- 1Department of Rehabilitation Sciences, KU Leuven—University of Leuven, Leuven, Belgium
- 2RevArte—Rehabilitation Hospital Antwerp, Antwerp, Belgium
- 3Jessa Hospital Herk-de-Stad, Herk-de-Stad, Belgium
- 4Department Acquired Brain Injury, University Hospitals Leuven, Pellenberg, Belgium
Background: Somatosensory function plays an important role in motor learning. More than half of the stroke patients have somatosensory impairments in the upper limb, which could hamper recovery.
Question: Is sensorimotor upper limb (UL) therapy of more benefit for motor and somatosensory outcome than motor therapy?
Design: Randomized assessor- blinded multicenter controlled trial with block randomization stratified for neglect, severity of motor impairment, and type of stroke.
Participants: 40 first-ever stroke patients with UL sensorimotor impairments admitted to the rehabilitation center.
Intervention: Both groups received 16 h of additional therapy over 4 weeks consisting of sensorimotor (N = 22) or motor (N = 18) UL therapy.
Outcome measures: Action Research Arm test (ARAT) as primary outcome, and other motor and somatosensory measures were assessed at baseline, post-intervention and after 4 weeks follow-up.
Results: No significant between-group differences were found for change scores in ARAT or any somatosensory measure between the three time points. For UL impairment (Fugl-Meyer assessment), a significant greater improvement was found for the motor group compared to the sensorimotor group from baseline to post-intervention [mean (SD) improvement 14.65 (2.19) vs. 5.99 (2.06); p = 0.01] and from baseline to follow-up [17.38 (2.37) vs. 6.75 (2.29); p = 0.003].
Conclusion: UL motor therapy may improve motor impairment more than UL sensorimotor therapy in patients with sensorimotor impairments in the early rehabilitation phase post stroke. For these patients, integrated sensorimotor therapy may not improve somatosensory function and may be less effective for motor recovery.
Clinical Trial Registration: www.ClinicalTrials.gov, identifier NCT03236376.
Introduction
Somatosensory information is processed when interacting with the environment by touching and manipulating objects. Sensation arising from skin, muscles and joints constitutes the somatosensory ability. Somatosensory function can be divided in three modalities. First, the exteroceptive function, consisting of light touch, temperature and pain sensations. Second, proprioceptive function existing of position, movement and vibration sense. Last, the higher cortical or discriminative function consisting of sharp/dull discrimination, stereognosis, and graphesthesia (1). Somatosensory upper limb (UL) impairment is common after stroke and negatively impacts upon activities of daily living. Approximately 50% of patients encounter somatosensory dysfunction (2). Differences in prevalence rates are reported for different modalities. Exteroception is impaired in 7–53% of patients, 34–64% encounter proprioceptive deficits and 31–89% have impaired higher cortical function (3). Moreover, the majority of patients encounter an impairment in more than one modality. A longitudinal study of our research group indicated that in the first week and at 6 months post stroke, respectively, 66 and 28% of the patients with an UL impairment encounter somatosensory impairments in more than one modality, and 50 and 13% in all three modalities (4).
Somatosensory function is postulated to form an important factor within the motor learning feedforward- feedback mechanism (5–7). Lesion studies in animals and humans reported impaired motor control after a focal primary somatosensory cortex (S1) lesion (8). Since motor learning is a key mechanism for stroke recovery, somatosensory impairments may thus affect motor outcome (3, 7–9). A review of Coupar et al. (9) showed that an intact somatosensory function positively influences motor outcome. More specifically, the presence of somatosensory evoked potentials is reported as a predictor for improved motor recovery (9). Furthermore, the absence of cortical activation after peripheral somatosensory stimulation is associated with poorer outcome (9). Clinically, patients with more severe somatosensory impairments are reported to have more reduced recovery of dexterity, manipulation skills, grip force regulation and pincer grip (3). Additionally, longer hospital stay, more social isolation and lower perceived physical activity are reported in patients with somatosensory impairments compared to patients without somatosensory impairments (3). Recently, the study of Ingemanson et al. (10) described that proprioceptive impairments at baseline were a negative predictor for treatment outcome even after correction for baseline motor impairment. They reported that 56% of the variation in treatment outcome of robot-assisted finger therapy could be explained by somatosensory system injury together with ipsilesional primary motor cortex (M1) and secondary somatosensory cortex (S2) connectivity.
Somatosensory therapy can improve somatosensory function. Serrada et al. (11) reported a moderate positive effect for passive somatosensory therapy such as peripheral stimulation, thermal stimulation and intermittent compression therapy. No evidence was presented for active somatosensory therapy such as somatosensory discrimination training due to heterogeneity in outcome measures. However, a positive effect was suggested since all studies reported a positive effect on outcome. The effect of somatosensory therapy on motor function is debated. Grant and colleagues (12) reviewed the effect of somatosensory stimulation on motor performance. They found moderate evidence that somatosensory stimulation does not improve motor performance. Yilmazer et al. (13) on the other hand, showed limited evidence for passive somatosensory therapy and some evidence for the effect of active somatosensory therapy on motor function. Nevertheless, when aiming at improving motor function, a pure somatosensory approach may not be sufficient as it is known that task-specific motor training is effective in improving motor outcome. Due to the coupling between somatosensation and movement in the motor learning mechanism, it may be more beneficial for motor outcome to integrate somatosensory and motor therapy into a sensorimotor approach, than providing motor therapy alone.
The effect of integrated sensorimotor therapy is underinvestigated. A recent systematic scoping review on combined somatosensory and motor training concluded that “combined somatosensory and motor training interventions have potential but cannot be recommended to improve upper limb function after stroke in clinical practice due to insufficient evidence of their efficacy” (14). de Diego et al. (15) showed a positive effect of 10 sessions of sensory stimulation combined with functional activity training in chronic stroke patients on motor and somatosensory function compared to conventional therapy. Similarly, Machackova reported more motor improvement and additional sensory recovery in the group receiving 18 h of somatosensory stimulation combined with standard motor therapy compared to functional training (16). Furthermore, 30 h of afferent stimulation combined with mirror therapy was found to induce greater motor improvements and less synergetic shoulder abduction compared to mirror therapy or functional training only (17). Last, Byl et al. investigated the effect of dosage on learning-based sensorimotor training. They reported an improvement of functional independence and/or somatosensory function for all dosages but the greatest improvements for patients who received the highest intensity dosage of 72 h of therapy (18). However, no between-group differences in improvement were reported.
