- Department of Neurology, Johns Hopkins University School of Medicine, Baltimore, MD, United States
Background: Optic neuritis (ON) is a cardinal manifestation of multiple sclerosis (MS), aquaporin-4 (AQP4)-IgG-, and myelin oligodendrocyte glycoprotein (MOG)-IgG-associated disease. However, the prevalence of AQP4-IgG seropositivity and MOG-IgG seropositivity in isolated ON is unclear, and studies comparing visual outcomes and optical coherence tomography (OCT)-derived structural retinal measures between MS-ON, AQP4-ON, and MOG-ON eyes are limited by small sample sizes.
Objectives: (1) To assess the prevalence of AQP4-IgG and MOG-IgG seropositivity among patients presenting with isolated ON; (2) to compare visual outcomes and OCT measures between AQP4-ON, MOG-ON, and MS-ON eyes.
Methods: In this systematic review and meta-analysis, a total of 65 eligible studies were identified by PubMed search. Statistical analyses were performed with random effects models.
Results: In adults with isolated ON, AQP4-IgG seroprevalence was 4% in non-Asian and 27% in Asian populations, whereas MOG-IgG seroprevalence was 8 and 20%, respectively. In children, AQP4-IgG seroprevalence was 0.4% in non-Asian and 15% in Asian populations, whereas MOG-IgG seroprevalence was 47 and 31%, respectively. AQP4-ON eyes had lower peri-papillary retinal nerve fiber layer (pRNFL; −11.7 μm, 95% CI: −15.2 to −8.3 μm) and macular ganglion cell + inner plexiform layer (GCIPL; −9.0 μm, 95% CI: −12.5 to −5.4 μm) thicknesses compared with MS-ON eyes. Similarly, pRNFL (−11.2 μm, 95% CI: −21.5 to −0.9 μm) and GCIPL (−6.1 μm, 95% CI: −10.8 to −1.3 μm) thicknesses were lower in MOG-ON compared to MS-ON eyes, but did not differ between AQP4-ON and MOG-ON eyes (pRNFL: −1.9 μm, 95% CI: −9.1 to 5.4 μm; GCIPL: −2.6 μm, 95% CI: −8.9 to 3.8 μm). Visual outcomes were worse in AQP4-ON compared to both MOG-ON (mean logMAR difference: 0.60, 95% CI: 0.39 to 0.81) and MS-ON eyes (mean logMAR difference: 0.68, 95% CI: 0.40 to 0.96) but were similar in MOG-ON and MS-ON eyes (mean logMAR difference: 0.04, 95% CI: −0.05 to 0.14).
Conclusions: AQP4-IgG- and MOG-IgG-associated disease are important diagnostic considerations in adults presenting with isolated ON, especially in Asian populations. Furthermore, MOG-IgG seroprevalence is especially high in pediatric isolated ON, in both non-Asian and Asian populations. Despite a similar severity of GCIPL and pRNFL thinning in AQP4-ON and MOG-ON, AQP4-ON is associated with markedly worse visual outcomes.
Introduction
Optic neuritis (ON) is a cardinal manifestation of inflammatory conditions of the central nervous system (CNS), including multiple sclerosis (MS), aquaporin-4 (AQP4)-IgG-, and myelin oligodendrocyte glycoprotein (MOG)-IgG-associated disease (1–3). Early recognition of the underlying etiology of ON has important therapeutic implications, given that treatment approaches vary between these conditions, and therapies that are efficacious in MS may exacerbate or be ineffective in AQP4-IgG- or MOG-IgG-associated disease (4, 5). Furthermore, visual prognosis appears to differ between these conditions, with AQP4-IgG-associated ON (AQP4-ON) typically characterized by worse visual outcomes in comparison to MS-associated ON (MS-ON) and MOG-IgG-associated ON (MOG-ON) (6–8). In patients presenting with classic neuromyelitis optica (NMO) or acute disseminated encephalomyelitis (ADEM)-like phenotypes, clinical suspicion for AQP4-IgG- or MOG-IgG-associated disease is high, but diagnosis may be challenging and delayed in limited forms, such as isolated ON. Notably, the reported prevalence of AQP4-IgG and MOG-IgG seropositivity among patients presenting with isolated ON varies significantly between studies, and the available literature suggests that seropositivity for these antibodies is more common in non-white populations with ON (9, 10).
Optic nerve injury results in thinning of the retinal nerve fiber layer (RNFL), which is mainly composed of the unmyelinated axons of the retinal ganglion cells (RGCs), and the ganglion cell layer, which contains the cell bodies of the RGCs (1). Optical coherence tomography (OCT) is an imaging technique that utilizes near-infrared light to obtain high-resolution images of the retina in vivo and enables the quantitative evaluation of individual retinal layers, allowing assessment of the integrity of the RGC axons [peri-papillary RNFL thickness (pRNFL)] and RGC cell bodies [composite thickness of the macular ganglion cell + inner plexiform layer (GCIPL)] (11, 12). OCT studies have generally demonstrated increased severity of pRNFL and GCIPL thinning following AQP4-ON or MOG-ON, as compared to MS-ON (8). However, given the rarity of AQP4-IgG- and MOG-IgG-associated disease, OCT studies have examined relatively small numbers of participants, not permitting an in-depth characterization and comparison of the retinal neuro-axonal injury that occurs in these conditions.
The primary objectives of this systematic review and meta-analysis were as follows: (1) To determine the seroprevalence of AQP4-IgG and MOG-IgG among patients presenting with isolated ON, and to explore variation in prevalence by geographical location/ethnicity. (2) To assess pRNFL and GCIPL thicknesses in AQP4-ON and MOG-ON eyes (including comparisons to MS-ON and healthy controls), and to investigate whether distinct patterns of retinal injury are associated with AQP4-ON or MOG-ON. (3) To compare visual outcomes between AQP4-ON, MOG-ON, and MS-ON eyes.
Methods
The present systematic review and meta-analysis is reported according to the Preferred Reporting Items of Systematic Reviews and Meta-Analyses (PRISMA) statement and the Meta-analysis of Observational Studies in Epidemiology (MOOSE) guidelines (13, 14).
Search Strategy and Study Selection
The PubMed electronic database was queried using search algorithms (available in detail in Supplementary Table 1) including the following keywords: “mog,” “myelin oligodendrocyte glycoprotein,” “nmo,” “neuromyelitis optica,” “aquaporin 4,” “aqp4,” “aquaporin-4,” “optic neuritis,” “optical coherence tomography,” “retina,” “nerve fiber layer,” “ganglion cell,” “vision,” “visual outcome,” and “disability.” Databases were last accessed on October 29, 2019.
All retrieved studies were imported into the Covidence platform for study eligibility screening and inclusion. The studies were screened independently by two reviewers (AGF and LM), and in cases of disagreement, another reviewer (ESS) was consulted.
For our first study objective (assessing the prevalence of AQP4-IgG and MOG-IgG seropositivity in isolated ON), we identified all studies that reported the frequency of AQP4-IgG and/or MOG-IgG seropositivity in a cohort of patients presenting with an initial episode of isolated (monosymptomatic) unilateral or bilateral ON. Study exclusion criteria included the following: (1) studies that did not report the number of patients with pre-existing diagnoses of MS or neuromyelitis optica spectrum disorder (NMOSD) or with prior episodes of neurological dysfunction, (2) n < 10 participants, and (3) unclear criteria for participant inclusion or inclusion only of selected high-risk patient subgroups (e.g., bilateral or recurrent ON, normal brain MRI). As secondary analyses, we also identified studies reporting the prevalence of AQP4-IgG and MOG-IgG seropositivity in patients presenting with recurrent isolated (unilateral or bilateral) ON or bilateral simultaneous/rapidly sequential ON.
For our second study objective (comparison of OCT measures between AQP4-ON, MOG-ON, and MS-ON eyes), we identified studies that reported OCT measures from patients with AQP4-ON and/or MOG-ON and included data permitting at least one of the following comparisons: (1) AQP4-ON vs. healthy control (HC) eyes, (2) MOG-ON vs. HC eyes, (3) AQP4-ON vs. MOG-ON eyes, (4) AQP4-ON vs. MS-ON eyes, and (5) MOG-ON vs. MS-ON eyes. Comparison of MS-ON vs HC eyes was not performed as this was not the focus of our study and this has been reported in a recent large meta-analysis (15).
Similarly, for our third study objective (comparison of visual outcomes in AQP4-ON, MOG-ON, and MS-ON eyes), studies were included that reported visual outcomes in AQP4-ON and/or MOG-ON and included data permitting at least one of the following comparisons: (1) AQP4-ON vs. MOG-ON, (2) AQP4-ON vs. MS-ON, and (3) MOG-ON vs. MS-ON.
For our analyses of OCT and visual outcomes, we only included articles with assessments of ON eyes performed at least 3 months after an episode of acute ON. For studies that collected the data necessary for our analyses but did not report the results in a manner appropriate for our purposes (e.g., not separating eyes by ON history, reporting combined estimates for AQP4-IgG seropositive and seronegative NMOSD patients), corresponding authors were contacted and were asked to provide additional information. If this information was not made available, these studies were excluded. Additional unpublished data from the cohorts included in the manuscripts was occasionally provided, at the discretion of the corresponding authors. For the OCT component, studies were also excluded if they did not utilize spectral-domain OCT.
When two or more similar studies (fulfilling inclusion criteria) were reported from the same institution or author with unclear participant overlap between studies, authors were contacted to provide clarification. When unable to obtain this information, the publication with the highest number of participants was included in the analysis. Case reports, reviews, or studies published in a non-English language were excluded. Reference lists of relevant review articles were also examined to identify studies that may have been missed during the initial database search.
Data Extraction and Outcomes
Two investigators (AGF and LM) independently conducted the data extraction, and any discrepancies were resolved by consensus.
For assessment of the prevalence of AQP4-IgG and MOG-IgG in isolated ON, we recorded the total number of patients presenting with an isolated ON in each study (excluding patients with a prior neurological history), and the number of patients that tested positive for AQP4-IgG or MOG-IgG.
The main outcome measures for OCT analyses were the thicknesses (μm) of the pRNFL and the macular GCIPL [or macular ganglion cell layer complex (GCC), which additionally includes the macular RNFL] of eyes with a history of ON, and this information was recorded for each group as the mean ± SD. Additional data on quadrantal pRNFL thicknesses were collected, if available. For studies that reported OCT measures as median/interquartile range and the corresponding authors had not provided the mean ± SD, a normal distribution was assumed to calculate the SD. If macular OCT measures were reported as volumes, they were converted to thicknesses according to the formula: Thickness = Volume/Surface Area. For macular measures, the region of interest varied between studies (e.g., perifoveal area of 3 or 6 mm in diameter, including or excluding the foveal subfield); thus, the surface area was calculated separately for each study, depending on the utilized protocol. While not a primary focus of this study, we also recorded (when available) the prevalence of microcystoid macular pathology (MMP; also referred to as microcystic macular edema in the literature) in AQP4-ON and MOG-ON eyes (16–18).
For visual outcomes, the main outcome measures were the logarithm of the minimum angle of resolution (logMAR) in eyes with a history of ON and the percentage of affected eyes with high-contrast visual acuity (VA) worse than 20/200.
For MOG-IgG serostatus, only studies that reported using cell-based assays (CBAs) for testing were included, whereas for AQP4-IgG serostatus, studies utilized a variety of assays, including CBAs, indirect tissue immunofluorescence, enzyme-linked immunosorbent assay (ELISA) or fluorescence-based immunoprecipitation assay (FIPA).
Data were extracted from cross-sectional cohorts and from a single time point from longitudinal studies (typically the baseline assessment).
Data Synthesis and Statistical Analysis
For all study objectives, studies of pediatric participants were examined separately.
We estimated the pooled AQP4-IgG and MOG-IgG prevalence in isolated ON separately for Asian and non-Asian populations, given the divergence of prevalence between studies in these populations, and evidence supporting higher prevalence of NMOSD in Asian populations (10). Given the relatively low prevalence of these disorders in some of the included studies (estimates close to 0%), we utilized the variance-stabilizing double arcsine transformation method (19).
OCT measures were handled as continuous variables. Results are presented as mean differences between the groups of interest. OCT measures from different spectral-domain OCT devices were analyzed together, similar to a prior large meta-analysis in MS, given that, at a group level, it appears that data are comparable across devices and segmentation algorithms (15, 20). In terms of macular OCT measures, the GCIPL and GCC were analyzed together, given that the GCIPL accounts for the majority of the thickness of the GCC. Additionally, we estimated the pooled prevalence of MMP in AQP4-ON and MOG-ON eyes.
For studies reporting VA measurements in logMAR format, logMAR was handled as a continuous variable and results are presented as mean differences between groups of interest. For studies reporting visual outcomes as percentage of eyes with VA worse than 20/200, we calculated the relative risk of this unfavorable visual outcome (i.e., VA < 20/200). Studies reporting visual outcomes in any other formats were included in the qualitative, but not the quantitative, synthesis.
All analyses were performed with random effects models, since the heterogeneity was expected to be high due to varying OCT devices, differing scan protocols and macular regions of interest, and differences in the demographic and clinical characteristics of the participants across studies. To minimize the impact of the study heterogeneity, we did not compare OCT measures or visual outcomes across studies; rather, we estimated between-group differences in each study and then performed a pooled analysis of these estimated differences. We assessed for heterogeneity between the included studies using the I2 estimate. I2 > 75% was considered to indicate significant heterogeneity.
Statistical analyses were performed with Stata version 16 (StataCorp, College Station, TX). For the meta-analysis of prevalence, the Stata package “metaprop” was used (21).
Results
Prevalence of AQP4-IgG and MOG-IgG Seropositivity in Monosymptomatic ON
Study Selection and Study Characteristics
A PubMed search identified 1,187 records. Of these, 197 articles were selected and assessed for eligibility at the full-text level. After careful evaluation, 21 studies, comprising 1,876 patients, were included that met the inclusion criteria (22–42). The detailed flow chart is presented in Supplementary Figure 1. For our secondary analysis in patients with recurrent ON, six studies, comprising 510 patients, were included that met our inclusion criteria (26, 43–47). There was an insufficient number of studies/participants to analyze the prevalence of AQP4-IgG or MOG-IgG seropositivity among patients presenting with isolated bilateral simultaneous or sequential ON. The included studies are summarized in Table 1.
