- 1Laboratory of Neuromodulation & Center for Clinical Research Learning, Department of Physical Medicine and Rehabilitation, Harvard Medical School, Spaulding Rehabilitation Hospital, Boston, MA, United States
- 2Faculdade Israelita de Ciências da Saúde, São Paulo, Brazil
- 3Hospital Israelita Albert Einstein, São Paulo, Brazil
Phantom limb pain (PLP) affects up to 80% of amputees. Despite the lack of consensus about the etiology and pathophysiology of phantom experiences, previous evidence pointed out the role of changes in motor cortex excitability as an important factor associated with amputation and PLP. In this systematic review, we investigated changes in intracortical inhibition as indexed by transcranial magnetic stimulation (TMS) in amputees and its relationship to pain. Four electronic databases were screened to identify studies using TMS to measure cortical inhibition, such as short intracortical inhibition (SICI), long intracortical inhibition (LICI) and cortical silent period (CSP). Seven articles were included and evaluated cortical excitability comparing the affected hemisphere with the non-affected hemisphere or with healthy controls. None of them correlated cortical disinhibition and clinical parameters, such as the presence or intensity of PLP. However, most studies showed decreased SICI in amputees affected hemisphere. These results highlight that although SICI seems to be changed in the affected hemisphere in amputees, most of the studies did not investigate its clinical correlation. Thus, the question of whether they are a valid diagnostic marker remains unanswered. Also, the results were highly variable for both measurements due to the heterogeneity of study designs and group comparisons in each study. Although these results underscore the role of inhibitory networks after amputation, more studies are needed to investigate the role of a decreased inhibitory drive in the motor cortex to the cause and maintenance of PLP.
Introduction
Amputation is associated with significant cortical reorganization. After amputation, cortex's afferent inputs from amputated limb are interrupted, resulting in decreased cortical excitation, affecting local inhibitory drive (1). Decreased cortical inhibition seems to be maladaptive and can be related with other dysfunctional behaviors, like PLP (2, 3).
One approach to appraise maladaptive cortical reorganization is Transcranial Magnetic Stimulation (TMS), a non-invasive brain stimulation technique that induces small current resulted from magnetic fields, allowing assessment of both cortical excitability parameters and therapeutic modulation, inducing plasticity (4). TMS has been used to evaluate changes in cortical excitability following amputations. Different groups have shown changes in motor evoked potential (MEP), including decreased intracortical inhibition (ICI), cortical silent period (CSP), and increased intracortical facilitation (5).
TMS evaluates cortical excitability by different parameters: motor threshold, motor evoked potential, intracortical facilitation and intracortical inhibition (measured by intracortical inhibition, ICI, or cortical silent period, CSP). Short intracortical inhibition (SICI) and ICI are responses triggered when subthreshold stimulus is followed by a suprathreshold, within a range of 1–6 ms of interstimuli interval. Usually SICI is performed with a <5 ms interval and is thought to be related with GABAA receptors (6) while ICI with GABAB (7). LICI can be elicited by a subthreshold stimuli followed by a test stimulus with a 50–200 ms inter-interval (7). The mechanism is related to suppression of neuronal activity by GABA receptors. Lastly, CSP is defined as an interruption of electromyography activity following a suprathreshold TMS pulse (8), being related to GABA interneurons activation (9).
One study Cohen et al. (10) showed that amputees presented larger MEP in affected hemisphere and increased number of excitable stimulation sites for muscles proximal to the stump. However, few studies analyzed these parameters appropriately (11). Amputation studies are heterogeneous regarding amputated limb location, time since amputation and reimplantation. Investigation of maladaptive cortical reorganization could contribute to developing novel treatments for PLP.
Phantom pain and sensations affect up to 98% of amputees (12). PLP is the most prevalent phantom phenomena (50–80%) (11), with negative impact on quality of life (11, 13). Studies suggest that cortical reorganization is reversible and related to pain levels (14), sheding light on the potential minimization or reversal of maladaptive plasticity through brain stimulation. However, most articles do not correlate changes in excitability with presence or intensity of PLP, implicating that changes in these parameters have unknown mechanisms.