In summary, the important role of somatosensory function for motor performance is well-established. Post stroke, the additional effect of somatosensory function and the integration of this function in a sensorimotor therapy program on motor recovery is still poorly understood. Therefore, in this study we compared the effect of a newly developed UL sensorimotor therapy vs. motor therapy on UL motor and somatosensory function and functional outcome post stroke. Our research question is “Is sensorimotor UL therapy of more benefit for motor and somatosensory outcome than motor therapy?” We hypothesized integrated sensorimotor therapy to be more beneficial for improving UL motor and somatosensory function than motor therapy.
Materials and Methods
Design
The methods of our assessor-blinded multicenter randomized controlled trial are described in detail elsewhere (19). We provide a summary below. This trial is registered at clinicaltrails.gov (NCT03236376) and was approved by the ethical committee of UZ/KU Leuven (s60278). The study has been performed following the principles of the Declaration of Helsinki. Before inclusion, participants received both verbal and written information about the study prior to providing written informed consent. Patients within 8 weeks post stroke were randomized (computer-generated) to a 4 weeks additional intervention, based on a block randomization with type of stroke, presence of neglect and UL motor impairment severity (based on the ability to perform active wrist and finger extension) as stratification factors. We used concealed allocation with opaque envelopes based on an a priori computer generated allocation list with an allocation ratio of 1:1 and stratified blocks of 1, 2, or 3. Allocation was conducted by the principal investigator of the trial, who had no contact with the eligible patients and who was not involved in assessment or therapy provision. The experimental group received 16 h of additional sensorimotor therapy and the control group received 16 h of additional motor therapy. Patients were assessed by a blinded assessor at three time points: T1: baseline (pre-intervention) assessment; T2: post-intervention assessment after 4 weeks of additional therapy; and T3: after 4 weeks follow-up.
Participants, Therapists, and Centers
First-ever stroke patients were recruited on admission to the rehabilitation ward from four rehabilitation centers in Belgium: UZ Leuven (Pellenberg); Jessa Hospitals (Herk-de-Stad), RevArte (Antwerp) and Heilig Hart Ziekenhuis (Leuven). Inclusion criteria were: first-ever supratentorial stroke within 8 weeks post stroke, presence of sensorimotor impairment of the UL based on action research arm test (ARAT) score <52 out of 57 and a negative composited standardized somatosensory deficit index [see for explanation of this index (20)], aged 18 years or older and sufficient cooperation. Patients with musculoskeletal or other neurological disorders, severe communication or cognitive deficits or no informed consent were excluded from this trial. The additional intervention was delivered by the same trained study therapist (ND) in all centers, and conventional therapy was provided by the therapists of the rehabilitation centers involved.
Intervention
The experimental sensorimotor therapy consisted of 30 min of sensory re-learning training based on the SENSe approach (20) and 30 min of newly developed sensorimotor training with sensory integrated task-specific motor exercises for the UL, such as sliding over different textures or reaching toward and sorting bottles with a different weight, as described elsewhere (19). Learning principles of motor and somatosensory learning such as attentive exploration, feedback, calibration with the unaffected arm or vision, repetition and progression as well as transfer were implemented in both the SENSe and the sensorimotor training (21–23). The control “motor group” received 30 min of cognitive table-top games with the non-affected UL and 30 min of task-specific motor exercises comparable to the sensorimotor exercises, such as sliding over the table or reaching toward the same bottle, but without any emphasis on sensory components. Both groups received 16 one-hour therapy sessions within 4 weeks as an addition to their conventional inpatient therapy program. This inpatient therapy program consisted of a multidisciplinary approach potentially consisting of physiotherapy, occupational therapy, speech and language therapy, neuropsychology and sports therapy.
Outcome Measures
The ARAT, investigating UL activity, was defined as our primary outcome measure (24). Secondary outcome measures were motor outcome measures including the Fugl-Meyer assessment for the upper extremity (FMA-UE), (25) evaluating motor impairment, the stroke UL capacity scale (SULCS), (26) assessing functional upper limb use, ABILHAND questionnaire (ABIL) evaluating perceived upper limb function (27) and somatosensory outcome measures including Erasmus modified Nottingham sensory assessment (Em-NSA) (28) for evaluating exteroception, proprioception and higher cortical functions, perceptual threshold of touch (PTT) (29), assessing light touch perception, texture discrimination test (TDT) (30) for texture discrimination, wrist position sense test (WPST) (31) for proprioceptive discrimination and functional tactile object recognition test (fTORT) (32) to evaluate stereognosis.
Data Analysis
Patient characteristics were analyzed with descriptive statistics. Normality was checked with the Shapiro-Wilk test (p < 0.05). Since all outcome measures were not normally distributed, variables were analyzed with counts (percentages) for frequency, and median with interquartile range for ordinal and continuous measures. Between-group differences at baseline were investigated using chi-square or Mann-Whitney U tests. Change scores were calculated between all-time points (T2 – T1; T3 – T2; T3 – T1) for experimental and control groups. Effect of treatment group was then investigated with mixed models controlling for age to compare change scores between both groups. Two-tailed p-values, estimated mean differences, and standard error were calculated. Effect sizes corrected for small and different group sizes were calculated with GHedges, and interpreted as small (G = 0.2) medium (G = 0.5) and large (G = 0.8) effects (33). Correction for multiple comparison (Bonferroni) was applied and corrected p-value was set at p < 0.02.
Secondary Analysis
Per protocol, subgroup analysis investigating the effect of therapy group was performed as explained above for patients with mild to moderate, and severe initial motor impairments separately. Subgroups were based on stratification criteria; the ability to perform wrist and finger extension for patients with mild to moderate motor impairments. Similarly, subgroup analysis based on mild to moderate and severe initial somatosensory texture discrimination impairment was performed. Patients with a TDT standardized deficit range score lower than −66.67 were classified as having a severe somatosensory texture discrimination impairment (34). The a priori power analysis is presented in our protocol (19).
