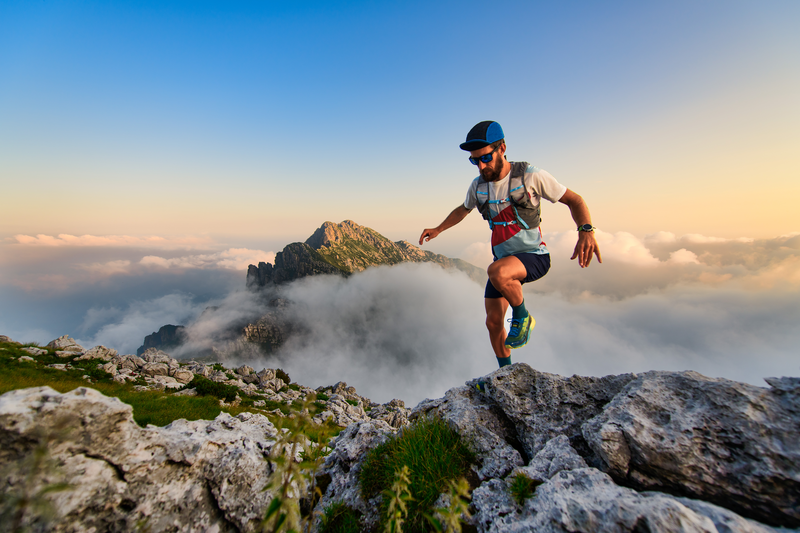
94% of researchers rate our articles as excellent or good
Learn more about the work of our research integrity team to safeguard the quality of each article we publish.
Find out more
MINI REVIEW article
Front. Neurol. , 24 May 2021
Sec. Stroke
Volume 12 - 2021 | https://doi.org/10.3389/fneur.2021.600365
This article is part of the Research Topic Therapeutic Agents and Targets to Minimize Ischemic Brain Injury View all 18 articles
Stroke is one of the leading causes of death and disability in the world. Stroke not only affects the patients, but also their families who serve as the primary caregivers. Discovering novel therapeutic targets for stroke is crucial both from a quality of life perspective as well as from a health economic perspective. Exercise is known to promote neuroprotection in the context of stroke. Indeed, exercise induces the release of blood-borne factors that promote positive effects on the brain. Identifying the factors that mediate the positive effects of exercise after ischemic stroke is crucial for the quest for novel therapies. This approach will yield endogenous molecules that normally cross the blood brain barrier (BBB) and that can mimic the effects of exercise. In this minireview, we will discuss the roles of exercise factors released by the liver such as beta-hydroxybutyrate (DBHB), by the muscle such as lactate and irisin and by the bones such as osteocalcin. We will also address their therapeutic potential in the context of ischemic stroke.
Stroke is the fifth major cause of death and a leading cause of disability in the United States. This is due to the lack of neuroprotective agents that are able to decrease the associated neuronal damage and loss (1, 2). Neurotrophins such as brain-derived neurotrophic factor (BDNF) mediate protection and recovery following stroke. BDNF promotes regeneration and restores damaged neural tissue. The use of exogenous BDNF after stroke is hindered by its rapid degradation and its inability to cross the blood-brain barrier (BBB). Hence, therapeutics that can modulate endogenous BDNF signaling in the brain may be useful in the context of stroke (3).
Physical exercise increases Bdnf expression in the hippocampus to promote learning, and memory formation (4). Exercise mediates these positive effects by inducing the release of metabolites and proteins from the liver, muscle, bones and platelets (Figure 1). These factors have been shown to be protective in the context of traumatic brain injury (12) and depression (13). In addition, exercise can prevent and alleviate many of the detrimental effects of stroke. Understanding which factors mediate the protective effects of exercise in ischemic stroke and deciphering the involvement of BDNF signaling will allow us to fully harness exercise's therapeutic potential.
Figure 1. Exercise induces the release of metabolites and proteins that promote learning and memory formation through activation of Bdnf expression and signaling in the hippocampus. Bones release osteocalcin that promotes Bdnf expression through an epigenetic mechanism involving RbAP48 (5, 6). The liver releases the ketone body betahydroxy-butyrate that induces hippocampal Bdnf expression by inhibiting class I HDACs, namely HDAC2 and HDAC3 (4, 7). The muscle releases FNDC5/irisin that activate hippocampal Bdnf expression through an unknown mechanism (8). In addition, the muscle release lactate that activates the hippocampal SIRT1/PGC1-alpha/FNDC5 pathway and in turn Bdnf expression (9). Finally activated platelets have been shown to store and secrete BDNF (10, 11). Whether these factors mediate the positive effects of exercise in stroke is not clear and needs to be addressed. In addition, the role of BDNF signaling in exercise-mediated neuroprotection also needs to be assessed. BDNF, brain-derived neurotrophic factor; FDNC5, fibronectin type III domain-containing protein 5; HDACs, histone deacetylases; SIRT1, sirtuin 1; PGC1-alpha, Peroxisome proliferator-activated receptor gamma coactivator 1-alpha.
In this minireview, we will focus on how newly identified blood-borne exercise factors that induce hippocampal BDNF signaling to promote learning and memory formation are protective after ischemic stroke. We will also address the current research gaps that link these factors to the positive effects of exercise. Indeed, this minireview will highlight the urgent need for systemic experimentation to identify which factors are responsible for exercise's prophylactic and therapeutic effects in the context of stroke and the role of BDNF signaling in these effects.
It is important to distinguish between the preventative roles of exercise and its therapeutic role. Exercise pre-conditioning enhances neuroprotection and decreases brain edema (14–23). Treadmill exercise prior to middle cerebral artery occlusion (MCAO) in rodent models improves motor function, decreases infarct volumes, reduces neuronal apoptosis and oxidative stress, enhances angiogenesis and induces Bdnf expression (24–27). The level of neuroprotection that is achieved varies with the duration and intensity of exercise. For example, even though short bouts of exercise before stroke induce the expression of angiogenesis markers, they may not be enough to rescue neurological deficits post-ischemic stroke (26). Alternatively, high intensity interval training (HIIE) alleviates the symptoms of stroke more efficiently than moderate continuous training (28). Moderate exercise may protect the brain against MCAO by enhancing the release of miR-126 enriched endothelial progenitor cell-derived exosomes (29).
Exercise is also a safe and cost-effective therapeutic strategy post-stroke, in which the optimal time and intensity of exercise is critical (30). Indeed, animal studies have revealed that both high intensity exercise or exercise initiated only 24 h after stroke promote inflammation and cell death (30). In contrast, exercise initiated on later time periods (as early as 48 h post-stroke) as well as low intensity and moderate intensity exercise improve infarct volume and neurological severity scores 14 days post-stroke (30). Interestingly, prolonged treadmill exercise promotes neurogenesis and improves motor function and short-term memory by increasing the expression of hippocampal BDNF in photothrombotic stroke mice (31). In addition, treadmill exercise enhances neurogenesis and myelin repair by activating the Wnt and BDNF pathways after focal cerebral ischemia/reperfusion (32).