Despite no consensus about etiology and pathophysiology of phantom experiences, studies associate PLP with peripheral, psychogenic, and central neural mechanisms (15, 16) and with cortical reorganization after an amputation (17, 18). Other studies show that peripheral systems contribute to neuromas' formation, followed by hyperexcitability and spontaneous discharges (19), while psychological systems may influence its intensity (20). Different patterns of change were observed in amputees with or without PLP: (1) decreased ICI in affected hemisphere; (2) decreased ICI in non-affected hemisphere; (3) unchanged ICI in affected hemisphere; and (4) changes in CSP response pattern.
Therefore, the purpose of this review is to evaluate if CSP and SICI are modified when comparing the affected vs. unaffected motor cortex and whether it provides additional insights to the role of motor cortex in the modulatory circuitry of chronic pain.
Materials and Methods
Sources and Study Selection
Literature search was performed in four electronic databases (PubMed, Web of Science, ScienceDirect, and LILACS) until February 2018, using multiple keywords and combinations—“phantom limb” AND “neuromodulation” OR “transcranial magnetic stimulation” OR “cortical excitability” OR “neuronal plasticity.” The conjunction “phantom limb AND transcranial magnetic stimulation” was combined with “intracortical inhibition” OR “cortical silent period” OR “neuromodulation.” Initial search identified 2,284 articles.
Pairs of researchers analyzed selection criteria and a third person resolved conflicts. Included articles had to: (1) be related to amputation; (2) evaluate phantom sensation; (3) use TMS as an assessment tool; (4) have data on ICI or CSP. Studies were excluded if: (a) not related to amputation; (b) related to congenitally absent limbs; (c) just included finger amputation; (d) applied different techniques of stimulation as DBS, spinal cord stimulation, tDCS, TENS, fMRI; (e) applied TMS for cortical mapping; (f) did not use neurostimulation; (g) had only pharmacologic interventions; (h) had solely psychotherapeutic approaches; (i) analyzed mirror therapy not combined with TMS/TDCS; (j) had different studies designs as posters, reviews or meta-analysis; (k) were not in English; (l) studies in animals.
The selected articles were inputted into COVIDENCE® software, which excluded duplicates, resulting in 1313 articles. Three articles were included in a manual search due to discussion of cortical excitability using TMS in amputees with PLP (21).
Results
Studies Selection
After screening titles and abstracts, 42 articles remained. After full text reading, seven articles were then selected.
Demographic Findings
One hundred and eighteen patients were analyzed, healthy subjects (45) and amputees (73). Most amputees were male young adults with traumatic upper limb amputation (5, 22–24). Six articles reported PLP (5, 22–26). Sample size, population profile, measurements, comparisons, and etiology of amputation were diverse (Table 1).
Study Design
From the studies selected, there were three cross-sectional (22, 25, 27), two case reports (5, 24), one part of a clinical trial (23), and one case-control (26). Regarding technical aspects, some (5, 24–26) used a figure-of-eight-coil, while others (22, 23, 27), a circular coil. The muscles chosen for surface electromyography were deltoid (5, 22–24), biceps (22, 23), first dorsal interosseous (FDI) (23–25), and quadriceps (26, 27) (Table 1).
Five articles used TMS as assessment tool for cortical excitability: one study (5) performed functional MRI and TMS and four studies used TMS only (22, 25–27). Furthermore, one article (23) added pharmacological intervention (memantine) and another (24) applied TMS-fMRI as intervention.
Qualitative Analysis of the Studies
Single and paired-pulse TMS were applied to investigate SICI; however, paired-pulse TMS assessment protocols were highly heterogeneous (Table 1). Conventionally (28), subthreshold conditioning stimulus (usually at 80% of the motor threshold) is followed by suprathreshold test stimulus at interstimulus intervals (ISIs) of 1–5 ms. Therefore, SICI is measured by the reduction of relative MEP amplitude by subthreshold conditioning stimuli, compared to average MEP size. In this review, ISIs varied from 1 to 5 ms and were reported separately or as intervals average.
