- 1Henan Key Laboratory of Child Brain Injury, Third Affiliated Hospital and Institute of Neuroscience of Zhengzhou University, Zhengzhou, China
- 2Center for Brain Repair and Rehabilitation, Institute of Neuroscience and Physiology, Sahlgrenska Academy, University of Gothenburg, Gothenburg, Sweden
- 3Department of Women's and Children's Health, Karolinska Institutet, Stockholm, Sweden
- 4Center of Perinatal Medicine and Health, Institute of Neuroscience and Physiology, Sahlgrenska Academy, University of Gothenburg, Gothenburg, Sweden
Objective: To assess the association between birth asphyxia—as defined by the pH of umbilical cord blood—and cerebral palsy in asphyxiated neonates ≥35 weeks' gestation.
Methods: Two reviewers independently selected English-language studies that included data on the incidence of cerebral palsy in asphyxiated neonates ≥35 weeks' gestation. Studies were searched from the Embase, Google Scholar, PubMed, and Cochrane Library databases up to 31 December 2019, and the references in the retrieved articles were screened.
Results: We identified 10 studies that met the inclusion criteria for our meta-analysis, including 8 randomized controlled trials and 2 observational studies. According to a random effects model, the pooled rate of cerebral palsy in the randomized controlled trials was 20.3% (95% CI: 16.0–24.5) and the incidence of cerebral palsy in the observational studies was 22.2% (95% CI: 8.5–35.8). Subgroup analysis by treatment for hypoxic ischemic encephalopathy in asphyxiated neonates showed that the pooled rates of cerebral palsy were 17.3% (95% CI: 13.3–21.2) and 23.9% (95% CI: 18.1–29.7) for the intervention group and non-intervention group, respectively.
Conclusion: Our findings suggest that the incidence of cerebral palsy in neonates (≥35 weeks' gestation) with perinatal asphyxia is significantly higher compared to that in the healthy neonate population. With the growing emphasis on improving neonatal neurodevelopment and reducing neurological sequelae, we conclude that the prevention and treatment of perinatal asphyxia is essential for preventing the development of cerebral palsy.
Introduction
Cerebral palsy (CP) is a group of syndromes caused by non-progressive brain injury in the fetus or infant and leads to lifelong disability (1). The prevalence of CP is 2.11 per 1,000 live births globally (2), which remained relatively stable from 1950 to 1980, but increased moderately between 1980 and 1990, probably due to the increased survival of very premature infants as a result of improvements in perinatal care (3, 4). The etiology of CP is complex and multifactorial (5). Although premature birth is a risk factor, the causes of CP for children born at term remain unclear (6–8). Birth asphyxia has been involved, but its contribution to CP is debatable. Evidence has suggested that most cases of CP are caused by prenatal factors and that the role of birth asphyxia is relatively small (<10% of cases) (9, 10). However, other studies have shown that birth asphyxia is one of the main causes of CP, accounting for more than 30% of cases (11–13).
Birth asphyxia is one of the important causes of neonatal morbidity and mortality (14, 15). Birth asphyxia refers to interruption of the blood flow to the placenta, leading to hypoxia and ischemia. When hypoxia–ischemia persists long enough, it will cause permanent neurologic injury, which may eventually develop into neurodevelopmental disorders such as developmental delay and CP (16, 17). The inconsistencies in the diagnosis of birth asphyxia contribute to variation in the prognosis of birth asphyxia. A previous study found that in studies with different diagnostic criteria of birth asphyxia, the proportion of CP cases with birth asphyxia ranged from <3% to over 50% (18). Metabolic acidosis in the umbilical cord has been recognized internationally as a necessary criterion for defining intrapartum hypoxia (19, 20) and has been used as the definition of asphyxia (21). Thus, the pH value of umbilical cord blood was used as the diagnostic criteria of birth asphyxia for conducting a meta-analysis of human studies to investigate the case exposure rates linking birth asphyxia to CP.