In most of the paradigms in which exercise was studied as a prophylactic (14–23) or as a therapy for ischemic stroke (33–37), the neuroprotective roles of exercise were associated with restoration of BDNF levels (38, 39). In humans, decreases in BDNF levels are correlated with an increased risk of stroke, worse functional outcomes and higher mortality (40). Indeed, BDNF levels are decreased in acute ischemic-stroke patients. Interestingly, patients that carry the BDNF Val66Met allele, known to decrease BNDF levels by 30%, have worse outcomes and prognosis after stroke (40).
Exercise enhances neurogenesis, mediates synaptic plasticity and promotes learning and memory formation. These effects are thought to be mediated in part by activation of hippocampal BDNF signaling [reviewed in (4)]. Recent work has revealed that injection of blood from young exercising mice is able to rescue learning and memory defects in old mice by inducing hippocampal BDNF levels (41). Several blood-borne exercise factors comprised of proteins and metabolites released by the liver, muscle and bones have been identified through their ability to induce BDNF signaling, and have been implicated in mediating the positive effects of exercise on the brain. Since decreases in BDNF levels are correlated with negative outcomes after stroke (40), studying the exercise factors that induce BDNF signaling and assessing their neuroprotective abilities may allow us to identify novel endogenous therapeutic agents for stroke. For this reason, we will discuss what is known about blood-borne factors released by the liver, muscle and bones. Interestingly, even though some of these factors have neuroprotective effects in animal models of stroke, very little has been done to directly demonstrate that they are responsible for the preventative or therapeutic effects of exercise. These studies remain necessary to further our understanding of the molecular mechanisms underlying the effects of exercise.
Exercise induces the release of multiple factors from the liver into the blood that can transfer its benefits to the brain. These exercise factors include metabolites, such as the ketone body beta-hydroxybutyrate (DBHB) (7) and proteins, such as glycosylphosphatidylinositol (GPI)–specific phospholipase D1(Gpld1)(41).
During exercise, the liver releases DBHB into the blood. DBHB crosses the BBB and accumulates in the hippocampus, where it induces Bdnf expression by acting as a class I histone deacetylase inhibitor (7). Multiple studies have demonstrated the beneficial effects of ketone bodies and ketogenic diets for brain health. Ketogenic diets rescue neurogenesis defects and prevent memory abnormalities in Kabuki syndrome by inducing transcriptional changes through histone deacetylase (HDAC) inhibition (42). They also extend longevity, improve memory and enhance brain health in aging mice (43, 44). DBHB improves multiple cellular pathologies in Parkinson's disease (PD) [reviewed in (45)] and improves learning and memory formation in a mouse model of Alzheimer's disease (AD) (46). In addition, DBHB has antidepressant effects: it decreases depressive behaviors in mice by increasing histone3-lysine9-β-hydroxybutyrylation and promoting BDNF expression (47). Interestingly, DBHB and ketogenic diets also have promising neuroprotective potential against stroke.
Both DBHB and ketogenic diets promote neuroprotection after stroke. They decrease infarct volume after permanent and transient MCAO (48, 49). DBHB improves cerebral energy metabolism during ischemia and inhibits lipid peroxidation after reperfusion (49). A ketogenic diet improves ischemic tolerance to MCAO and inhibits the nucleotide-binding domain (NOD)-like receptor protein 3 (NLRP3) inflammasome in the brain (50). DBHB also inhibits dynamin-related protein 1 (Drp1)-mediated mitochondrial fission and suppresses endoplasmic reticulum stress-activated NLRP3 inflammasome in oxygen-glucose deprived (OGD) neuroblastoma cells (50). This later pathway is involved in detecting cellular damage and mediating inflammation during ischemic stroke. Combined treatment of DBHB with another ketone body, acetoacetate decreases infarct volume, improves neurologic function, and increases the NAD+/NADH ratio, Sirtuin 3 (Sirt3), Forkhead Box O3a, and Superoxide Dismutase 2 expression in the penumbra (51). Interestingly, knockdown of Sirt3 in primary neurons attenuates the ability of ketone bodies to promote cell survival in a rotenone-dependent model of neuronal death, suggesting that SIRT3 may mediate the pro-survival effects of ketone bodies (51). More work is needed to fully decipher this pathway and to assess its contribution to neuroprotection in vivo.
The hydroxy-carboxylic acid receptor 2 (HCA2) mediates the neuroprotective effect of ketogenic diets and DBHB in cerebral ischemia (52) (Figure 2). Indeed, while both ketogenic diets and DBHB significantly decrease infarct size after MCAO, this protective effect is lost in the HCA2 knockout mice despite higher plasma levels of ketone bodies (52). Interestingly, the HCA2 protective effect is mediated by infiltrating macrophages and monocytes, as activation of HCA2 in these cells is neuroprotective (52). This work suggests that the DBHB released from the liver mediates neuroprotection by modulating neuroinflammation. As a result, it is important to understand whether DBHB also activates its receptors in neuronal cells after ischemic stroke to mediate its neuroprotective role. Tissue-specific knockouts of this receptor will help determine whether its roles are restricted to immune cells or whether it plays important signaling effects in neurons. More studies are also needed to determine whether DBHB is responsible for mediating exercise's neuroprotective effects in cerebral ischemia, and to identify the molecular mechanism underlying these neuroprotective effects. Since we already know that exercise increases DBHB levels in the hippocampus where it increases Bdnf expression through HDAC inhibition (7) and that DBHB induces resistance to oxidative stress via HDAC inhibition (53), it is important to assess whether epigenetic mechanisms are involved in DBHB's neuroprotective effects considering the efficacy of HDAC inhibition as a therapy in mouse models of stroke (54, 55).
Figure 2. The exercise factors, beta-hydroxybutyrate, irisin and lactate protect against ischemic stroke.