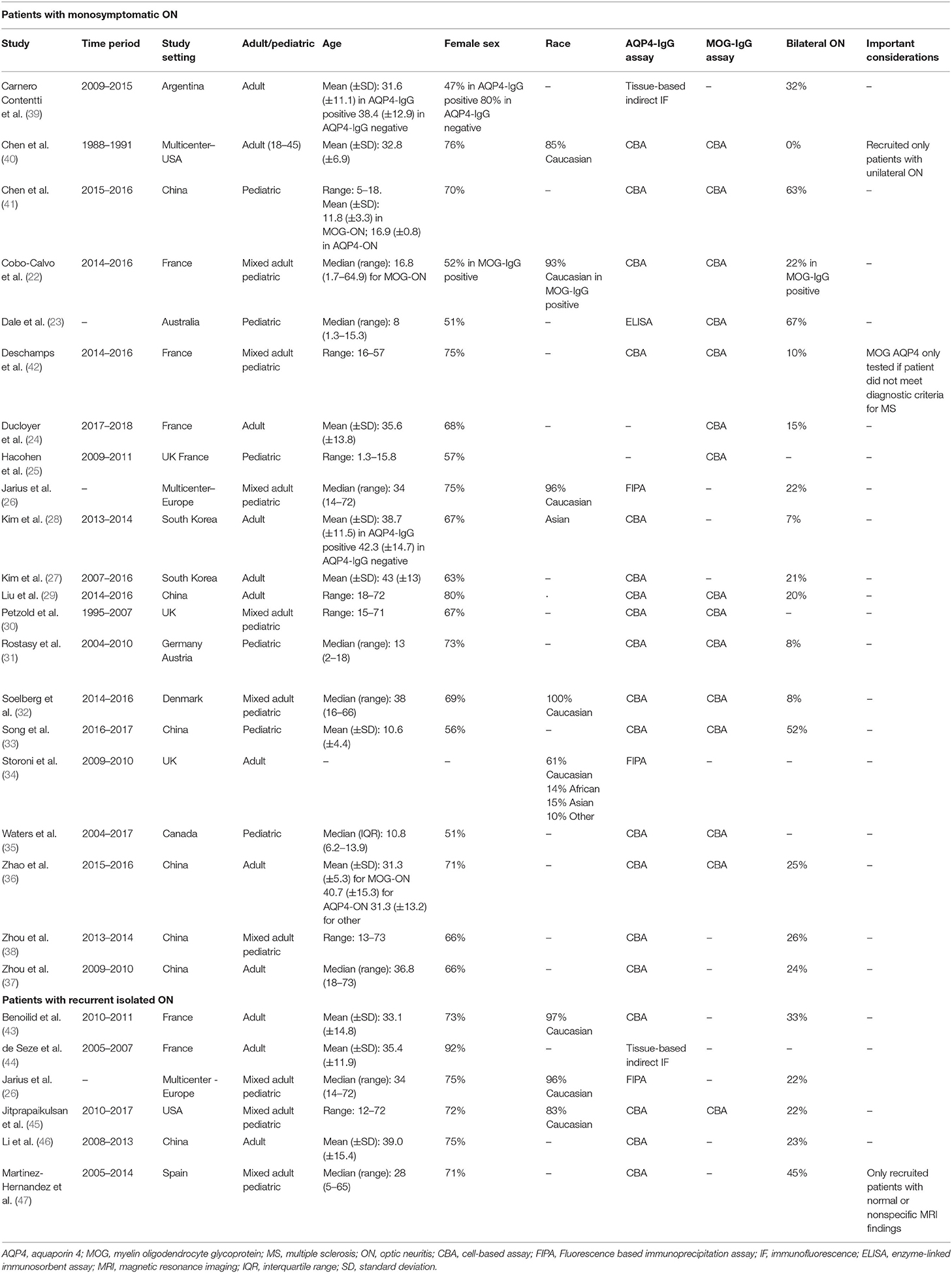
Table 1. Characteristics of studies included in the meta-analysis for our first study objective (assessing the prevalence of AQP4-IgG and MOG-IgG seropositivity in isolated ON).
AQP4-IgG Prevalence in Monosymptomatic ON
The pooled prevalence of AQP4-IgG seropositivity in adults with isolated ON (Figure 1) was 4% in non-Asian cohorts (95% CI: 0 to 11%) and 27% in Asian cohorts (95% CI: 19 to 36%). In pediatric cohorts (Figure 2), similar to adults, AQP4-IgG seroprevalence was again higher in Asian cohorts (15%; 95% CI: 9 to 23%), whereas in the three available studies of non-Asian populations, the prevalence of AQP4-IgG seropositivity was 0.4% (95% CI: 0 to 3.2%).
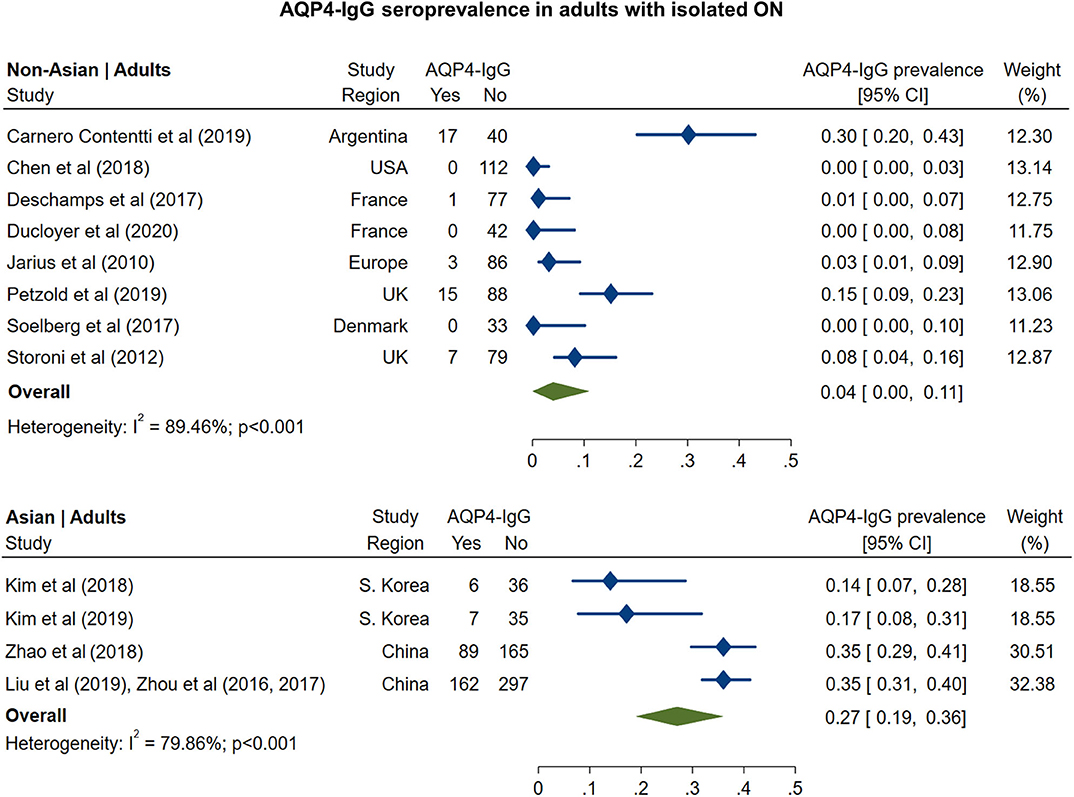
Figure 1. Forest plot of the prevalence of AQP4-IgG seropositivity in adults with monosymptomatic isolated ON.
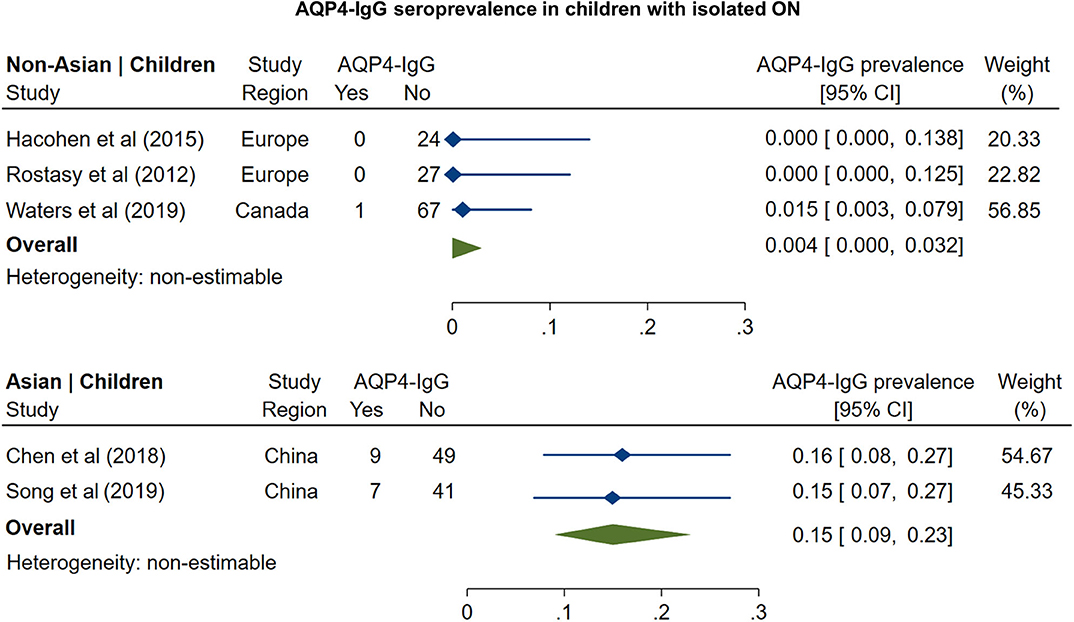
Figure 2. Forest plot of the prevalence of AQP4-IgG seropositivity in children with monosymptomatic isolated ON.
MOG-IgG Prevalence in Monosymptomatic ON
The prevalence of MOG-IgG seropositivity in adults with isolated ON (Figure 3) was 8% in non-Asian cohorts (95% CI: 4 to 13%) and 20% in Asian cohorts (95% CI: 16 to 24%). In pediatric cohorts (Figure 4), in contrast to adults, MOG-IgG seroprevalence was higher in non-Asian populations (47%; 95% CI: 36 to 58%) relative to Asian populations (31%; 95% CI: 22 to 40%), but both had higher prevalence compared to adults.
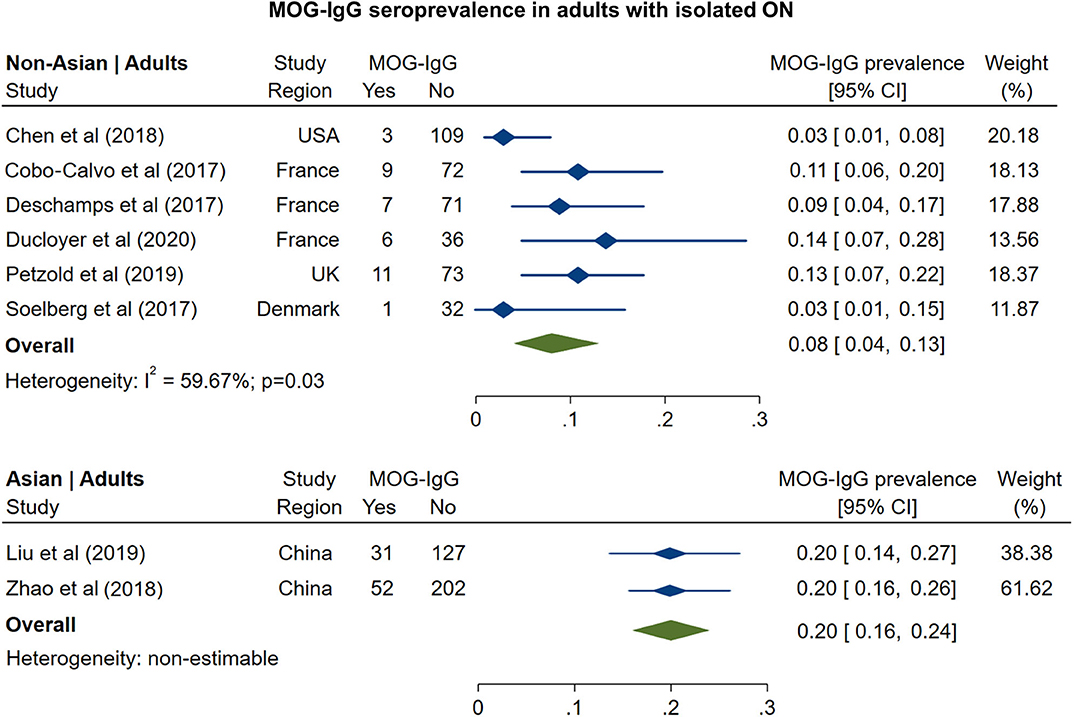
Figure 3. Forest plot of the prevalence of MOG-IgG seropositivity in adults with monosymptomatic isolated ON.
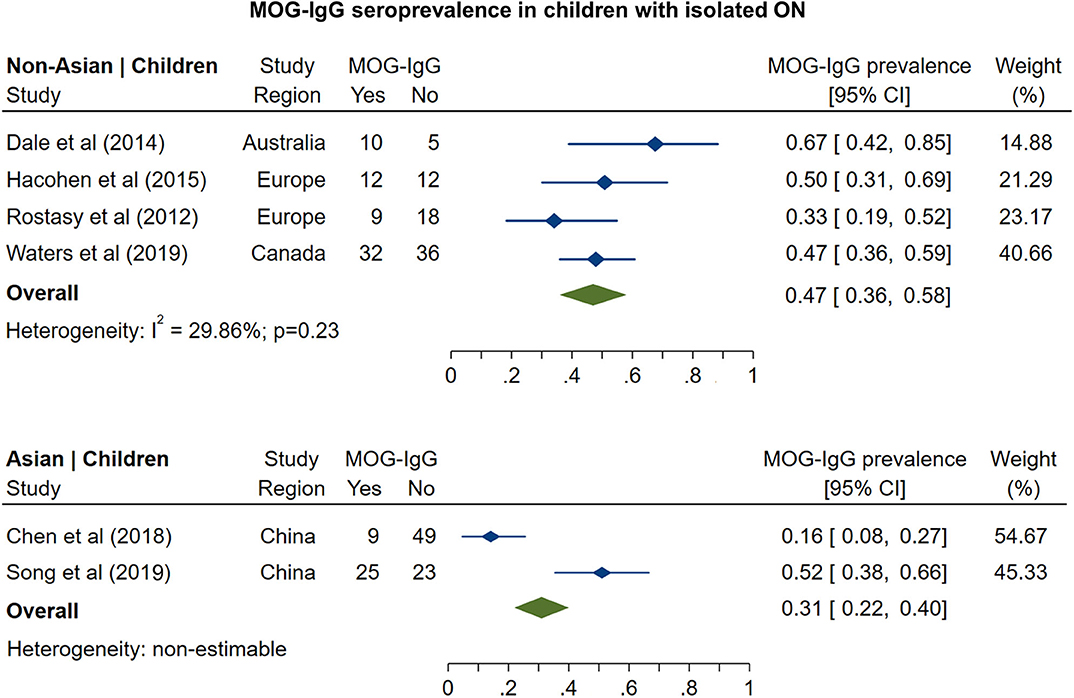
Figure 4. Forest plot of the prevalence of MOG-IgG seropositivity in children with monosymptomatic isolated ON.