Methods
Literature Search
Relevant studies were searched from the PubMed, Google Scholar, Embase, and Cochrane Library databases up to 31 December 2019. The search was performed using keywords and subject terms related to “birth asphyxia.” The keywords and subject terms related to “cerebral palsy” or “neurodevelopmental outcome” were used to acquire studies related to CP. We combined the two parts of the search terms using “AND” to retrieve the studies (Supplementary Table 1). In order to supplement the electronic searches, we also searched the reference lists of previous reviews, key papers, and other relevant literature screened by the electronic search. Two investigators independently reviewed the titles, abstracts, and full-text publications.
Inclusion Criteria
Eligible studies were limited to research focusing on the following: (1) newborn infants who were born at ≥35 weeks' gestation, (2) evidence of birth asphyxia based on a pH ≤ 7.0 and/or a base deficit ≥12 mmol/L in an umbilical cord blood sample during the first hour after birth, and (3) clinical hypoxic ischemic encephalopathy (HIE) manifestation as well as neurodevelopmental outcomes that included data on CP. Additionally, when multiple studies based on the same population were published, only the most complete one was included.
Exclusion Criteria
The following exclusion criteria were applied: (1) reviews, meta-analyses, or case reports, (2) studies not published in English, (3) studies using evidence for birth asphyxia that was inconsistent with our inclusion criteria described above, and (4) studies reporting overlapping data.
Data Extraction
Information relating to data extraction was gathered individually from each identified article, including the name of the first author, study design, publication year, the size of sample, gestational age, asphyxiation criteria, follow-up period, and neurodevelopmental outcome regarding CP.
Quality Assessment
Of the included studies, eight were randomized controlled trials and two were observational studies. The Newcastle-Ottawa Scale was used to evaluate the quality of the observational studies (22) (Supplementary Table 2), including the choice of the research population (0–4 points), the study comparability (0–2 points), and the evaluation of exposure factor and outcome (0–3 points). The Cochrane collaboration's tool for assessing risk of bias, which is based on the important elements of reducing bias, including selection bias, performance bias, detection bias, attrition bias, reporting bias, and other biases, was used to assess the quality of the randomized controlled trials (23) (Supplementary Figure 1).
Statistical Analysis
Stata software version 12.0 (Stata Corporation, College Station, TX, US) was used for data analysis. The combined rate of CP and 95% confidence intervals (CIs) were calculated for all predetermined groups. A random effects model was used to give a pooled estimate of prevalence because of the small number of studies and the heterogeneity across studies in this meta-analysis. Heterogeneity was estimated by the Q statistic and the I2 statistic. Sensitivity analyses were performed to identify any potential influence between the included studies on the pooled prevalence of CP. Possible publication bias was tested by Egger's and Begg's tests. The significance level of Q statistic for the heterogeneity test was set to 0.10 and p < 0.05 were considered statistically significant.
Results
Search Results
The electronic database searches initially yielded 5,671 studies, and 5,616 studies were deleted due to either repetition or lack of relevance. A total of 55 full-text studies were retrieved and critically appraised. Of these articles, 45 did not satisfy the inclusion criteria (9 studies performed secondary analyses on the same study populations, 17 studies did not report the neurodevelopment outcomes, 4 studies did not have data for the pH of umbilical cord blood, and 15 studies were excluded due to unusable data such as articles that reported the prevalence of CP between those with birth asphyxia and those without birth asphyxia and articles for which the exact association between asphyxia and CP could not be determined). Of the remaining 10 acceptable studies, 8 studies were randomized controlled trials and 2 studies were observational studies (Figure 1).
Characteristics of the Studies
We compiled a dataset of 1,665 infants from the 10 studies. All of the neonates in the included studies meeting the asphyxia criteria were diagnosed as HIE. Of the eight randomized controlled trials (24–31), six studies used moderate hypothermia as a treatment for HIE in asphyxiated neonates, one used erythropoietin treatment in asphyxiated neonates, and one used a combination of moderate hypothermia and topiramate treatment in asphyxiated neonates. The two observational studies (32, 33) investigated the neurodevelopment outcomes in asphyxiated neonates without any interventions. The characteristics of included trials were summarized in Table 1.