In addition to ketone bodies, exercise induces the liver to release multiple proteins into the blood. A recent study has revealed that exercise increases the levels of a GPI- degrading enzyme, Gpld1, in the blood of mice and of healthy elderly humans (41). Interestingly, unlike other exercise factors discussed in this minireview, liver-derived Gpld1 does not readily enter the brain (41). However, overexpression of Gpld1 in the liver of aged mice increases its levels in the plasma, significantly induces BDNF protein expression in the hippocampus and rescues impaired neurogenesis as well as age-dependent cognitive impairments observed in the radial arm water maze, Y maze and novel object recognition paradigms (41). Coagulation and complement signaling are the major cascades that are altered in response to Gpld1 overexpression and exercise. These cascades were suggested to be involved in mediating Gpld1 and exercise's effects on neurogenesis and cognition (41). Interestingly, previous work has shown that exercise activates platelets and that activated platelets promote neurogenesis by releasing factors such as platelet factor 4 (10). Indeed, platelet depletion abolishes exercise-induced neurogenesis in the hippocampus (10). It would be important to determine whether liver-derived Gpld1 is involved in the exercise-mediated platelet responses. Interestingly, in the context of stroke, Gpld1 was identified as a critical regulator of platelet activity (56). Both pharmacological inhibition and genetic ablation of Gpld1 protected from pathological thrombus formation and ischemic stroke (56, 57). As a result, Glpd1 mediates both positive and negative effects on the brain depending on context. Resolving the contradictory roles played by Gpld1is necessary and can be achieved by studying the role of exercise-induced Gpld1in stroke mouse models. This will help in determining whether this protein is involved in mediating the neuroprotective effects of exercise in stroke or whether its neuroprotective roles are restricted to healthy subjects.
In addition to the liver, exercise induces the muscle to release multiple factors that have been shown to play important roles in regulating brain health by regulating BDNF levels. These include proteins such as Cathepsin B, FNDC5, and its cleavage product irisin as well as metabolites such as lactate.
Cathepsin B (CTSB), a lysosomal cysteine protease, is released by the muscle during exercise. It mediates the positive effects of exercise on cognition by increasing adult hippocampal neurogenesis and promoting spatial memory formation (58, 59). Indeed, CTSB increases BDNF and doublecortin (DCX) levels in adult hippocampal progenitor cells (58). Exercise also increases CTSB levels in the plasma of humans, where its levels are correlated with hippocampus-dependent memory formation (58). CTSB also controls neurite outgrowth by modulating lysosomal trafficking in neurons (60). In humans, the effects of exercise on plasma CTSB levels are variable with some studies showing that long-term treadmill running increase plasma CTSB levels (58), while others showing no changes in plasma CTSB levels after 6 weeks of HIIE, or a single bout of HIIE in sedentary males (61, 62). Interestingly, studies that focus on the role of muscle-released CTSB in cerebral ischemia are sparse. In contrast, a negative role for brain-derived CTSB in cerebral ischemia has been established. For example, both genetic ablation and pharmacological inhibition of CTSB in mice protect hippocampal neurons from hypoxic/ischemic injury (63) and significantly decrease reactive oxygen species (ROS) production and neuroinflammation (64). CTSB mediates the neurotoxic polarization of microglia/macrophages, worsening hypoxia/ischemia-induced neuronal injury (63). Moreover, CTSB mediates secondary degeneration in the ipsilateral thalamus and substania nigra after focal cortical infarction (65, 66). Based on what is known, CTSB is unlikely to mediate the neuroprotective effects of exercise in stroke patients. However, more work is needed to identify which exercise paradigms consistently induce release of CTSB from the muscle and to determine whether the role of the muscle-released CTSB protein is distinct from the neuronal protein.
Other myokines such as the Fibronectin type III domain-containing protein 5 (FNDC5) and its secreted cleavage product, irisin, also mediate the positive effects of exercise on the brain by inducing hippocampal Bdnf expression (8, 67). Lactate, a metabolite released by the muscle during exercise, crosses the blood-brain barrier and activates the hippocampal PGC1a/FNDC5 pathway (9). Lactate increases the levels and activity of the lysine deacetylase Sirtuin 1(SIRT1). SIRT 1 activates the transcriptional activation complex PGC-1alpha/ERRa, which increases hippocampal Fndc5 expression. FNDC5, in turn, activates Bdnf expression, promoting learning and memory formation (9). Moreover, peripheral delivery of FNDC5 increases blood irisin levels, and also induces hippocampal Bdnf expression (8). In addition to mediating exercise's positive effects on cognition, these proteins have been shown to rescue cognitive deficits associated with neurodegenerative diseases such as Alzheimer's disease (68, 69) by inducing BDNF signaling (70) as well as behavioral deficits observed in mouse models of depression (71, 72). Irisin's antidepressant effect also involves modulation of Bdnf expression (73).
Interestingly, irisin plays important neuroprotective roles. The levels of irisin in the blood decrease after ischemic stroke in mouse models (74) and in humans (75). Decreased irisin levels were associated with poor prognosis in patients who have suffered from an ischemic stroke (75) (Figure 2). Irisin decreases brain edema through the ERK pathway, decreases infarct size through the Notch pathway, decreases oxidative stress through the TLR4/Myd8 pathway and decreases the inflammatory response through the ROS/NLP3 pathway (76). Indeed, irisin is neuroprotective both in vitro and in vivo. Irisin administration protects against OGD-induced neuronal death in vitro (74) in part by inhibiting the ROS-NLRP3 signaling pathway (77). Moreover, irisin protects against damage induced by a cerebral ischemia/reperfusion (I/R) model by modulating the Notch signaling pathway (78). Irisin treatment decreases the infarct size, brain edema and neurological deficits in mice subjected to MCAO (74). This irisin-mediated rescue of brain damage is associated with decreased apoptosis and increased cortical levels of BDNF (79). It is thought that the Akt and ERK1/2 pathways, known to be downstream effectors of BDNF signaling, mediate irisin's neuroprotective effects (74). Interestingly, 3 weeks of high intensity training resulted in increased BDNF in the brain and plasma following MCAO and this increase was dependent on the PGC-1a pathway (80). Based on these observations, both FNDC5 and irisin are likely involved in mediating the neuroprotective effects of exercise against ischemic stroke. More work is needed to establish this direct link by assessing the neuroprotective effects of exercise in Fndc5 knockout mice.
Another exercise factor that is released by the muscle is lactate. It is well-established that lactate is used as an energy substrate by the brain (81) and that neuronal uptake of astrocytic lactate is required for long-term memory formation (82). Indeed, RNA sequencing data reveals that lactate increases the expression of both neuroprotective and synaptic plasticity genes such as Bdnf , Arc, c-Fos, and Zif268 by inducing NMDA receptor activity and its downstream signaling pathway Erk1/2 in primary neuronal cultures and in cortical tissues (83, 84). We only recently identified lactate as a muscle-released exercise factor that enhances spatial memory by activating the PGC1a/FNDC5/BDNF signaling pathway in the hippocampus (9). Like other exercise factors, lactate promotes brain health and rescues from a variety of central nervous system (CNS) disorders. It enhances neurogenesis by activating the NF-kB signaling pathway following intracerebral hemorrhage (85) and rescues cognitive defects in mice subjected to fluid percussion injury [reviewed in (12)]. It also acts as an antidepressant (13, 86). Interestingly, lactate also has extensive neuroprotective roles.
