- 1Swiss Children's Rehab, University Children's Hospital Zurich, Affoltern am Albis, Switzerland
- 2Children's Research Center, University Children's Hospital Zurich, Zurich, Switzerland
- 3Institute for Biomechanics, ETH Zurich, Zurich, Switzerland
- 4Doctoral Program Clinical Science, Faculty of Medicine, University of Zurich, Zurich, Switzerland
Background: Impaired selective voluntary motor control is defined as “the reduced ability to isolate the activation of muscles in response to demands of a voluntary posture or movement.” It is a negative motor sign of an upper motor neuron lesion.
Objective: This paper reviews interventions that may improve selective motor control in children and youths with spastic cerebral palsy. The aim was to systematically evaluate the methodological quality and formulate the level of evidence from controlled studies.
Methods: Six databases (Scopus, Web of Science, PubMed, Embase, MEDLINE, and CINAHL) were searched with predefined search terms for population, interventions, and outcomes. Two reviewers independently completed study selection and ratings of methodological quality and risk of bias. Evidence was summarized in a best evidence synthesis.
Results: Twenty-three studies from initially 2,634 papers were included. The interventions showed a wide variety of approaches, such as constraint-induced movement therapy (CIMT), electrical stimulation, robot-assisted therapy, and functional training. The evidence synthesis revealed conflicting evidence for CIMT, robot-assisted rehabilitation and mirror therapy for the upper extremities in children with cerebral palsy.
Conclusions: Final recommendations are difficult due to heterogeneity of the reviewed studies. Studies that include both an intervention and an outcome that specifically focus on selective voluntary motor control are needed to determine the most effective therapy.
Introduction
A wide variety of acquired or congenital brain injuries can cause lesions of the upper motor neuron, which results in conditions like stroke, traumatic brain injury, or spastic cerebral palsy (CP). Patients with upper motor neuron lesions typically show impairments like decreased muscle force, increased muscle tone/spasticity, and loss of motor control (1, 2). Loss of selective voluntary motor control (SVMC) is defined as “the impaired ability to isolate the activation of muscles in a selected pattern in response to demands of a voluntary posture or movement” (3). This means that patients with impaired SVMC do not show the activation pattern expected in healthy subjects, either due to excessive or lack of muscle activity. SVMC refers to the ability to perform isolated joint movements deliberately and has to be distinguished from habitual selective joint motions during functional tasks such as walking (4). Clinically, reduced SVMC could manifest in mirror movements, which are simultaneous contralateral, involuntary, identical movements that accompany voluntary movements (5) or synergistic muscle activation and movement patterns (obligatory grouped multi-joint movements, e.g., co-activation of M. gastrocnemius and M. quadriceps during knee extension while sitting) (6).
These signs of reduced SVMC can have different neurophysiological origins. Two mechanisms are proposed to contribute to the occurrence of mirror movements (7). First, ipsilateral corticospinal tract projections from the non-lesioned motor cortex to the affected side, and second, insufficient interhemispheric inhibition resulting in bilateral cortical activation. Synergistic and antagonistic co-activations of muscles are addressed to a compensatory reliance on the extrapyramidal rubrospinal tract, which is relatively prominent in infants, in case of corticospinal tract injury (2).
Using the nomenclature of the International Classification of Functioning, Disability and Health (8), SVMC should be considered a body function. Research has shown, though, that impairments in SVMC can contribute to limitations in activities of daily life that some patients experience. For example, children with unilateral spastic CP who show mirror movements require more time for bimanual activities of daily life (9). The abnormal co-activation of shoulder abductor and elbow flexor muscles (“flexion synergy”) reduces the reaching work space in stroke patients (10). A loss of SVMC in the lower extremity has a negative impact on walking ability. For children with spastic CP, impaired SVMC relates to a certain degree to gait abnormalities (11), gait velocity (12), and impaired gross motor function (13).
Despite many cross-sectional studies exploring limitations in SVMC in children with spastic CP, only few interventions actually intend to improve SVMC. These interventions are heterogeneous in terms of the strategies they adapt to enhance SVMC (14–18) and included strength training, performing independent hand movements with suppression or control of mirror movements, and video game-based training of joint movement control. One of the reasons, why not many studies have targeted improving SVMC, could be because many assessments specifically quantifying impairments in SVMC have only recently been established (2).
For children with CP, systematic summaries of the evidence exist about interventions that target improvements in upper limb function, gross motor function, physical activity, or gait speed (19–22). However, a systematic summary of interventions that can lead to improvements in SVMC, which could help to decide which strategy is most promising to train SVMC, is lacking. Other interventional studies might not have primarily focused on improving SVMC, but as they included assessments that quantify SVMC, they could contribute to our understanding what interventions seem beneficial to ameliorate SVMC. Therefore, the aim of this review is to evaluate the quality of the studies and the efficacy of interventions that may improve SVMC. Therefore, the research question is: What is the evidence from controlled interventional trials that may improve SVMC of the upper or lower extremities in children and adolescents with spastic CP?
Methods
Search Strategy
We conducted a search in the databases Scopus, Web of Science, PubMed, Embase, MEDLINE (accessed via Ovid), and CINAHL, from their inception to present. The initial search was run on December 4, 2018 and updated on November 26, 2019. The search strategy was developed according to the PICOS approach (23). It combined terms for the population of patients with upper motor neuron lesions with terms describing interventions, and keywords and synonyms for SVMC and outcome measures thereof. If applicable, the search strategy also included suitable terms from the databases' controlled vocabulary. The full search strategy for PubMed can be found in the Supplementary Material. This search strategy was adapted for use with other bibliographic databases. We identified additional records by screening the references of relevant reviews retrieved in the search.
Study Selection
The results retrieved with the search strategy were imported to a reference manager (Mendeley 1.17.12, Mendeley Ltd., London, United Kingdom) where duplicates were removed. Abstract screening and full-text review was conducted with a specialized software (Covidence systematic review software, Veritas Health Innovation, Melbourne, Australia). Two reviewers (AF and JK) independently screened the titles and abstracts to identify studies that were potentially relevant. Cases of disagreement were discussed until consensus was reached. The same two researches then independently reviewed the full-text articles for eligibility. We discussed any disagreements with a third reviewer (HvH).
Studies were included if they met the following criteria: (a) study participants with spastic CP; (b) participants younger than 18 years; (c) include a rehabilitative intervention and the content of the therapy is described; (d) have at least one outcome measure or subscale of an outcome measure (i.e., part of an assessments with a separate score) that assessed SVMC; (e) were peer-reviewed original research articles; and (f) written in English or German. Studies were excluded if (a) the participants had a lesion of the lower motor neuron or degenerative disease; (b) <75% of the study population fulfilled the aforementioned criteria; (c) the intervention was invasive (botulinum toxin therapy, surgery) or concerned drugs; (d) they lacked a control group; and (e) they were only cross-sectional studies.