The comparison types of SICI varied (Table 1). Some compared non affected with affected hemispheres (5, 22, 24, 26, 27), others compared amputees with healthy controls (22, 23, 25–27). However, Schwenkreis et al. (23) evaluated SICI measures before and after treatment (memantine vs. placebo), and Bestmann et al. (24) contrasted right with left deltoid, and also with FDI muscle independent of stimulation side. All CSP values were measured for both non-affected and affected hemispheres.
Studies Showing Decreased ICI in the Affected Hemisphere
Regarding SICI findings, three studies (5, 22, 24, 27) compared amputee's SICI in affected vs. non-affected hemispheres, verifying a larger conditioned MEP amplitude in affected hemisphere (lower SICI).
Both Schwenkreis studies (22, 23) reported a significant decreased SICI response in affected side compared to controls (Figure 1).
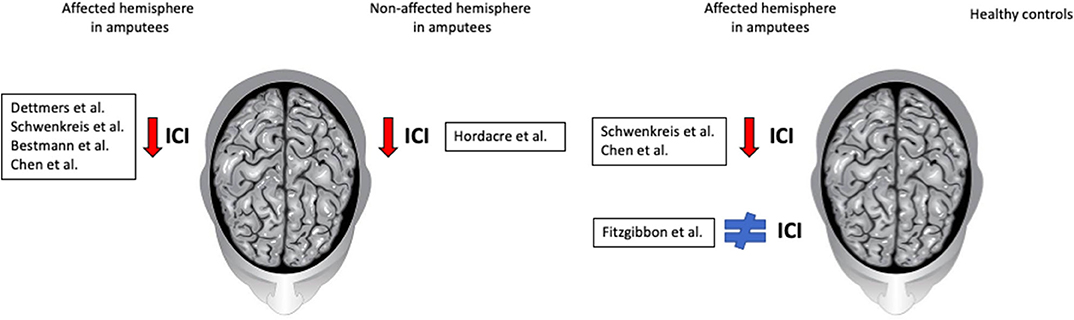
Figure 1. Summary results and comparisons of intracortical inhibition. The first panel shows comparisons between affected and non-affected hemisphere in amputees; most authors reported a decreased SICI in the affected hemisphere. The second panel shows the comparison between amputees and healthy subjects; while some authors report a decreased SICI in the affected hemisphere of amputees, others found no difference.
Studies Showing Decreased ICI in the Non-affected Hemisphere
Only Hordacre et al. (26) found reduced SICI response in non-affected hemisphere (Figure 1). He assessed amputees's SICI at four time points: admission, prosthetic casting, first walk and discharge, and contrasted with healthy controls' SICI at admission. The mean SICI response was reduced in amputees' non-affected hemisphere compared to controls.
Studies Showing Unchanged ICI in the Affected Hemisphere
Concerning SICI, while Schwenkreis et al. (22) found no differences between affected and non-affected hemispheres within each participant, Fitzgibbon et al. (25) found no differences between amputees and healthy controls' SICI (Figure 1). Subgroup analysis within amputees showed no differences between patients regardless of mirror pain. Chen et al. (27) found that mean MEP amplitude was significantly larger in affected hemisphere, compared with healthy controls.
Changes in CSP Response Pattern
Regarding CSP, three articles (5, 22, 24) measured it once and compared affected with non-affected hemispheres (Table 1). While Schwenkreis et al. (22) found similar CSP measurements for both hemispheres, Bestmann et al. (24) suggested it was markedly longer when recorded at the affected side and finally Dettmers et al. (5) showed a shortened silent period on the affected side. Only Schwenkreis et al. (22) compared CSP between amputees and healthy controls and found no statistically significant differences.
Discussion
In this review, we evaluated cortical excitability changes following upper/lower limb amputation to investigate whether it helps understand the cortical mechanisms associated with PLP development. Both measurements can be assessed by TMS and were investigated due to their role in cortical motor functioning. Most studies (5, 22, 24, 27) found a decreased mean SICI in the affected hemisphere compared with non-affected hemisphere and healthy controls. CSP results were inconclusive, especially because of scarcity of data. However, contrary to what was initially expected, most studies did not show any correlation of cortical excitability changes with presence or intensity of pain.