Lactate mediates neuroprotection against glutamate-mediated excitotoxicity in mouse cortical neurons by engaging a network of cellular pathways involving ATP production, and activation of KATP channels (87). It also promotes resistance to H2O2-induced death in neuroblastoma cells by activating the Unfolded Protein Response (UPR) and nuclear factor erythroid 2-related factor 2 (NRF2) (88). In addition, lactate protects against OGD-induced neuronal death in rat organotypic hippocampal slices (89, 90). This pro-survival effect involves increasing the expression of the potassium channel TREK1 by activating the PKA pathway in astrocytes during ischemia (91).
Lactate also enhances neuroplasticity post-stroke. Both intracerebroventricular and systemic injections of lactate directly after reperfusion improve neurologic outcomes 48 h after cerebral ischemia (89, 90). These beneficial effects of lactate appear to be long-lasting. Improved neurological scores in the rotarod test and the beam walking test can still be observed 2 weeks after ischemia in mice subjected to MCAO and receiving intraventricular injections of lactate after reperfusion (89). There is evidence that the HCA1 receptor may mediate lactate's protective effects in neurons of the ischemic cortex after MCAO (92) (Figure 2).
Taken together, the data suggest that lactate plays important neuroprotective roles and enhances positive functional outcomes after stroke. Direct evidence that lactate is mediating exercise's protective roles after ischemic stroke remain elusive. Genetic or pharmacological inhibition of the monocarboxylate transporters (MCT2), particularly the MCT2, may aid in understanding whether both lactate and DBHB mediate exercise's neuroprotective effects after cerebral ischemia.
While traditionally regarded as a structural organ, bone has attracted attention in recent years for its endocrine functions. One protein released by osteoblasts in response to endurance exercise in mice and humans is osteocalcin (OCN) (93). A single bout of HIIE in healthy male individuals increased corticospinal excitability, BDNF and uncarboxylated OCN (uncOCN). Indeed, greater increases in BDNF were linked to increases in unOCN and irisin only in the exercising individuals, suggesting that these factors may contribute to exercise-induced BDNF increases (61). Interestingly, OCN delivery was previously shown to be sufficient to improve memory and decrease anxiety-like behaviors in aging mice (94). These positive effects of OCN were mediated by directly increasing hippocampal BDNF levels through activation of the Gpr158, an orphan G protein-coupled receptor (5).
Recent studies have shown that OCN enhances neuroplasticity by improving outcomes after ischemic stroke (95). Stroke patients who had better outcomes had higher serum osteocalcin levels than those whose National Institutes of Health Stroke Scale (NIHSS) scores did not improve. At the molecular level, metabolic reprogramming and decreased pyroptosis were responsible for the neuroprotective effect of OCN in an OGD model (95). Even though current work has not shown that OCN directly mediates the beneficial effects of exercise in the brain, the available evidence suggests that it may be a candidate exercise factor that is worth assessing in animal models of stroke.
Exercise has profound positive effects on the brain including induction of synaptic plasticity, neurogenesis and enhancement of learning and memory formation. The positive effects of exercise are thought to be mediated by multiple exercise factors that induce BDNF signaling. Exercise is also effective in ameliorating the detrimental symptoms of ischemic strokes in animal models and in humans. Even though some of the molecular pathways underlying the neuroprotective effects of exercise are known, it is clear that not all the currently known exercise factors are involved in mediating these effects (Figure 2). It is important to conduct a systemic analysis to identify which exercise-induced blood-borne factors, alone or in combination, mediate exercise's neuroprotective effects in cerebral ischemia. Indeed, systematic studies assessing how the frequency, intensity, and duration of the exercise impact its ability to enhance the production of these blood-borne factors are needed. Moreover, it is important to understand how the levels of these factors are modulated when individuals exercise prior or after stroke. These along with experiments designed to assess which exercise factors are responsible for mediating exercise's prophylactic and therapeutic effects in the context of stroke will allow us to develop targeted therapeutic approaches. Metabolites such as DBHB, lactate and proteins such as irisin, initially identified as exercise factors that induce Bdnf expression (7–9) and promote learning and memory formation, are the leading candidates of the currently known exercise factors (Figure 1).
JS and SS wrote and edited the manuscript. All authors contributed to the article and approved the submitted version.
This work was supported by the Lebanese American University.
The authors declare that the research was conducted in the absence of any commercial or financial relationships that could be construed as a potential conflict of interest.
1. Di Carlo A. Human and economic burden of stroke. Age Ageing. (2009) 38:4–5. doi: 10.1093/ageing/afn282
2. Rajsic S, Gothe H, Borba HH, Sroczynski G, Vujicic J, Toell T, et al. Economic burden of stroke: a systematic review on post-stroke care. Eur J Health Econ. (2019) 20:107–34. doi: 10.1007/s10198-018-0984-0
3. Di Raimondo D, Rizzo G, Musiari G, Tuttolomondo A, Pinto A. Role of regular physical activity in neuroprotection against acute ischemia. Int J Mol Sci. (2020) 21:86. doi: 10.3390/ijms21239086
4. Sleiman SF, Chao MV. Downstream consequences of exercise through the action of BDNF. Brain Plast. (2015) 1:143–8. doi: 10.3233/BPL-150017
5. Khrimian L, Obri A, Ramos-Brossier M, Rousseaud A, Moriceau S, Nicot AS, et al. Gpr158 mediates osteocalcin's regulation of cognition. J Exp Med. (2017) 214:2859–73. doi: 10.1084/jem.20171320
6. Kosmidis S, Polyzos A, Harvey L, Youssef M, Denny CA, Dranovsky A, et al. RbAp48 protein is a critical component of GPR158/OCN signaling and ameliorates age-related memory loss. Cell Rep. (2018) 25:959–73.e6. doi: 10.1016/j.celrep.2018.09.077
7. Sleiman SF, Henry J, Al-Haddad R, El Hayek L, Abou Haidar E, Stringer T, et al. Exercise promotes the expression of brain derived neurotrophic factor (BDNF) through the action of the ketone body beta-hydroxybutyrate. Elife. (2016) 5:e15092. doi: 10.7554/eLife.15092
8. Wrann CD, White JP, Salogiannnis J, Laznik-Bogoslavski D, Wu J, Ma D, et al. Exercise induces hippocampal BDNF through a PGC-1alpha/FNDC5 pathway. Cell Metab. (2013) 18:649–59. doi: 10.1016/j.cmet.2013.09.008
9. El Hayek L, Khalifeh M, Zibara V, Abi Assaad R, Emmanuel N, Karnib N, et al. Lactate mediates the effects of exercise on learning and memory through SIRT1-dependent activation of hippocampal brain-derived neurotrophic factor (BDNF). J Neurosci. (2019) 39:2369–82. doi: 10.1523/JNEUROSCI.1661-18.2019
10. Leiter O, Seidemann S, Overall RW, Ramasz B, Rund N, Schallenberg S, et al. Exercise-induced activated platelets increase adult hippocampal precursor proliferation and promote neuronal differentiation. Stem Cell Rep. (2019) 12:667–79. doi: 10.1016/j.stemcr.2019.02.009
11. Fujimura H, Altar CA, Chen R, Nakamura T, Nakahashi T, Kambayashi J, et al. Brain-derived neurotrophic factor is stored in human platelets and released by agonist stimulation. Thromb Haemost. (2002) 87:728–34. doi: 10.1055/s-0037-1613072
12. Stephan JS, Sleiman SF. Exercise factors as potential mediators of cognitive rehabilitation following traumatic brain injury. Curr Opin Neurol. (2019) 32:808–14. doi: 10.1097/WCO.0000000000000754
13. Karnib N, El-Ghandour R, El Hayek L, Nasrallah P, Khalifeh M, Barmo N, et al. Lactate is an antidepressant that mediates resilience to stress by modulating the hippocampal levels and activity of histone deacetylases. Neuropsychopharmacology. (2019) 44:1152–62. doi: 10.1038/s41386-019-0313-z
14. Aboutaleb N, Shamsaei N, Khaksari M, Erfani S, Rajabi H, Nikbakht F. Pre-ischemic exercise reduces apoptosis in hippocampal CA3 cells after cerebral ischemia by modulation of the Bax/Bcl-2 proteins ratio and prevention of caspase-3 activation. J Physiol Sci. (2015) 65:435–43. doi: 10.1007/s12576-015-0382-7
15. Aboutaleb N, Shamsaei N, Rajabi H, Khaksari M, Erfani S, Nikbakht F, et al. Protection of hippocampal CA1 neurons against ischemia/reperfusion injury by exercise preconditioning via modulation of Bax/Bcl-2 ratio and prevention of caspase-3 activation. Basic Clin Neurosci. (2016) 7:21–9.
