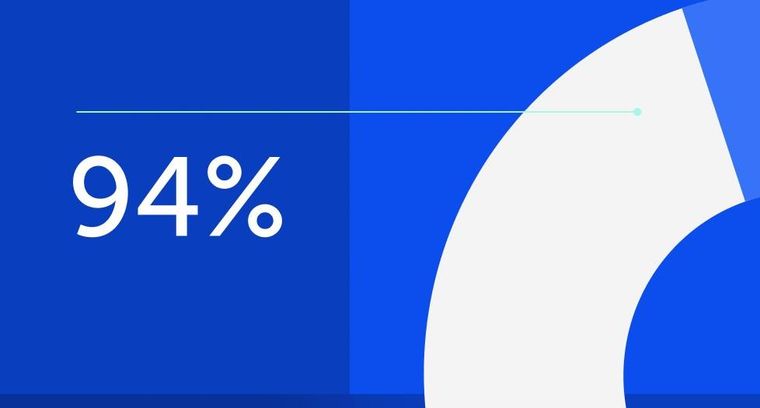
94% of researchers rate our articles as excellent or good
Learn more about the work of our research integrity team to safeguard the quality of each article we publish.
Find out more
ORIGINAL RESEARCH article
Front. Neurol., 25 August 2021
Sec. Neurotrauma
Volume 12 - 2021 | https://doi.org/10.3389/fneur.2021.624378
Traumatic brain injury (TBI) can cause damage to peripheral organ systems, such as digestive organ system, and alterations of gut microbiota in addition to brain injury. Our previous study found that TBI induced gastrointestinal dysfunction accompanied by alterations of bile acid metabolism. Bile acid and its receptors have been reported to play important roles in various neurological diseases. To further examine the changes of bile acid metabolism in TBI patients, we performed a retrospective clinical analysis. In this study, 177 patients were included, and the results showed that TBI patients had more frequent antibiotic use compared with a control group. Regression analysis identified TBI as an independent factor for reduction of serum bile acid level (B = −1.762, p = 0.006), even with antibiotic use taken into a regression model. Sub-group regression analysis of TBI patients showed that antibiotic use was negatively associated with bile acid level, while creatinine and triglyceride were positively associated with bile acid level. In conclusion, these data indicated that TBI could greatly reduce serum bile acid. This study provided preliminary but novel clinical evidence of TBI interfering with bile acid metabolism, and further studies with large sample sizes are needed to validate these findings in the future.
Traumatic brain injury (TBI) is a worldwide major cause of death and disability and leads to heavy medical burden to the society (1). TBI not only deals primary damage to the central nervous system (CNS) but also causes secondary disorder to multiple peripheral organs (2–4). One of the most affected peripheral systems is the digestive system, which will suffer various pathological changes and present with different kinds of abnormalities (5, 6). These abnormalities include digestive dysfunction, gut inflammation, barrier damage, gut microbiota disturbance, and other gastrointestinal changes. Clinical expressions caused by these changes are also common. More than 50% of TBI patients suffer from gastrointestinal symptoms, and the rate of severe complications such as gastrointestinal hemorrhage varies from 5 to 12% in different studies (7–9). This high incidence of gastrointestinal complications has drawn the attention of researchers to relevant physiological or pathological progresses.
Bile acid metabolism is an important physiology progress which involves the intestine, gut microbiota, liver, and gallbladder (10, 11). Bile acid is related to multiple diseases and has been considered to be a potential therapy tool especially in gastrointestinal abnormalities (12). Apart from its close connection to the digestive system, it also showed influence on neurological diseases especially in stroke (13) and intracranial hemorrhage (ICH) (14). Bile acid possibly plays a positive role in stroke through the activation of its receptors such as TGR5 and is able to attenuate neuroinflammation through different pathways, including neuron apoptosis inhibition and microglia functioning (15, 16). Moreover, bile acid reduction has been reported to correlate with higher stroke risk in a prospective study (17). In recent studies, bile acid and its receptors have also been reported to connect with TBI and related pathological changes (18–21). Experimental evidence indicates that bile acid transporters expressed by hypothalamus neurons are changed post injury. Certain bile acids and analogs are also able to influence the neuroinflammation and neuron apoptosis after TBI events. These results are promising and indicate that bile acid has a role in the disease progress of TBI.