Since the type of CP was not always reported, we made the following assumptions: (i) unilateral/hemiplegia or di-/quadriplegia refers to the spastic type of CP (24), (ii) reporting other indicators that reflect a spastic component (e.g., including an outcome measure for spasticity). We did not restrict this review on a particular treatment modality, but focused specifically on outcomes for SVMC. Besides established assessments of SVMC, we also included other measures if they covered aspects of SVMC in accordance with the aforementioned definition. We understand measures of SVMC as instruments or subscales thereof that assess selectivity of individual joint movements or voluntary multi-joint movement outside of synergies or mass patterns but not functional gross motor tasks like walking (25). The assessment scoring criteria should consider signs of reduced SVMC like mirror movements and/or compensatory or synergistic movement patterns.
The study protocol was published on Prospero (CRD42019117407). We deviated from the protocol regarding the patient population and the study designs included. First, we initially intended to include studies which had included patients with acquired and congenital upper motor neuron lesions, as this represents the heterogeneous population of children and adolescents with reduced SVMC treated daily in rehabilitation clinics. Later, we limited the search to children with spastic CP, because this allowed more specific conclusions. Second, we initially searched for all study designs, in spite of finally including only controlled studies. Thereby, we aimed to identify whether there are SVMC specific interventions that have not been studied in an RCT yet, since we already expected to find few SVMC specific interventions.
Data Collection and Quality Assessment
One reviewer (AF) extracted the data into a customized spread sheet, the other reviewer (JK) critically reviewed it. Extracted information included (a) parameters describing the study population (number of participants, age, diagnosis, disease severity); (b) a description of the intervention and control condition (tasks, setting, duration); (c) the outcome measures; and (d) the results.
The studies were assigned a level of evidence based on the study design, as recommended by the guidelines of the American Academy for Cerebral Palsy and Developmental Medicine (AACPDM) (26). We evaluated the methodological quality of studies according to several aspects described in these guidelines. Studies only reaching a weak quality rating (“yes” score on <4 of 7 questions) were excluded from further analyses. A description of the levels of evidence and the methodological conduct questions can be found in the Supplementary Tables 1, 2. Additionally, we evaluated the risk of bias in the seven domains of the risk of bias assessment described by the Cochrane Collaboration (27). Two reviewers (AF and JK) independently conducted the ratings (AACPDM and Cochrane tool) and resolved any discrepancies through discussion.
Data Analysis
Studies of moderate or strong methodological quality were included in a best evidence synthesis according to van Tulder et al. (28) if they reported results of between-group comparisons. We scored interventions as “improved SVMC” when between-group results were statistically significant and as having “no effect” when results were not statistically significant. When possible, we calculated Hedges' g (29), an unbiased estimate of the standardized mean difference in change, for a rough comparison of significance and effect size for each study.
In accordance with van Tulder et al. (28), the overall level of evidence was considered “strong” if there were consistent findings among multiple high quality (AACPDM quality rating: strong) RCTs, “moderate” for consistent findings among multiple low quality (quality rating: moderate) RCTs and/or one high quality RCT, “limited” if there was one low quality RCT and “conflicting” in case of inconsistent findings. Consistency was defined as ≥75% of studies showing the same effect.
Mixed populations were allocated to specific groups if ≥75% of participants had the same diagnosis, i.e., a study population encompassing eight children with unilateral and one with bilateral spastic CP was labeled as unilateral spastic CP. The studies were further categorized into groups of similar interventions. The categorization into groups of similar types of interventions was based on the intervention that differentiated between the intervention and control group. The overall evidence was determined for specific groups, i.e., based on type of intervention and whether it involved the upper or lower extremity.
Results
The search retrieved 4,407 hits. We found another 426 articles when updating the search and 470 additional records were identified from screening the references of 11 relevant reviews contained in the search results. In total, 2,634 abstracts were screened for eligibility after removal of duplicates. After reviewing 292 full-texts, 29 studies were retained. Further, four studies were excluded because of insufficient quality (17, 30–32) and two because between-group comparisons were missing (33, 34). Figure 1 outlines the selection process and shows reasons for exclusion at full-text review.
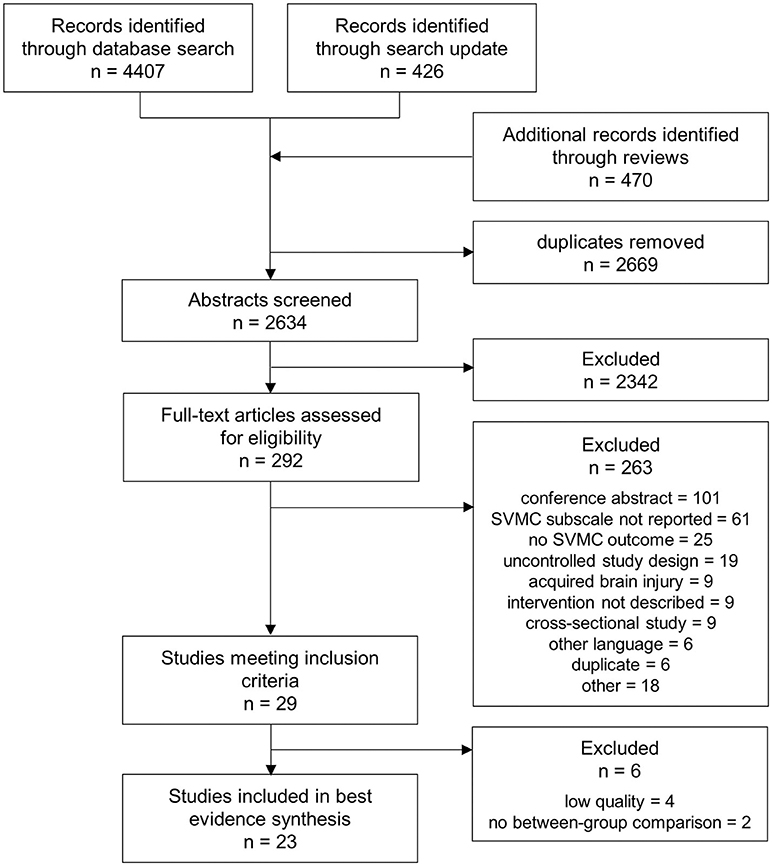
Figure 1. PRISMA (Preferred Reporting Items for Systematic Reviews and Meta-Analyses) flow chart showing the process of study selection.
An overview of all studies is provided in Table 1. One study that matched the inclusion criteria used a single subject research design, namely a randomized controlled N-of-1 trial (AACPDM evidence level I). Among the group design studies, there were 21 RCTs (level II) and one randomized cross-over trial (level II).
Characteristics of study participants were heterogeneous in terms of age and severity of disability. The majority of studies included uniquely children with unilateral spastic CP (n = 17). Further, there we four studies investigating bilateral spastic CP and mixed groups in two studies.
SVMC was quantified with a wide variety of assessments listed in Table 2. Most studies had a single outcome parameter that measured SVMC; three measured two SVMC outcomes (35–37). The dissociated movement subscale of quality of upper extremity skills test was the most common assessment (used in 13 of 23 studies) followed by the selective control assessment of the lower extremity (used four times). Despite that the quality of upper extremity skills test was not specifically designed to measure SVMC, we considered this subscale appropriate. The dissociated movement section assesses the ability to perform single-joint movements over the whole range of motion while maintaining other joints in a defined position, i.e., abducting the shoulder with the elbow extended.