16. Arrick DM, Yang S, Li C, Cananzi S, Mayhan WG. Vigorous exercise training improves reactivity of cerebral arterioles and reduces brain injury following transient focal ischemia. Microcirculation. (2014) 21:516–23. doi: 10.1111/micc.12127
17. Chaudhry K, Rogers R, Guo M, Lai Q, Goel G, Liebelt B, et al. Matrix metalloproteinase-9 (MMP-9) expression and extracellular signal-regulated kinase 1 and 2 (ERK1/2) activation in exercise-reduced neuronal apoptosis after stroke. Neurosci Lett. (2010) 474:109–14. doi: 10.1016/j.neulet.2010.03.020
18. Davis W, Mahale S, Carranza A, Cox B, Hayes K, Jimenez D, et al. Exercise pre-conditioning ameliorates blood-brain barrier dysfunction in stroke by enhancing basal lamina. Neurol Res. (2007) 29:382–7. doi: 10.1179/016164107X204701
19. Deplanque D, Masse I, Libersa C, Leys D, Bordet R. Previous leisure-time physical activity dose dependently decreases ischemic stroke severity. Stroke Res Treat. (2012) 2012:614925. doi: 10.1155/2012/614925
20. Islam MR, Young MF, Wrann CD. Neuroprotective potential of exercise preconditioning in stroke. Cond Med. (2017) 1:27–34.
21. Mattson MP. Energy intake and exercise as determinants of brain health and vulnerability to injury and disease. Cell Metab. (2012) 16:706–22. doi: 10.1016/j.cmet.2012.08.012
22. Oberlin LE, Waiwood AM, Cumming TB, Marsland AL, Bernhardt J, Erickson KI. Effects of physical activity on poststroke cognitive function: a meta-analysis of randomized controlled trials. Stroke. (2017) 48:3093–100. doi: 10.1161/STROKEAHA.117.017319
23. Shamsaei N, Erfani S, Fereidoni M, Shahbazi A. Neuroprotective effects of exercise on brain edema and neurological movement disorders following the cerebral ischemia and reperfusion in rats. Basic Clin Neurosci. (2017) 8:77–84. doi: 10.15412/J.BCN.03080110
24. Gao Y, Zhao Y, Pan J, Yang L, Huang T, Feng X, et al. Treadmill exercise promotes angiogenesis in the ischemic penumbra of rat brains through caveolin-1/VEGF signaling pathways. Brain Res. (2014) 1585:83–90. doi: 10.1016/j.brainres.2014.08.032
25. Otsuka S, Sakakima H, Sumizono M, Takada S, Terashi T, Yoshida Y. The neuroprotective effects of preconditioning exercise on brain damage and neurotrophic factors after focal brain ischemia in rats. Behav Brain Res. (2016) 303:9–18. doi: 10.1016/j.bbr.2016.01.049
26. Pianta S, Lee JY, Tuazon JP, Castelli V, Mantohac LM, Tajiri N, et al. A short bout of exercise prior to stroke improves functional outcomes by enhancing angiogenesis. Neuromolecular Med. (2019) 21:517–28. doi: 10.1007/s12017-019-08533-x
27. Tang Y, Zhang Y, Zheng M, Chen J, Chen H, Liu N. Effects of treadmill exercise on cerebral angiogenesis and MT1-MMP expression after cerebral ischemia in rats. Brain Behav. (2018) 8:e01079. doi: 10.1002/brb3.1079
28. Rezaei R, Nasoohi S, Haghparast A, Khodagholi F, Bigdeli MR, Nourshahi M. High intensity exercise preconditioning provides differential protection against brain injury following experimental stroke. Life Sci. (2018) 207:30–5. doi: 10.1016/j.lfs.2018.03.007
29. Wang J, Liu H, Chen S, Zhang W, Chen Y, Yang Y. Moderate exercise has beneficial effects on mouse ischemic stroke by enhancing the functions of circulating endothelial progenitor cell-derived exosomes. Exp Neurol. (2020) 330:113325. doi: 10.1016/j.expneurol.2020.113325
30. Zhang L, Yang X, Yin M, Yang H, Li L, Parashos A, et al. An animal trial on the optimal time and intensity of exercise after stroke. Med Sci Sports Exerc. (2020) 52:1699–709. doi: 10.1249/MSS.0000000000002318
31. Hong M, Kim M, Kim TW, Park SS, Kim MK, Park YH, et al. Treadmill exercise improves motor function and short-term memory by enhancing synaptic plasticity and neurogenesis in photothrombotic stroke mice. Int Neurourol J. (2020) 24(Suppl. 1):S28–38. doi: 10.5213/inj.2040158.079
32. Cheng J, Shen W, Jin L, Pan J, Zhou Y, Pan G, et al. Treadmill exercise promotes neurogenesis and myelin repair via upregulating Wnt/betacatenin signaling pathways in the juvenile brain following focal cerebral ischemia/reperfusion. Int J Mol Med. (2020) 45:1447–63. doi: 10.3892/ijmm.2020.4515
33. Ciancarelli I, De Amicis D, Di Massimo C, Carolei A, Ciancarelli MG. Oxidative stress in post-acute ischemic stroke patients after intensive neurorehabilitation. Curr Neurovasc Res. (2012) 9:266–73. doi: 10.2174/156720212803530717
34. Li F, Geng X, Huber C, Stone C, Ding Y. In search of a dose: the functional and molecular effects of exercise on post-stroke rehabilitation in rats. Front Cell Neurosci. (2020) 14:186. doi: 10.3389/fncel.2020.00186
35. Li F, Pendy JT Jr., Ding JN, Peng C, Li X, Shen J, et al. Exercise rehabilitation immediately following ischemic stroke exacerbates inflammatory injury. Neurol Res. (2017) 39:530–7. doi: 10.1080/01616412.2017.1315882
36. Ma Y, He M, Qiang L. Exercise therapy downregulates the overexpression of TLR4, TLR2, MyD88 and NF-kappaB after cerebral ischemia in rats. Int J Mol Sci. (2013) 14:3718–33. doi: 10.3390/ijms14023718
37. Zhang Q, Wu Y, Zhang P, Sha H, Jia J, Hu Y, et al. Exercise induces mitochondrial biogenesis after brain ischemia in rats. Neuroscience. (2012) 205:10–7. doi: 10.1016/j.neuroscience.2011.12.053