Sensitivity Analysis and Publication Bias
Sensitivity analysis was conducted on the eight randomized controlled trials, and none of them had a significant impact on the results of the meta-analysis, suggesting that this study had good stability (Supplementary Figure 2). Publication bias was evaluated by Egger's (P = 0.134) and Begg's (P = 0.536) tests (Supplementary Figure 3). The pooled results demonstrated that there was no significant publication bias.
Pooled Rate of CP
In the eight randomized controlled trials, the number of infants with CP was 308 for a pooled rate of 20.3% (95% CI: 16.0–24.5, I2 = 76.3%). In the two observational studies, the number of infants with CP was 24 and the combined incidence was 22.2% (95% CI: 8.5–35.8, I2 = 65.1%) (Figure 2). In the randomized controlled trials, the infants were divided into intervention and non-intervention groups. The number of infants with CP was 166 in the intervention group and was 142 in the non-intervention group. The pooled rate of CP was 17.3% (95% CI: 13.3–21.2, I2 = 57.6%) in the intervention group and was 23.9% (95% CI: 18.1–29.7, I2 = 63.5%) in the non-intervention group (Figure 3), indicating that whether interventions were performed or not led to the high heterogeneity between studies.
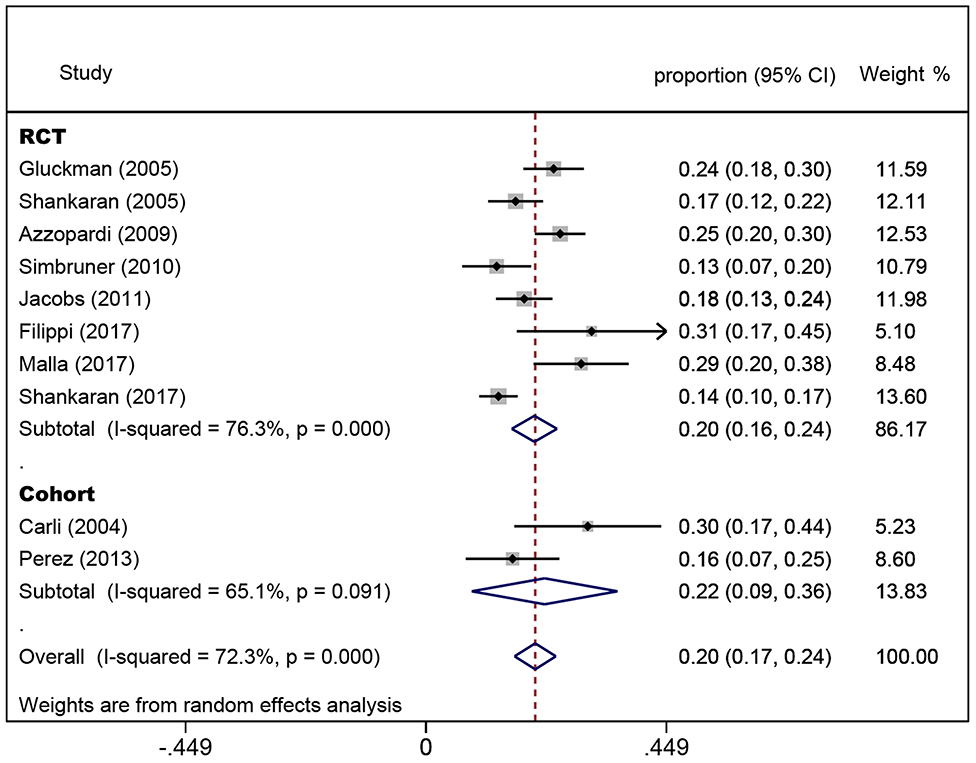
Figure 2. Forest plot of the pooled rate of cerebral palsy. The solid diamonds and horizontal solid lines represent the proportions and 95% CIs of each included study. The size of the gray area indicates the study-specific statistical weight. The hollow diamonds show the pooled proportions and 95% CIs of each group and the overall population. The vertical red dotted line shows the combined effect estimate.