Given the potential function of bile acid, researchers have been making efforts to figure out whether TBI could alter bile acid metabolism. The existing hypothesis that TBI and bile acid have bidirectional interaction is supported by considerable experimental evidence. Bile acid metabolism has been well-acknowledged to rely on the gut microbiota (22, 23). It was also reported in a previous study that TBI could significantly influence the intestinal bacteria and reduce its abundance (24, 25). Our recent study has proved that the microbiota change was accompanied by bile acid alteration in serum and feces. Specifically, the serum bile acid changes of mice involved both primary and secondary bile acids (26). Under such circumstance, it is natural for researchers to consider TBI affective to bile acid through gut microbiota. Yet, the current studies are mostly confined to animal models. Compared with experimental studies, direct clinical evidence showing TBI influences bile acid metabolism is still lacking.
In this study, based on existing laboratory evidence, we intended to research the influence of TBI events on the bile acid metabolism through a retrospective clinical analysis involving patients with or without TBI. We also did a sub-group analysis to study other potential factors that possibly affect bile acid level in TBI patients.
This retrospective study screened TBI and trigeminal neuralgia (TN)/hemifacial spasm (HFS) patients who were admitted to our medical center from January 2019 to January 2020. The inclusion required patients to have laboratory test results from standard fasting blood samples upon admission, which should include serum bile acid level as well. The exclusion criteria included (1) patients had original hepatobiliary, pancreatic, or gastrointestinal diseases; (2) patients had been under full parenteral nutrition support which would cause decreased bile acid synthesis (27); (3) patients were 1 week post any sort of surgical procedure or non-TBI trauma events; and (4) patients had already shown multi-organ failure or other terminal stage performance.
The TN/HFS patients were included in this study as a control group. These TN/HFS patients were not accompanied by CNS injuries, and TN/HFS had never been found related to bile acid metabolism. Since serum bile acid level is not routinely included in the periodic health examination service of our center, the data of healthy volunteers were not available in this single-center retrospective study.
This study set a relatively strict standard inclusion criteria regarding blood sample acquirement so as to obtain reliable bile acid data. The test results of included patients were all obtained by our medical center so that deviation between different labs could be prevented. The blood samples were obtained on the morning of the admission after at least 8-h fasting. This would be before any invasive treatment for patients, so that they were at a most consistent status. Specifically, the laboratory tests should at least cover liver function, kidney function, lipid metabolism, serum bile acid, blood routine, and coagulation function.
It is worthy to note that this strategy would not include emergency TBI patients since their pre-operational tests were urgent and would not meet the inclusion criteria. Mild TBI patients would not be included either since they did not need to be hospitalized.
Collected clinical data included gender, age, diagnosis, medical history, initial Glasgow Coma Scale (GCS) upon first admission, alanine transaminase (ALT) level, aspartate aminotransferase (AST) level, antibiotic use, creatinine level, total bilirubin, cholesterol level, triglyceride level, and serum bile acid level. For TBI patients, the post-trauma duration and injury category are also documented. No personal information is traceable in this report, and the study is in keeping with the ethical standards of the 1964 Declaration of Helsinki and its later amendments. The study is approved by the ethics committee of our medical center with the approval number 2020IIT1102.
Statistical analysis was performed using SPSS version 19.0 (IBM Corp, Armonk, NY). Continuous variables are given as means and standard deviations. Categorical variables are given as numbers and percentages. To compare continuous variables, Student's t-test (if normally distributed) or Mann–Whitney U-test (if not normally distributed) were performed. To compare categorical variables, chi-square test was performed. Linear regression analysis was performed to determine the factors that influence serum bile acid level, and variance inflation factor (VIF) values were calculated in the process to rule out the possible impact of multi-colinearity. Statistical significance was determined when p < 0.05.