38. El-Tamawy MS, Abd-Allah F, Ahmed SM, Darwish MH, Khalifa HA. Aerobic exercises enhance cognitive functions and brain derived neurotrophic factor in ischemic stroke patients. NeuroRehabilitation. (2014) 34:209–13. doi: 10.3233/NRE-131020
39. Stanne TM, Aberg ND, Nilsson S, Jood K, Blomstrand C, Andreasson U, et al. Low circulating acute brain-derived neurotrophic factor levels are associated with poor long-term functional outcome after ischemic stroke. Stroke. (2016) 47:1943–5. doi: 10.1161/STROKEAHA.115.012383
40. Kotlega D, Peda B, Zembron-Lacny A, Golab-Janowska M, Nowacki P. The role of brain-derived neurotrophic factor and its single nucleotide polymorphisms in stroke patients. Neurol Neurochir Pol. (2017) 51:240–6. doi: 10.1016/j.pjnns.2017.02.008
41. Horowitz AM, Fan X, Bieri G, Smith LK, Sanchez-Diaz CI, Schroer AB, et al. Blood factors transfer beneficial effects of exercise on neurogenesis and cognition to the aged brain. Science. (2020) 369:167–73. doi: 10.1126/science.aaw2622
42. Benjamin JS, Pilarowski GO, Carosso GA, Zhang L, Huso DL, Goff LA, et al. A ketogenic diet rescues hippocampal memory defects in a mouse model of Kabuki syndrome. Proc Natl Acad Sci USA. (2017) 114:125–30. doi: 10.1073/pnas.1611431114
43. Newman JC, Covarrubias AJ, Zhao M, Yu X, Gut P, Ng CP, et al. Ketogenic diet reduces midlife mortality and improves memory in aging mice. Cell Metab. (2017) 26:547–57.e8. doi: 10.1016/j.cmet.2017.08.004
44. Roberts MN, Wallace MA, Tomilov AA, Zhou Z, Marcotte GR, Tran D, et al. A ketogenic diet extends longevity and healthspan in adult mice. Cell Metab. (2018) 27:1156. doi: 10.1016/j.cmet.2018.04.005
45. Norwitz NG, Hu MT, Clarke K. The mechanisms by which the ketone body D-beta-hydroxybutyrate may improve the multiple cellular pathologies of Parkinson's disease. Front Nutr. (2019) 6:63. doi: 10.3389/fnut.2019.00063
46. Yin JX, Maalouf M, Han P, Zhao M, Gao M, Dharshaun T, et al. Ketones block amyloid entry and improve cognition in an Alzheimer's model. Neurobiol Aging. (2016) 39:25–37. doi: 10.1016/j.neurobiolaging.2015.11.018
47. Chen L, Miao Z, Xu X. beta-hydroxybutyrate alleviates depressive behaviors in mice possibly by increasing the histone3-lysine9-beta-hydroxybutyrylation. Biochem Biophys Res Commun. (2017) 490:117–22. doi: 10.1016/j.bbrc.2017.05.184
48. Puchowicz MA, Zechel JL, Valerio J, Emancipator DS, Xu K, Pundik S, et al. Neuroprotection in diet-induced ketotic rat brain after focal ischemia. J Cereb Blood Flow Metab. (2008) 28:1907–16. doi: 10.1038/jcbfm.2008.79
49. Suzuki M, Suzuki M, Kitamura Y, Mori S, Sato K, Dohi S, et al. Beta-hydroxybutyrate, a cerebral function improving agent, protects rat brain against ischemic damage caused by permanent and transient focal cerebral ischemia. Jpn J Pharmacol. (2002) 89:36–43. doi: 10.1254/jjp.89.36
50. Guo M, Wang X, Zhao Y, Yang Q, Ding H, Dong Q, et al. Ketogenic diet improves brain ischemic tolerance and inhibits NLRP3 inflammasome activation by preventing Drp1-mediated mitochondrial fission and endoplasmic reticulum stress. Front Mol Neurosci. (2018) 11:86. doi: 10.3389/fnmol.2018.00086
51. Yin J, Han P, Tang Z, Liu Q, Shi J. Sirtuin 3 mediates neuroprotection of ketones against ischemic stroke. J Cereb Blood Flow Metab. (2015) 35:1783–9. doi: 10.1038/jcbfm.2015.123
52. Rahman M, Muhammad S, Khan MA, Chen H, Ridder DA, Muller-Fielitz H, et al. The beta-hydroxybutyrate receptor HCA2 activates a neuroprotective subset of macrophages. Nat Commun. (2014) 5:3944. doi: 10.1038/ncomms4944
53. Shimazu T, Hirschey MD, Newman J, He W, Shirakawa K, Le Moan N, et al. Suppression of oxidative stress by beta-hydroxybutyrate, an endogenous histone deacetylase inhibitor. Science. (2013) 339:211–4. doi: 10.1126/science.1227166
54. Langley B, Brochier C, Rivieccio MA. Targeting histone deacetylases as a multifaceted approach to treat the diverse outcomes of stroke. Stroke. (2009) 40:2899–905. doi: 10.1161/STROKEAHA.108.540229
55. Langley B, D'Annibale MA, Suh K, Ayoub I, Tolhurst A, Bastan B, et al. Pulse inhibition of histone deacetylases induces complete resistance to oxidative death in cortical neurons without toxicity and reveals a role for cytoplasmic p21(waf1/cip1) in cell cycle-independent neuroprotection. J Neurosci. (2008) 28:163–76. doi: 10.1523/JNEUROSCI.3200-07.2008
56. Elvers M, Stegner D, Hagedorn I, Kleinschnitz C, Braun A, Kuijpers ME, et al. Impaired alpha(IIb)beta(3) integrin activation and shear-dependent thrombus formation in mice lacking phospholipase D1. Sci Signal. (2010) 3:ra1. doi: 10.1126/scisignal.2000551
57. Stegner D, Thielmann I, Kraft P, Frohman MA, Stoll G, Nieswandt B. Pharmacological inhibition of phospholipase D protects mice from occlusive thrombus formation and ischemic stroke–brief report. Arterioscler Thromb Vasc Biol. (2013) 33:2212–7. doi: 10.1161/ATVBAHA.113.302030
58. Moon HY, Becke A, Berron D, Becker B, Sah N, Benoni G, et al. Running-induced systemic cathepsin B secretion is associated with memory function. Cell Metab. (2016) 24:332–40. doi: 10.1016/j.cmet.2016.05.025