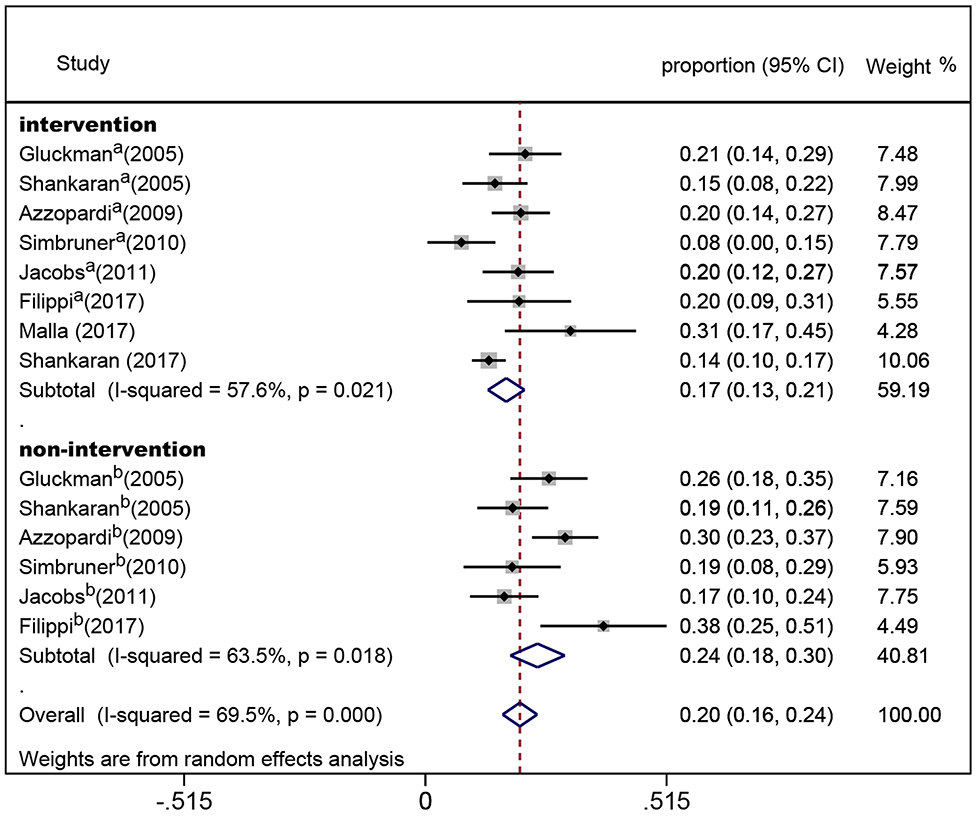
Figure 3. Forest plot of the pooled rate of cerebral palsy in the intervention and non-intervention groups in randomized controlled trials. The solid diamonds and horizontal solid lines represent the proportions and 95% CIs of each included study. The size of the gray area indicates the study-specific statistical weight. The hollow diamonds show the pooled proportions and 95% CIs of each subgroup and the overall population. The vertical red dotted line shows the combined effect estimate.
Discussion
To our knowledge, this is the first evaluation of the link between birth asphyxia and CP using the pH value of umbilical cord blood as a diagnostic criterion for birth asphyxia in addition to clinical HIE manifestations. The results of this meta-analysis indicated that birth asphyxia is associated with CP in both term and near-term infants.
Birth asphyxia might affect the outcomes of neurodevelopment in infants through a variety of mechanisms. Prolonged or intense asphyxia will cause energy depletion in tissues that depend on aerobic metabolism, such as the central nervous system (34, 35). Lack of energy can lead to the failure of ATP-dependent pumps resulting in the loss of neuronal transmembrane potential (36), and thus the most sensitive areas of the brain will die (37–39). In areas that are more resistant, excessive excitability of neurons, abundant ionic calcium influx, free radical generation, and changes in mitochondrial metabolism (40–42) might cause secondary energy exhaustion and programmed neuronal death (43, 44). Thus, these irreversible brain injuries during early brain development might ultimately result in CP.