We retrospectively screened 368 TBI or TN/HFS patients, among whom 177 were analyzed after exclusion. The inclusion flow chart is shown in Figure 1. It is worthy to note that no patients with repeated TBI were finally included.
Among the 177 patients included in this study, 104 (58.8%) were TBI patients. The average age of all 177 patients was 57.6 ± 13.2 years, and 103 (58.2%) patients were male. The initial GCS was 13.1 ± 3.19. Thirty-eight (21.5%) patients were under antibiotic treatment when the blood samples were obtained. The serum bile acid level was 5.18 ± 3.41 μmol/L. Twenty-one patients had a history of hypertension, seven had benign tumor, one patient had a history of chronic nephritis, four had a history of heart disease, and two had a history of asthma. TBI patients included 79 with cerebral contusion and/or laceration, 11 with only epidural hematoma, 6 with only subdural hematoma, 4 with epidural and subdural hematoma, and 4 with only skull fracture (no intracranial lesion). The detailed basic characteristics can be found in Table 1.
The median post-injury time of sample collection for TBI patients was 30.0 days. Compared with the control group, TBI patients had higher ALT (24.5 ± 21.8 vs. 17.7 ± 11.3 u/L, p = 0.007) and AST (25.0 ± 16.8 vs. 18.5 ± 5.70 u/L, p < 0.001) levels, but their creatinine (59.0 ± 14.9 vs. 65.8 ± 13.0, p = 0.002) and cholesterol (4.08 ± 0.96 vs. 4.54 ± 0.87, p = 0.002) levels were lower. TBI patients also had a greater ratio of antibiotic use (36.5 vs. 0%, p < 0.001) and lower initial GCS (11.7 ± 3.59 vs. 15.0 ± 0.00, p < 0.001) because TN/HFS patients had no need of anti-infection treatment before surgery and suffered no CNS damage leading to unconsciousness. The serum bile acid level of TBI patients was about two-thirds of that in the control group patients, and the difference was statistically significant (4.31 ± 2.70 vs. 6.41 ± 3.93 μmol/L, p < 0.001). The age, total bilirubin, and triglyceride were similar between the two groups. The differences are presented in Table 2.
Given the different characteristics of TBI patients compared with the control group, we performed regression analysis to determine whether TBI has independent effect on bile acid metabolism. The results indicated that TBI was the only independent factor that had statistically significant influence on serum bile acid level in our study cohort (B = −1.762, 95% CI: −3.020 to −0.505, p = 0.006). Other factors including antibiotic use and initial GCS were not independently effective when TBI was included in the regression model. The regression analysis of all patients is shown in Table 3.
It is worthy to note that the AST and ALT levels presented a co-linearity problem that cannot be ignored; thus, in the regression analysis (both in this section and the sub-group section), only ALT was included.
To explore the potential effect of other factors on bile acid level in TBI patients, we also did sub-group analysis using regression method. The data showed that in the TBI group of our study, antibiotic use (B = −1.204, 95% CI: −2.356 to −0.052, p = 0.041) was associated with a lower serum bile acid level, while creatinine (B = 0.051, 95% CI: 0.009–0.093, p = 0.018) and triglyceride (B = 0.758, 95% CI: 0.032–1.485, p = 0.041) had positive association with the serum bile acid level. Other factors including gender, age, post-injury time, total bilirubin, ALT, GCS, and cholesterol did not independently influence serum bile acid according to our dataset. The detailed sub-group analysis is presented in Table 4.
In this study, we illustrated that TBI might interfere with bile acid metabolism and, as a result, reduce the serum bile acid level. These results provide novel clinical evidence regarding the relationship between bile acid and TBI. Hopefully, they can work as a clue for future bile acid study in neuroscience.