We categorized the interventions into three broad groups: constraint-based therapies, technological interventions, and other approaches. With constraint-based therapies, we mean any use of restraint to limit compensatory strategies, e.g., use of less affected limb. Under rehabilitation technologies, sometimes equaled with robots, we include all electrically powered systems, devices or tools used to meet the needs of rehabilitation. One RCT (38) used a three group design comparing two different interventions to a control group and is thus listed in two categories.
Nine studies (35, 38–45) investigated (modified) constraint-induced movement therapy (CIMT). Constraint was thereby either continuous with a cast or periodically applied during therapy sessions with a splint (or similar). One study used a constraint-based approach other than CIMT. They compared task-oriented upper limb training with and without trunk restraint (46).
Varying rehabilitation technologies were investigated. Three studies evaluated robot-assisted movement therapy of the upper (n = 2) or lower extremities (n = 1). Assistance was either weight support (47) or physical support of the desired movements (48, 49). Further technological interventions encompassed: (i) electrical stimulation applied peripherally to muscles of the lower extremities while walking (36), (ii) an exergame (50), i.e., game controlled by body movements without any physical support, and therefore, it does not fall into the first group of assisted therapies, (iii) a study that compared indoor cycling to exercising on an elliptical trainer (51), and (iv) one investigating acoustic stimulation (52).
Among the remaining studies, we identified four other approaches. The first two encompass studies on the efficacy of mirror therapy (53–55) and action observation therapy (56). Thirdly, one study investigated lower extremity strength training (57). The fourth category comprises functional training programs (trunk and bimanual training) (37, 38).
Ratings of conduct quality (AACPDM) and risk of bias (Cochrane) of included studies can be found in Tables 3, 4. Methodological conduct quality ranged from strong (7/7 points) to moderate (4/7 points). Studies most often did not report adherence to the intervention and power calculations. Ratings of studies excluded due to insufficient quality (<4 points) can be found in the Supplementary Table 3. Concerning the Cochrane risk of bias assessment, studies received a low risk rating on 2/7 to 6/7 domains of possible sources of risk of bias. Most common sources for high or unclear risk of bias were no or unclear description of the allocation concealment, a lack of information about missing data, and the unavailability of a study protocol to rule out selective reporting.
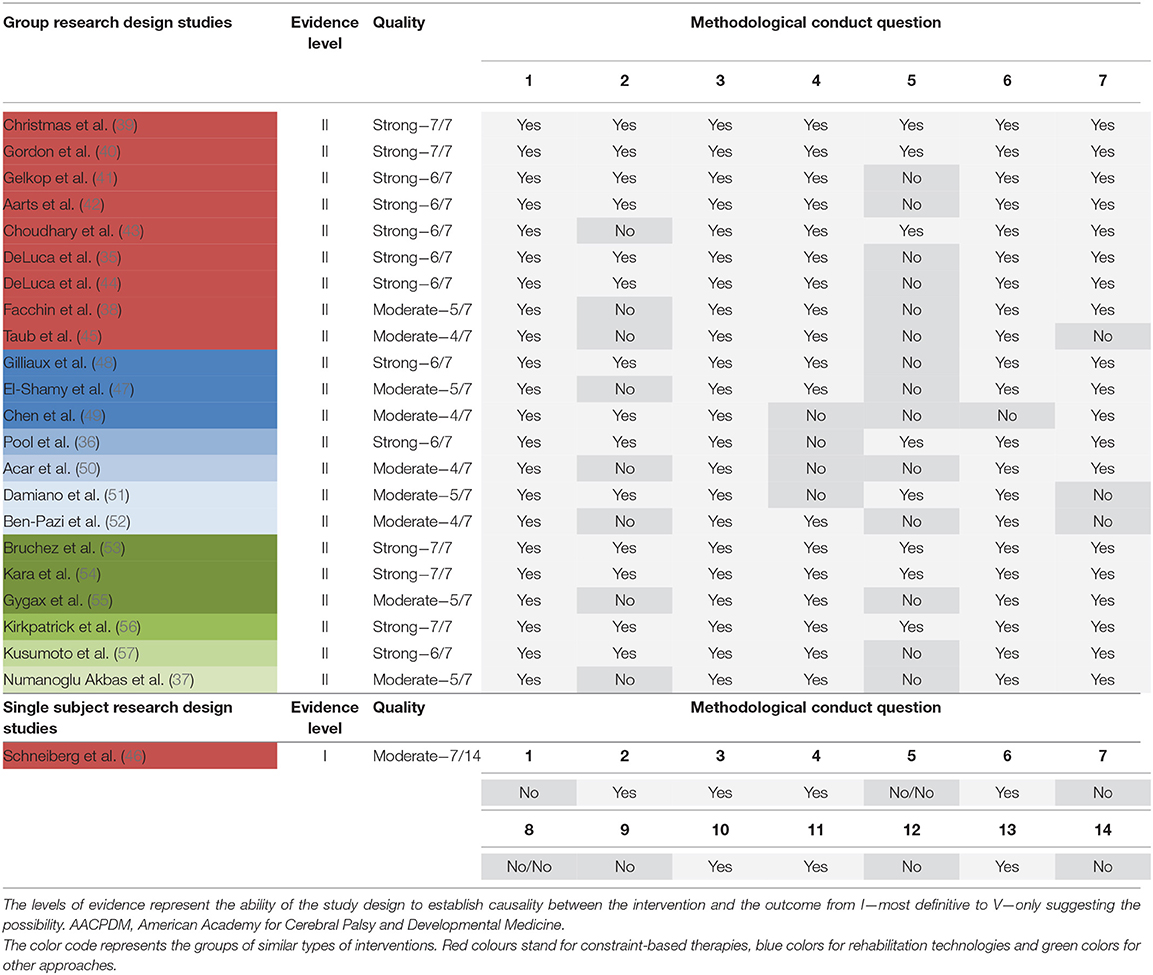
Table 3. Levels of evidence and methodological quality rating of included studies in accordance with AACPDM guidelines.
Best Evidence Synthesis
The overall level of evidence is summarized below and Table 5 shows the best evidence synthesis. When available, the effect sizes confirmed the results based on statistical significance. Hedges' g exceeded 0.79 for significant comparisons and was smaller than 0.50 for all non-significant comparisons.