Results
Flow of Participants, Therapists, and Centers Trough the Study
A total of 40 stroke patients were recruited with a mean time post stroke of 41 days (SD = 13) between September 2017 and October 2019. Of these patients, 22 were allocated to the sensorimotor group and 18 to the motor group. In each group, one patient dropped out from therapy, the first because of medical reasons unrelated to the trial and the second decided to leave the rehabilitation center. The post-intervention assessment was not performed for two patients because of acute illness in one patient and due to logistic issues in the other patient. The latter did perform the follow-up assessment. Two other individuals, one in each group, were lost to follow-up due to readmission to the acute hospital and because of decline of further participation. No adverse events associated with the interventions were reported. The vast majority of the other patients that were screened were not eligible due to not meeting the criteria “first stroke” or “no other neurological or musculoskeletal disorders present affecting the upper limb.”
The flowchart of the study is presented in Figure 1.
Patient characteristics are listed in Table 1 and a lesion overlay map is available in Figure 2. The latter showing a recognizable distribution with common involvement of the middle cerebral artery region. No significant differences were found between groups at baseline, except for age, and lateralization; participants in the experimental group were significantly older and had more right hemispheric lesions. Other baseline characteristics were similar for both groups such as time post stroke with a median of 38.5 days (IQR = 31–48) for the sensorimotor group and 40 days (IQR = 29–54) for the motor group. Baseline performance on the ARAT was 8 points out of 57 (IQR = 0–41) for the sensorimotor group and 12 points (IQR = 0–35) for the motor group.

Figure 2. Lesion overlay map of stroke lesion location of patients with available magnetic resonance imaging (MRI) scan (n = 30). Color indicates increasing number of patients with inclusion of that voxel into the lesion from blue to red (low number: blue; high number: red).
Between-Group Intervention Effect
Results of between-group comparisons are presented in Table 2 and Figures 3, 4. A trend toward between-group difference in favor of the motor group was found for our primary outcome measure (ARAT) in changes between all-time points. From baseline to post-intervention, a significant greater improvement was found for the motor group in comparison to the sensorimotor group for FMA-UE, and a similar trend was found for SULCS both showing medium to large effect sizes (Ghedges: −1.02 and −0.69, respectively). For motor impairment (FMA-UE), mean pre-to-post improvement in the motor group was 14.65 points, compared to 5.99 points in the sensorimotor group, resulting in a mean difference in improvement (age-adjusted) in favor of the motor group of 8.66 points [standard error (SE) 3.12, t = −2.77, p = 0.01]. From pre-intervention to follow-up, the significant greater improvement for the motor group in comparison to the sensorimotor group for FMA-UE and a trend for SULCS were retained with large effect sizes (Ghedges: −1.16 and −0.98, respectively). For motor impairment (FMA-UE), age-adjusted mean difference in favor of the motor group was 10.63 points (SE = 3.39, t = −3.14, p = 0.003). No significant between-group difference in changes between time points was found for any of our somatosensory measures nor for the ABILHAND questionnaire. Individual delta changes over time and individual time courses of motor recovery can be found in Supplementary Figures 1, 2, respectively.
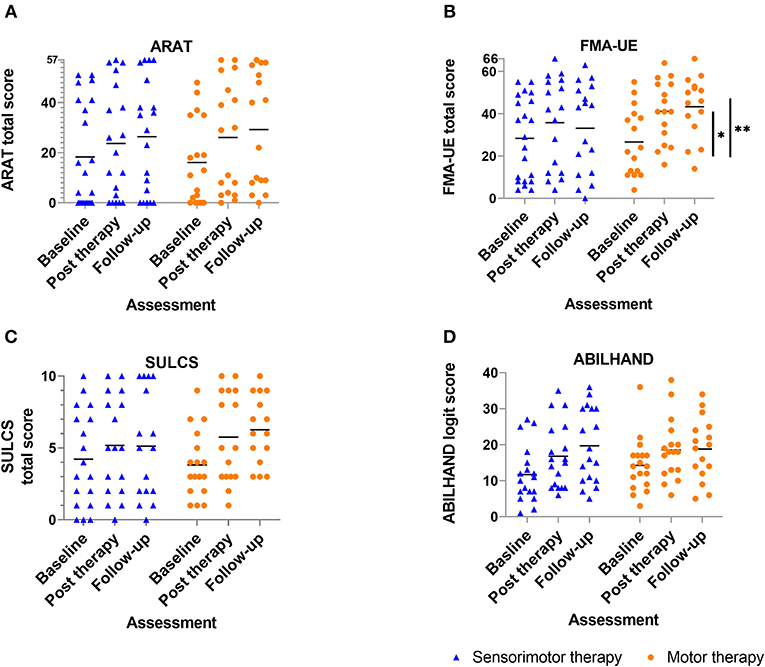
Figure 3. Scatterplot of motor outcome variables for each group at each time point ? every dot (motor therapy) or triangle (sensorimotor therapy) at one time point represents the raw value of a patient; raw median scores indicated with horizontal bar. Vertical bars indicate significant differences in change scores between both groups for *p = 0.01, **p = 0.003; (A) ARAT: Action Research Arm Test, (B) FMA-UE: Fugl-Meyer assessment upper extremity part, (C) SULCS stroke upper limb capacity scale, (D) ABILHAND: ABILHAND questionnaire (logits).