As mentioned, the role of bile acid and its receptors in neurological diseases has been noticed by researchers a decade ago. Within the recent several years, multiple studies have reported that bile acid receptors or transporters [such as TGR5 and apical sodium-dependent bile acid (ASBT) transporter] were involved in the pathological progress after brain injury in animal models (15, 20). Bile acid receptors and transporters were reported to decrease as early as 2 h post injury, and ASBT-transporter expressing neurons in the hypothalamus area were revealed to suffer a significant decrease following TBI events (19). The activators of these receptors also showed biological effects in vivo (21). Such results all indicated that bile acid had potential significance in TBI pathologies. However, there has been limited clinical evidence linking TBI with bile acid, and it was unclear whether TBI could directly disturb the normal bile acid circulation in patients. Our data indicated that TBI could make a difference to bile acid metabolism. This relationship existing in patients might put more confidence in future studies focused on bile acid function in TBI treatment.
In previous studies, bile acid metabolism was reported to be influenced by gut microbiota (23). This could be one of the mechanisms by which TBI changes serum bile acid level since TBI is able to disturb intestinal bacteria (25). As mentioned, our recent results in TBI in mice proved that the microbiota change was accompanied by bile acid alteration in serum and feces, which put more reliability on the relationship. The possible mechanism behind this correlation could be related to the characteristic of bile acid metabolism. It has been widely accepted that bile acid is one of the major metabolites of gut microbiota (28). To be noted, in our previous study, both primary and secondary bile acid levels were reduced. Since primary bile acids are synthesized in the liver and then secreted into the intestinal tract, it is possible that apart from the microbiota, the influence of TBI on gastrointestinal absorption function may also be important, which needs further studies to confirm. Of course, TBI is not the only factor in microbiota alteration. Antibiotics, especially broad-spectrum categories, are highly effective in reducing gut microbiota abundance. In fact, antibiotics are well-accepted tools in building intestinal germ-free animal models (29, 30). At the same time, TBI patients often have much higher rates of antibiotic use than normal people. This could bring possible bias (31, 32). In our study, TBI patients had been given antibiotics much more frequently than control group patients. Thus, to avoid bias, we performed regression analysis. It turned out that even when taking antibiotic use into consideration, TBI still acted as an independent risk factor for serum bile acid reduction.
Apart from injury events, bile acid metabolism is also supposed to be associated with other physiological progresses (33, 34). In our regression analysis, due to the strong effect of TBI, all other factors showed no statistical significance. To gain further understanding, we also performed sub-group analysis in TBI patients. The results indicated antibiotics, serum creatinine, and triglyceride were related to the change of serum bile acid level. Antibiotics are able to further reduce bile acid level in TBI patients possibly due to their effects on microbiota as mentioned. Triglyceride showed a positive correlation with bile acid level, which might indicate the relationship between the bile acid and the lipid metabolism. It is worthy to note that in our study cholesterol is not significantly related to bile acid level, and the reason is not very clear. Serum creatinine also showed association with bile acid, but compared with triglyceride and antibiotics, its influence was rather minor according to the regression results. The correlation analysis of non-TBI patients indicated that the positive correlations of both triglyceride and creatinine were unique in TBI patients (see Supplementary Table 1). It is possible that the central nervous injury drives these related variations of different metabolism processes. Given the limitations of our study, based on current data it is hard to confirm these relationships and their clinical meanings; thus, further large-scale study is needed.
We have to state that this study had included limited amount of patients and the control group did not consist of healthy volunteers because of the retrospective design. Thus, apart from the strong effects of TBI and antibiotics, the influence of other factors such as creatinine on bile acid level might not be universal and should be interpreted with caution. Moreover, many TBI patients requiring immediate operation at the time of admission were not included because their pre-operational laboratory tests would only be minimally essential for the neurosurgical procedure. As a result, they would not meet the inclusion criteria. Mild TBI patients who did not need hospitalization were out of our study scope either, which made it difficult to determine whether the injury severity would affect bile acid level. Given these limitations, more large-scale studies will be needed to determine the biological role of bile acid metabolism in TBI disease and its interaction with other physiological progresses.
In conclusion, TBI is an independent factor that is able to lower serum bile acid level. Among TBI patients, antibiotic use will reduce bile acid further. Higher triglyceride and creatinine are possibly related to higher serum bile acid level in TBI patients, which requires more detailed studies to confirm in the future.
The original contributions presented in the study are included in the article/Supplementary Material, further inquiries can be directed to the corresponding authors.