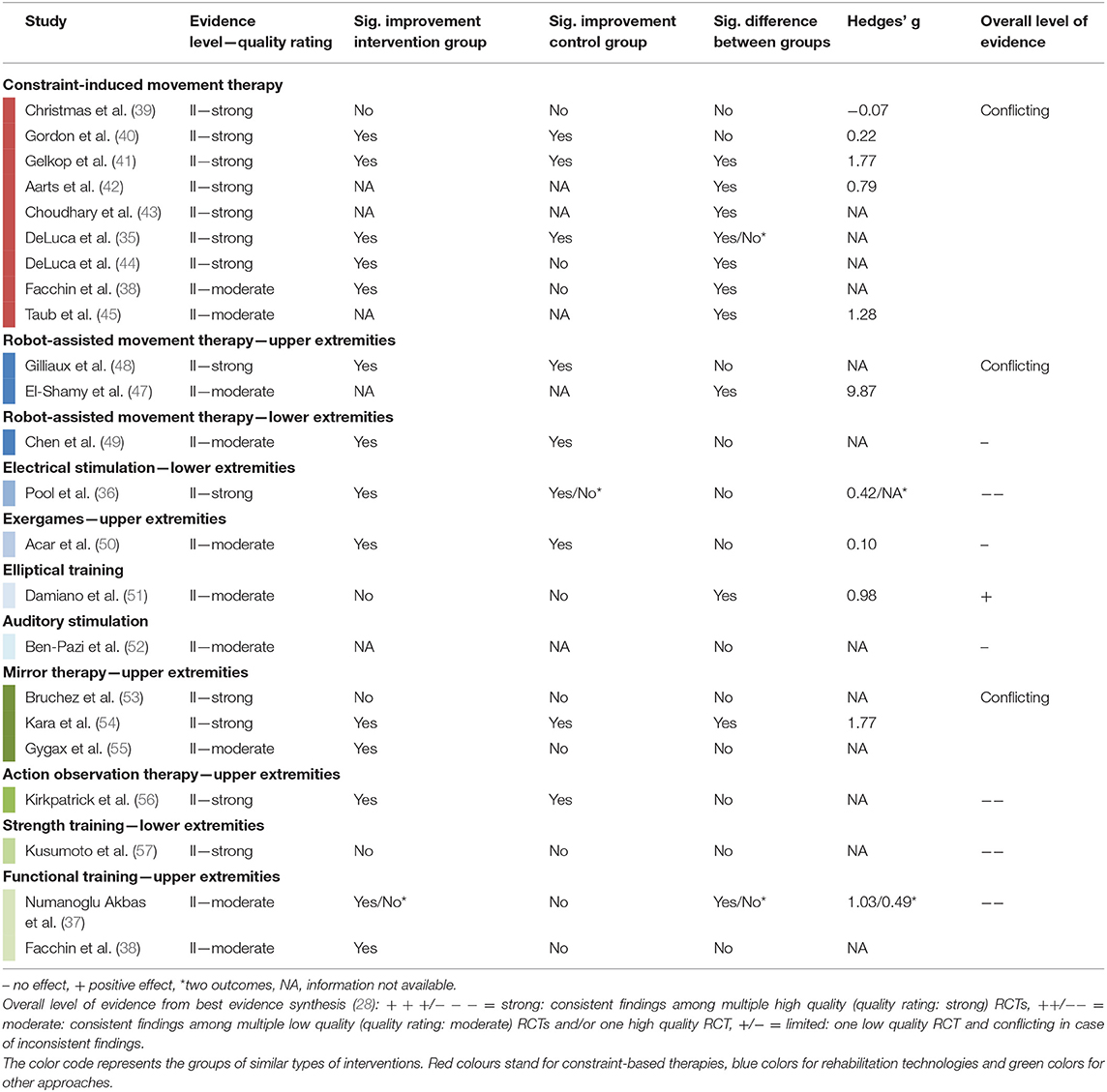
Table 5. Summary of evidence and best evidence synthesis according to van Tulder et al. (28) of controlled group design studies with moderate or strong methodological quality.
Constraint-Based Therapies
There were nine RCTs (35, 38–45) summarized in the evidence synthesis for CIMT in children with unilateral CP. The trials included between 12 and 72 children (total n = 325), aged from 0.6 to 10 years, with MACS levels I to IV. Intensity of therapy varied between 42 and 210 h administered over 3 to 10 weeks. We observed conflicting evidence concerning the efficacy of CIMT on SVMC, mostly assessed with the quality of upper extremity skills test. Some studies showed a significantly improved SVMC outcome after CIMT compared to the control condition, while other studies did not find differences between the interventions.
Regarding other constraint-based approaches, a randomized N-of-1 trial found that more children reduced their trunk compensation during reach-to-grasp movements after task-oriented training with trunk restraint compared to children training without trunk constraint (46).
Rehabilitation Technology
Two RCTs (total n = 46 children) compared robot-assisted upper limb training to conventional therapy, one in children with unilateral CP (47), the other in children with bilateral CP (48). Due to inconsistent findings, the overall evidence for robot-assisted upper limb training is conflicting. For the lower extremities, only one RCT was eligible for the best evidence synthesis (49). In 41 children with varying types of CP, the efficacy of a robotic ankle training program was compared between different settings. Both the home-based and the lab-based group significantly improved movement selectivity but no difference between settings could be shown.
From the other interventions, a study in 32 children with unilateral CP indicated that using a device for electrical stimulation of ankle dorsiflexion during the swing phase of gait was not more efficacious than conventional care (36). Concerning exergames, the single RCT found that playing games with the Nintendo Wii console had no additional effect on SVMC compared to neurodevelopmental treatment alone in 30 children with unilateral CP (50). Acoustic stimulation did also not change upper limb selective control (52). The change of lower limb SVMC in 27 children with bilateral CP after training on an elliptical compared to indoor cycling was significantly different, favoring the elliptical trainer (51).
Other Approaches
Two RCTs (53, 54) and one cross-over trial (55) investigated the efficacy of mirror therapy in totally 130 children with unilateral spastic CP (MACS I–IV). The evidence is conflicting whether offering a visual impression of a functional limb (by illusion through the mirror) has a superior effect on SVMC compared to repetitively practicing the same movement without vision of correct movement execution. The efficacy of action observation therapy, hence preceding movement practice by watching somebody performing that movement, was not different from movement practice alone (56).
The strength training category included only one RCT eligible for the evidence synthesis. Kusumoto et al. (57) compared loaded sit-to-stand exercises performed at slow vs. self-paced speed in 16 children with bilateral spastic CP (GMFCS I–III). From the results, no speed of movement execution could be favored in terms of its effect on SVMC.
Functional training programs were studied in two RCTs but with a different focus. The first study compared the efficacy of trunk training to regular physiotherapy alone in 36 children with bilateral spastic CP (37). Trunk training led to significant improvements in selective trunk control but not the scores of the quality of upper extremity skills test. The second study emphasized the use of both hands in 66 young children with unilateral CP (38). This intensive bimanual training (210 h) did not differ from a less intensive conventional rehabilitation program (10–20 h) concerning its effect on SVMC.
Discussion
This review provides an overview of interventions that might affect SVMC in children with spastic CP and systematically summarizes the evidence on the efficacy of these interventions to improve SVMC. Most of the 23 studies recruited children with unilateral CP. The methodological quality of the studies varied widely, as did the characteristics of participants and dosage of treatments. The interventions covered several therapeutic approaches, broadly grouped into constraint-based interventions, rehabilitation technologies, and other approaches. The consecutive evidence synthesis was performed for specific subgroups, i.e., based on interventions and upper or lower extremity. The overall level of evidence for interventions to improve SVMC in children with spastic CP is conflicting for the subgroups CIMT, robot-assisted therapy approaches and mirror therapy for the upper extremities and absent for trunk or bimanual training. Only a single study in each subgroup prevented a synthesis of results across studies for the effect of robot-assisted lower extremity training, electrical stimulation, exergames, auditory stimulation, elliptical training, action observation therapy, or strength training.