59. van Praag H, Christie BR, Sejnowski TJ, Gage FH. Running enhances neurogenesis, learning, and long-term potentiation in mice. Proc Natl Acad Sci USA. (1999) 96:13427–31. doi: 10.1073/pnas.96.23.13427
60. Jiang M, Meng J, Zeng F, Qing H, Hook G, Hook V, et al. Cathepsin B inhibition blocks neurite outgrowth in cultured neurons by regulating lysosomal trafficking and remodeling. J Neurochem. (2020) 155:300–12. doi: 10.1111/jnc.15032
61. Nicolini C, Michalski B, Toepp SL, Turco CV, D'Hoine T, Harasym D, et al. A Single bout of high-intensity interval exercise increases corticospinal excitability, brain-derived neurotrophic factor, and uncarboxylated osteolcalcin in sedentary, healthy males. Neuroscience. (2020) 437:242–55. doi: 10.1016/j.neuroscience.2020.03.042
62. Nicolini C, Toepp S, Harasym D, Michalski B, Fahnestock M, Gibala MJ, et al. No changes in corticospinal excitability, biochemical markers, and working memory after six weeks of high-intensity interval training in sedentary males. Physiol Rep. (2019) 7:e14140. doi: 10.14814/phy2.14140
63. Ni J, Wu Z, Peterts C, Yamamoto K, Qing H, Nakanishi H. The critical role of proteolytic relay through cathepsins B and E in the phenotypic change of microglia/macrophage. J Neurosci. (2015) 35:12488–501. doi: 10.1523/JNEUROSCI.1599-15.2015
64. Ni J, Wu Z, Stoka V, Meng J, Hayashi Y, Peters C, et al. Increased expression and altered subcellular distribution of cathepsin B in microglia induce cognitive impairment through oxidative stress and inflammatory response in mice. Aging Cell. (2019) 18:e12856. doi: 10.1111/acel.12856
65. Zuo X, Hou Q, Jin J, Chen X, Zhan L, Tang Y, et al. Inhibition of cathepsins B induces neuroprotection against secondary degeneration in ipsilateral substantia nigra after focal cortical infarction in adult male rats. Front Aging Neurosci. (2018) 10:125. doi: 10.3389/fnagi.2018.00125
66. Zuo X, Hou Q, Jin J, Zhan L, Li X, Sun W, et al. Inhibition of cathepsin B alleviates secondary degeneration in ipsilateral thalamus after focal cerebral infarction in adult rats. J Neuropathol Exp Neurol. (2016) 75:816–26. doi: 10.1093/jnen/nlw054
67. Belviranli M, Okudan N. Exercise training protects against aging-induced cognitive dysfunction via activation of the hippocampal PGC-1alpha/FNDC5/BDNF pathway. Neuromolecular Med. (2018) 20:386–400. doi: 10.1007/s12017-018-8500-3
68. Chen X, Gan L. An exercise-induced messenger boosts memory in Alzheimer's disease. Nat Med. (2019) 25:20–1. doi: 10.1038/s41591-018-0311-4
69. Lourenco MV, Frozza RL, de Freitas GB, Zhang H, Kincheski GC, Ribeiro FC, et al. Exercise-linked FNDC5/irisin rescues synaptic plasticity and memory defects in Alzheimer's models. Nat Med. (2019) 25:165–75. doi: 10.1038/s41591-018-0275-4
70. Xia DY, Huang X, Bi CF, Mao LL, Peng LJ, Qian HR. PGC-1alpha or FNDC5 is involved in modulating the effects of abeta1-42 oligomers on suppressing the expression of BDNF, a beneficial factor for inhibiting neuronal apoptosis, abeta deposition and cognitive decline of APP/PS1 Tg mice. Front Aging Neurosci. (2017) 9:65. doi: 10.3389/fnagi.2017.00065
71. Uysal N, Yuksel O, Kizildag S, Yuce Z, Gumus H, Karakilic A, et al. Regular aerobic exercise correlates with reduced anxiety and incresed levels of irisin in brain and white adipose tissue. Neurosci Lett. (2018) 676:92–7. doi: 10.1016/j.neulet.2018.04.023
72. Wang S, Pan J. Irisin ameliorates depressive-like behaviors in rats by regulating energy metabolism. Biochem Biophys Res Commun. (2016) 474:22–8. doi: 10.1016/j.bbrc.2016.04.047
73. Siteneski A, Cunha MP, Lieberknecht V, Pazini FL, Gruhn K, Brocardo PS, et al. Central irisin administration affords antidepressant-like effect and modulates neuroplasticity-related genes in the hippocampus and prefrontal cortex of mice. Prog Neuropsychopharmacol Biol Psychiatry. (2018) 84:294–303. doi: 10.1016/j.pnpbp.2018.03.004
74. Li DJ, Li YH, Yuan HB, Qu LF, Wang P. The novel exercise-induced hormone irisin protects against neuronal injury via activation of the Akt and ERK1/2 signaling pathways and contributes to the neuroprotection of physical exercise in cerebral ischemia. Metabolism. (2017) 68:31–42. doi: 10.1016/j.metabol.2016.12.003
75. Tu WJ, Qiu HC, Cao JL, Liu Q, Zeng XW, Zhao JZ. Decreased concentration of irisin is associated with poor functional outcome in ischemic stroke. Neurotherapeutics. (2018) 15:1158–67. doi: 10.1007/s13311-018-0651-2
76. Liu Y, Zhu C, Guo J, Chen Y, Meng C. The neuroprotective effect of irisin in ischemic stroke. Front Aging Neurosci. (2020) 12:588958. doi: 10.3389/fnagi.2020.588958
77. Peng J, Deng X, Huang W, Yu JH, Wang JX, Wang JP, et al. Irisin protects against neuronal injury induced by oxygen-glucose deprivation in part depends on the inhibition of ROS-NLRP3 inflammatory signaling pathway. Mol Immunol. (2017) 91:185–94. doi: 10.1016/j.molimm.2017.09.014
78. Jin Z, Guo P, Li X, Ke J, Wang Y, Wu H. Neuroprotective effects of irisin against cerebral ischemia/ reperfusion injury via Notch signaling pathway. Biomed Pharmacother. (2019) 120:109452. doi: 10.1016/j.biopha.2019.109452
79. Asadi Y, Gorjipour F, Behrouzifar S, Vakili A. Irisin peptide protects brain against ischemic injury through reducing apoptosis and enhancing BDNF in a rodent model of stroke. Neurochem Res. (2018) 43:1549–60. doi: 10.1007/s11064-018-2569-9