The studies involving human participants were reviewed and approved by The First Affiliated Hospital, School of Medicine, Zhejiang University. The patients/participants provided their written informed consent to participate in this study.
YZ, ZC, WY, YW, MT, PZ, LW, and XY conceived and planned the study. YZ, ZC, WY, YW, MT, and PZ carried out the data collection and analysis. LW and XY checked and supervised the collected data and analysis progress. YZ, ZC, and WY took part in writing the manuscript. All authors provided critical feedback and helped shape the research, analysis, and manuscript.
The authors declare that the research was conducted in the absence of any commercial or financial relationships that could be construed as a potential conflict of interest.
All claims expressed in this article are solely those of the authors and do not necessarily represent those of their affiliated organizations, or those of the publisher, the editors and the reviewers. Any product that may be evaluated in this article, or claim that may be made by its manufacturer, is not guaranteed or endorsed by the publisher.
The Supplementary Material for this article can be found online at: https://www.frontiersin.org/articles/10.3389/fneur.2021.624378/full#supplementary-material
1. Carney N, Totten AM, O'reilly C, Ullman JS, Hawryluk GW, Bell MJ, et al. Guidelines for the management of severe traumatic brain injury, fourth edition. Neurosurgery. (2017) 80:6–15. doi: 10.1227/NEU.0000000000001432
2. Bansal V, Costantini T, Kroll L, Peterson C, Loomis W, Eliceiri B, et al. Traumatic brain injury and intestinal dysfunction: uncovering the neuro-enteric axis. J Neurotrauma. (2009) 26:1353–9. doi: 10.1089/neu.2008.0858
3. Kerr NA, De Rivero Vaccari JP, Abbassi S, Kaur H, Zambrano R, Wu S, et al. Traumatic brain injury-induced acute lung injury: evidence for activation and inhibition of a neural-respiratory-inflammasome axis. J Neurotrauma. (2018) 35:2067–76. doi: 10.1089/neu.2017.5430
4. Garcia JM, Lopez-Rodriguez AB. Editorial: neuroendocrine disorders after traumatic brain injury: past, present and future. Front Endocrinol (Lausanne). (2019) 10:386. doi: 10.3389/fendo.2019.00386
5. Ma EL, Smith AD, Desai N, Cheung L, Hanscom M, Stoica BA, et al. Bidirectional brain-gut interactions and chronic pathological changes after traumatic brain injury in mice. Brain Behav Immun. (2017) 66:56–69. doi: 10.1016/j.bbi.2017.06.018
6. Pan P, Song Y, Du X, Bai L, Hua X, Xiao Y, et al. Intestinal barrier dysfunction following traumatic brain injury. Neurol Sci. (2019) 40:1105–10. doi: 10.1007/s10072-019-03739-0
7. Cook DJ, Fuller HD, Guyatt GH, Marshall JC, Leasa D, Hall R, et al. Risk factors for gastrointestinal bleeding in critically ill patients. Canadian Critical Care Trials Group. N Engl J Med. (1994) 330:377–81. doi: 10.1056/NEJM199402103300601
8. Olsen AB, Hetz RA, Xue H, Aroom KR, Bhattarai D, Johnson E, et al. Effects of traumatic brain injury on intestinal contractility. Neurogastroenterol Motil. (2013) 25:593–e463. doi: 10.1111/nmo.12121
9. Yeh CC, Wang HH, Chou YC, Hu CJ, Chou WH, Chen TL, et al. High risk of gastrointestinal hemorrhage in patients with epilepsy: a nationwide cohort study. Mayo Clin Proc. (2013) 88:1091–8. doi: 10.1016/j.mayocp.2013.06.024
10. Russell DW. The enzymes, regulation, and genetics of bile acid synthesis. Annu Rev Biochem. (2003) 72:137–74. doi: 10.1146/annurev.biochem.72.121801.161712
11. Bajaj JS. Alcohol, liver disease and the gut microbiota. Nat Rev Gastroenterol Hepatol. (2019) 16:235–46. doi: 10.1038/s41575-018-0099-1