Often, the aims of the studies were formulated rather general like improving upper limb- (39) or motor function (37). Sometimes, the studies investigated a (novel) intervention without explicitly stating the target of the therapy (48, 53, 54). We still included these studies not specifically targeting selective control because they assessed outcomes that cover SVMC and thus could contribute to identify interventions that might improve SVMC. However, the unspecific nature of activity-based interventions can explain why we found only few indications for SVMC improvements.
Among the outcome measures that were included in the studies, there are only a few assessments that specifically measure SVMC (i.e., the selective control assessment of the lower extremity, the selective motor control assessment of ankle dorsiflexion, and the trunk control measurement scale). Indeed, the assessment of SVMC has only recently advanced and there exists only a limited number of tools (25). For the upper extremities, the majority of assessments covered a broad range of motor functions, which included aspects of SVMC in their scoring. A limitation of the SVMC measures for the lower extremity is that their responsiveness to changes has not been evaluated yet (25). In line with the lack of information of the responsiveness, also the minimal clinically important difference has not been determined for most assessments (especially subscales). However, both aspects are relevant when evaluating treatment effects, as done in this review.
Constraint-Based Therapies
CIMT is a commonly used approach for improving upper limb function in children with hemiplegic CP with a lot of variation in its implementation (setting, duration of restraint and therapy) (58). The effect of CIMT on arm function has repeatedly been systematically reviewed in children with CP (59–61). The conclusion was that CIMT provides a beneficial effect compared to a low-dose comparison but is not (59, 60) or only slightly more effective (61) than a control intervention of the same dose. There is a similar trend for the effect of CIMT on SVMC. Studies that found no differences in SVMC between intervention and control groups also applied intensive, dose-matched protocols (39, 40). On the contrary, those studies with a low-dose comparison comprising regular therapy found CIMT to be more efficacious in improving SVMC (38, 43–45). Two studies are inconsistent with this trend by showing CIMT to be more effective than dose-matched control treatments. Although the interventions of Aarts et al.'s study (42) were dose-matched, CIMT was provided by dedicated therapists while the control intervention was mostly parental stimulation of bimanual activities, which might be less intensive. The result of Gelkop et al. (41) might be explained by differences in the outcome existing previous to the intervention that influenced the potential for improvement.
Several studies did not consist of only CIMT sessions, but they either included a small number of bimanual sessions after completion of the CIMT program (35, 42, 45) or low-dose concomitant conventional therapy including bimanual activities (43). The idea of this combination is that bimanual training promotes incorporation of skills acquired during CIMT to meaningful activities (62). Further studies are needed to determine whether combining CIMT and bimanual training is more efficacious for improving SVMC than each intervention alone.
Rehabilitation Technology
Technological advances have led to an increased use of technology for rehabilitation over the last years. The field being under development might explain why our initial search retrieved many uncontrolled studies. These investigations focused on technological development and provided a proof of concept rather than establishing efficacy. Studies including rehabilitation technologies showed a wide variety of approaches. Only for robot-assisted upper limb training, there was more than one study to summarize. In Gilliaux et al.'s study (48), 40% of the training sessions differed between the groups (i.e., the intervention group received robotic therapy for 2/5 weekly sessions) while 60% were similar (i.e., 3/5 weekly sessions for the intervention and 5/5 weekly sessions for the control group consisted of conventional therapy), i.e., a relatively low contrast between intervention and control group. Improvements in SVMC were not significantly different. On the contrary, El-Shamy (47) found a significantly higher improvement in the intervention group, which received only robotic therapy, compared to the control group, which received only conventional therapy, i.e., a large contrast between groups. Although both studies had dose-matched intervention and control conditions, conflicting evidence might be the result of a different degree of contrast between the control and intervention group. Selection of the control condition is also an issue for robot-assisted training of the lower extremities. Comparing the efficacy of a robotic ankle movement training between a home-based and a research setting did not show differences in SVMC improvements but does not allow to draw conclusions about its effect on SVMC compared to standard therapies (49).
Other Approaches
The evidence synthesis did not reveal positive effects on SVMC for any other intervention category. Among three studies on mirror therapy, only Kara et al. (54) found an effect of the intervention on SVMC. Their intervention lasted considerably longer than in the other studies, but intervention and control also differed in a second aspect besides mirror therapy. They complemented mirror therapy with power and strength exercises, while the control group additionally received routine occupational therapy. This design does not allow differentiating which part of the intervention caused the effect. For upper limb action observation and the other two studies on mirror therapy, the reason why they were not more efficacious than the control might be that conditions were too similar (53, 55, 56). Apart from letting the child observe the visual illusion, the intervention did not differ from the control condition. Observation had to be guaranteed by the parents delivering the therapy.
Previous studies showed strength being related to the selective control assessment of the lower extremity (63, 64). Therefore, it could be expected that strengthening programs for the lower extremity might also improve selective control. The efficacy of sit-to-stand exercises to compared to another intervention remains unknown, since Kusumoto et al. (57) only compared different movement speeds. As they also could not find significant within-group improvements, sit-to-stand exercises do not seem to improve SVMC.
Functional training approaches focused on the trunk and bimanual activities. Trunk control is important for upper limb movements by providing a stable base. In children with CP, better trunk control was related to better upper extremity function (65, 66). Therefore, functional trunk training might improve both, selective control of the trunk and the upper extremities, which was however not proven for the latter (37). Concerning bimanual training, the non-significant result of Facchin et al.'s study (38) is surprising because of the huge dose difference between intervention and control group.
Methodological Considerations and Limitations
As the definition of SVMC includes various aspects, defining appropriate search terms was difficult. Our comprehensive search strategy included a variety of terms that describe SVMC. Thereby, we aimed to find publications that investigated selective control but used another wording. However, we still might have missed some studies, which could change the evidence. We grouped the studies by type of intervention, but there are more factors that should be taken into account: a) dosage, which ranged from below 10 h to more than 200 h of therapy; b) selection of the control condition and whether it is dose-matched or a different dosage; c) form of CP and severity of impairment; and d) the outcome measure used. The outcome measure was indeed an important criterion to decide whether a study would be in- or excluded from this systematic review. We studied the test manuals and discussed various assessments extensively before deciding whether an assessment was considered to measure SVMC. We acknowledge that many more studies have been conducted about each type of intervention but these were not included because they lacked a measure of SVMC, which was the focus of this review.
One limitation is the small number of studies that included an intervention specifically targeting SVMC. Gordon (67) claimed that reduced SVMC is one of the least appreciated clinical features of CP, which could explain why we found only the robotic ankle movement training program (49) as an example of an intervention specifically targeting SVMC. The application of more specific outcome measures and interventions would allow more precise conclusions about the efficacy of interventions to enhance SVMC. Initially, we found two other studies on SVMC specific approaches, but these had to be excluded during the review process. The first one encompassed a commercial video game, which is controlled by surface electromyography signals to reinforce desired muscle activity and reduce co-contraction of an agonist-antagonist muscle pair, thus to train selective muscle activation (15). The second study by Adler et al. (18) aimed to reduce mirror movements with a bimanual therapy program developed for this purpose. Since, tailored interventions likely have the potential for yielding larger improvements, they should be further investigated in controlled studies.
Future studies should also clarify whether children can translate improvements in SVMC to more independence in daily life activities, which is an important goal for the patients and their families.