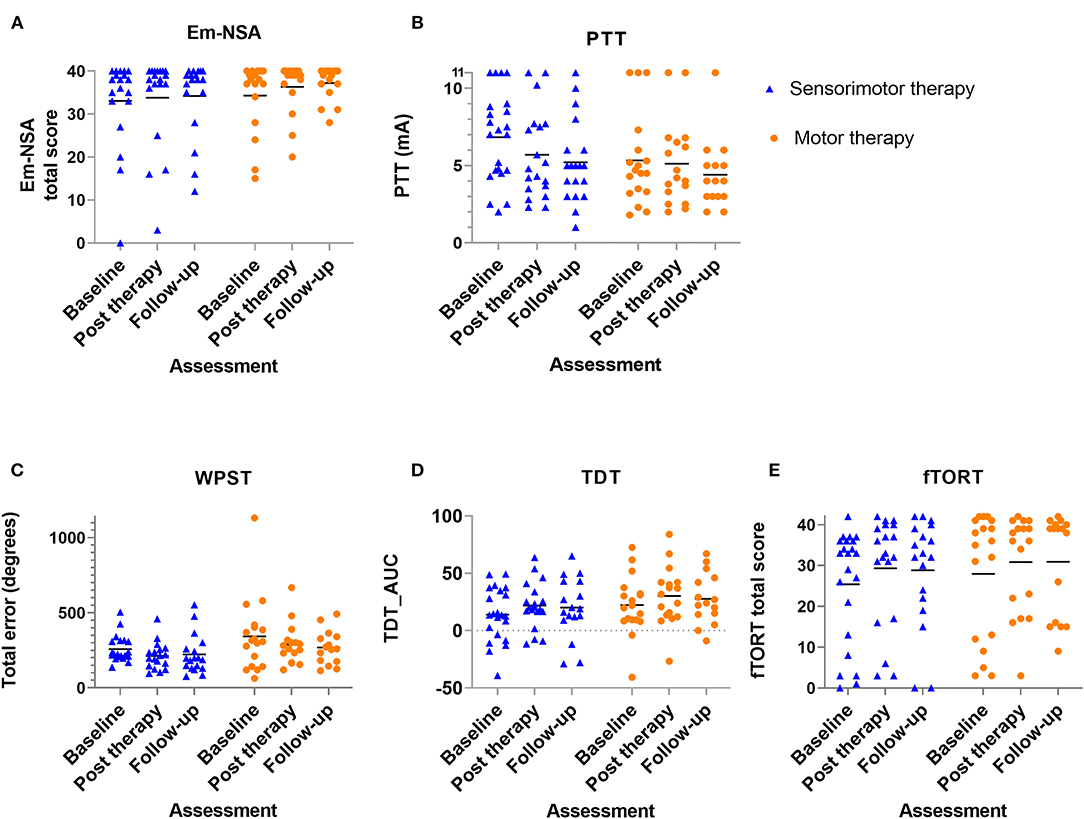
Figure 4. Scatterplot of somatosensory outcome variables for each group at each time point - every dot (motor therapy) or triangle (sensorimotor therapy) at one time point represents the raw value of a patient; raw median scores indicated with horizontal bar. (A) Em-NSA: Erasmus modification of Nottingham Sensory Assessment, (B) PTT: perceptual threshold of touch (mA), (C) WPST: wrist position sense test mean error (degrees), (D) TDT_AUC: texture discrimination test area under curve score, (E) fTORT: functional tactile object recognition test.
Secondary Analysis
Similar results were found for the subgroup of patients with initial severe motor UL impairments (see Supplementary Table 1). A trend of between group differences toward higher change scores was found for the motor group from baseline to post-intervention for FMA-UE, and ARAT; from post-intervention to follow-up for SULCS and ARAT; and from baseline to follow-up for FMA-UE and ARAT. Significant higher change scores were found for the motor group from baseline to follow-up for SULCS. Patients with initial mild to moderate motor UL impairments showed significant higher change scores in the motor group for SULCS from baseline to follow-up. Trends toward significant higher change scores were found for FMA-UE in the motor group and for PTT in the sensorimotor group.
For subgroup analysis based on severity of somatosensory texture discrimination impairments, results for the group with mild to moderate impairments were in line with the results of the main analysis (see Supplementary Table 2). Significant baseline to post-intervention differences (p = 0.02) and large effect sizes (Ghedges: −1.36 and −1.51, respectively) were found between both therapy groups for ARAT and ABILHAND in favor of the motor group. No significant differences were found for the somatosensory measurements. No significant differences were found for the group with severe somatosensory texture discrimination impairments.
Discussion
In this study, we compared the effect of a newly developed UL sensorimotor therapy vs. motor therapy on UL motor and somatosensory function and functional outcome in the early rehabilitation phase post stroke. In contrast to our hypothesis, we could not show a beneficial effect of sensorimotor therapy. Moreover, the results suggest a better improvement in UL motor impairment from baseline to post-intervention and to follow-up assessment for the motor group.
These results are surprising in that we assumed that the integration of a somatosensory component in a motor therapy approach would improve motor recovery due to sensorimotor coupling and the importance of somatosensory function for motor learning (2). When considering integrated sensorimotor training, we have to highlight the two components of our sensorimotor therapy program; consisting of somatosensory training with consecutively the integrated sensorimotor task specific training. Only the second part of this training offered an integrated training approach (14). Reasons for our finding that contrasts our hypothesis could be attributed to trial characteristics as well as our integrated approach. First, the sensorimotor group was older compared to the motor group and it is known that age is a predictor of stroke outcome (35). Thus, we corrected for age in our analysis but still found significant between-group differences. Second, we conducted a dose-matched trial for additional therapy time, which, for both groups, was two times 30 min per day. However, number of repetitions could be different between both groups with a higher number of repetitions for the motor group, which is known to be a beneficial factor for recovery (36, 37). This difference in number of repetitions could be explained by the nature of the exercises. In the sensorimotor group, patients were asked to focus on the somatosensory input during motor execution, which could have reduced the number of movements performed. Additionally, this focus on somatosensory input could change the prioritization of attention toward the somatosensory task inducing mutual interference. This mutual interference is characterized by a deterioration of performance of both tasks (38, 39). Further, within the motor learning literature, dual task training consisting of a motor task with a cognitive task has shown to improve motor performance less effectively than motor task training on his own (40, 41). However, after a motor-cognitive training, performance on this motor-cognitive task is improved but performance of the single motor task is still at the baseline level (42). This mechanism of context and task specific improvements could be an explanation of our findings in favor of the motor group, which were tested into the same context and task as practiced during therapy. So it could be that we were not able to measure the improvements of the sensorimotor therapy group since we were not able to measure the improvement in integration of somatosensory and motor function due to the lack of assessment method available. To further elaborate on the cognitive sensorimotor interference hypothesis, differences between therapy groups could exist of additional somatosensory integration task for the sensorimotor group, which could lead to cognitive sensorimotor interference with prioritization of the sensory input. In healthy adults, these kinds of daily life movements such as reaching toward a cup or sliding over a surface are automated and thus allow the person to divide the attention toward other (sensory) input without any influence on motor performance. However, in stroke patients in the early rehabilitation phase, high attention levels are needed to perform even simple sliding or reaching exercises (38). The addition of somatosensory input could thus induce an allocation of the attention toward the sensory input resulting in impaired performance of the primary (motor) movements. Hence, the integration of a clinical somatosensory component into motor therapy may not be of added value for motor recovery in the early rehabilitation phase. Similar, in the review of Gopaul et al. improvements after sensorimotor therapy were found in a trial with stroke patients in the chronic phase but not in other studies with subacute stroke patients (14, 43, 44). Further research, implementing and evaluating the effect of a revised sensorimotor therapy approach, is needed to provide better insight in effective sensorimotor therapy models, the long-term effects of sensorimotor therapy and the optimal rehabilitation phase.