AQP4-IgG and MOG-IgG Prevalence in Recurrent Isolated ON
In non-Asian cohorts, the prevalence of AQP4-IgG seropositivity in patients with recurrent isolated ON (Figure 5) was 16% (95% CI: 12 to 21%). Only one study reported the frequency of AQP4-IgG seropositivity in Asian patients with recurrent ON (41%; 95% CI: 31 to 51%). For MOG-IgG, we were able to identify only two studies fulfilling the inclusion criteria; based on these studies (Figure 6), the prevalence of MOG-IgG seropositivity in non-Asian cohorts with recurrent ON was 15% (95% CI: 11 to 19%). No eligible pediatric studies were identified.
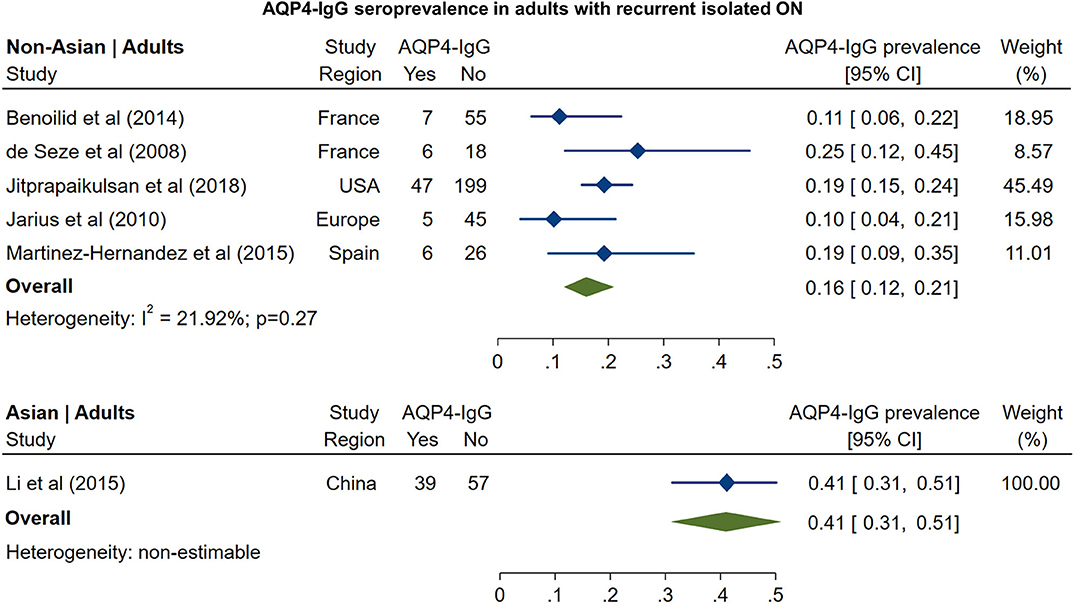
Figure 5. Forest plot of the prevalence of AQP4-IgG seropositivity in adults with recurrent isolated ON.
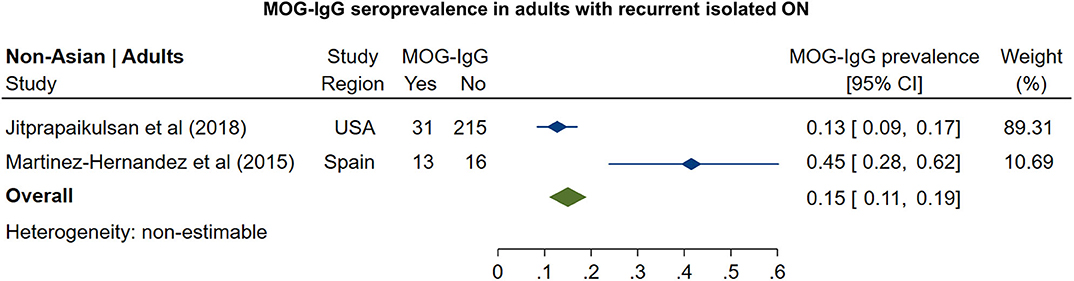
Figure 6. Forest plot of the prevalence of MOG-IgG seropositivity in adults with recurrent isolated ON.
OCT Findings in AQP4-ON and MOG-ON
Study Selection and Study Characteristics
A PubMed search identified 351 records. Of these, 98 articles were selected and assessed for eligibility at the full-text level. After careful evaluation, 31 studies were included that met the inclusion criteria (8, 29, 33, 36, 41, 48–73). The detailed flow chart is presented in Supplementary Figure 2. The included studies, comprising a total of 814 HC eyes, 611 AQP4-ON eyes, 237 MOG-ON eyes, and 361 MS-ON eyes, are summarized in Table 2.
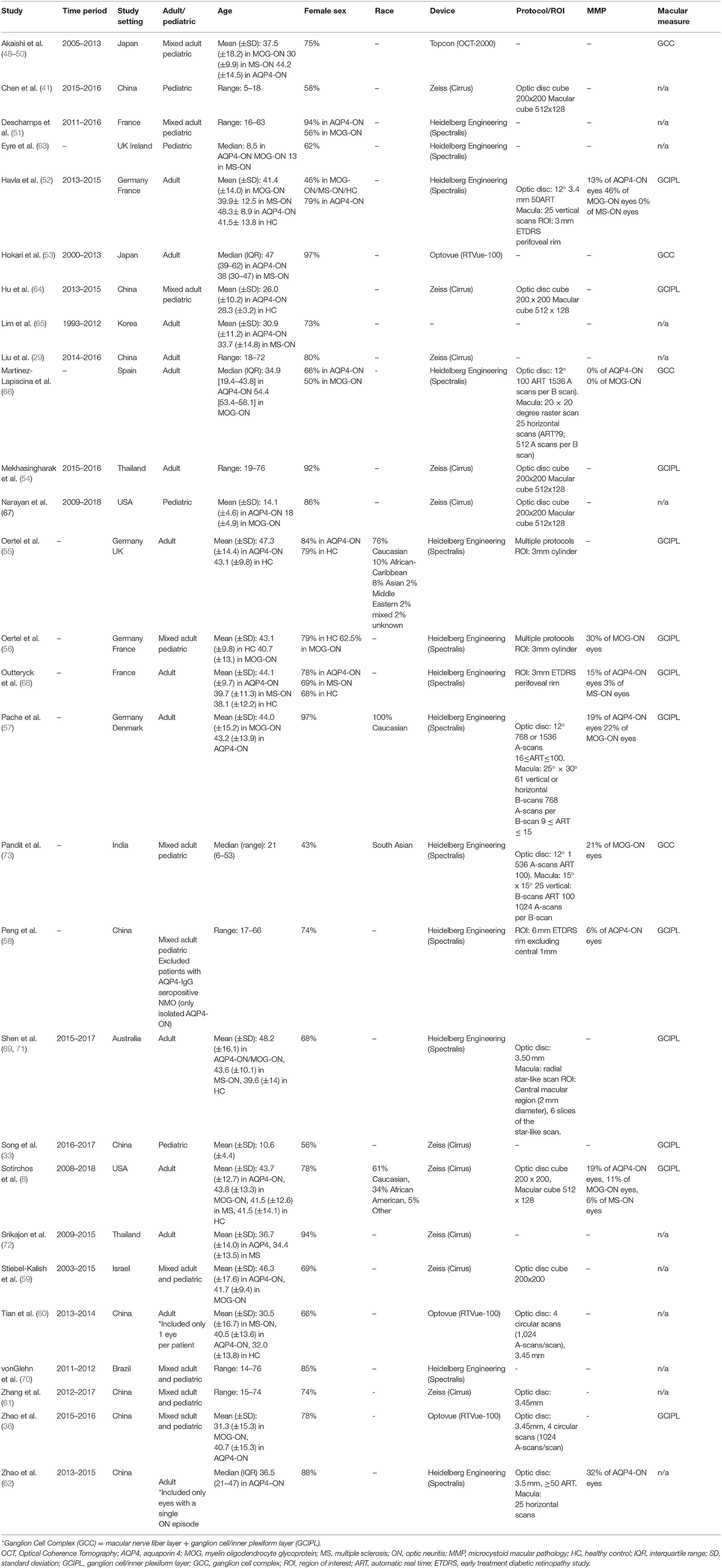
Table 2. Characteristics of studies included in the meta-analysis for our second study objective (comparison of OCT measures between AQP4-ON, MOG-ON, and MS-ON eyes).
OCT Measures in Adult ON
As expected, pRNFL and GCIPL thicknesses were lower in AQP4-ON and MOG-ON eyes, as compared with HC eyes (Supplementary Figures 3, 4). The pooled mean pRNFL difference for AQP4-ON eyes was −38.0 μm (95% CI: −46.5 to −29.6 μm) and −35.7 μm (95% CI: −43.1 to −28.4 μm) for MOG-ON eyes. The pooled mean GCIPL difference was −25.8 μm (95% CI: −29.1 to −22.5 μm) for AQP4-ON eyes and −26.7 μm (95% CI: −32.6 to −20.8 μm) for MOG-ON eyes.
AQP4-ON eyes had lower pRNFL (−11.7 μm; 95% CI: −15.2 to −8.3 μm) and GCIPL (−9.0 μm; 95% CI: −12.5 to −5.4 μm) thicknesses compared with MS-ON (Figure 7), but there were no differences in these OCT measures between AQP4-ON and MOG-ON eyes (pRNFL: −1.9 μm; 95% CI: −9.1 to 5.4 μm; GCIPL: −2.6 μm; 95% CI: −8.9 to 3.8 μm; Figure 8). Similar to AQP4-ON, when comparing MOG-ON to MS-ON eyes (Figure 9), we found that MOG-ON eyes had lower pRNFL (−11.2 μm; 95% CI: −21.5 to −0.9 μm) and GCIPL thicknesses (−6.1 μm; 95% CI −10.8 to −1.3 μm).
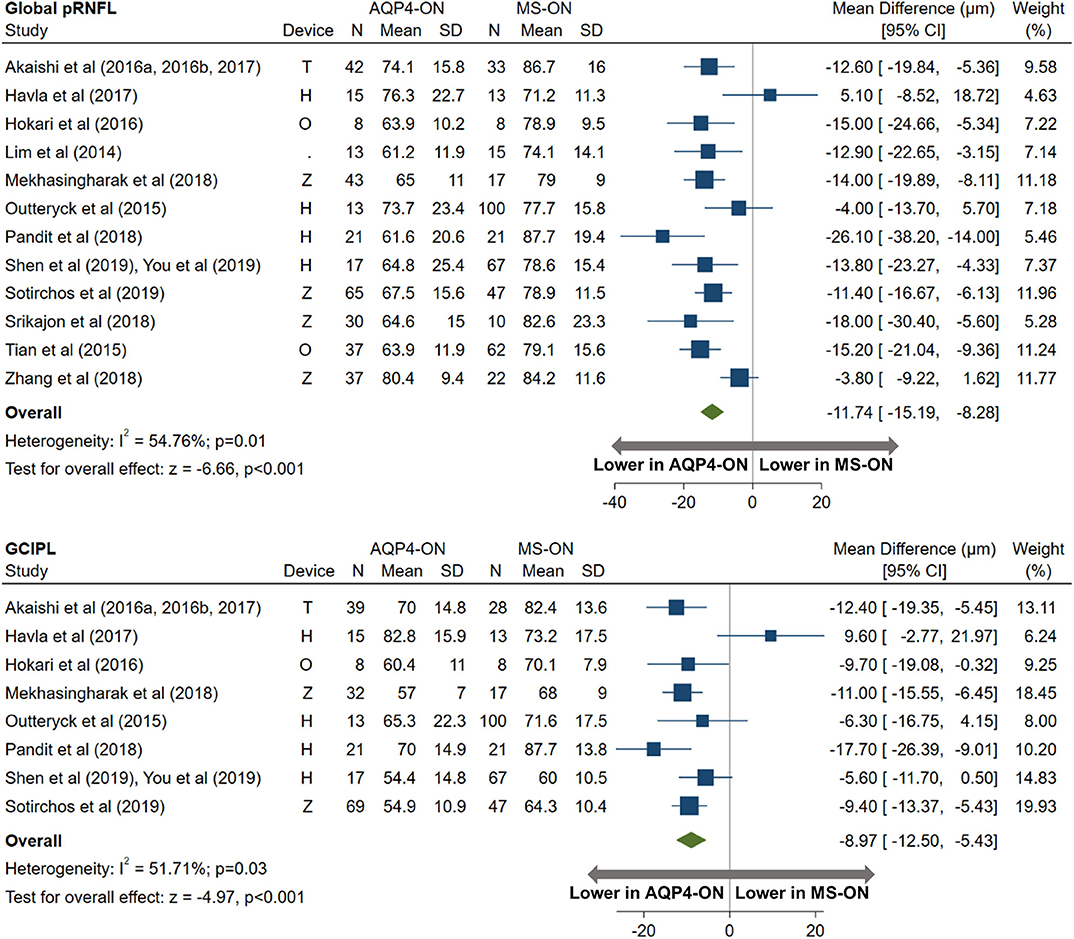
Figure 7. Forest plot of the mean difference in global pRNFL and GCIPL thickness between AQP4-ON and MS-ON. The SD-OCT devices used are indicated as H (Spectralis, Heidelberg Engineering; Heidelberg, Germany), O (RTVue, Optovue Inc; Fremont, CA, USA), and T (3D OCT-2000, Topcon Corporation; Tokyo, Japan), Z (Cirrus, Carl Zeiss Meditec; Dublin, CA, USA).