Conclusions
We systematically reviewed the evidence on the efficacy of interventions that may improve SVMC and found a wide variety of approaches. Some studies could show a positive effect on SVMC but the overall level of evidence was conflicting for (m)CIMT, robot-assisted therapy and mirror therapy of the upper extremities in children with CP. We noted a large variability in outcome measures, dosage, and selection of the control intervention.
Data Availability Statement
The original contributions presented in the study are included in the article/Supplementary Materials, further inquiries can be directed to the corresponding author/s.
Author Contributions
AF developed the search strategy, performed the search, study selection and data extraction, and wrote the manuscript. JK was involved in developing the search strategy, study selection, and data extraction. HvH was involved in the search design, study selection, interpretation of results, supervision, and provided the funding. All authors critically reviewed the manuscript and agreed with the final version.
Funding
This work was funded by the Swiss National Science Foundation (No. 32003B_156646, 32003B_179471) and the Mäxi-Foundation.
Conflict of Interest
The authors declare that the research was conducted in the absence of any commercial or financial relationships that could be construed as a potential conflict of interest.
Supplementary Material
The Supplementary Material for this article can be found online at: https://www.frontiersin.org/articles/10.3389/fneur.2020.572038/full#supplementary-material
References
1. Nonnekes J, Benda N, van Duijnhoven H, Lem F, Keijsers N, Louwerens JWK, et al. Management of gait impairments in chronic unilateral upper motor neuron lesions: a review. JAMA Neurol. (2018) 75:751–8. doi: 10.1001/jamaneurol.2017.5041
2. Cahill-Rowley K, Rose J. Etiology of impaired selective motor control: emerging evidence and its implications for research and treatment in cerebral palsy. Dev Med Child Neurol. (2014) 56:522–8. doi: 10.1111/dmcn.12355
3. Sanger TD, Chen D, Delgado MR, Gaebler-Spira D, Hallett M, Mink JW. Definition and classification of negative motor signs in childhood. Pediatrics. (2006) 118:2159–67. doi: 10.1542/peds.2005-3016
4. Fowler EG, Staudt LA, Greenberg MB, Oppenheim WL. Selective Control Assessment of the Lower Extremity (SCALE): development, validation, and interrater reliability of a clinical tool for patients with cerebral palsy. Dev Med Child Neurol. (2009) 51:607–14. doi: 10.1111/j.1469-8749.2008.03186.x
5. Bhattacharya A, Lahiri A. Mirror movement in clinical practice. J Indian Acad Clin Med. (2002) 3:177–81.
6. Rose J, Martin JG, Torburn L, Rinsky LA, Gamble JG. Electromyographic differentiation of diplegic cerebral palsy from idiopathic toe walking: involuntary coactivation of the quadriceps and gastrocnemius. J Pediatr Orthop. (1999) 19:677. doi: 10.1097/01241398-199909000-00025
7. Kuo H-C, Friel KM, Gordon AM. Neurophysiological mechanisms and functional impact of mirror movements in children with unilateral spastic cerebral palsy. Dev Med Child Neurol. (2018) 60:155–61. doi: 10.1111/dmcn.13524
8. WHO. International Classification of Functioning, Disability, and Health: Children & Youth Version: ICF-CY. Geneva: World Health Organization (2007).
9. Adler C, Berweck S, Lidzba K, Becher T, Staudt M. Mirror movements in unilateral spastic cerebral palsy: specific negative impact on bimanual activities of daily living. Eur J Paediatr Neurol. (2015) 19:504–9. doi: 10.1016/j.ejpn.2015.03.007
10. Sukal TM, Ellis MD, Dewald JPA. Shoulder abduction-induced reductions in reaching work area following hemiparetic stroke: neuroscientific implications. Exp Brain Res. (2007) 183:215–23. doi: 10.1007/s00221-007-1029-6
11. Chruscikowski E, Fry NRD, Noble JJ, Gough M, Shortland AP. Selective motor control correlates with gait abnormality in children with cerebral palsy. Gait Posture. (2017) 52:107–9. doi: 10.1016/j.gaitpost.2016.11.031
12. Zhou JY, Lowe E, Cahill-Rowley K, Mahtani GB, Young JL, Rose J. Influence of impaired selective motor control on gait in children with cerebral palsy. J Child Orthop. (2019) 13:73–81. doi: 10.1302/1863-2548.13.180013
13. Noble JJ, Gough M, Shortland AP. Selective motor control and gross motor function in bilateral spastic cerebral palsy. Dev Med Child Neurol. (2019) 61:57–61. doi: 10.1111/dmcn.14024
14. Bryanton C, Bossé J, Brien M, Mclean J, McCormick A, Sveistrup H. Feasibility, motivation, and selective motor control: virtual reality compared to conventional home exercise in children with cerebral palsy. Cyberpsychol Behav. (2006) 9:123–8. doi: 10.1089/cpb.2006.9.123
15. Rios DC, Gilbertson T, McCoy SW, Price R, Gutman K, Miller KEF, et al. NeuroGame Therapy to improve wrist control in children with cerebral palsy: a case series. Dev Neurorehabil. (2013) 16:398–409. doi: 10.3109/17518423.2013.766818
16. Jung JW, Her JG, Ko J. Effect of strength training of ankle plantarfexors on selective voluntary motor control, gait parameters, and gross motor function of children with cerebral palsy. J Phys Ther Sci. (2013) 25:1259–63. doi: 10.1589/jpts.25.1259
17. Kumari R, Verma M, Gupta A. To determine effectiveness of closed-kinetic chain exercise on motor control and function as compared to open-kinetic chain exercise in children with spastic diplegic CP. Indian J Physiother Occup Ther. (2014) 8:33–7. doi: 10.5958/j.0973-5674.8.2.055
18. Adler C, Hessenauer M, Lipp J, Kunze S, Geigenberger C, Hörning A, et al. Learning to cope with mirror movements in unilateral spastic cerebral palsy: a brief report. Dev Neurorehabil. (2019) 22:141–6. doi: 10.1080/17518423.2018.1474501
19. Plasschaert VFP, Vriezekolk JE, Aarts PBM, Geurts ACH, Van den Ende CHM. Interventions to improve upper limb function for children with bilateral cerebral palsy: a systematic review. Dev Med Child Neurol. (2019) 61:899–907. doi: 10.1111/dmcn.14141
20. Lucas BR, Elliott EJ, Coggan S, Pinto RZ, Jirikowic T, McCoy SW, et al. Interventions to improve gross motor performance in children with neurodevelopmental disorders: a meta-analysis. BMC Pediatr. (2016) 16:193. doi: 10.1186/s12887-016-0731-6
21. Reedman S, Boyd RN, Sakzewski L. The efficacy of interventions to increase physical activity participation of children with cerebral palsy: a systematic review and meta-analysis. Dev Med Child Neurol. (2017) 59:1011–8. doi: 10.1111/dmcn.13413
22. Moreau NG, Bodkin AW, Bjornson K, Hobbs A, Soileau M, Lahasky K. Effectiveness of rehabilitation interventions to improve gait speed in children with cerebral palsy: systematic review and meta-analysis. Phys Ther. (2016) 96:1938–54. doi: 10.2522/ptj.20150401
23. Liberati A, Altman DG, Tetzlaff J, Mulrow C, Gøtzsche PC, Ioannidis JPA, et al. The PRISMA statement for reporting systematic reviews and meta-analyses of studies that evaluate health care interventions: explanation and elaboration. PLoS Med. (2009) 6:e1000100. doi: 10.1371/journal.pmed.1000100
24. Cans C, Dolk H, Platt MJ, Colver A others. Recommendations from the SCPE collaborative group for defining and classifying cerebral palsy. Dev Med Child Neurol. (2007) 49:35. doi: 10.1111/j.1469-8749.2007.tb12626.x
25. Balzer J, van der Linden ML, Mercer TH, van Hedel HJA. Selective voluntary motor control measures of the lower extremity in children with upper motor neuron lesions: a systematic review. Dev Med Child Neurol. (2017) 59:699–705. doi: 10.1111/dmcn.13417
26. Darrah J, Hickman R, O'Donnell M, Vogtle L, Wiart L. AACPDM Methodology to Develop Systematic Reviews of Treatment Interventions (Revision 1.2). (2008). Available online at: https://www.aacpdm.org/UserFiles/file/systematic-review-methodology.pdf (accessed November 19, 2020).