Central structural and function brain changes are described in several chronic pain conditions (29–32); while some are thought to be consequences of pain (29), others are not well-characterized. Regarding PLP, these alterations have gained more attention because different imaging studies have showed that cortical and plastic changes are involved with the presence of pain. In fact, these changes have opposite direction—while some showed PLP correlation with strong motor cortex reorganization and the missing representation of the amputated area (18, 33–35), others showed that it is actually correlated with the maintenance of amputated area representation (3). Despite the direction, all seem to agree on a maladaptive reorganization of the sensorimotor cortex after amputation involving a reduction in ICI mechanisms, an imbalance between inhibitory and excitatory neurotransmitters, and increased excitability of corticospinal neurons (36). Nonetheless, this reorganization does not seem to be related to pain intensity, being therefore a consequence or a cause of pain; but not related if the intensity perception or only a response to the deafferentation process occurring in amputees.
Studies Showing Decreased ICI in the Affected Hemisphere
After amputation, the motor cortex undergoes modifications previously associated with the presence or intensity of PLP. However, reorganization is observed in amputees with Lotze et al. (35) and without pain, or with other chronic pain syndromes (37). Although mechanisms that lead to pain after amputation remains unknown, some TMS studies showed that changes in cortical excitability are frequently observed in amputees that experience PLP (10). They early found excitability enhancement in amputee's affected hemisphere due to larger MEPs and increased number of excitable stimulation sites, when compared with the intact limb (10). Moreover, studies using techniques such as functional magnetic resonance (fMRI) and positron emission tomography (PET) demonstrated larger blood-oxygen-level-dependent (BOLD) activity in the affected hemisphere of amputees with PLP, compared with amputees without PLP (38). This data suggests lack of affected hemisphere's inhibitory function after amputation. Mechanistic studies showed that in early phases after amputation, motor cortex reorganization is partially driven by downregulation of GABA-related inhibitory circuits (39), which also contributes to increased excitability observed then. Indeed lack of sensory afference likely drives changes that decrease inhibitory drive in cortical circuits, and also in pain-related circuits, resulting in PLP. Accordingly, SICI can be used to measure intracortical circuits within the motor cortex and is an indirect measure of GABA-mediated inhibition. Altered SICI can modify motor outputs and cortical-subcortical connectivity. A recent meta-analysis (37) showed a significant SICI reduction in patients with chronic pain when compared with healthy subjects, possibly relating to pain chronicity.
These data indicate motor cortex disinhibition in amputees' affected hemisphere, remaining unclear its relation with pain. Therefore, rather than predicting pain intensity, decreased inhibitory drive may relate to its presence according to studies that compared amputees against healthy subjects, however does not detangle amputation as its leading factor.
Studies Showing Decreased ICI in the Non-affected Hemisphere
Longitudinal studies aiming to report changes in corticomotor excitability pre and post-amputation are uncommon. Hordacre et al. (26) compared motor cortex excitability before and after transtibial elective amputation and observed SICI reduction in both hemispheres after amputation (40). Whereas, decreased amputee's SICI agrees with our findings, most reviewed studies showed a reduction in the affected hemisphere, not bilaterally. The assessment shortly after an amputation allowed Hordacre et al. (26) to document the early modulation of intracortical excitability. This could indicate that cortical environment at this period is optimized for reorganization, representing potential timeframe favorable to successful interventions.
Studies Showing Unchanged ICI in the Affected Hemisphere
Most studies (5, 22, 24, 27) showed decreased mean SICI in affected hemisphere, but Fitzgibbon et al. (25) showed no difference in SICI comparing amputees with healthy controls, and amputee's cortical excitability with and without mirror pain with no difference to healthy controls. The authors concluded that cortical disinhibition seems to be disassociated with mirror pain. However, acquired mirror pain likely has different mechanisms (2) compared to PLP.
Changes in CSP Response Pattern
Mixed results were observed in three manuscripts (5, 22, 24) that compared affected vs. non-affected hemispheres on amputees, with similar findings for both groups (amputees vs. healthy controls. Future studies on CSP can elucidate ICI mechanisms in amputated patients.
