. Morone G, Tramontano M, Iosa M, Shofany J, Iemma A, Musicco M, et al. The efficacy of balance training with video game-based therapy in subacute stroke patients: a randomized controlled trial. Biomed Res Int. (2014) 2014:580861. doi: 10.1155/2014/580861
56. Brunner I, Skouen JS, Hofstad H, Aßmuss J, Becker F, Pallesen H, et al. Is upper limb virtual reality training more intensive than conventional training for patients in the subacute phase after stroke? An analysis of treatment intensity and content. BMC Neurol. (2016) 16:219. doi: 10.1186/s12883-016-0740-y
57. Iosa M, Morone G, Fusco A, Castagnoli M, Fusco FR, Pratesi L, et al. Leap motion controlled videogame-based therapy for rehabilitation of elderly patients with subacute stroke: a feasibility pilot study. Top Stroke Rehabil. (2015) 22:306–16. doi: 10.1179/1074935714Z.0000000036
58. Dobkin BH. Training and exercise to drive poststroke recovery. Nat Clin Pract Neurol. (2008) 4:76–85. doi: 10.1038/ncpneuro0709
59. Langhorne P, Coupar F, Pollock A. Motor recovery after stroke: a systematic review. Lancet Neurol. (2009) 8:741–54. doi: 10.1016/S1474-4422(09)70150-4
60. Lucca LF. Virtual reality and motor rehabilitation of the upper limb after stroke: a generation of progress? J Rehabil Med. (2009) 41:1003–100. doi: 10.2340/16501977-0405
61. Sheehy L, Taillon-Hobson A, Sveistrup H, Bilodeau M, Yang C, Finestone H. Sitting balance exercise performed using virtual reality training on a stroke rehabilitation inpatient service: a randomized controlled study. Pmr. (2020) 12:754–65. doi: 10.1002/pmrj.12331
62. Laver KE, George S, Thomas S, Deutsch JE, Crotty M. Virtual reality for stroke rehabilitation. Cochrane Database Syst Rev. (2015) 2015:Cd008349. doi: 10.1002/14651858.CD008349.pub3
63. García-Rudolph A, Sánchez-Pinsach D, Salleras EO, Tormos JM. Subacute stroke physical rehabilitation evidence in activities of daily living outcomes: a systematic review of meta-analyses of randomized controlled trials. Medicine. (2019) 98:e14501. doi: 10.1097/MD.0000000000014501
Keywords: subacute stroke, rehabilitation, virtual reality, conventional therapy 3, meta
Citation: Peng Q-c, Yin L and Cao Y (2021) Effectiveness of Virtual Reality in the Rehabilitation of Motor Function of Patients With Subacute Stroke: A Meta-Analysis. Front. Neurol. 12:639535. doi: 10.3389/fneur.2021.639535
Received: 11 December 2020; Accepted: 15 March 2021;
Published: 05 May 2021.
Edited by:
Nam-Jong Paik, Seoul National University, South KoreaReviewed by:
Maria Grazia Maggio, University of Catania, ItalyMarco Iosa, Sapienza University of Rome, Italy
Copyright © 2021 Peng, Yin and Cao. This is an open-access article distributed under the terms of the Creative Commons Attribution License (CC BY). The use, distribution or reproduction in other forums is permitted, provided the original author(s) and the copyright owner(s) are credited and that the original publication in this journal is cited, in accordance with accepted academic practice. No use, distribution or reproduction is permitted which does not comply with these terms.
*Correspondence: Yi Cao, Y2FveWkxMjc3X2hwaEAxNjMuY29t
†These authors have contributed equally to this work