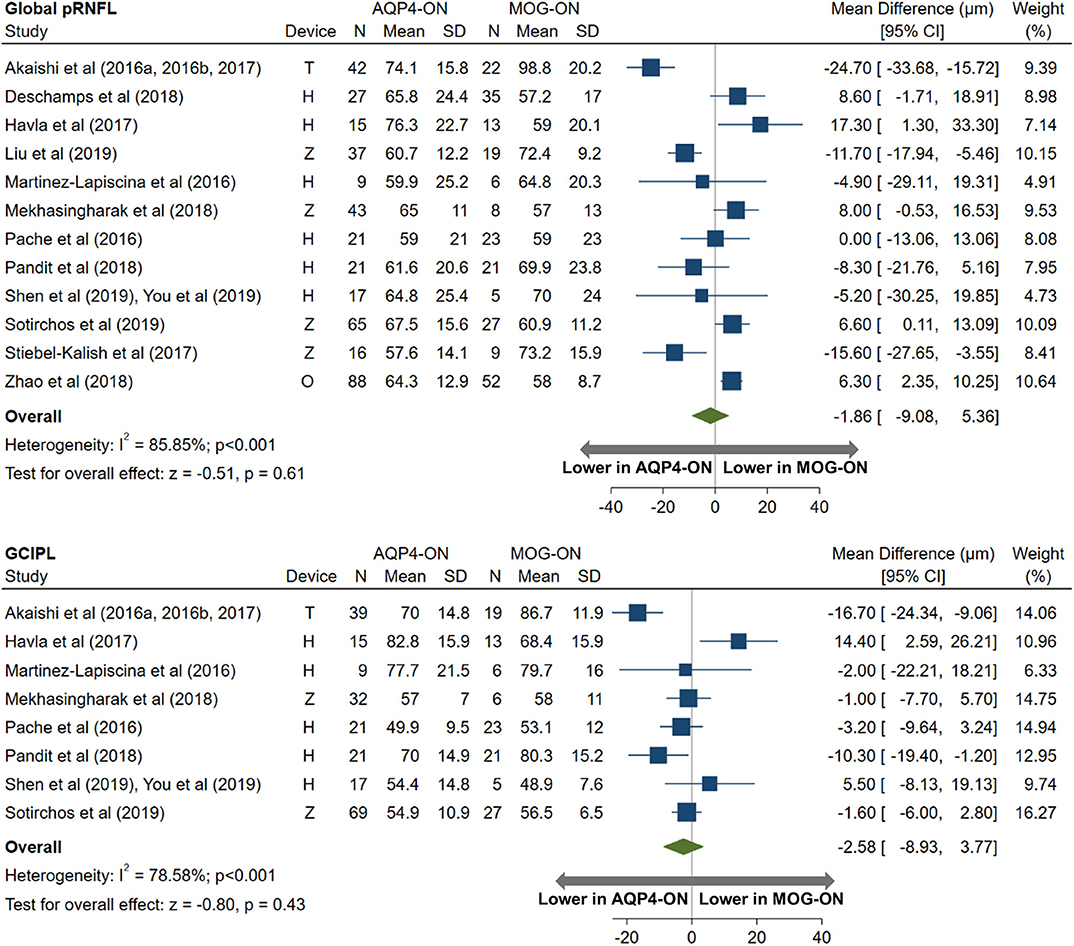
Figure 8. Forest plot of the mean difference in global pRNFL and GCIPL thickness between AQP4-ON and MOG-ON. The SD-OCT devices used are indicated as H (Spectralis, Heidelberg Engineering; Heidelberg, Germany), O (RTVue, Optovue Inc; Fremont, CA, USA), and T (3D OCT-2000, Topcon Corporation; Tokyo, Japan), Z (Cirrus, Carl Zeiss Meditec; Dublin, CA, USA).
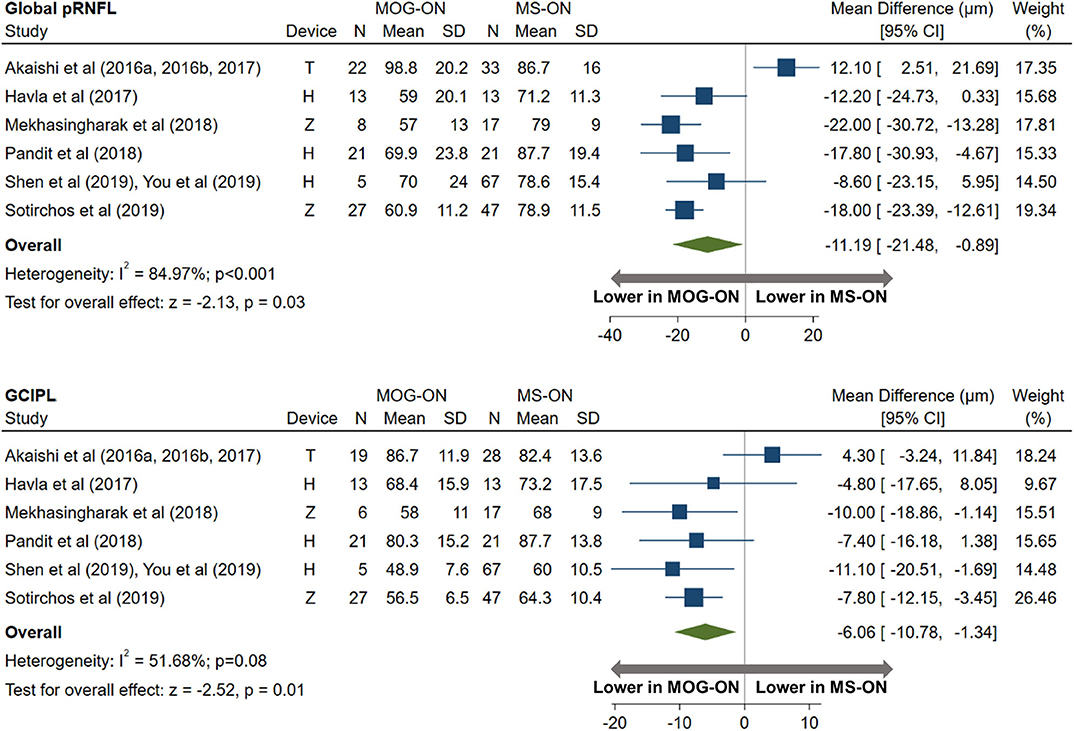
Figure 9. Forest plot of the mean difference in global pRNFL and GCIPL thickness between MOG-ON and MS-ON. The SD-OCT devices used are indicated as H (Spectralis, Heidelberg Engineering; Heidelberg, Germany), O (RTVue, Optovue Inc; Fremont, CA, USA), and T (3D OCT-2000, Topcon Corporation; Tokyo, Japan), Z (Cirrus, Carl Zeiss Meditec; Dublin, CA, USA).
When examining quadrantal pRNFL thicknesses, we did not observe any differences between AQP4-ON and MOG-ON (Supplementary Figure 5). However, AQP4-ON was associated with lower nasal, inferior, and superior quadrant pRNFL thicknesses compared with MS-ON (Supplementary Figure 6), but no difference was observed in temporal pRNFL thickness between AQP4-ON and MS-ON eyes (−1.4 μm, 95% CI: −5.9 to 3.1 μm). All quadrantal pRNFL thicknesses were lower in MOG-ON compared to MS-ON eyes (Supplementary Figure 7), but these findings did not achieve statistical significance, likely due to the small sample size.
The prevalence of MMP in ON eyes was reported in a small number of studies. The pooled prevalence of MMP was 15% in AQP4-ON eyes (95% CI: 7 to 24%; n = 7 studies) and 21% in MOG-ON eyes (95% CI: 11 to 32%; n = 6 studies), which is higher compared to the reported prevalence of MMP in MS-ON eyes (~6%) (16, 17).
OCT Measures in Pediatric ON
We were able to identify four studies reporting OCT findings in pediatric ON, and OCT measures could be pooled for three studies (33, 41, 67). Similar to adults, pRNFL thickness did not differ between pediatric AQP4-ON and MOG-ON eyes (7.4 μm, 95% CI: −17.1 to 32.0 μm; Supplementary Figure 8). Further comparisons between groups of interest were not possible based on the available data.
Visual Outcomes in AQP4-ON and MOG-ON
Study Selection and Study Characteristics
A PubMed search identified 624 records. Of these, 202 articles were selected and assessed for eligibility at the full-text level. After careful evaluation, 35 studies were included that met the inclusion criteria (8, 29, 30, 33, 36, 39, 41, 45, 47, 48, 51–54, 57, 59, 63, 65, 66, 68, 69, 71, 72, 74–85). The detailed flow chart is presented in Supplementary Figure 9.
The included studies with their baseline characteristics are summarized in Table 3. In our quantitative synthesis, we included 26 studies comprising 747 AQP4-ON eyes, 426 MOG-ON eyes, and 524 MS-ON eyes.
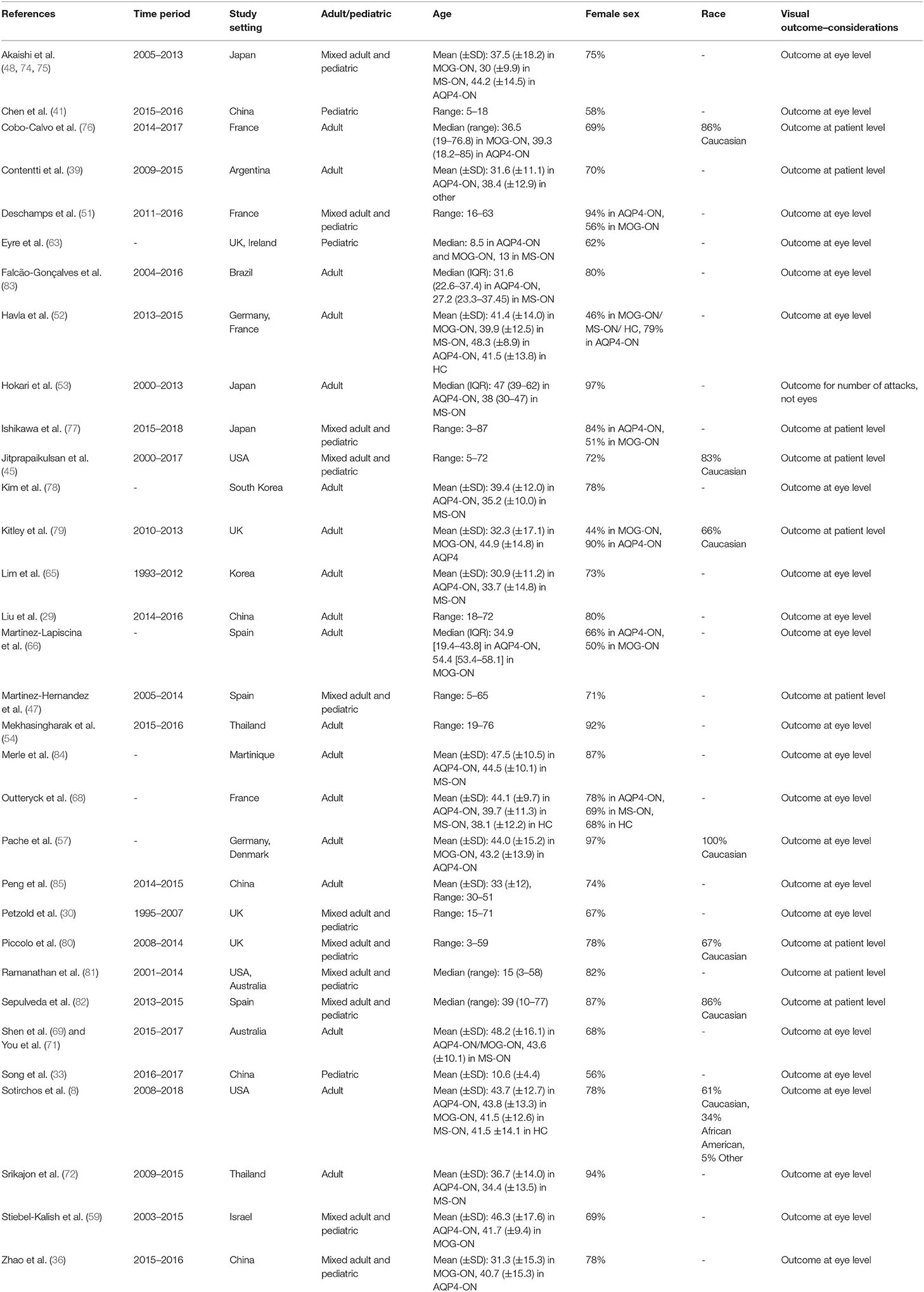
Table 3. Characteristics of studies included in the meta-analysis for our third study objective (comparison of visual outcomes in AQP4-ON, MOG-ON and MS-ON eyes).
Visual Outcomes in Adult ON
AQP4-ON eyes had worse high contrast VA when compared to both MOG-ON (mean logMAR difference: 0.60, 95% CI: 0.39 to 0.81) and MS-ON (mean logMAR difference: 0.68, 95% CI: 0.40 to 0.96; Figures 10, 11). Visual outcomes did not differ between MOG-ON and MS-ON (mean logMAR difference: 0.04, 95% CI: −0.05 to 0.14; Figure 12). Moreover, the risk of a poor visual outcome (VA ≤ 20/200) was higher for AQP4-ON compared to MOG-ON [relative risk (RR): 5.39, 95% CI: 2.95 to 9.86; Figure 10] and compared to MS-ON (RR: 3.76, 95% CI: 1.71 to 8.25; Figure 11).
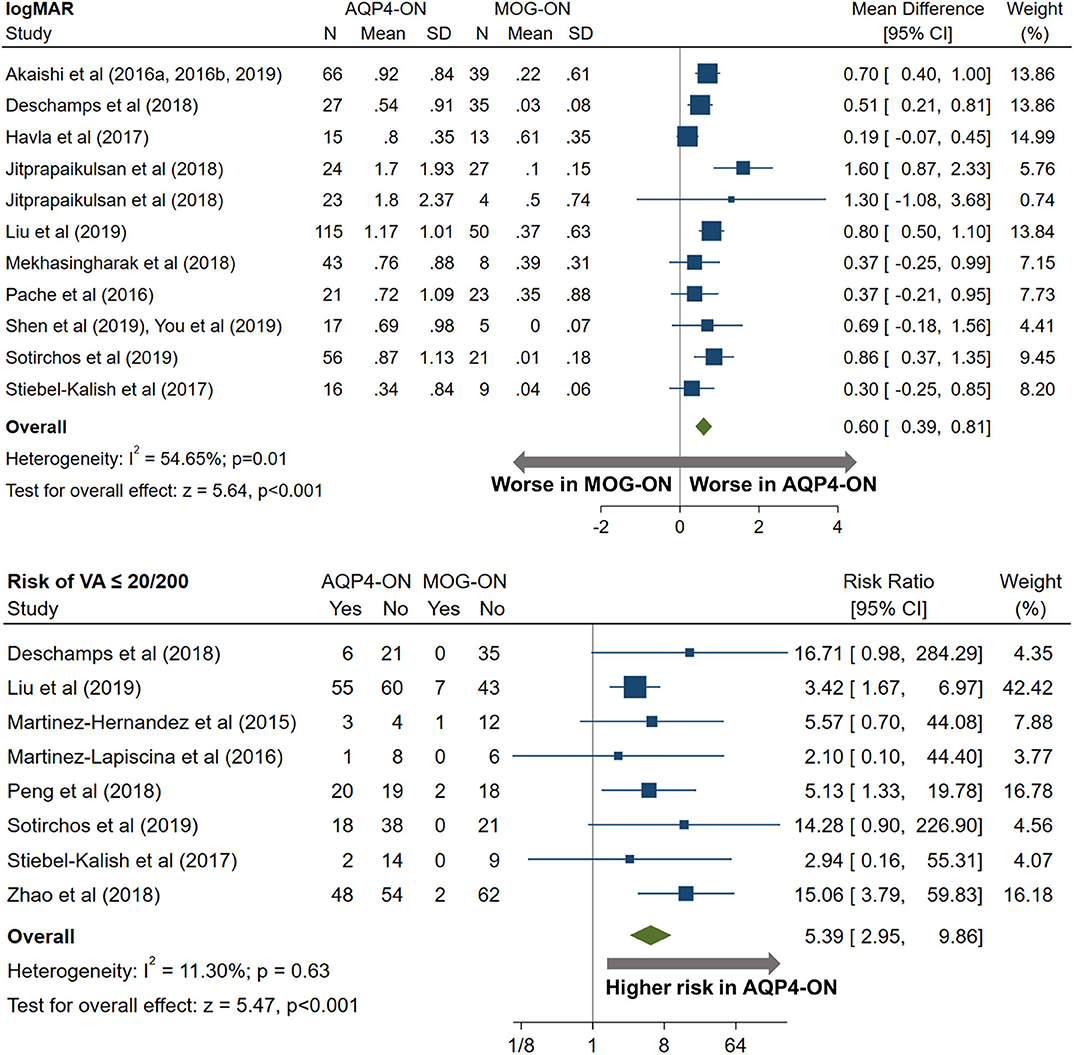
Figure 10. Forest plot of the mean difference in logMAR (high-contrast visual acuity) between AQP4-ON and MOG-ON; forest plot of the relative risk of a poor visual outcome (VA worse than 20/200) in AQP4-ON vs MOG-ON.