12. Blanchet M, Brunel JM. Bile acid derivatives: from old molecules to a new potent therapeutic use: an overview. Curr Med Chem. (2018) 25:3613–36. doi: 10.2174/0929867325666180309113737
13. Rodrigues CM, Spellman SR, Sola S, Grande AW, Linehan-Stieers C, Low WC, et al. Neuroprotection by a bile acid in an acute stroke model in the rat. J Cereb Blood Flow Metab. (2002) 22:463–71. doi: 10.1097/00004647-200204000-00010
14. Rodrigues CM, Sola S, Nan Z, Castro RE, Ribeiro PS, Low WC, et al. Tauroursodeoxycholic acid reduces apoptosis and protects against neurological injury after acute hemorrhagic stroke in rats. Proc Natl Acad Sci USA. (2003) 100:6087–92. doi: 10.1073/pnas.1031632100
15. Yanguas-Casás N, Barreda-Manso MA, Nieto-Sampedro M, Romero-Ramírez L. TUDCA: an agonist of the bile acid receptor GPBAR1/TGR5 with anti-inflammatory effects in microglial cells. J Cell Physiol. (2017) 232:2231–45. doi: 10.1002/jcp.25742
16. Liang H, Matei N, Mcbride DW, Xu Y, Zhou Z, Tang J, et al. TGR5 activation attenuates neuroinflammation via Pellino3 inhibition of caspase-8/NLRP3 after middle cerebral artery occlusion in rats. J Neuroinflammation. (2021) 18:40. doi: 10.1186/s12974-021-02087-1
17. Charach G, Karniel E, Novikov I, Galin L, Vons S, Grosskopf I, et al. Reduced bile acid excretion is an independent risk factor for stroke and mortality: a prospective follow-up study. Atherosclerosis. (2020) 293:79–85. doi: 10.1016/j.atherosclerosis.2019.12.010
18. Gronbeck KR, Rodrigues CM, Mahmoudi J, Bershad EM, Ling G, Bachour SP, et al. Application of tauroursodeoxycholic acid for treatment of neurological and non-neurological diseases: is there a potential for treating traumatic brain injury? Neurocrit Care. (2016) 25:153–66. doi: 10.1007/s12028-015-0225-7
19. Nizamutdinov D, Demorrow S, Mcmillin M, Kain J, Mukherjee S, Zeitouni S, et al. Hepatic alterations are accompanied by changes to bile acid transporter-expressing neurons in the hypothalamus after traumatic brain injury. Sci Rep. (2017) 7:40112. doi: 10.1038/srep40112
20. Sun D, Gu G, Wang J, Chai Y, Fan Y, Yang M, et al. Administration of tauroursodeoxycholic acid attenuates early brain injury via akt pathway activation. Front Cell Neurosci. (2017) 11:193. doi: 10.3389/fncel.2017.00193
21. Zuo G, Zhang T, Huang L, Araujo C, Peng J, Travis Z, et al. Activation of TGR5 with INT-777 attenuates oxidative stress and neuronal apoptosis via cAMP/PKCepsilon/ALDH2 pathway after subarachnoid hemorrhage in rats. Free Radic Biol Med. (2019) 143:441–53. doi: 10.1016/j.freeradbiomed.2019.09.002
22. Enright EF, Griffin BT, Gahan CGM, Joyce SA. Microbiome-mediated bile acid modification: role in intestinal drug absorption and metabolism. Pharmacol Res. (2018) 133:170–86. doi: 10.1016/j.phrs.2018.04.009
23. Jia W, Xie G, Jia W. Bile acid-microbiota crosstalk in gastrointestinal inflammation and carcinogenesis. Nat Rev Gastroenterol Hepatol. (2018) 15:111–28. doi: 10.1038/nrgastro.2017.119
24. Sundman MH, Chen NK, Subbian V, Chou YH. The bidirectional gut-brain-microbiota axis as a potential nexus between traumatic brain injury, inflammation, and disease. Brain Behav Immun. (2017) 66:31–44. doi: 10.1016/j.bbi.2017.05.009
25. Treangen TJ, Wagner J, Burns MP, Villapol S. Traumatic Brain injury in mice induces acute bacterial dysbiosis within the fecal microbiome. Front Immunol. (2018) 9:2757. doi: 10.3389/fimmu.2018.02757
26. You W, Zhu Y, Wei A, Du J, Wang Y, Zheng P, et al. Traumatic brain injury induces gastrointestinal dysfunction and dysbiosis of gut microbiota accompanied by alterations of bile acid profile. J Neurotrauma. (2021). doi: 10.1089/neu.2020.7526. [Epub ahead of print].