Another result of our trial is that somatosensory function may not improve differently between groups. Only a trend toward better light touch improvement in the sensorimotor group for patients with initial mild to moderate UL impairments was found. We hypothesized the group receiving sensorimotor therapy to have greater improvements of somatosensory function, compared to the pure motor group, since the former received 30 min of specific somatosensory training based on the SENSe approach (20). In contrast to other somatosensory and sensorimotor therapy stroke trials, we could not find differences in improvements in somatosensory function. Differences in methodology exist between our trial and previous studies and could have influenced the results, such as phase post stroke (subacute in our trial vs. chronic in earlier work), initial UL somatosensory or motor impairment severity and time spent per session (30 min in our trial compared to 60–90 min). However, a similar amount of total training time was provided. The majority of other trials in this domain have focused on patients with chronic stroke (15, 17, 20, 45) recruiting people with persistent somatosensory impairments. Initial rather mild somatosensory impairments of our sample could further explain the lack of between-group differences. Only PTT showed a trend toward between-group differences within the initial mild to moderately impaired patients. Biological recovery could explain the overall lack of difference between groups. Proportional recovery of exteroceptive and proprioceptive somatosensory function is reported to be higher than motor recovery (46). Additionally, most studies focused on patients with initial mild to moderate motor impairments, (16, 17, 45) which allows the patient to divide the attention more toward other (sensory) input, with less influence on motor performance. Last, most studies provided somatosensory stimulation in addition to motor training, without implementing the integration of both, as we provided first somatosensory stimulation and then sensorimotor integration within the same session. Borstad et al. included integration of somatosensory and motor function by performing sorting exercises with different features such as weight or size (45). This study only reported two cases and did not include control participants. Interestingly and similar to our study, they did find improvements in motor, but not somatosensory function for one of both cases. Another study of Byl et al in chronic stroke patients provided learning-based sensorimotor training by providing both active and passive somatosensory discrimination and integrated sensorimotor training such as texture matching tasks, manipulation of objects with varying weights, or shapes and fine functional activities by focusing on the sensory aspect (18). In this trial, training time varied between groups from 12 to 13.3 h and 72 h of therapy. Improvement in both somatosensory and motor function was only found for the high dose therapy group. The other groups improved in either somatosensory or motor function. Furthermore, our findings are in line with the systematic review by Grant and colleagues who concluded that there is low to moderate quality evidence that somatosensory stimulation does not improve motor function, impairment or UL activity (12).
Further, subgroup analysis based on baseline motor and texture discrimination impairments show similar results as the main analysis. Nevertheless, some differences can be noted between subgroups. Patients with severe baseline motor impairments show more beneficial effects on motor improvements, again in favor of the motor therapy group, compared to patients with mild to moderate baseline motor impairments. In contrast, patients with baseline mild to moderate texture discrimination impairments show better improvements in motor function compared to the patients having severe texture discrimination impairments at baseline. Despite that these results have to be interpreted with caution due to the very small sample sizes, they may support the importance of good somatosensory function for better motor recovery. This is supported by findings of Ingemanson et al. (10), who found that somatosensory-related variables such as good neural integrity of somatosensory system explains better treatment response compared to measures of motor behavior.
This trial was preregistered at clinicaltrails.gov (NCT03236376) and the protocol was published (19). Some adaptations occurred after registration and publication. First, predetermined sample size was not reached due to slow recruitment. Therefore, we included two additional rehabilitation centers but we were only able to include a limited number of participants from these centers. Thus, our study should be considered a phase II trial. Second, the nine hole peg test was not included in further analysis since too many patients were not able to perform this test due to severity of upper limb impairment. Third, composited standardized somatosensory deficit index was not used to perform analysis since combining scores of the three subtests could average the severity of a specific category of impairment. Therefore, we decided to use each assessment independently when conducting our analysis. Fourth, subgroup analyzes based on level of cognition is not reported, due to homogeneity of patient performance. Subgroup analysis based on the presence of neglect was also not performed since only three patients had visuospatial neglect based on the star cancelation test. Results of brain imaging analysis as described in the protocol paper will be published in a separate report.
This study has some limitations. First, the sample size is rather small and leads to reduced power of the study, but comparable with phase II studies in the field (47–50). Results should be interpreted with caution due to the large confidence intervals but the higher between-group motor improvement for motor therapy could be considered substantial and clinically relevant. Hence, our trial may inform necessary further studies in this domain. Second, the follow-up was only 4 weeks after additional therapy, which may be too short to find retention effects. Evaluation after 3 or 6 months could reveal interesting insights in the long-term effect of therapy. Third, blinding of the assessor was not always possible due to reactions of the patients. Certainly, patients who received sensorimotor therapy could react to the assessment with a response of recognition. However, the assessor was instructed to not pay attention to these reactions. Fourth, lack of sensitivity and the presence of ceiling effects have to be considered for the motor and somatosensory assessments. Minimal detectable difference are only available for ARAT and FMA and are 4 points for ARAT and 5.2 points for FMA (51, 52). Possible small improvements could not be captured by the current assessments. Despite reliable outcome measures for motor and somatosensory function, one other limitation of the RCT was the inability to measure sensorimotor function as a whole. Since one of the fundamental training principles is that you improve what you train, a specific measurement of integrated sensorimotor function is required. Both motor and somatosensory function can be assessed as separate factors, however the experimental sensorimotor therapy aimed to improve somatosensory integration within motor function. Up till now, no clinical assessment is available to measure this somatosensory integration. Hence, the real effect of sensorimotor therapy could not be assessed. Fifth, concealment was done by the principle investigator, who was not involved in clinical assessment or therapy provision. However, it could be preferred to involve an independent person for this aspect of the methodology. Last, hours of conventional therapy were registered, however the content of this therapy was not. On the other hand, a good balance was obtained in number of patients with mild to moderate or severe impairments receiving both therapy approaches over the different centers. The additional therapy was provided by one therapist in all centers to offer standardization.