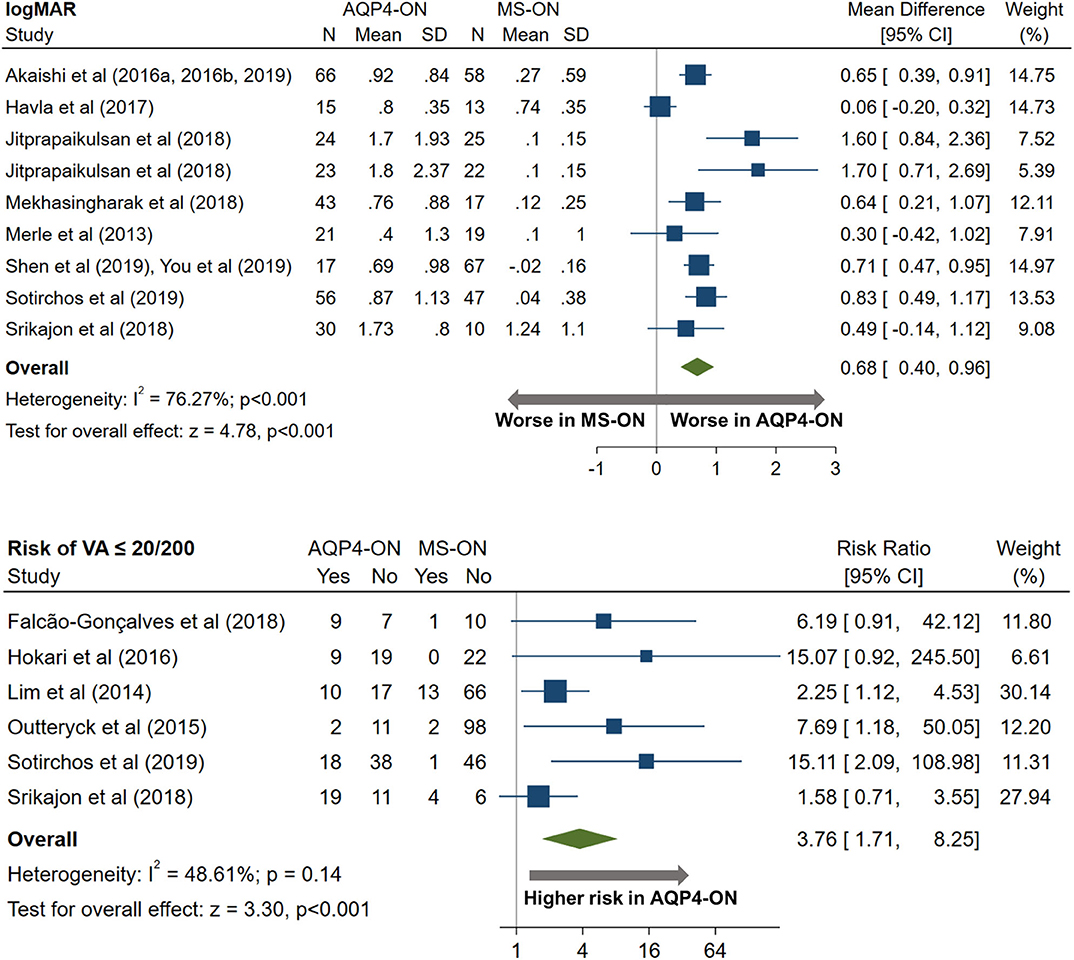
Figure 11. Forest plot of the mean difference in logMAR (high-contrast visual acuity) between AQP4-ON and MS-ON; forest plot of the relative risk of a poor visual outcome (VA worse than 20/200) in AQP4-ON vs MS-ON.
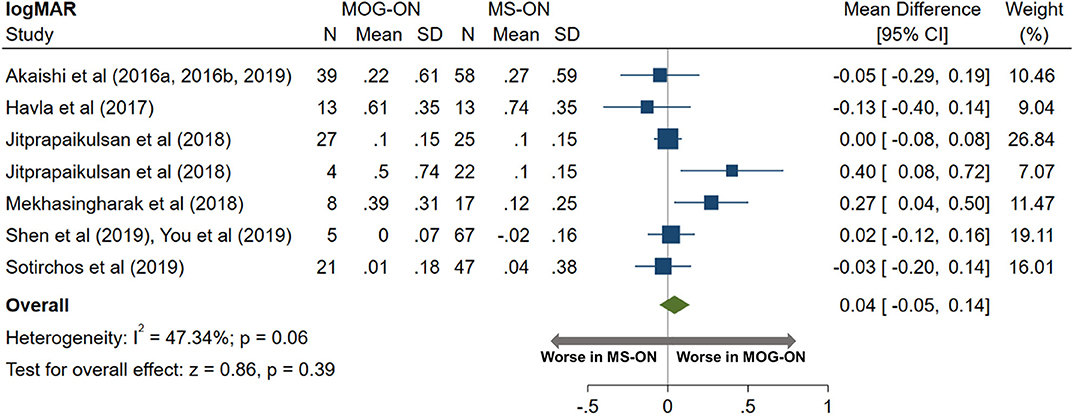
Figure 12. Forest plot of the mean difference in logMAR (high-contrast visual acuity) between MOG-ON and MS-ON.
Nine studies were excluded from our quantitative synthesis, since the visual outcomes were not presented in a format that was consistent with the other studies. The findings of the studies are presented in Supplementary Table 2. Importantly, all these studies reported that visual outcomes were markedly better in MOG-ON eyes, as compared with AQP4-ON eyes, in line with the results from the quantitative synthesis.
Visual Outcomes in Pediatric ON
We were able to identify three studies reporting visual outcomes in pediatric ON associated with seropositivity for AQP4-IgG and MOG-IgG (33, 41, 63). Similar to adults, the risk of a poor visual outcome (VA ≤ 20/200) was higher for AQP4-ON compared to MOG-ON (RR: 20.11, 95% CI: 4.79 to 84.34), but the sample sizes of the studies were rather small (Supplementary Figure 10).
Discussion
The present systematic review and meta-analysis revealed variable patterns of seroprevalence of AQP4-IgG and MOG-IgG among patients presenting with isolated ON, with overall higher seroprevalence of both antibodies among Asian populations. Moreover, MOG-IgG-associated ON accounted for a large proportion of pediatric isolated ON (over a third of cases), and high MOG-IgG seroprevalence was noted across the pediatric populations included in our study. Furthermore, despite a similar severity of GCIPL and pRNFL thinning in AQP4-ON and MOG-ON, AQP4-ON was associated with markedly worse visual outcomes, compared to both MOG-ON and MS-ON.
Overall, our results support the idea that AQP4-IgG- and MOG-IgG-associated disorders are not rare entities in Asian populations and are important diagnostic considerations during the initial evaluation of ON in these populations. Notably, cohorts from China comprised the vast majority of the Asian cohorts in our study. However, relatively high seroprevalence of AQP4-IgG and/or MOG-IgG in ON has been reported in several studies (that did not however fully fulfill our inclusion criteria) from Japan, Thailand, Malaysia, and additional Chinese centers (74, 77, 86–89). Importantly, while population-based studies support the notion that Eastern Asian populations have a higher prevalence of NMOSD compared to Caucasian populations, MOG-IgG-associated disease does not appear to exhibit a significant racial preponderance based on data from existing hospital-based studies (90). This suggests that our findings of high AQP4-IgG seroprevalence in ON in Asian populations are likely accounted for by both a higher prevalence of NMOSD and a lower prevalence of MS, whereas for MOG-IgG seroprevalence, the latter may be a more important factor. A noteworthy exception to our finding of overall lower seroprevalence of AQP4-IgG seropositivity in non-Asian populations was the study by Carnero-Contentti et al. (39), which enrolled patients from Buenos Aires, Argentina, and reported an AQP4-IgG seroprevalence of 30% among patients with ON (39). This finding is unexpected, given evidence supporting that the relative frequency of NMO vs. MS in Buenos Aires is low, and similar to that observed in Caucasian populations (91). Notably, this study did not report the ethnic/racial composition of the cohort, and it is possible that referral bias or other factors, which we were unable to detect on our review of the manuscript, contributed to this observation. While the frequency of AQP4-IgG and MOG-IgG seropositivity in ON appears to be lower in non-Asian populations, it remains crucial to consider these entities, especially in patients with atypical characteristics including recurrent or bilateral ON, longitudinally extensive optic nerve lesions, peri-neuritis (MOG-IgG), chiasmal/optic tract involvement (AQP4-IgG >> MOG-IgG), and/or poor visual recovery (AQP4-IgG) (6, 92). As expected, we found markedly higher seroprevalence of AQP4-IgG and MOG-IgG in recurrent isolated ON; however, the number of available studies was small, and mainly limited to non-Asian adult populations. A similar finding was expected in bilateral ON; however, there was an insufficient number of studies/participants eligible to systematically study this. Finally, in children with isolated ON, our results show that MOG-IgG is very commonly detected, across both Asian and non-Asian populations. However, AQP4-IgG seropositivity was exceedingly rare among non-Asian pediatric populations, but relatively common (15%) in Asian pediatric cohorts. The causes of these ethnic and age disparities are poorly understood, but it is likely that there is a genetic component, although environmental factors may also play a role (93, 94).
An important consideration is the fact that the included studies recruited very few patients of African ancestry. This is a critical point since NMOSD occurs frequently in individuals of African ancestry, and African-Americans/Europeans with NMOSD are more likely to experience severe attacks with poor recovery and appear to have higher mortality (95–97). Nevertheless, the frequency of AQP4-IgG and MOG-IgG seropositivity in isolated ON in these populations could not be investigated in the present meta-analysis.
Furthermore, we have found that AQP4-ON and MOG-ON eyes exhibited similarly severely decreased pRNFL and macular GCIPL thicknesses after ON, which was greater than that observed in MS-ON eyes. When examining quadrantal pRNFL thicknesses, we were unable to identify any quadrantal patterns of retinal injury that were specific to MOG-ON. However, when comparing AQP4-ON and MS-ON, AQP4-ON was associated with decreased inferior, superior, and nasal pRNFL thickness, but the temporal pRNFL did not appear to differ between the two groups. This finding suggests that the temporal pRNFL is relatively preserved in AQP4-ON or disproportionally affected in MS-ON. Temporal preponderance of pRNFL damage in MS-ON compared to AQP4-ON was also reported in a study by Schneider et al. (98), which, however, did not fulfill inclusion criteria for our meta-analysis. The pathophysiology underlying the observed differences is not clear; however, the arcuate fibers (located in the superior and inferior quadrants) are commonly injured in vascular optic neuropathies (99). This pattern of quadrantal thinning may suggest that vascular compromise is a mechanism of tissue injury in AQP4-ON. Notably, retinal vascular alterations have been reported in vivo in NMO and pathologic studies have identified prominent vascular fibrosis and hyalinization in NMO lesions (99, 100).
Interestingly, and in line with our prior observations (8), we found that, despite a similar severity of pRNFL and GCIPL thinning in AQP4-ON and MOG-ON, visual outcomes clearly diverged between these two entities, with MOG-ON eyes having relatively preserved visual acuity, whereas AQP4-ON eyes experienced markedly worse visual outcomes compared to both MOG-ON and MS-ON. The biological underpinnings of this observation remain unclear. AQP4-IgG-associated disease is recognized as an autoimmune astrocytopathy with secondary demyelination (101). In pathologic studies, a spectrum of changes in astrocytes has been described, including astrocyte necrosis and dystrophic astrocytic profiles (101). AQP4 is highly expressed in the retina, predominantly in retinal astrocytes and Müller glial cells; it is therefore conceivable that AQP4-IgG may cause direct retinal injury. Interestingly, foveal thinning and altered foveal morphology have been reported in AQP4-IgG seropositive eyes without a history of ON, suggesting that subclinical direct retinal involvement may occur in AQP4-IgG-associated disease (102–104). In a pathological study of human retinas, AQP4-IgG seropositivity was associated with loss of AQP4 immunoreactivity on Müller cells, while intravitreal AQP4-IgG injection in mice resulted in reduced AQP4 expression by Müller cells, reactive retinal gliosis and loss of RGCs (53, 105). Notably, AQP4 deletion renders Müller cells incapable of handling osmotic stress and may induce an inflammatory response in the retina (106). These findings suggest that the poor visual prognosis in AQP4-ON may be partially mediated by alterations in the dynamics of astrocyte and Müller cell function. MMP has also been proposed as a factor that is associated with poor outcomes following ON, since MMP eyes have worse visual outcomes and more severe GCIPL and pRNFL thinning (16–18). However, when accounting for GCIPL thickness and ON etiology, MMP does not appear to be independently associated with visual acuity, suggesting that MMP may represent a marker of optic neuropathy severity, rather than a direct contributor to visual dysfunction following ON (8). The prevalence of MMP was reported by a small number of studies included in our meta-analysis but appeared to be overall similar in AQP4-ON (15%) and MOG-ON (21%) and higher in both compared to the reported prevalence in MS-ON (~6%). Further work is needed to clarify the pathoetiology of MMP and whether MMP is causally associated with poor visual outcomes after ON.