Current evidence supports CSP association with GABA interneurons activation (37, 41), hypothesizing that irregular GABA activation could be monitored during increased CSP values in the affected hemisphere. Analyzing CSP alone could bring ambiguous results; the silent period cannot predict the motor cortex excitatory state, as other variables change simultaneously in a dynamic pattern. More extensive trials focused on CSP could address the issue of heterogeneity of study designs, sample size, and parameters. Then, it would be possible to analyze its applicability, investigating its potential use to tailor therapies focused on cortical activity and neuroplasticity, rather than only treating effects.
The persistent cortical representation of the missing limb and reassignment of brain areas, may explain why mirror therapy (42) is known as a promising tool for PLP management: volitive activation of cortex area of the phantom limb allows modulation and decreases thalamic processing (12, 15, 43). Mirror therapy studies showed that pain relief may be due to neuron firing when a person performs actions with the contralateral limb or observes someone's movements (44). Some studies correlated PLP severity and location with the onset of pain relief, indicating that more severe and intense types of PLP take longer periods to respond to mirror therapy. Still, patient variability and pain subtypes might interfere on the efficacy of mirror therapy (33, 45).
There is evidence that magnitude of cortical reorganization is associated with pain severity, and that the extent of somatosensory cortex involvement is related to intensity of phantom limb experience (46, 47).
Limitations
This review included a limited amount of studies (7), composed of small samples (1 to 25) and a total of 118 individuals. Heterogeneity of parameters was concerning, as authors diverged on concept definitions for SICI, LICI, and CSP. Moreover, three of eight selected studies (22, 23, 27) used circular coil in TMS, which is not used anymore, thus not comparable to current studies.
These considerations emphasize the importance of leading research on biomarkers for PLP, so its underlying mechanisms could be better understood. These tools would enable follow up of patients' progression and allow individualized treatments, potentially decreasing the condition's burden.
Conclusion
In conclusion, the SICI changes in amputees' affected motor cortex demonstrate lack of inhibitory stimuli, suggesting it could be a useful marker to understand the consequences of amputation. However, none of the studies were able to associate this finding with clinical correlates; thus additional studies would be worthwhile to answer this question. Regardless, we showed combined evidence that amputees have decreased cortical inhibition in the affected motor cortex. Future studies evaluating differences in SICI and CSP between amputees with and without pain could provide new insights regarding maladaptive changes occurring after limb amputation and its relationship with PLP.
Author Contributions
FF and CP contributed as senior authors, aiding in the study design, and conceptual ideas. BD, EZ, FG, GG, LC, MB, PP, and SM contributed equally in the manuscript writing, design of figures, and data analysis. All authors contributed to the article and approved the submitted version.
Funding
FF was funded by a NIH RO1 grant (1R01HD082302-01A1).
Conflict of Interest
The authors declare that the research was conducted in the absence of any commercial or financial relationships that could be construed as a potential conflict of interest.
References
1. Chen A, Yao J, Kuiken T, Dewald JPA. Cortical motor activity and reorganization following upper-limb amputation and subsequent targeted reinnervation. NeuroImage Clin. (2013) 3:498–506. doi: 10.1016/j.nicl.2013.10.001