To conclude, our results suggest that motor therapy may improve UL motor function to a greater extent compared to sensorimotor therapy in the early rehabilitation phase post stroke for patients with sensorimotor upper limb deficits. Further research is warranted, to investigate whether patients with sensorimotor UL deficits benefit from integrated sensorimotor training and how this training can be delivered effectively.
Data Availability Statement
The raw data supporting the conclusions of this article will be made available by the authors, without undue reservation, to any qualified researcher.
Ethics Statement
The studies involving human participants were reviewed and approved by the ethical committee of UZ/KU Leuven. The patients/participants provided their written informed consent to participate in this study.
Author Contributions
ND: conceptualization, data curation, formal analysis, funding acquisition, investigation, methodology, visualization, resources, writing original draft, and writing editing. LS, LT, AVG, EC, and BE: investigation, resources, methodology, and writing review. CL, MM, HB, and FS: resources, project administration, and writing review. KA: conceptualization, methodology, resources, writing review, supervision, and funding acquisition. GV: conceptualization, methodology, resources, data curation, writing —review, supervision, project administration, and funding acquisition. All authors contributed to the article and approved the submitted version.
Funding
This work was supported by Flanders Research Fund (FWO) (1189819N and 1519719N).
Conflict of Interest
The authors declare that the research was conducted in the absence of any commercial or financial relationships that could be construed as a potential conflict of interest.
Acknowledgments
We would like to acknowledge the contribution of all physiotherapists, occupational therapists and nursing staff of the neurorehabilitation wards of UZ Leuven, campus Pellenberg; Jessa hospitals Herk-de-Stad; Heilig Hart Hospital Leuven; and RevArte Antwerp. More specifically, we would like to acknowledge Anne-Leen Hindrikx, Elke Janssens, Peter Popelier, Margreet Van Dijk, Bea Slaets, Walter Habils, Sabine Vermeiren and Karen Deceuninck who assisted with recruitment and scheduling of therapy and assessment. Clara De Somer is acknowledged for her support within the research project. Finally, we are grateful to all patients who participated in this trial.
Supplementary Material
The Supplementary Material for this article can be found online at: https://www.frontiersin.org/articles/10.3389/fneur.2020.597666/full#supplementary-material
Supplementary Figure 1. Individual delta changes over time; every dot (motor therapy) or triangle (sensorimotor therapy) at one time point represents the delta change score of a patient; raw median scores indicated with horizontal bar. (A) ARAT: Action Research Arm Test, (B) FMA-UE: Fugl-Meyer assessment upper extremity part, (C) SULCS stroke upper limb capacity scale, (D) PTT: perceptual threshold of touch (mA), (E) Em-NSA: Erasmus modification of Nottingham Sensory Assessment, (F) fTORT functional tactile object recognition test, (G) WPST: wrist position sense test mean error (degrees), (H) TDT_AUC: texture discrimination test area under curve score.
Supplementary Figure 2. Individual time courses of motor recovery. Every dot/square (sensorimotor therapy) or triangle (motor therapy) at one time point represents the raw value of a patient. Subdivision is made for patients with mild to moderate and severe baseline motor impairments. ARAT, Action Research Arm Test; FMA-UE, Fugl-Meyer assessment upper extremity part; SULCS, stroke upper limb capacity scale.
Supplementary Table 1. Subgroup analysis of baseline motor impairments of between group comparisons of an intervention effect corrected for age.
Supplementary Table 2. Subgroup analysis of baseline texture discrimination impairments of between group comparisons of an intervention effect corrected for age.
Supplementary Table 3. Number of participants. Overview of number of participants included for each analysis.
References
2. Kessner SS, Bingel U, Thomalla G. Somatosensory deficits after stroke: a scoping review. Top Stroke Rehabil. (2016) 23:136–46. doi: 10.1080/10749357.2015.1116822
3. Meyer S, Karttunen AH, Thijs V, Feys H, Verheyden G. How do somatosensory deficits in the arm and hand relate to upper limb impairment, activity, and participation problems after stroke? Syst Rev Phys Ther. (2014) 94:1220–31. doi: 10.2522/ptj.20130271
4. Meyer S, De Bruyn N, Krumlinde-Sundholm L, Peeters A, Feys H, Thijs V, et al. Associations between sensorimotor impairments in the upper limb at 1 week and 6 months after stroke. J Neurol Phys Ther. (2016) 40:186–95. doi: 10.1097/NPT.0000000000000138
5. Frey SH, Fogassi L, Grafton S, Picard N, Rothwell JC, Schweighofer N, et al. Neurological principles and rehabilitation of action disorders: computation, anatomy, and physiology (CAP) model. Neurorehabil Neural Repair. (2011) 25(5 Suppl):6S−20. doi: 10.1177/1545968311410940
6. Vidoni ED, Acerra NE, Dao E, Meehan SK, Boyd LA. Role of the primary somatosensory cortex in motor learning: an rTMS study. Neurobiol Learn Mem. (2010) 93:532–9. doi: 10.1016/j.nlm.2010.01.011
7. Edwards LL, King EM, Buetefisch CM, Borich MR. Putting the “sensory” into sensorimotor control: the role of sensorimotor integration in goal-directed hand movements after stroke. Front Integr Neurosci. (2019) 13:16. doi: 10.3389/fnint.2019.00016
8. Borich MR, Brodie SM, Gray WA, Ionta S, A L. Boyd: understanding the role of the primary somatosensory cortex: opportunities for rehabilitation. Neuropsychologia. (2015) 79(Pt B):246–55. doi: 10.1016/j.neuropsychologia.2015.07.007