Furthermore, we observed an impressive discordance between structural and functional outcomes in MOG-ON; even though MOG-ON was associated with severe pRNFL and GCIPL thinning, high-contrast visual acuity was remarkably preserved and did not differ from MS-ON. Contrary to AQP4, MOG is not expressed in the human retina; therefore, the observed inner retinal thinning is expected to be due to secondary change due to retrograde degeneration and not primary retinal pathology. The pathophysiology underlying the observed structure-function mismatch in MOG-ON is unclear; however, an important consideration is that, with OCT, we are not able to visualize the histological composition of each retinal layer. Therefore, it is conceivable that the relative contributions of the RGCs and their axons to GCIPL and RNFL thicknesses differ between AQP4-ON and MOG-ON, despite a similar severity of retinal layer thinning. In fact, the glial content of the RNFL is considerable and microglia constitute a significant component of the inner plexiform layer, whose thickness is measured as a composite with the ganglion cell layer as GCIPL (107, 108). Given the markedly different pathogenic mechanisms in these disorders, it is conceivable that the observed discrepancies may be related to differences in glial activation and migration, resulting in differing compositions of the pRNFL and the GCIPL and, consequently, different functional capacity of the retina. Another important consideration is that there is a floor effect present for OCT measures, and a single AQP4-ON or MOG-ON attack can lead to marked pRNFL and GCIPL atrophy, while subsequent attacks may not lead to appreciable changes in inner retinal layer thicknesses, despite worsening visual function (109). Analyses comparing visual and structural measures between groups after a single attack of ON would be useful to address this issue; however, the vast majority of studies included in our meta-analysis did not report OCT or visual acuity separately for patients with single and recurrent ON. However, since both AQP4-ON and MOG-ON frequently relapse, we do not expect that this may have significantly affected our findings when comparing outcomes in AQP4-ON vs. MOG-ON, although this may have influenced comparisons with MS-ON (6).
In this meta-analysis, we also attempted to examine OCT findings and visual outcomes in pediatric ON associated with AQP4-IgG and MOG-IgG seropositivity. However, this population has not been studied extensively and a systematic review of the literature revealed only four studies, with small numbers of participants (33, 41, 63, 67). OCT measures could be pooled for three of these, two of which included Asian children (33, 41). Therefore, our meta-analysis is clearly underpowered to study characteristics of pediatric AQP4-ON and MOG-ON. Nevertheless, the OCT findings and visual outcomes appear to be similar to those observed in adults. The inclusion of pediatric cases should be an important consideration for future studies, especially since MOG-IgG antibodies are commonly detected in children with ON.
Despite the strengths of the present report, several limitations must be acknowledged. Firstly, the majority of the included prevalence studies were performed at tertiary academic referral centers, with clinical expertise in neuro-ophthalmology. Therefore, it is conceivable that the patients who were recruited in these studies are not a representative sample of patients presenting with isolated ON and are likely enriched for cases with increased severity or atypical characteristics. Thus, it is possible that our results may overestimate the true prevalence rate of these disorders in the general population due to referral bias. This issue should also be considered when interpreting the OCT and visual outcomes, since patients with more severe attacks of ON and poor recovery are potentially more likely to be referred to a tertiary center for further management, and mild cases with favorable outcomes may be underrepresented in the existing literature. Furthermore, between-study heterogeneity was considerable in almost all pooled analyses of OCT measures or visual outcomes. A potential source of heterogeneity in analyses of OCT measures is the fact that the included studies utilized a variety of spectral-domain OCT devices, as well as scanning and segmentation protocols. Moreover, participants' demographics and clinical characteristics varied considerably between studies and it is likely that there is variability in the phenotype, disease course, and outcomes among different racial or age groups. To minimize the impact of these differences on our results, we did not compare OCT measures or visual outcomes across studies; rather, we estimated the differences in retinal layer thicknesses or logMAR between groups that were included in the same study and performed a pooled analysis of these estimated differences. In analyses of OCT measures and visual outcomes, we were also notably unable to account for the number of ON attacks, since some studies included patients with a single event, while others recruited patients with multiple ON episodes. It is expected that the number of ON episodes has an impact on OCT findings and final visual acuity, especially since recurrent ON in common in cases of AQP4-ON and MOG-ON; this should be a consideration in future studies. Additionally, even though we attempted to analyze findings in adult ON separately from pediatric ON, some studies (noted in Tables 1–3) recruited mixed adult and pediatric or adolescent populations; this is an important consideration when attempting to draw conclusions regarding potential differences in the characteristics of these disease entities between these age groups. Finally, AQP4-IgG serostatus was determined using a variety of assays, including ELISA in some studies, which is known to have an inferior performance in terms of sensitivity and specificity compared to CBAs (110, 111). This is a relevant point, since the use of an assay with sub-optimal diagnostic accuracy may have led to misclassification of patients. Nevertheless, the majority of studies included in our meta-analysis (including 79% of studies assessing the prevalence of AQP4-IgG in ON) utilized CBA to determine the AQP4-IgG serostatus of their participants. MOG-IgG serostatus was determined exclusively using CBAs with full-length human MOG, given that MOG-IgG detected by ELISA or Western blot lacks disease specificity. Notably, commonly used MOG-IgG CBAs demonstrate overall good agreement for high-positive and negative samples, although agreement is lower for borderline results, and this is another factor that could potentially influence diagnostic accuracy in the included studies (112).
Conclusions
Our systematic review and meta-analysis provides a comprehensive overview of the epidemiology and structural and functional outcomes in ON associated with AQP4-IgG and MOG-IgG seropositivity. Our findings support the idea that AQP4-IgG- and MOG-IgG-related disease are more common causes of ON in Asian vs. non-Asian populations and that MOG-IgG seroprevalence is especially high in pediatric ON, and we provide estimates of seroprevalence in these groups. We have also shown that MOG-ON and AQP4-ON are associated with similar severity of retinal thinning; however, visual outcomes appear to be markedly worse in AQP4-ON. Future studies should seek to investigate the pathoetiology of these findings, as well as to provide insights regarding optimal acute and chronic treatment strategies for these disorders.
Data Availability Statement
The datasets generated for this study are available on request to the corresponding author.
Author Contributions
AF and ES: study conception and design, data acquisition, analysis and interpretation, drafting, and revision of the manuscript for important intellectual content. LM: data acquisition and interpretation and revision of the manuscript for important intellectual content. SS and PC: data interpretation and revision of the manuscript for important intellectual content. All authors contributed to the article and approved the submitted version.
Funding
This study was funded by the National MS Society (RG-1606-08768 to SS; FP-1607-24999 to ES), NIH/NINDS (R01NS082347 to PC), and the Caring Friends NMO Research fund.
Conflict of Interest
SS had received consulting fees from Medical Logix for the development of CME programs in neurology and had served on scientific advisory boards for Biogen, Genzyme, Genentech Corporation, EMD Serono, and Celgene. SS was the PI of investigator-initiated studies funded by Genentech Corporation and Biogen and received support from the Race to Erase MS foundation. SS had received equity compensation for consulting from JuneBrain LLC, a retinal imaging device developer. SS was also the site investigator of a trial sponsored by MedDay Pharmaceuticals. PC had received consulting fees from Disarm Therapeutics and Biogen and was PI on grants to JHU from Biogen and Annexon. ES had served on scientific advisory boards for Viela Bio and Genentech.
The remaining authors declare that the research was conducted in the absence of any commercial or financial relationships that could be construed as a potential conflict of interest.
The handling Editor declared a past collaboration with the authors.
Acknowledgments
We would like to acknowledge all corresponding authors who responded to our communication and provided additional data and/or clarifications regarding their manuscripts, and in particular Drs. Tetsuya Akaishi, Romain Deschamps, Alessandra Billi Falcão-Gonçalves, Joachim Havla, Pei-Rong Lu, Young-Min Lim, Kwang-Kuk Kim, Elena Martinez-Lapiscina, Harold Merle, Oliver Outteryck, Felipe vonGlehn, Clarissa Lin Yasuda, Shi Hui Wei, Chunxia Peng, Yuyi You, Sasithorn Siritho, John J. Chen, Takeshi Kezuka, Romain Marignier, Lekha Pandit, and Chaithra Malli.
Supplementary Material
The Supplementary Material for this article can be found online at: https://www.frontiersin.org/articles/10.3389/fneur.2020.540156/full#supplementary-material
Supplementary Figure 1. Study selection for our first study objective (assessing the prevalence of AQP4-IgG and MOG-IgG seropositivity in isolated ON).
Supplementary Figure 2. Study selection for our second study objective (comparison of OCT measures between AQP4-ON, MOG-ON and MS-ON eyes).
Supplementary Figure 3. Forest plot of the mean difference in global pRNFL and GCIPL thickness between AQP4-ON and HC.
Supplementary Figure 4. Forest plot of the mean difference in global pRNFL and GCIPL thickness between MOG-ON and HC.
Supplementary Figure 5. Forest plot of the mean difference in quadrantal pRNFL thicknesses between AQP4-ON and MOG-ON.
Supplementary Figure 6. Forest plot of the mean difference in quadrantal pRNFL thicknesses between AQP4-ON and MS-ON.
Supplementary Figure 7. Forest plot of the mean difference in quadrantal pRNFL thicknesses between MOG-ON and MS-ON.
Supplementary Figure 8. Forest plot of the mean difference in global pRNFL thickness between AQP4-ON and MOG-ON in pediatric ON.
Supplementary Figure 9. Study selection for our third study objective (assessment of the visual outcome in AQP4-ON, MOG-ON and MS-ON eyes).
Supplementary Figure 10. Forest plot of the relative risk of a poor visual outcome (VA worse than 20/200) in AQP4-ON vs MOG-ON in pediatric ON.
Supplementary Table 1. Search terms.
Supplementary Table 2. Visual outcome in AQP4-ON, MOG-ON and MS-ON; qualitative synthesis.
Abbreviations
AQP4, aquaporin 4; MOG, myelin oligodendrocyte glycoprotein; MS, multiple sclerosis; ON, optic neuritis; pRNFL, peripapillary nerve fiber layer; GCIPL, ganglion cell/inner plexiform layer; VA, visual acuity; OCT, optical coherence tomography; logMAR, logarithm of the minimum angle of resolution; N, number of eyes; SD, standard deviation; CI, confidence interval.
References
1. Toosy AT, Mason DF, Miller DH. Optic neuritis. Lancet Neurol. (2014) 13:83–99. doi: 10.1016/S1474-4422(13)70259-X
2. Petzold A, Wattjes MP, Costello F, Flores-Rivera J, Fraser CL, Fujihara K, et al. The investigation of acute optic neuritis: a review and proposed protocol. Nat Rev Neurol. (2014) 10:447–58. doi: 10.1038/nrneurol.2014.108
3. Reindl M, Waters P. Myelin oligodendrocyte glycoprotein antibodies in neurological disease. Nat Rev Neurol. (2019) 15:89–102. doi: 10.1038/s41582-018-0112-x
4. Jarius S, Paul F, Aktas O, Asgari N, Dale RC, de Seze J, et al. MOG encephalomyelitis: international recommendations on diagnosis and antibody testing. J Neuroinflammation. (2018) 15:134. doi: 10.1186/s12974-018-1144-2
5. Borisow N, Mori M, Kuwabara S, Scheel M, Paul F. Diagnosis and Treatment of NMO Spectrum Disorder and MOG-Encephalomyelitis. Front Neurol. (2018) 9:888. doi: 10.3389/fneur.2018.00888
6. Chen JJ, Flanagan EP, Jitprapaikulsan J, López-Chiriboga ASS, Fryer JP, Leavitt JA, et al. Myelin Oligodendrocyte glycoprotein antibody-positive optic neuritis: clinical characteristics, radiologic clues, and outcome. Am J Ophthalmol. (2018) 195:8–15. doi: 10.1016/j.ajo.2018.07.020
7. Sato DK, Callegaro D, Lana-Peixoto MA, Waters PJ, de Haidar Jorge, Frederico M, Takahashi T, et al. Distinction between MOG antibody-positive and AQP4 antibody-positive NMO spectrum disorders. Neurology. (2014) 82:474–81. doi: 10.1212/WNL.0000000000000101
8. Sotirchos ES, Filippatou A, Fitzgerald KC, Salama S, Pardo S, Wang J, et al. Aquaporin-4 IgG seropositivity is associated with worse visual outcomes after optic neuritis than MOG-IgG seropositivity and multiple sclerosis, independent of macular ganglion cell layer thinning. Mult Scler. (2019). doi: 10.1177/1352458519864928. [Epub ahead of print].