2. Collins KL, Russell HG, Schumacher PJ, Robinson-Freeman KE, O'Conor EC, Gibney KD, et al. A review of current theories and treatments for phantom limb pain. J Clin Invest. (2018) 128:2168–76. doi: 10.1172/JCI94003
3. Kikkert S, Johansen-Berg H, Tracey I, Makin TR. Reaffirming the link between chronic phantom limb pain and maintained missing hand representation. Cortex. (2018) 106:174–84. doi: 10.1016/j.cortex.2018.05.013
4. Klomjai W, Katz R, Lackmy-Vallée A. Basic principles of transcranial magnetic stimulation (TMS) and repetitive TMS (rTMS). Ann Phys Rehabil Med. (2015) 58:208–13. doi: 10.1016/j.rehab.2015.05.005
5. Dettmers C, Liepert J, Adler T, Rzanny R, Rijntjes M, van Schayck R, et al. Abnormal motor cortex organization contralateral to early upper limb amputation in humans. Neurosci Lett. (1999) 263:41–4. doi: 10.1016/S0304-3940(99)00105-6
6. Nicoll RA, Malenka RC, Kauer JA. Functional comparison of neurotransmitter receptor subtypes in mammalian central nervous system. Physiol Rev. (1990) 70:513–65. doi: 10.1152/physrev.1990.70.2.513
7. Ni Z, Gunraj C, Chen R. Short interval intracortical inhibition and facilitation during the silent period in human. J Physiol. (2007) 583:971–82. doi: 10.1113/jphysiol.2007.135749
8. Poston B, Kukke SN, Paine RW, Francis S, Hallett M. Cortical silent period duration and its implications for surround inhibition of a hand muscle. Eur J Neurosci. (2012) 36:2964–71. doi: 10.1111/j.1460-9568.2012.08212.x
9. Fuhr P, Agostino R, Hallett M. Spinal motor neuron excitability during the silent period after cortical stimulation. Electroencephalogr Clin Neurophysiol. (1991) 81:257–62. doi: 10.1016/0168-5597(91)90011-L
10. Cohen LG, Bandinelli S, Findley TW, Hallett M. Motor reorganization after upper limb amputation in man. Brain. (1991) 114:615–27. doi: 10.1093/brain/114.1.615
11. Richardson C, Kulkarni J. A review of the management of phantom limb pain: challenges and solutions. J Pain Res. (2017) 10:1861–70. doi: 10.2147/JPR.S124664
12. Ramachandran VS, Hirstein W. The perception of phantom limbs. The D. O. Hebb lecture. Brain. (1998) 121(Pt. 9):1603–30. doi: 10.1093/brain/121.9.1603
13. Ahmed A, Bhatnagar S, Mishra S, Khurana D, Joshi S, Ahmad SM. Prevalence of phantom limb pain, stump pain, and phantom limb sensation among the amputated cancer patients in India: a prospective, observational study. Indian J Palliat Care. (2017) 23:24–35. doi: 10.4103/0973-1075.197944
14. Ni Z, Anastakis DJ, Gunraj C, Chen R. Reversal of cortical reorganization in human primary motor cortex following thumb reconstruction. J Neurophysiol. (2010) 103:65–73. doi: 10.1152/jn.00732.2009
15. Subedi B, Grossberg GT. Phantom limb pain: mechanisms and treatment approaches. Pain Res Treat. (2011) 2011:1–8. doi: 10.1155/2011/864605
16. Nikolajsen L, Christensen KF. Phantom Limb Pain. In: Tubbs RS, Rizk E, Shoja M, Loukas M, Barbaro B, Spinner R, editors. Nerves and Nerve Injuries. Cambridge: Elsevier (2015). p. 23–34. doi: 10.1016/B978-0-12-802653-3.09986-3
17. Birbaumer N, Lutzenberger W, Montoya P, Larbig W, Unertl K, Töpfner S, et al. Effects of regional anesthesia on phantom limb pain are mirrored in changes in cortical reorganization. J Neurosci. (1997) 17:5503–8. doi: 10.1523/JNEUROSCI.17-14-05503.1997
18. Flor H, Elbert T, Mühlnickel W, Pantev C, Wienbruch C, Taub E. Cortical reorganization and phantom phenomena in congenital and traumatic upper-extremity amputees. Exp Brain Res. (1998) 119:205–12. doi: 10.1007/s002210050334
19. Dickinson BD, Head CA, Gitlow S, Osbahr AJ. Maldynia: pathophysiology and management of neuropathic and maladaptive pain–a report of the AMA Council on Science and Public Health. Pain Med. (2010) 11:1635–53. doi: 10.1111/j.1526-4637.2010.00986.x