27. Dawes LG, Laut HC, Woodruff M. Decreased bile acid synthesis with total parenteral nutrition. Am J Surg. (2007) 194:623–7. doi: 10.1016/j.amjsurg.2007.07.017
28. Winston JA, Theriot CM. Diversification of host bile acids by members of the gut microbiota. Gut Microbes. (2020) 11:158–71. doi: 10.1080/19490976.2019.1674124
29. Kennedy EA, King KY, Baldridge MT. Mouse microbiota models: comparing germ-free mice and antibiotics treatment as tools for modifying gut bacteria. Front Physiol. (2018) 9:1534. doi: 10.3389/fphys.2018.01534
30. Chu C, Murdock MH, Jing D, Won TH, Chung H, Kressel AM, et al. The microbiota regulate neuronal function and fear extinction learning. Nature. (2019) 574:543–8. doi: 10.1038/s41586-019-1644-y
31. Behr C, Slopianka M, Haake V, Strauss V, Sperber S, Kamp H, et al. Analysis of metabolome changes in the bile acid pool in feces and plasma of antibiotic-treated rats. Toxicol Appl Pharmacol. (2019) 363:79–87. doi: 10.1016/j.taap.2018.11.012
32. Chen L, Young W, Moviglia GA, Al Zoubi ZM. Summary report of the 11th Annual Conference of International Association of Neurorestoratology (IANR). J Neurorestoratology. (2019) 7:1–7. doi: 10.26599/JNR.2019.9040004
33. Ahlin S, Cefalo C, Bondia-Pons I, Capristo E, Marini L, Gastaldelli A, et al. Bile acid changes after metabolic surgery are linked to improvement in insulin sensitivity. Br J Surg. (2019) 106:1178–1186. doi: 10.1002/bjs.11208
Keywords: traumatic brain injury, bile acid, retrospective study, brain-gut axis, gut microbiota
Citation: Zhu Y, Chen Z, You W, Wang Y, Tu M, Zheng P, Wen L and Yang X (2021) A Retrospective Clinical Analysis of the Serum Bile Acid Alteration Caused by Traumatic Brain Injury. Front. Neurol. 12:624378. doi: 10.3389/fneur.2021.624378
Received: 02 November 2020; Accepted: 09 July 2021;
Published: 25 August 2021.
Edited by:
Cameron Bass, Duke University, United StatesReviewed by:
Lyndsey E. Collins-Praino, University of Adelaide, AustraliaCopyright © 2021 Zhu, Chen, You, Wang, Tu, Zheng, Wen and Yang. This is an open-access article distributed under the terms of the Creative Commons Attribution License (CC BY). The use, distribution or reproduction in other forums is permitted, provided the original author(s) and the copyright owner(s) are credited and that the original publication in this journal is cited, in accordance with accepted academic practice. No use, distribution or reproduction is permitted which does not comply with these terms.
*Correspondence: Xiaofeng Yang, empjc3drQHpqdS5lZHUuY24=; Liang Wen, d2VubGlhbmdAemp1LmVkdS5jbg==
†These authors have contributed equally to this work
Disclaimer: All claims expressed in this article are solely those of the authors and do not necessarily represent those of their affiliated organizations, or those of the publisher, the editors and the reviewers. Any product that may be evaluated in this article or claim that may be made by its manufacturer is not guaranteed or endorsed by the publisher.
Research integrity at Frontiers
Learn more about the work of our research integrity team to safeguard the quality of each article we publish.