9. Flanagan EP, Cabre P, Weinshenker BG, Sauver JS, Jacobson DJ, Majed M, et al. Epidemiology of aquaporin-4 autoimmunity and neuromyelitis optica spectrum. Ann Neurol. (2016) 79:775–83. doi: 10.1002/ana.24617
10. Kim S, Kim HJ. Central nervous system neuroinflammatory disorders in Asian/Pacific regions. Curr Opin Neurol. (2016) 29:372–80. doi: 10.1097/WCO.0000000000000315
11. Huang D, Swanson EA, Lin CP, Schuman JS, Stinson WG, Chang W, et al. Optical coherence tomography. Science. (1991) 254:1178–81. doi: 10.1126/science.1957169
12. Britze J, Frederiksen JL. Optical coherence tomography in multiple sclerosis. Eye. (2018) 32:884–8. doi: 10.1038/s41433-017-0010-2
13. Liberati A, Altman DG, Tetzlaff J, Mulrow C, Gøtzsche PC, Ioannidis JPA, et al. The PRISMA statement for reporting systematic reviews and meta-analyses of studies that evaluate health care interventions: explanation and elaboration. J Clin Epidemiol. (2009) 62:1. doi: 10.1016/j.jclinepi.2009.06.006
14. Stroup DF, Berlin JA, Morton SC, Olkin I, Williamson GD, Rennie D, et al. Meta-analysis of observational studies in epidemiology: a proposal for reporting. Meta-analysis of observational studies in epidemiology (MOOSE) group. JAMA. (2000) 283:2008–12. doi: 10.1001/jama.283.15.2008
15. Petzold A, Balcer L, Balcer LJ, Calabresi PA, Calabresi P, Costello F, et al. Retinal layer segmentation in multiple sclerosis: a systematic review and meta-analysis. Lancet Neurol. (2017) 16:797–812. doi: 10.1016/S1474-4422(17)30278-8
16. Gelfand JM, Nolan R, Schwartz DM, Graves J, Green AJ. Microcystic macular oedema in multiple sclerosis is associated with disease severity. Brain. (2012) 135:1786–93. doi: 10.1093/brain/aws098
17. Saidha S, Sotirchos ES, Ibrahim MA, Crainiceanu CM, Gelfand JM, Sepah YJ, et al. Microcystic macular oedema, thickness of the inner nuclear layer of the retina, and disease characteristics in multiple sclerosis: a retrospective study. Lancet Neurol. (2012) 11:963–72. doi: 10.1016/S1474-4422(12)70213-2
18. Sotirchos ES, Saidha S, Byraiah G, Mealy MA, Ibrahim MA, Sepah YJ, et al. In vivo identification of morphologic retinal abnormalities in neuromyelitis optica. Neurology. (2013) 80:1406–14. doi: 10.1212/WNL.0b013e31828c2f7a
19. Freeman MF, Tukey JW. Transformations related to the angular and the square root. Ann Math Stat. (1950). doi: 10.1214/aoms/1177729756
20. Warner CV, Syc SB, Stankiewicz AM, Hiremath G, Farrell SK, Crainiceanu CM, et al. The impact of utilizing different optical coherence tomography devices for clinical purposes and in multiple sclerosis trials. PLoS ONE. (2011) 6:e22947. doi: 10.1371/journal.pone.0022947
21. Nyaga VN, Arbyn M, Aerts M. Metaprop: a Stata command to perform meta-analysis of binomial data. Arch Public Health. (2014) 72:39. doi: 10.1186/2049-3258-72-39
22. Cobo-Calvo Á, Ruiz A, D'Indy H, Poulat A, Carneiro M, Philippe N, et al. MOG antibody-related disorders: common features and uncommon presentations. J Neurol. (2017) 264:1945–55. doi: 10.1007/s00415-017-8583-z
23. Dale RC, Tantsis EM, Merheb V, Kumaran RA, Sinmaz N, Pathmanandavel K, et al. Antibodies to MOG have a demyelination phenotype and affect oligodendrocyte cytoskeleton. Neurol Neuroimmunol Neuroinflamm. (2014) 1:e12. doi: 10.1212/NXI.0000000000000012
24. Ducloyer J, Caignard A, Aidaoui R, Ollivier Y, Plubeau G, Santos-Moskalyk S, et al. MOG-Ab prevalence in optic neuritis and clinical predictive factors for diagnosis. Br J Ophthalmol. (2020) 104:842–5. doi: 10.1136/bjophthalmol-2019-314845
25. Hacohen Y, Absoud M, Deiva K, Hemingway C, Nytrova P, Woodhall M, et al. Myelin oligodendrocyte glycoprotein antibodies are associated with a non-MS course in children. Neurol Neuroimmunol Neuroinflamm. (2015) 2:e81. doi: 10.1212/NXI.0000000000000081
26. Jarius S, Frederikson J, Waters P, Paul F, Akman-Demir G, Marignier R, et al. Frequency and prognostic impact of antibodies to aquaporin-4 in patients with optic neuritis. J Neurol Sci. (2010) 298:158–62. doi: 10.1016/j.jns.2010.07.011
27. Kim H, Park KA, Oh SY, Min JH, Kim BJ. Association of optic neuritis with neuromyelitis optica spectrum disorder and multiple sclerosis in Korea. Korean J Ophthalmol. (2019) 33:82–90. doi: 10.3341/kjo.2018.0050
28. Kim KM, Kim US. Aquaporin 4 antibody in the Korean patient with new-onset optic neuritis. Semin Ophthalmol. (2018) 33:215–8. doi: 10.1080/08820538.2016.1208761
29. Liu H, Zhou H, Wang J, Sun M, Teng D, Song H, et al. The prevalence and prognostic value of myelin oligodendrocyte glycoprotein antibody in adult optic neuritis. J Neurol Sci. (2019) 396:225–31. doi: 10.1016/j.jns.2018.11.029
30. Petzold A, Woodhall M, Khaleeli Z, Tobin WO, Pittock SJ, Weinshenker BG, et al. Aquaporin-4 and myelin oligodendrocyte glycoprotein antibodies in immune-mediated optic neuritis at long-term follow-up. J Neurol Neurosurg Psychiatry. (2019) 90:1021–6. doi: 10.1136/jnnp-2019-320493
31. Rostasy K, Mader S, Schanda K, Huppke P, Gärtner J, Kraus V, et al. Anti-myelin oligodendrocyte glycoprotein antibodies in pediatric patients with optic neuritis. Arch Neurol. (2012) 69:752–6. doi: 10.1001/archneurol.2011.2956
32. Soelberg K, Jarius S, Skejoe H, Engberg H, Mehlsen JJ, Nilsson AC, et al. A population-based prospective study of optic neuritis. Mult Scler. (2017) 23:1893–901. doi: 10.1177/1352458517734070
33. Song H, Zhou H, Yang M, Tan S, Wang J, Xu Q, et al. Clinical characteristics and prognosis of myelin oligodendrocyte glycoprotein antibody-seropositive paediatric optic neuritis in China. Br J Ophthalmol. (2019) 103:831–6. doi: 10.1136/bjophthalmol-2018-312399
34. Storoni M, Pittock SJ, Weinshenker BG, Plant GT. Optic neuritis in an ethnically diverse population: higher risk of atypical cases in patients of African or African-Caribbean heritage. J Neurol Sci. (2012) 312:21–5. doi: 10.1016/j.jns.2011.08.030
35. Waters P, Fadda G, Woodhall M, O'Mahony J, Brown RA, Castro DA, et al. Serial anti-myelin oligodendrocyte glycoprotein antibody analyses and outcomes in children with demyelinating syndromes. JAMA Neurol. (2019) 77:82–93. doi: 10.1001/jamaneurol.2019.2940
36. Zhao G, Chen Q, Huang Y, Li Z, Sun X, Lu P, et al. Clinical characteristics of myelin oligodendrocyte glycoprotein seropositive optic neuritis: a cohort study in Shanghai, China. J Neurol. (2018) 265:33–40. doi: 10.1007/s00415-017-8651-4
37. Zhou H, Zhao S, Yin D, Chen X, Xu Q, Chen T, et al. Optic neuritis: a 5-year follow-up study of Chinese patients based on aquaporin-4 antibody status and ages. J Neurol. (2016) 263:1382–9. doi: 10.1007/s00415-016-8155-7
38. Zhou H, Xu Q, Zhao S, Wang W, Wang J, Chen Z, et al. Distinct clinical characteristics of atypical optic neuritis with seronegative aquaporin-4 antibody among Chinese patients. Br J Ophthalmol. (2017) 101:1720–4. doi: 10.1136/bjophthalmol-2017-310157
39. Carnero Contentti E, De Virgiliis M, Hryb JP, Gomez A, Morales S, Celso J, et al. Aquaporin-4 serostatus and visual outcomes in clinically isolated acute optic neuritis. J Neuroophthalmol. (2019) 39:165–9. doi: 10.1097/WNO.0000000000000668
40. Chen JJ, Tobin WO, Majed M, Jitprapaikulsan J, Fryer JP, Leavitt JA, et al. Prevalence of myelin oligodendrocyte glycoprotein and aquaporin-4-IgG in patients in the optic neuritis treatment trial. JAMA Ophthalmol. (2018) 136:419–22. doi: 10.1001/jamaophthalmol.2017.6757
41. Chen Q, Zhao G, Huang Y, Li Z, Sun X, Lu P, et al. Clinical characteristics of pediatric optic neuritis with myelin oligodendrocyte glycoprotein seropositive: a cohort study. Pediatr Neurol. (2018) 83:42–9. doi: 10.1016/j.pediatrneurol.2018.03.003
42. Deschamps R, Lecler A, Lamirel C, Aboab J, Gueguen A, Bensa C, et al. Etiologies of acute demyelinating optic neuritis: an observational study of 110 patients. Eur J Neurol. (2017) 24:875–9. doi: 10.1111/ene.13315
43. Benoilid A, Tilikete C, Collongues N, Arndt C, Vighetto A, Vignal C, et al. Relapsing optic neuritis: a multicentre study of 62 patients. Mult Scler. (2014) 20:848–53. doi: 10.1177/1352458513510223
44. de Seze J, Arndt C, Jeanjean L, Zephir H, Blanc F, Labauge P, et al. Relapsing inflammatory optic neuritis: is it neuromyelitis optica? Neurology. (2008) 70:2075–6. doi: 10.1212/01.wnl.0000313375.20123.25
45. Jitprapaikulsan J, Chen JJ, Flanagan EP, Tobin WO, Fryer JP, Weinshenker BG, et al. Aquaporin-4 and myelin oligodendrocyte glycoprotein autoantibody status predict outcome of recurrent optic neuritis. Ophthalmology. (2018) 125:1628–37. doi: 10.1016/j.ophtha.2018.03.041
46. Li H, Wang Y, Xu Q, Zhang A, Zhou H, Zhao S, et al. Features of anti-aquaporin 4 antibody-seropositive Chinese patients with neuromyelitis optica spectrum optic neuritis. J Neurol. (2015) 262:2293–304. doi: 10.1007/s00415-015-7844-y
47. Martinez-Hernandez E, Sepulveda M, Rostásy K, Höftberger R, Graus F, Harvey RJ, et al. Antibodies to aquaporin 4, myelin-oligodendrocyte glycoprotein, and the glycine receptor α1 subunit in patients with isolated optic neuritis. JAMA Neurol. (2015) 72:187–93. doi: 10.1001/jamaneurol.2014.3602
48. Akaishi T, Nakashima I, Takeshita T, Mugikura S, Sato DK, Takahashi T, et al. Lesion length of optic neuritis impacts visual prognosis in neuromyelitis optica. J Neuroimmunol. (2016) 293:28–33. doi: 10.1016/j.jneuroim.2016.02.004
49. Akaishi T, Sato DK, Nakashima I, Takeshita T, Takahashi T, Doi H, et al. MRI and retinal abnormalities in isolated optic neuritis with myelin oligodendrocyte glycoprotein and aquaporin-4 antibodies: a comparative study. J Neurol Neurosurg Psychiatry. (2016) 87:446–8. doi: 10.1136/jnnp-2014-310206
50. Akaishi T, Kaneko K, Himori N, Takeshita T, Takahashi T, Nakazawa T, et al. Subclinical retinal atrophy in the unaffected fellow eyes of multiple sclerosis and neuromyelitis optica. J Neuroimmunol. (2017) 313:10–5. doi: 10.1016/j.jneuroim.2017.10.001
51. Deschamps R, Gueguen A, Lecler A, Lecouturier K, Lamirel C, Bensa C, et al. Acute idiopathic optic neuritis: not always benign. Eur J Neurol. (2018) 25:1378–83. doi: 10.1111/ene.13753
52. Havla J, Kümpfel T, Schinner R, Spadaro M, Schuh E, Meinl E, et al. Myelin-oligodendrocyte-glycoprotein (MOG) autoantibodies as potential markers of severe optic neuritis and subclinical retinal axonal degeneration. J Neurol. (2017) 264:139–51. doi: 10.1007/s00415-016-8333-7
53. Hokari M, Yokoseki A, Arakawa M, Saji E, Yanagawa K, Yanagimura F, et al. Clinicopathological features in anterior visual pathway in neuromyelitis optica. Ann Neurol. (2016) 79:605–24. doi: 10.1002/ana.24608
54. Mekhasingharak N, Laowanapiban P, Siritho S, Satukijchai C, Prayoonwiwat N, Jitprapaikulsan J, et al. Optical coherence tomography in central nervous system demyelinating diseases related optic neuritis. Int J Ophthalmol. (2018) 11:1649–56. doi: 10.18240/ijo.2018.10.12
55. Oertel FC, Havla J, Roca-Fernández A, Lizak N, Zimmermann H, Motamedi S, et al. Retinal ganglion cell loss in neuromyelitis optica: a longitudinal study. J Neurol Neurosurg Psychiatry. (2018) 89:1259–65. doi: 10.1136/jnnp-2018-318382
56. Oertel FC, Outteryck O, Knier B, Zimmermann H, Borisow N, Bellmann-Strobl J, et al. Optical coherence tomography in myelin-oligodendrocyte-glycoprotein antibody-seropositive patients: a longitudinal study. J Neuroinflammation. (2019) 16:154. doi: 10.1186/s12974-019-1521-5
57. Pache F, Zimmermann H, Mikolajczak J, Schumacher S, Lacheta A, Oertel FC, et al. MOG-IgG in NMO and related disorders: a multicenter study of 50 patients. Part 4: afferent visual system damage after optic neuritis in MOG-IgG-seropositive versus AQP4-IgG-seropositive patients. J Neuroinflammation. (2016) 13:282. doi: 10.1186/s12974-016-0720-6
58. Peng CX, Li HY, Wang W, Wang JQ, Wang L, Xu QG, et al. Retinal segmented layers with strong aquaporin-4 expression suffered more injuries in neuromyelitis optica spectrum disorders compared with optic neuritis with aquaporin-4 antibody seronegativity detected by optical coherence tomography. Br J Ophthalmol. (2017) 101:1032–7. doi: 10.1136/bjophthalmol-2016-309412
59. Stiebel-Kalish H, Lotan I, Brody J, Chodick G, Bialer O, Marignier R, et al. Retinal nerve fiber layer may be better preserved in MOG-IgG versus AQP4-IgG optic neuritis: a cohort study. PLoS ONE. (2017) 12:e0170847. doi: 10.1371/journal.pone.0170847
60. Tian G, Li Z, Zhao G, Feng C, Li M, Huang Y, et al. Evaluation of retinal nerve fiber layer and ganglion cell complex in patients with optic neuritis or neuromyelitis optica spectrum disorders using optical coherence tomography in a Chinese cohort. J Ophthalmol. (2015) 2015:832784. doi: 10.1155/2015/832784
61. Zhang X, Yu H, Zhang Y, Liu X, Yu X, Chen X. The application of RNFL thickness detection in early differential diagnosis among various types of idiopathic optic neuritis. J Clin Neurosci. (2018) 55:82–5. doi: 10.1016/j.jocn.2018.06.052
62. Zhao X, Qiu W, Zhang Y, Luo Y, Zhang X, Lu L, et al. A prospective case-control study comparing optical coherence tomography characteristics in neuromyelitis optica spectrum disorder- optic neuritis and idiopathic optic neuritis. BMC Ophthalmol. (2018) 18:247. doi: 10.1186/s12886-018-0902-3