20. Jensen KB, Berna C, Loggia ML, Wasan AD, Edwards RR, Gollub RL. The use of functional neuroimaging to evaluate psychological and other non-pharmacological treatments for clinical pain. Neurosci Lett. (2012) 520:156–64. doi: 10.1016/j.neulet.2012.03.010
21. Liberati A, Altman DG, Tetzlaff J, Mulrow C, Gotzsche PC, Ioannidis JPA, et al. The PRISMA statement for reporting systematic reviews and meta-analyses of studies that evaluate healthcare interventions: explanation and elaboration. BMJ. (2009) 339:b2700. doi: 10.1136/bmj.b2700
22. Schwenkreis P, Witscher K, Janssen F, Dertwinkel R, Zenz M, Malin JP, et al. Changes of cortical excitability in patients with upper limb amputation. Neurosci Lett. (2000) 293:143–6. doi: 10.1016/S0304-3940(00)01517-2
23. Schwenkreis P, Maier C, Pleger B, Mansourian N, Dertwinkel R, Malin J-P, et al. NMDA-mediated mechanisms in cortical excitability changes after limb amputation. Acta Neurol Scand. (2003) 108:179–84. doi: 10.1034/j.1600-0404.2003.00114.x
24. Bestmann S, Oliviero A, Voss M, Dechent P, Lopez-Dolado E, Driver J, et al. Cortical correlates of TMS-induced phantom hand movements revealed with concurrent TMS-fMRI. Neuropsychologia. (2006) 44:2959–71. doi: 10.1016/j.neuropsychologia.2006.06.023
25. Fitzgibbon BM, Enticott PG, Bradshaw JL, Giummarra MJ, Georgiou-Karistianis N, Chou M, et al. Motor cortical excitability and inhibition in acquired mirror pain. Neurosci Lett. (2012) 530:161–5. doi: 10.1016/j.neulet.2012.09.036
26. Hordacre B, Bradnam LV, Barr C, Patritti BL, Crotty M. Intracortical inhibition is modulated by phase of prosthetic rehabilitation in transtibial amputees. Front Hum Neurosci. (2015) 9:276. doi: 10.3389/fnhum.2015.00276
27. Chen R, Corwell B, Yaseen Z, Hallett M, Cohen LG. Mechanisms of cortical reorganization in lower-limb amputees. J Neurosci. (1998) 18:3443–50. doi: 10.1523/JNEUROSCI.18-09-03443.1998
28. Ilić TV, Meintzschel F, Cleff U, Ruge D, Kessler KR, Ziemann U. Short-interval paired-pulse inhibition and facilitation of human motor cortex: The dimension of stimulus intensity. J Physiol. (2002) 545:153–67. doi: 10.1113/jphysiol.2002.030122
29. Rodriguez-Raecke R, Niemeier A, Ihle K, Ruether W, May A. Brain gray matter decrease in chronic pain is the consequence and not the cause of pain. J Neurosci. (2009) 29:13746–50. doi: 10.1523/JNEUROSCI.3687-09.2009
30. Ivo R, Nicklas A, Dargel J, Sobottke R, Delank K-S, Eysel P, et al. Brain structural and psychometric alterations in chronic low back pain. Eur Spine J. (2013) 22:1958–64. doi: 10.1007/s00586-013-2692-x
31. Lai T-H, Protsenko E, Cheng Y-C, Loggia ML, Coppola G, Chen W-T. Neural plasticity in common forms of chronic headaches. Neural Plast. (2015) 2015:1–14. doi: 10.1155/2015/205985
32. Tatu K, Costa T, Nani A, Diano M, Quarta DG, Duca S, et al. How do morphological alterations caused by chronic pain distribute across the brain? A meta-analytic co-alteration study. NeuroImage Clin. (2018) 18:15–30. doi: 10.1016/j.nicl.2017.12.029
33. Flor H, Elbert T, Knecht S, Wienbruch C, Pantev C, Birbaumers N, et al. Phantom-limb pain as a perceptual correlate of cortical reorganization following arm amputation. Nature. (1995) 375:482–4. doi: 10.1038/375482a0
34. Flor H, Nikolajsen L, Staehelin Jensen T. Phantom limb pain: a case of maladaptive CNS plasticity? Nat Rev Neurosci. (2006) 7:873–81. doi: 10.1038/nrn1991
35. Lotze M, Grodd W, Birbaumer N, Erb M, Huse E, Flor H. Does use of a myoelectric prosthesis prevent cortical reorganization and phantom limb pain? Nat Neurosci. (1999) 2:501–2. doi: 10.1038/9145