80. Abbasian S, Asghar Ravasi A. The effect of antecedent-conditioning high-intensity interval training on BDNF regulation through PGC-1alpha pathway following cerebral ischemia. Brain Res. (2020) 1729:146618. doi: 10.1016/j.brainres.2019.146618
81. Wyss MT, Jolivet R, Buck A, Magistretti PJ, Weber B. In vivo evidence for lactate as a neuronal energy source. J Neurosci. (2011) 31:7477–85. doi: 10.1523/JNEUROSCI.0415-11.2011
82. Suzuki A, Stern SA, Bozdagi O, Huntley GW, Walker RH, Magistretti PJ, et al. Astrocyte-neuron lactate transport is required for long-term memory formation. Cell. (2011) 144:810–23. doi: 10.1016/j.cell.2011.02.018
83. Margineanu MB, Mahmood H, Fiumelli H, Magistretti PJ. L-lactate regulates the expression of synaptic plasticity and neuroprotection genes in cortical neurons: a transcriptome analysis. Front Mol Neurosci. (2018) 11:375. doi: 10.3389/fnmol.2018.00375
84. Yang J, Ruchti E, Petit JM, Jourdain P, Grenningloh G, Allaman I, et al. Lactate promotes plasticity gene expression by potentiating NMDA signaling in neurons. Proc Natl Acad Sci USA. (2014) 111:12228–33. doi: 10.1073/pnas.1322912111
85. Zhou J, Liu T, Guo H, Cui H, Li P, Feng D, et al. Lactate potentiates angiogenesis and neurogenesis in experimental intracerebral hemorrhage. Exp Mol Med. (2018) 50:1–12. doi: 10.1038/s12276-018-0113-2
86. Carrard A, Elsayed M, Margineanu M, Boury-Jamot B, Fragniere L, Meylan EM, et al. Peripheral administration of lactate produces antidepressant-like effects. Mol Psychiatry. (2018) 23:488. doi: 10.1038/mp.2016.237
87. Jourdain P, Allaman I, Rothenfusser K, Fiumelli H, Marquet P, Magistretti PJ. L-Lactate protects neurons against excitotoxicity: implication of an ATP-mediated signaling cascade. Sci Rep. (2016) 6:21250. doi: 10.1038/srep21250
88. Tauffenberger A, Fiumelli H, Almustafa S, Magistretti PJ. Lactate and pyruvate promote oxidative stress resistance through hormetic ROS signaling. Cell Death Dis. (2019) 10:653. doi: 10.1038/s41419-019-1877-6
89. Berthet C, Castillo X, Magistretti PJ, Hirt L. New evidence of neuroprotection by lactate after transient focal cerebral ischaemia: extended benefit after intracerebroventricular injection and efficacy of intravenous administration. Cerebrovasc Dis. (2012) 34:329–35. doi: 10.1159/000343657
90. Berthet C, Lei H, Thevenet J, Gruetter R, Magistretti PJ, Hirt L. Neuroprotective role of lactate after cerebral ischemia. J Cereb Blood Flow Metab. (2009) 29:1780–9. doi: 10.1038/jcbfm.2009.97
91. Banerjee A, Ghatak S, Sikdar SK. l-Lactate mediates neuroprotection against ischaemia by increasing TREK1 channel expression in rat hippocampal astrocytes in vitro. J Neurochem. (2016) 138:265–81. doi: 10.1111/jnc.13638
92. Castillo X, Rosafio K, Wyss MT, Drandarov K, Buck A, Pellerin L, et al. A probable dual mode of action for both L- and D-lactate neuroprotection in cerebral ischemia. J Cereb Blood Flow Metab. (2015) 35:1561–9. doi: 10.1038/jcbfm.2015.115
93. Mera P, Laue K, Ferron M, Confavreux C, Wei J, Galan-Diez M, et al. Osteocalcin signaling in myofibers is necessary and sufficient for optimum adaptation to exercise. Cell Metab. (2016) 23:1078–92. doi: 10.1016/j.cmet.2016.05.004
94. Oury F, Khrimian L, Denny CA, Gardin A, Chamouni A, Goeden N, et al. Maternal and offspring pools of osteocalcin influence brain development and functions. Cell. (2013) 155:228–41. doi: 10.1016/j.cell.2013.08.042
Keywords: exercise factors, beta-hydroxybutyrate, irisin, lactate, BDNF, stroke, osteocalcin
Citation: Stephan JS and Sleiman SF (2021) Exercise Factors Released by the Liver, Muscle, and Bones Have Promising Therapeutic Potential for Stroke. Front. Neurol. 12:600365. doi: 10.3389/fneur.2021.600365
Received: 29 August 2020; Accepted: 03 May 2021;
Published: 24 May 2021.
Edited by:
Rajiv Ram Ratan, Burke Neurological Institute (BNI), United StatesReviewed by:
Dafin F. Muresanu, Iuliu Haţieganu University of Medicine and Pharmacy, RomaniaCopyright © 2021 Stephan and Sleiman. This is an open-access article distributed under the terms of the Creative Commons Attribution License (CC BY). The use, distribution or reproduction in other forums is permitted, provided the original author(s) and the copyright owner(s) are credited and that the original publication in this journal is cited, in accordance with accepted academic practice. No use, distribution or reproduction is permitted which does not comply with these terms.
*Correspondence: Sama F. Sleiman, c2FtYS5zbGVpbWFuMDFAbGF1LmVkdS5sYg==; Joseph S. Stephan, am9zZXBoLnN0ZXBoYW5AbGF1LmVkdS5sYg==
Disclaimer: All claims expressed in this article are solely those of the authors and do not necessarily represent those of their affiliated organizations, or those of the publisher, the editors and the reviewers. Any product that may be evaluated in this article or claim that may be made by its manufacturer is not guaranteed or endorsed by the publisher.
Research integrity at Frontiers
Learn more about the work of our research integrity team to safeguard the quality of each article we publish.