63. Eyre M, Hameed A, Wright S, Brownlee W, Ciccarelli O, Bowman R, et al. Retinal nerve fibre layer thinning is associated with worse visual outcome after optic neuritis in children with a relapsing demyelinating syndrome. Dev Med Child Neurol. (2018) 60:1244–50. doi: 10.1111/dmcn.13757
64. Hu S, Lu P. Retinal ganglion cell-inner plexiform and nerve fiber layers in neuromyelitis optica. Int J Ophthalmol. (2018) 11:89–93. doi: 10.18240/ijo.2018.01.16
65. Lim Y, Pyun SY, Lim HT, Jeong IH, Kim K. First-ever optic neuritis: distinguishing subsequent neuromyelitis optica from multiple sclerosis. Neurol Sci. (2014) 35:781–3. doi: 10.1007/s10072-014-1635-6
66. Martinez-Lapiscina EH, Sepulveda M, Torres-Torres R, Alba-Arbalat S, Llufriu S, Blanco Y, et al. Usefulness of optical coherence tomography to distinguish optic neuritis associated with AQP4 or MOG in neuromyelitis optica spectrum disorders. Ther Adv Neurol Disord. (2016) 9:436–40. doi: 10.1177/1756285616655264
67. Narayan RN, McCreary M, Conger D, Wang C, Greenberg BM. Unique characteristics of optical coherence tomography (OCT) results and visual acuity testing in myelin oligodendrocyte glycoprotein (MOG) antibody positive pediatric patients. Mult Scler Relat Disord. (2019) 28:86–90. doi: 10.1016/j.msard.2018.11.026
68. Outteryck O, Majed B, Defoort-Dhellemmes S, Vermersch P, Zéphir H. A comparative optical coherence tomography study in neuromyelitis optica spectrum disorder and multiple sclerosis. Mult Scler. (2015) 21:1781–93. doi: 10.1177/1352458515578888
69. Shen T, You Y, Arunachalam S, Fontes A, Liu S, Gupta V, et al. Differing structural and functional patterns of optic nerve damage in multiple sclerosis and neuromyelitis optica spectrum disorder. Ophthalmology. (2019) 126:445–53. doi: 10.1016/j.ophtha.2018.06.022
70. von Glehn F, Jarius S, Cavalcanti Lira RP, Alves Ferreira MC, von Glehn FH, Costa ECSM, et al. Structural brain abnormalities are related to retinal nerve fiber layer thinning and disease duration in neuromyelitis optica spectrum disorders. Mult Scler. (2014) 20:1189–97. doi: 10.1177/1352458513519838
71. You Y, Zhu L, Zhang T, Shen T, Fontes A, Yiannikas C, et al. Evidence of müller glial dysfunction in patients with aquaporin-4 immunoglobulin g-positive neuromyelitis optica spectrum disorder. Ophthalmology. (2019) 126:801–10. doi: 10.1016/j.ophtha.2019.01.016
72. Srikajon J, Siritho S, Ngamsombat C, Prayoonwiwat N, Chirapapaisan N. Differences in clinical features between optic neuritis in neuromyelitis optica spectrum disorders and in multiple sclerosis. Mult Scler J Exp Transl Clin. (2018) 4:2055217318791196. doi: 10.1177/2055217318791196
73. Pandit L, Mustafa S, Nakashima I, Takahashi T, Kaneko K. MOG-IgG-associated disease has a stereotypical clinical course, asymptomatic visual impairment and good treatment response. Mult Scler J Exp Transl Clin. (2018) 4:2055217318787829. doi: 10.1177/2055217318787829
74. Akaishi T, Nakashima I, Takeshita T, Kaneko K, Mugikura S, Sato DK, et al. Different etiologies and prognoses of optic neuritis in demyelinating diseases. J Neuroimmunol. (2016) 299:152–7. doi: 10.1016/j.jneuroim.2016.09.007
75. Akaishi T, Sato DK, Takahashi T, Nakashima I. Clinical spectrum of inflammatory central nervous system demyelinating disorders associated with antibodies against myelin oligodendrocyte glycoprotein. Neurochem Int. (2019) 130:104319. doi: 10.1016/j.neuint.2018.10.016
76. Cobo-Calvo A, Ruiz A, Maillart E, Audoin B, Zephir H, Bourre B, et al. Clinical spectrum and prognostic value of CNS MOG autoimmunity in adults: the MOGADOR study. Neurology. (2018) 90:e1858–69. doi: 10.1212/WNL.0000000000005560
77. Ishikawa H, Kezuka T, Shikishima K, Yamagami A, Hiraoka M, Chuman H, et al. Epidemiologic and clinical characteristics of optic neuritis in Japan. Ophthalmology. (2019) 126:1385–98. doi: 10.1016/j.ophtha.2019.04.042
78. Kim N, Kim HJ, Park C, Jeong KS. Retinal Degeneration after first-ever optic neuritis helps differentiate multiple sclerosis and neuromyelitis optica spectrum disorder. Front Neurol. (2019) 10:1076. doi: 10.3389/fneur.2019.01076
79. Kitley J, Waters P, Woodhall M, Leite MI, Murchison A, George J, et al. Neuromyelitis optica spectrum disorders with aquaporin-4 and myelin-oligodendrocyte glycoprotein antibodies: a comparative study. JAMA Neurol. (2014) 71:276–83. doi: 10.1001/jamaneurol.2013.5857
80. Piccolo L, Woodhall M, Tackley G, Juryńczyk M, Kong Y, Domingos J, et al. Isolated new onset 'atypical' optic neuritis in the NMO clinic: serum antibodies, prognoses and diagnoses at follow-up. J Neurol. (2016) 263:370–9. doi: 10.1007/s00415-015-7983-1
81. Ramanathan S, Prelog K, Barnes EH, Tantsis EM, Reddel SW, Henderson APD, et al. Radiological differentiation of optic neuritis with myelin oligodendrocyte glycoprotein antibodies, aquaporin-4 antibodies, and multiple sclerosis. Mult Scler. (2016) 22:470–82. doi: 10.1177/1352458515593406
82. Sepúlveda M, Armangué T, Sola-Valls N, Arrambide G, Meca-Lallana JE, Oreja-Guevara C, et al. Neuromyelitis optica spectrum disorders: comparison according to the phenotype and serostatus. Neurol Neuroimmunol Neuroinflamm. (2016) 3:e225. doi: 10.1212/NXI.0000000000000225
83. Falcão-Gonçalves AB, Bichuetti DB, de Oliveira, Enedina Maria Lobato. Recurrent optic neuritis as the initial symptom in demyelinating diseases. J Clin Neurol. (2018) 14:351–8. doi: 10.3988/jcn.2018.14.3.351
84. Merle H, Olindo S, Jeannin S, Hage R, Donnio A, Richer R, et al. Visual field characteristics in neuromyelitis optica in absence of and after one episode of optic neuritis. Clin Ophthalmol. (2013) 2013:1145–53. doi: 10.2147/OPTH.S43894
85. Peng Y, Liu L, Zheng Y, Qiao Z, Feng K, Wang J. Diagnostic implications of MOG/AQP4 antibodies in recurrent optic neuritis. Exp Ther Med. (2018) 16:950–8. doi: 10.3892/etm.2018.6273
86. Hansapinyo L, Vivattanaseth C. Clinical characteristics, treatment outcomes and predictive factors in optic neuritis. Open Ophthalmol J. (2018) 12:247–55. doi: 10.2174/1874364101812010247
87. Lai C, Tian G, Takahashi T, Liu W, Yang L, Zhang X. Neuromyelitis optica antibodies in patients with severe optic neuritis in China. J Neuroophthalmol. (2011) 31:16–9. doi: 10.1097/WNO.0b013e3181f8a693
88. Nakajima H, Motomura M, Tanaka K, Fujikawa A, Nakata R, Maeda Y, et al. Antibodies to myelin oligodendrocyte glycoprotein in idiopathic optic neuritis. BMJ Open. (2015) 5:e007766. doi: 10.1136/bmjopen-2015-007766
89. Viswanathan S, Arip M, Mustafa N, Dhaliwal JS, Rose N, Muda S, et al. The frequency of anti-aquaporin-4 Ig g antibody in neuromyelitis optica and its spectrum disorders at a single tertiary referral center in malaysia. Mult Scler Int. (2014) 2014:568254. doi: 10.1155/2014/568254
90. Hor JY, Asgari N, Nakashima I, Broadley SA, Leite MI, Kissani N, et al. Epidemiology of neuromyelitis optica spectrum disorder and its prevalence and incidence worldwide. Front Neurol. (2020) 11:501. doi: 10.3389/fneur.2020.00501
91. Alvarenga MP, Schimidt S, Alvarenga RP. Epidemiology of neuromyelitis optica in Latin America. Mult Scler J Exp Transl Clin. (2017) 3:2055217317730098. doi: 10.1177/2055217317730098
92. Wingerchuk DM, Banwell B, Bennett JL, Cabre P, Carroll W, Chitnis T, et al. International consensus diagnostic criteria for neuromyelitis optica spectrum disorders. Neurology. (2015) 85:177–89. doi: 10.1212/WNL.0000000000001729
93. Wang H, Dai Y, Qiu W, Zhong X, Wu A, Wang Y, et al. HLA-DPB1 0501 is associated with susceptibility to anti-aquaporin-4 antibodies positive neuromyelitis optica in southern Han Chinese. J Neuroimmunol. (2011) 233:181–4. doi: 10.1016/j.jneuroim.2010.11.004
94. Zéphir H, Fajardy I, Outteryck O, Blanc F, Roger N, Fleury M, et al. Is neuromyelitis optica associated with human leukocyte antigen? Mult Scler. (2009) 15:571–9. doi: 10.1177/1352458508102085
95. Kim S, Mealy MA, Levy M, Schmidt F, Ruprecht K, Paul F, et al. Racial differences in neuromyelitis optica spectrum disorder. Neurology. (2018) 91:e2089–99. doi: 10.1212/WNL.0000000000006574
96. O'Connell K, Hamilton-Shield A, Woodhall M, Messina S, Mariano R, Waters P, et al. Prevalence and incidence of neuromyelitis optica spectrum disorder, aquaporin-4 antibody-positive NMOSD and MOG antibody-positive disease in Oxfordshire, UK. J Neurol Neurosurg Psychiatry. (2020) 91:1126–28. doi: 10.1136/jnnp-2020-323158
97. Mealy M, Kessler R, Rimler Z, Reid A, Totonis L, Cutter G, et al. Mortality in neuromyelitis optica is strongly associated with African ancestry. Neurology. (2018) 5:e468. doi: 10.1212/NXI.0000000000000468
98. Schneider E, Zimmermann H, Oberwahrenbrock T, Kaufhold F, Kadas EM, Petzold A, et al. Optical coherence tomography reveals distinct patterns of retinal damage in neuromyelitis optica and multiple sclerosis. PLoS ONE. (2013) 8:e66151. doi: 10.1371/journal.pone.0066151
99. Green AJ, Cree BaC. Distinctive retinal nerve fibre layer and vascular changes in neuromyelitis optica following optic neuritis. J Neurol Neurosurg Psychiatry. (2009) 80:1002–5. doi: 10.1136/jnnp.2008.166207
100. Lucchinetti CF, Mandler RN, McGavern D, Bruck W, Gleich G, Ransohoff RM, et al. A role for humoral mechanisms in the pathogenesis of Devic's neuromyelitis optica. Brain. (2002) 125:1450–61. doi: 10.1093/brain/awf151
101. Lucchinetti CF, Guo Y, Popescu BFG, Fujihara K, Itoyama Y, Misu T. The pathology of an autoimmune astrocytopathy: lessons learned from neuromyelitis optica. Brain Pathol. (2014) 24:83–97. doi: 10.1111/bpa.12099
102. Oertel FC, Kuchling J, Zimmermann H, Chien C, Schmidt F, Knier B, et al. Microstructural visual system changes in AQP4-antibody-seropositive NMOSD. Neurol Neuroimmunol Neuroinflamm. (2017) 4:e334. doi: 10.1212/NXI.0000000000000334
103. Motamedi S, Oertel FC, Yadav SK, Kadas EM, Weise M, Havla J, et al. Altered fovea in AQP4-IgG-seropositive neuromyelitis optica spectrum disorders. Neurol Neuroimmunol Neuroinflamm. (2020) 7:e805. doi: 10.1212/NXI.0000000000000805
104. Yamamura T, Nakashima I. Foveal thinning in neuromyelitis optica: a sign of retinal astrocytopathy? Neurology Neuroimmunol Neuroinflamm. (2017) 4:e347. doi: 10.1212/NXI.0000000000000347
105. Felix CM, Levin MH, Verkman AS. Complement-independent retinal pathology produced by intravitreal injection of neuromyelitis optica immunoglobulin G. J Neuroinflammation. (2016) 13:275. doi: 10.1186/s12974-016-0746-9
106. Pannicke T, Wurm A, Iandiev I, Hollborn M, Linnertz R, Binder DK, et al. Deletion of aquaporin-4 renders retinal glial cells more susceptible to osmotic stress. J Neurosci Res. (2010) 88:2877–88. doi: 10.1002/jnr.22437
107. Ogden TE. Nerve fiber layer of the primate retina: thickness and glial content. Vision Res. (1983) 23:581–7. doi: 10.1016/0042-6989(83)90063-9
108. Silverman SM, Wong WT. Microglia in the retina: roles in development, maturity, and disease. Annu Rev Vis Sci. (2018) 4:45–77. doi: 10.1146/annurev-vision-091517-034425
109. Costello F. Optical coherence tomography in neuro-ophthalmology. Neurol Clin. (2017) 35:153–63. doi: 10.1016/j.ncl.2016.08.012
110. Waters P, Reindl M, Saiz A, Schanda K, Tuller F, Kral V, et al. Multicentre comparison of a diagnostic assay: aquaporin-4 antibodies in neuromyelitis optica. J Neurol Neurosurg Psychiatry. (2016) 87:1005–15. doi: 10.1136/jnnp-2015-312601
111. Pittock SJ, Lennon VA, Bakshi N, Shen L, McKeon A, Quach H, et al. Seroprevalence of aquaporin-4-IgG in a northern California population representative cohort of multiple sclerosis. JAMA Neurol. (2014) 71:1433–6. doi: 10.1001/jamaneurol.2014.1581
Keywords: optic neuritis (ON), optical coherence tomography (OCT), neuromyelitis optica (NMO), neuromyelitis optica spectrum disorder (NMOsd), visual acuity, retina, aquaporin-4 (AQP4) IgG, myelin oligodendrocyte glycoprotein (MOG) IgG associated disease
Citation: Filippatou AG, Mukharesh L, Saidha S, Calabresi PA and Sotirchos ES (2020) AQP4-IgG and MOG-IgG Related Optic Neuritis—Prevalence, Optical Coherence Tomography Findings, and Visual Outcomes: A Systematic Review and Meta-Analysis. Front. Neurol. 11:540156. doi: 10.3389/fneur.2020.540156
Received: 03 March 2020; Accepted: 25 August 2020;
Published: 08 October 2020.
Edited by:
Michael Levy, Massachusetts General Hospital and Harvard Medical School, United StatesReviewed by:
Friedemann Paul, Charité – Universitätsmedizin Berlin, GermanyJohn Jing-Wei Chen, Mayo Clinic, United States
Copyright © 2020 Filippatou, Mukharesh, Saidha, Calabresi and Sotirchos. This is an open-access article distributed under the terms of the Creative Commons Attribution License (CC BY). The use, distribution or reproduction in other forums is permitted, provided the original author(s) and the copyright owner(s) are credited and that the original publication in this journal is cited, in accordance with accepted academic practice. No use, distribution or reproduction is permitted which does not comply with these terms.
*Correspondence: Elias S. Sotirchos, ZXNzQGpobWkuZWR1