36. Malavera A, Silva FA, Fregni F, Carrillo S, Garcia RG. Repetitive transcranial magnetic stimulation for phantom limb pain in land mine victims: a double-blinded, randomized, sham-controlled trial. J Pain. (2016) 17:911–8. doi: 10.1016/j.jpain.2016.05.003
37. Parker RS, Lewis GN, Rice DA, McNair PJ. Is motor cortical excitability altered in people with chronic pain? A systematic review and meta-analysis. Brain Stimul. (2016) 9:488–500. doi: 10.1016/j.brs.2016.03.020
38. Jutzeler CR, Curt A, Kramer JLK. Relationship between chronic pain and brain reorganization after deafferentation: a systematic review of functional MRI findings. NeuroImage Clin. (2015) 9:599–606. doi: 10.1016/j.nicl.2015.09.018
39. Wheaton LA. Neurorehabilitation in upper limb amputation: understanding how neurophysiological changes can affect functional rehabilitation. J Neuroeng Rehabil. (2017) 14:41. doi: 10.1186/s12984-017-0256-8
40. Hordacre B, Bradnam LV, Crotty M. Reorganization of the primary motor cortex following lower-limb amputation for vascular disease: a pre-post-amputation comparison. Disabil Rehabil. (2017) 39:1722–8. doi: 10.1080/09638288.2016.1207110
41. Jono Y, Iwata Y, Mizusawa H, Hiraoka K. Change in excitability of corticospinal pathway and GABA-mediated inhibitory circuits of primary motor cortex induced by contraction of adjacent hand muscle. Brain Topogr. (2016) 29:834–46. doi: 10.1007/s10548-016-0499-x
42. Wittkopf PG, Johnson MI. Mirror therapy: a potential intervention for pain management. Rev Assoc Med Bras. (2017) 63:1000–5. doi: 10.1590/1806-9282.63.11.1000
43. Rossi S, Tecchio F, Pasqualetti P, Ulivelli M, Pizzella V, Romani G, et al. Somatosensory processing during movement observation in humans. Clin Neurophysiol. (2002) 113:16–24. doi: 10.1016/S1388-2457(01)00725-8
44. Chan BL, Witt R, Charrow AP, Magee A, Howard R, Pasquina PF, et al. Mirror therapy for phantom limb pain. N Engl J Med. (2007) 357:2206–7. doi: 10.1056/NEJMc071927
45. Griffin SC, Curran S, Chan AWY, Finn SB, Baker CI, Pasquina PF, et al. Trajectory of phantom limb pain relief using mirror therapy: retrospective analysis of two studies. Scand J Pain. (2017) 15:98–103. doi: 10.1016/j.sjpain.2017.01.007
46. Roux F-E, Ibarrola D, Lazorthes Y, Berry I. Chronic motor cortex stimulation for phantom limb pain: a functional magnetic resonance imaging study: technical case report. Neurosurgery. (2008) 62:978–85. doi: 10.1227/01.NEU.0000333765.28198.18
Keywords: short intracortical inhibition, long intracortical inhibition, phantom limb pain, transcranial magnetic stimulation, cortical silent period
Citation: Candido Santos L, Gushken F, Gadotti GM, Dias BdF, Marinelli Pedrini S, Barreto MESF, Zippo E, Pinto CB, Piza PVdT and Fregni F (2020) Intracortical Inhibition in the Affected Hemisphere in Limb Amputation. Front. Neurol. 11:720. doi: 10.3389/fneur.2020.00720
Received: 10 December 2019; Accepted: 12 June 2020;
Published: 30 July 2020.
Edited by:
Stefano Tamburin, University of Verona, ItalyReviewed by:
Jack Tsao, University of Tennessee Health Science Center (UTHSC), United StatesWinnie Jensen, Aalborg University, Denmark
Copyright © 2020 Candido Santos, Gushken, Gadotti, Dias, Marinelli Pedrini, Barreto, Zippo, Pinto, Piza and Fregni. This is an open-access article distributed under the terms of the Creative Commons Attribution License (CC BY). The use, distribution or reproduction in other forums is permitted, provided the original author(s) and the copyright owner(s) are credited and that the original publication in this journal is cited, in accordance with accepted academic practice. No use, distribution or reproduction is permitted which does not comply with these terms.
*Correspondence: Felipe Fregni, ZnJlZ25pLmZlbGlwZSYjeDAwMDQwO21naC5oYXJ2YXJkLmVkdQ==
†These authors have contributed equally to this work and share first authorship