27. Higgins J, Green S, editors. Cochrane Handbook for Systematic Reviews of Interventions. Version 5.1.0 [updated March 2011]: The Cochrane Collaboration (2011). Available online at: www.handbook.cochrane.org (accessed December 11, 2018).
28. van Tulder M, Furlan A, Bombardier C, Bouter L, Editorial Board of the Cochrane Collaboration Back Review Group. Updated method guidelines for systematic reviews in the cochrane collaboration back review group. Spine. (2003) 28:1290–9. doi: 10.1097/01.BRS.0000065484.95996.AF
29. Borenstein M, Hedges L V, Higgins JPT, Rothstein HR. Effect sizes based on means. In: Borenstein M, Hedges LV, Higgins JPT and Rothstein HR, editors. Introduction to Meta-Analysis. Chichester: Wiley (2009). p. 25–8. doi: 10.1002/9780470743386
30. Kwon JY, Chang WH, Chang HJ, Yi SH, Kim MY, Kim EH, et al. Changes in diffusion tensor tractographic findings associated with constraint-induced movement therapy in young children with cerebral palsy. Clin Neurophysiol. (2014) 125:2397–403. doi: 10.1016/j.clinph.2014.02.025
31. Motta F, Antonello CE, Stignani C. Forced-use, without therapy, in children with hemiplegia: preliminary study of a new approach for the upper limb. J Pediatr Orthop. (2010) 30:582–7. doi: 10.1097/BPO.0b013e3181e88ee4
32. Hughes A, Franzsen D, Freeme J. The effect of neoprene thumb abduction splints on upper limb function in children with cerebral palsy. South African J Occup Ther. (2017) 47:3–10. doi: 10.17159/2310-3833/2017/v47n3a2
33. Abd El-Kafy EM, Elshemy SA, Alghamdi MS. Effect of constraint-induced therapy on upper limb functions: a randomized control trial. Scand J Occup Ther. (2014) 21:11–23. doi: 10.3109/11038128.2013.837505
34. Karabay I, Öztürk GT, Malas FÜ, Kara M, Tiftik T, Ersöz M, et al. Short-term effects of neuromuscular electrical stimulation on muscle architecture of the tibialis anterior and gastrocnemius in children with cerebral palsy: preliminary results of a prospective controlled study. Am J Phys Med Rehabil. (2015) 94:728–33. doi: 10.1097/PHM.0000000000000238
35. DeLuca SC, Case-Smith J, Stevenson R, Ramey SL. Constraint-induced movement therapy (CIMT) for young children with cerebral palsy: effects of therapeutic dosage. J Pediatr Rehabil Med. (2012) 5:133–42. doi: 10.3233/PRM-2012-0206
36. Pool D, Elliott C, Bear N, Donnelly CJ, Davis C, Stannage K, et al. Neuromuscular electrical stimulation-assisted gait increases muscle strength and volume in children with unilateral spastic cerebral palsy. Dev Med Child Neurol. (2016) 58:492–501. doi: 10.1111/dmcn.12955
37. Numanoglu Akbas A, Kerem Günel M. Effects of trunk training on trunk, upper and lower limb motor functions in children with spastic cerebral palsy: a stratified randomized controlled trial. Konuralp Med J. (2019) 11:253–9. doi: 10.18521/ktd.453532
38. Facchin P, Rosa-Rizzotto M, Visonà Dalla Pozza L, Turconi AC, Pagliano E, Signorini S, et al. Multisite trial comparing the efficacy of constraint-induced movement therapy with that of bimanual intensive training in children with hemiplegic cerebral palsy: postintervention results. Am J Phys Med Rehabil. (2011) 90:539–53. doi: 10.1097/PHM.0b013e3182247076
39. Christmas PM, Sackley C, Feltham MG, Cummins C. A randomized controlled trial to compare two methods of constraint-induced movement therapy to improve functional ability in the affected upper limb in pre-school children with hemiplegic cerebral palsy: CATCH TRIAL. Clin Rehabil. (2018) 32:909–18. doi: 10.1177/0269215518763512
40. Gordon AM, Hung Y-C, Brandao M, Ferre CL, Kuo H-C, Friel K, et al. Bimanual training and constraint-induced movement therapy in children with hemiplegic cerebral palsy: a randomized trial. Neurorehabil Neural Repair. (2011) 25:692–702. doi: 10.1177/1545968311402508
41. Gelkop N, Burshtein DG, Lahav A, Brezner A, Al-Oraibi S, Ferre CL, et al. Efficacy of constraint-induced movement therapy and bimanual training in children with hemiplegic cerebral palsy in an educational setting. Phys Occup Ther Pediatr. (2015) 35:24–39. doi: 10.3109/01942638.2014.925027
42. Aarts PB, Jongerius PH, Geerdink YA, van Limbeek J, Geurts AC. Modified constraint-induced movement therapy combined with bimanual training (mCIMT-BiT) in children with unilateral spastic cerebral palsy: how are improvements in arm-hand use established? Res Dev Disabil. (2011) 32:271–9. doi: 10.1016/j.ridd.2010.10.008
43. Choudhary A, Gulati S, Kabra M, Singh UP, Sankhyan N, Pandey RM, et al. Efficacy of modified constraint induced movement therapy in improving upper limb function in children with hemiplegic cerebral palsy: a randomized controlled trial. Brain Dev. (2013) 35:870–6. doi: 10.1016/j.braindev.2012.11.001
44. DeLuca SC, Echols K, Law CR, Ramey SL. Intensive pediatric constraint-induced therapy for children with cerebral palsy: randomized, controlled, crossover trial. J Child Neurol. (2006) 21:931–8. doi: 10.1177/08830738060210110401
45. Taub E, Griffin A, Uswatte G, Gammons K, Nick J, Law CR. Treatment of congenital hemiparesis with pediatric constraint-induced movement therapy. J Child Neurol. (2011) 26:1163–73. doi: 10.1177/0883073811408423
46. Schneiberg S, McKinley PA, Sveistrup H, Gisel E, Mayo NE, Levin MF. The effectiveness of task-oriented intervention and trunk restraint on upper limb movement quality in children with cerebral palsy. Dev Med Child Neurol. (2010) 52:e245–53. doi: 10.1111/j.1469-8749.2010.03768.x
47. El-Shamy SM. Efficacy of Armeo® robotic therapy versus conventional therapy on upper limb function in children with hemiplegic cerebral palsy. Am J Phys Med Rehabil. (2018) 97:164–9. doi: 10.1097/PHM.0000000000000852
48. Gilliaux M, Renders A, Dispa D, Holvoet D, Sapin J, Dehez B, et al. Upper limb robot-assisted therapy in cerebral palsy: a single-blind randomized controlled trial. Neurorehabil Neural Repair. (2015) 29:183–92. doi: 10.1177/1545968314541172