9. Coupar F, Pollock A, Rowe P, Weir C, Langhorne P. Predictors of upper limb recovery after stroke: a systematic review and meta-analysis. Clin Rehabil. (2012) 26:291–313. doi: 10.1177/0269215511420305
10. Ingemanson ML, Rowe JR, Chan V, Wolbrecht ET, Reinkensmeyer DJ, Cramer SC. Somatosensory system integrity explains differences in treatment response after stroke. Neurology. (2019) 92:e1098–108. doi: 10.1212/wnl.0000000000007041
11. Serrada I, Hordacre B, Hillier SL. Does sensory retraining improve sensation and sensorimotor function following stroke: a systematic review and meta-analysis. Front Neurosci. (2019) 13:402. doi: 10.3389/fnins.2019.00402
12. Grant VM, Gibson A, Shields N. Somatosensory stimulation to improve hand and upper limb function after stroke-a systematic review with meta-analyses. Top Stroke Rehabil. (2018) 25:150–60. doi: 10.1080/10749357.2017.1389054
13. Yilmazer C, Boccuni L, Thijs L, Verheyden G. Effectiveness of somatosensory interventions on somatosensory, motor and functional outcomes in the upper limb post-stroke: a systematic review and meta-analysis. NeuroRehabilitation. (2019) 44:459–77. doi: 10.3233/nre-192687
14. Gopaul U, Carey L, Callister R, Nilsson M P. van Vliet, Combined somatosensory and motor training to improve upper limb function following stroke: a systematic scoping review. Phys Ther Rev. (2018) 23:355–75. doi: 10.1080/10833196.2018.1553668
15. de Diego C, Puig S, Navarro X. A sensorimotor stimulation program for rehabilitation of chronic stroke patients. Restor Neurol Neurosci. (2013) 31:361–71. doi: 10.3233/RNN-120250
16. Macháčková K, Vyskotová J, Opavský J Recovery of somatosensory and motor functions of the paretic upper limb in patients after stroke: comparison of two therapeutic approaches. Acta Gymnica. (2016) 46:37–43. doi: 10.5507/ag.2015.026
17. Lin K-C, Huang P-C, Chen Y-T, Wu C-Y, Huang W-L. Combining afferent stimulation and mirror therapy for rehabilitating motor function, motor control, ambulation, and daily functions after stroke. Neurorehabil Neural Repair. (2014) 28:153–62. doi: 10.1177/1545968313508468
18. Byl NN, Pitsch EA, Abrams GM. Functional outcomes can vary by dose: learning-based sensorimotor training for patients stable poststroke. Neurorehabil Neural Repair. (2008) 22:494–504. doi: 10.1177/1545968308317431
19. De Bruyn N, Essers B, Thijs L, Van Gils A, Tedesco Triccas L, Meyer S, et al. Does sensorimotor upper limb therapy post stroke alter behavior and brain connectivity differently compared to motor therapy? Protocol of a phase II randomized controlled trial. Trials. (2018) 19:242. doi: 10.1186/s13063-018-2609-4
20. Carey L, Macdonell R, Matyas TA. SENSe: study of the effectiveness of neurorehabilitation on sensation: a randomized controlled trial. Neurorehabil Neural Repair. (2011) 25:304–13. doi: 10.1177/1545968310397705
21. Carey LM, Polatajko HJ, Connor LT, Baum CM. Stroke rehabilitation: a learning perspective. In: Carey L, editor. Stroke Rehabilitation. New York, NY: Oxford University Press (2012). p. 11–23. doi: 10.1093/med/9780199797882.003.0002
22. Kleim JA, Jones TA. Principles of experience-dependent neural plasticity: implications for rehabilitation after brain damage. J Speech Lang Hear Res. (2008) 51:S225–39. doi: 10.1044/1092-4388(2008/018)
23. Pekna M, Pekny M, Nilsson M. Modulation of neural plasticity as a basis for stroke rehabilitation. Stroke. (2012) 43:2819–28. doi: 10.1161/strokeaha.112.654228
24. Lin JH, Hsu MJ, Sheu CF, Wu TS, Lin RT, Chen CH, et al. Psychometric comparisons of 4 measures for assessing upper-extremity function in people with stroke. Phys Ther. (2009) 89:840–50. doi: 10.2522/ptj.20080285
25. Fugl-Meyer AR, Jaasko L, Leyman I, Olsson S, Steglind S. The post-stroke hemiplegic patient. 1. a method for evaluation of physical performance. Scand J Rehabil Med. (1975) 7:13–31.
26. Roorda LD, Houwink A, Smits W, Molenaar IW, Geurts AC. Measuring upper limb capacity in poststroke patients: development, fit of the monotone homogeneity model, unidimensionality, fit of the double monotonicity model, differential item functioning, internal consistency, and feasibility of the stroke upper limb capacity scale SULCS. Arch Phys Med Rehabil. (2011) 92:214–27. doi: 10.1016/j.apmr.2010.10.034
27. Penta M, Tesio L, Arnould C, Zancan A, Thonnard JL. The ABILHAND questionnaire as a measure of manual ability in chronic stroke patients: Rasch-based validation and relationship to upper limb impairment. Stroke. (2001) 32:1627–34. doi: 10.1161/01.str.32.7.1627
28. Stolk-Hornsveld F, Crow JL, Hendriks EP, van der Baan R, Harmeling-van der Wel BC. The Erasmus MC modifications to the (revised) Nottingham Sensory Assessment: a reliable somatosensory assessment measure for patients with intracranial disorders. Clin Rehabil. (2006) 20:160–72. doi: 10.1191/0269215506cr932oa
29. Eek E, Engardt M. Assessment of the perceptual threshold of touch (PTT) with high-frequency transcutaneous electric nerve stimulation (Hf/TENS) in elderly patients with stroke: a reliability study. Clin Rehabil. (2003) 17:825–34. doi: 10.1177/026921550301700803
30. Carey LM, Oke LE, Matyas TA. Impaired touch discrimination after stroke: a quantiative test. Neurorehabil Neural Repair. (1997) 11:219–32. doi: 10.1177/15459683970110x0404
31. Carey LM, Oke LE, Matyas TA. Impaired limb position sense after stroke: a quantitative test for clinical use. Arch Phys Med Rehabil. (1996) 77:1271–8. doi: 10.1016/s0003-9993(96)90192-6
32. NJ. Carey LM, LeBlanc S, Harvey L. A new functional tactual object recognition test (fTORT) for stroke clients: normative standards and discriminative validity. Paper presented at: 14th International Congress of the World Federation of Occupational Therapists. Sydney, NSW (2006).