49. Chen K, Wu Y-N, Ren Y, Liu L, Gaebler-Spira D, Tankard K, et al. Home-based versus laboratory-based robotic ankle training for children with cerebral palsy: a pilot randomized comparative trial. Arch Phys Med Rehabil. (2016) 97:1237–43. doi: 10.1016/j.apmr.2016.01.029
50. Acar G, Altun GP, Yurdalan S, Polat MG. Efficacy of neurodevelopmental treatment combined with the Nintendo® Wii in patients with cerebral palsy. J Phys Ther Sci. (2016) 28:774–80. doi: 10.1589/jpts.28.774
51. Damiano DL, Stanley CJ, Ohlrich L, Alter KE. Task-specific and functional effects of speed-focused elliptical or motor-assisted cycle training in children with bilateral cerebral palsy: randomized clinical trial. Neurorehabil Neural Repair. (2017) 31:736–45. doi: 10.1177/1545968317718631
52. Ben-Pazi H, Aran A, Pandyan A, Gelkop N, Ginsberg G, Pollak Y, et al. Auditory stimulation improves motor function and caretaker burden in children with cerebral palsy—a randomized double blind study. PLoS ONE. (2018) 13:e0208792. doi: 10.1371/journal.pone.0208792
53. Bruchez R, Gygax MJ, Roches S, Fluss J, Jacquier D, Ballabeni P, et al. Mirror therapy in children with hemiparesis: a randomized observer-blinded trial. Dev Med Child Neurol. (2016) 58:970–8. doi: 10.1111/dmcn.13117
54. Kara OK, Yardimci BN, Sahin S, Orhan C, Livanelioglu A, Soylu AR. Combined effects of mirror therapy and exercises on the upper extremities in children with unilateral cerebral palsy: a randomized controlled trial. Dev Neurorehabil. (2019) 23:253–64. doi: 10.1080/17518423.2019.1662853
55. Gygax MJ, Schneider P, Newman CJ. Mirror therapy in children with hemiplegia: a pilot study. Dev Med Child Neurol. (2011) 53:473–6. doi: 10.1111/j.1469-8749.2011.03924.x
56. Kirkpatrick E, Pearse J, James P, Basu A. Effect of parent-delivered action observation therapy on upper limb function in unilateral cerebral palsy: a randomized controlled trial. Dev Med Child Neurol. (2016) 58:1049–56. doi: 10.1111/dmcn.13109
57. Kusumoto Y, Nitta O, Takaki K. Impact of loaded sit-to-stand exercises at different speeds on the physiological cost of walking in children with spastic diplegia: a single-blind randomized clinical trial. Res Dev Disabil. (2016) 57:85–91. doi: 10.1016/j.ridd.2016.06.006
58. Eliasson AC, Krumlinde-Sundholm L, Gordon AM, Feys H, Klingels K, Aarts PBM, et al. Guidelines for future research in constraint-induced movement therapy for children with unilateral cerebral palsy: an expert consensus. Dev Med Child Neurol. (2014) 56:125–37. doi: 10.1111/dmcn.12273
59. Chiu H-C, Ada L. Constraint-induced movement therapy improves upper limb activity and participation in hemiplegic cerebral palsy: a systematic review. J Physiother. (2016) 62:130–7. doi: 10.1016/j.jphys.2016.05.013
60. Hoare BJ, Wallen MA, Thorley MN, Jackman ML, Carey LM, Imms C. Constraint-induced movement therapy in children with unilateral cerebral palsy. Cochrane Database Syst Rev. (2019) 4:CD004149. doi: 10.1002/14651858.CD004149.pub3
61. Chen Y, Pope S, Tyler D, Warren GL. Effectiveness of constraint-induced movement therapy on upper-extremity function in children with cerebral palsy: a systematic review and meta-analysis of randomized controlled trials. Clin Rehabil. (2014) 28:939–53. doi: 10.1177/0269215514544982
62. de Brito Brandão M, Mancini MC, Vaz DV, de Melo AP, Fonseca ST. Adapted version of constraint-induced movement therapy promotes functioning in children with cerebral palsy: a randomized controlled trial. Clin Rehabil. (2010) 24:639–47. doi: 10.1177/0269215510367974
63. Balzer J, Marsico P, Mitteregger E, van der Linden ML, Mercer TH, van Hedel HJA. Construct validity and reliability of the Selective Control Assessment of the Lower Extremity in children with cerebral palsy. Dev Med Child Neurol. (2016) 58:167–72. doi: 10.1111/dmcn.12805
64. Kusumoto Y, Takaki K, Matsuda T, Nitta O. Relation of selective voluntary motor control of the lower extremity and extensor strength of the knee joint in children with spastic diplegia. J Phys Ther Sci. (2016) 28:1868–71. doi: 10.1589/jpts.28.1868
65. Yildiz A, Yildiz R, Elbasan B. Trunk control in children with cerebral palsy and its association with upper extremity functions. J Dev Phys Disabil. (2018) 30:669–76. doi: 10.1007/s10882-018-9611-3
66. Kim DH, An D-H, Yoo W-G. The relationship between trunk control and upper limb function in children with cerebral palsy. Technol Heal Care. (2018) 26:421–7. doi: 10.3233/THC-171073
Keywords: selective voluntary motor control, involuntary movements, pediatric neurorehabilitation, cerebral palsy, best evidence synthesis
Citation: Fahr A, Keller JW and van Hedel HJA (2020) A Systematic Review of Training Methods That May Improve Selective Voluntary Motor Control in Children With Spastic Cerebral Palsy. Front. Neurol. 11:572038. doi: 10.3389/fneur.2020.572038
Received: 12 June 2020; Accepted: 09 November 2020;
Published: 04 December 2020.
Edited by:
Andrea Martinuzzi, Eugenio Medea (IRCCS), ItalyReviewed by:
Birgitta Langhammer, Oslo Metropolitan University, NorwayChiara Gagliardi, Eugenio Medea (IRCCS), Italy
Copyright © 2020 Fahr, Keller and van Hedel. This is an open-access article distributed under the terms of the Creative Commons Attribution License (CC BY). The use, distribution or reproduction in other forums is permitted, provided the original author(s) and the copyright owner(s) are credited and that the original publication in this journal is cited, in accordance with accepted academic practice. No use, distribution or reproduction is permitted which does not comply with these terms.
*Correspondence: Annina Fahr, YW5uaW5hLmZhaHImI3gwMDA0MDtraXNwaS51emguY2g=