33. Cohen J. Statistical Power Analysis for the Behavioral Sciences. 2nd edn. Hillsdale, NJ: Erbaum Press (1988).
34. Carey LM, Matyas TA. Frequency of discriminative sensory loss in the hand after stroke in a rehabilitation setting. J Rehabil Med. (2011) 43:257–63. doi: 10.2340/16501977-0662
35. Kwakkel G, Kollen BJ. Predicting activities after stroke: what is clinically relevant? Int J Stroke. (2013) 8:25–32. doi: 10.1111/j.1747-4949.2012.00967.x
36. Kleim JA, Barbay S, Nudo RJ. Functional reorganization of the rat motor cortex following motor skill learning. J Neurophysiol. (1998) 80:3321–5. doi: 10.1152/jn.1998.80.6.3321
37. Nudo RJ, Milliken GW, Jenkins WM, Merzenich MM. Use-dependent alterations of movement representations in primary motor cortex of adult squirrel monkeys. J Neurosci. (1996) 16:785–807. doi: 10.1523/jneurosci.16-02-00785.1996
38. Plummer P, Eskes G. Measuring treatment effects on dual-task performance: a framework for research and clinical practice. Front Hum Neurosci. (2015) 9:225. doi: 10.3389/fnhum.2015.00225
39. Bank PJM, van Marinus J, Tol RM, Groeneveld IF, Goossens PH, de Groot JH, et al. Cognitive-motor interference during goal-directed upper-limb movements. Eur J Neurosci. (2018) 48:3146–58. doi: 10.1111/ejn.14168
40. McDougle SD, Ivry RB, Taylor JA. Taking aim at the cognitive side of learning in sensorimotor adaptation tasks. Trends Cogn Sci (Regul Ed). (2016) 20:535–544. doi: 10.1016/j.tics.2016.05.002
41. Song J-H. The role of attention in motor control and learning. Curr Opin Psychol. (2019) 29:261–5. doi: 10.1016/j.copsyc.2019.08.002
42. Song J-H, Bédard P. Paradoxical benefits of dual-task contexts for visuomotor memory. Psychol Sci. (2015) 26:148–58. doi: 10.1177/0956797614557868
43. Winter JM, Crome P, Sim J, Hunter SM. Effects of mobilization and tactile stimulation on chronic upper-limb sensorimotor dysfunction after stroke. Arch Phys Med Rehabil. (2013) 94:693–702. doi: 10.1016/j.apmr.2012.11.028
44. Hunter SM, Crome P, Sim J, Pomeroy VM. Effects of mobilization and tactile stimulation on recovery of the hemiplegic upper limb: a series of replicated single-system studies. Arch Phys Med Rehabil. (2008) 89:2003–10. doi: 10.1016/j.apmr.2008.03.016
45. Borstad AL, Bird T, Choi S, Goodman L, Schmalbrock P, Nichols-Larsen DS. Sensorimotor training and neural reorganization after stroke: a case series. J Neurol Phys Ther. (2013) 37:27–36. doi: 10.1097/NPT.0b013e318283de0d
46. Boccuni L, Meyer S, Kessner SS, De Bruyn N, Essers B, Cheng B, et al. Is there full or proportional somatosensory recovery in the upper limb after stroke? Investigating behavioral outcome and neural correlates. Neurorehabil Neural Repair. (2018) 32:691–700. doi: 10.1177/1545968318787060
47. Lawrie S, Dong Y, Steins D, Xia Z, Esser P, Sun S, et al. Evaluation of a smartwatch-based intervention providing feedback of daily activity within a research-naive stroke ward: a pilot randomised controlled trial. Pilot Feasibility Stud. (2018) 4:157. doi: 10.1186/s40814-018-0345-x
48. Liu YC, Yang YR, Tsai YA, Wang RY. Cognitive and motor dual task gait training improve dual task gait performance after stroke - a randomized controlled pilot trial. Sci Rep. (2017) 7:4070. doi: 10.1038/s41598-017-04165-y
49. Rose DK, DeMark L, Fox EJ, Clark DJ, Wludyka P. A backward walking training program to improve balance and mobility in acute stroke, a pilot randomized controlled trial. J Neurol Phys Ther. (2018) 42:12–21. doi: 10.1097/npt.0000000000000210
50. Zhu Z, Cui L, Yin M, Yu Y, Zhou X, Wang H, et al. Hydrotherapy vs. conventional land-based exercise for improving walking and balance after stroke: a randomized controlled trial. Clin Rehabil. (2016) 30:587–93. doi: 10.1177/0269215515593392
51. Page SJ, Fulk GD, Boyne P. Clinically important differences for the upper-extremity Fugl-Meyer Scale in people with minimal to moderate impairment due to chronic stroke. Phys Ther. (2012) 92:791–8. doi: 10.2522/ptj.20110009
Keywords: stroke, upper extremity, treatment outcome, sensorimotor therapy, randomized controlled (clinical) trial
Citation: De Bruyn N, Saenen L, Thijs L, Van Gils A, Ceulemans E, Essers B, Lafosse C, Michielsen M, Beyens H, Schillebeeckx F, Alaerts K and Verheyden G (2020) Sensorimotor vs. Motor Upper Limb Therapy for Patients With Motor and Somatosensory Deficits: A Randomized Controlled Trial in the Early Rehabilitation Phase After Stroke. Front. Neurol. 11:597666. doi: 10.3389/fneur.2020.597666
Received: 21 August 2020; Accepted: 06 November 2020;
Published: 04 December 2020.
Edited by:
Margit Alt Murphy, University of Gothenburg, SwedenReviewed by:
Håkan Carlsson, Lund University, SwedenUrvashy Gopaul, University of Mauritius, Mauritius
Copyright © 2020 De Bruyn, Saenen, Thijs, Van Gils, Ceulemans, Essers, Lafosse, Michielsen, Beyens, Schillebeeckx, Alaerts and Verheyden. This is an open-access article distributed under the terms of the Creative Commons Attribution License (CC BY). The use, distribution or reproduction in other forums is permitted, provided the original author(s) and the copyright owner(s) are credited and that the original publication in this journal is cited, in accordance with accepted academic practice. No use, distribution or reproduction is permitted which does not comply with these terms.
*Correspondence: Nele De Bruyn, nele.debruyn@kuleuven.be