The accurate diagnosis of birth asphyxia is still a challenge worldwide, resulting in an unclear correlation between asphyxia and CP. Birth asphyxia is predicated by fetal metabolic acidosis, as measured by umbilical cord pH at birth (21, 45), and a recent study showed that a low umbilical cord pH was associated with the occurrence of CP but failed to prove that there was a link between the degree of acidosis and the prevalence or severity of CP (46). In two studies an umbilical arterial pH ≤ 7.00 was referred to pathological or severe fetal acidemia in which the risk of adverse neurological sequelae was increased (47, 48). Furthermore, different consensus statements have mentioned the diagnosis of intrapartum asphyxia since 1992. These statements point out that metabolic acidosis (pH < 7.0 and base deficit of 12 mmol/L or more) is the essential criterion for the diagnosis of asphyxia (49, 50). Therefore, an umbilical arterial pH ≤ 7.00 and/or a base deficit of 12 mmol/L or more was used as the standard of acidosis to diagnose birth asphyxia in this meta-analysis in addition to neonatal clinical HIE manifestations.
The American Academy of Pediatrics and the Society of Obstetrics and Gynecology suggests that infants suffering from “asphyxiation” near delivery, which is severe enough to result in acute neurologic injury, should meet the following criteria: (1) severe metabolic or mixed acidemia (pH < 7.00) on an umbilical arterial blood sample, (2) an Apgar score of 0 to 3 for longer than 5 min, (3) neurologic manifestation such as seizure, coma, or hypotonia, and (4) evidence of multiorgan dysfunction (51). However, it is difficult to measure all of the diagnostic criteria in the clinic, and currently the Apgar score system is most commonly used. Neither Apgar score nor umbilical arterial pH ≤ 7.00 and/or a base deficit ≥ 12 mmol/L as the diagnostic criterion of asphyxia is a complete definition of birth asphyxia, and fetal severe acidosis is instead considered to be a fairer and more objective standard (52).
The evidence reported in previous studies was unable to support a clear association between birth asphyxia and CP (9, 53). However, our pooled analysis of 1,665 infants in 10 studies showed that the CP incidence was 20.3% (95% CI: 16.0–24.5, I2 = 76.3%) in the randomized controlled trials and 22.2% (95% CI: 8.5–35.8, I2 = 65.1%) in the observational studies, which means a more relevant association between birth asphyxia and CP. With the popularization of blood gas analysis, the detection of umbilical arterial pH value at birth is easier to perform (54). Our results suggest that blood gas analysis should be used along with Apgar score in daily clinic work when there is the possibility of birth asphyxia. Considering that preterm birth is a risk factor of CP (6, 7), we only included studies with newborn infants who were born at term or near term. Some of the patients were treated with hypothermia and/or drugs in randomized controlled trials, so we divided these patients into intervention and non-intervention groups. The results showed that the incidence of CP in the intervention group had a slight decrease compared to that in the non-intervention group, which was consistent with the conclusion that hypothermia therapy can reduce the risk of neurological impairment in infants with HIE (55). Further treatment should be considered to prevent CP after hypothermia in the acute phase (56).
Previous analysis the association of birth asphyxia and CP showed a large variation from 3 to 50% (18). Other researchers also indicated that <12% of children who have CP were exposed to perinatal asphyxia, which contradicts our results (57–59). For such inconsistent results linking birth asphyxia and CP, the differences in the diagnosis criteria of birth asphyxia were probably the main problems (5, 60). The critical criteria might affect our results to a certain extent. Nevertheless, we still hope to emphasize the importance of severe acidosis as one of necessary criteria of asphyxia in clinical application through the results in this meta-analysis.
Our meta-analysis has some other limitations. First, we only searched literature published in English. Second, publication bias and incomplete ascertainment of published literature might exist. Third, the number of studies in our analysis was small, and the selection of the diagnostic criteria of birth asphyxia might have caused a selection bias in our study. Therefore, the results of this study should be interpreted with caution. Furthermore, some of the included studies used interventions, so measurement bias existed and some heterogeneity was inevitable.
In conclusion, our meta-analysis provides evidence that birth asphyxia is associated with CP in children. Thus, the prevention and treatment of birth asphyxia is of great significance for reducing the prevalence of CP.
Data Availability Statement
All datasets presented in this study are included in the article/Supplementary Material.
Author Contributions
SZ and BL searched the databases, screened the articles, and collected the data. SZ wrote the first draft of the manuscript. BL and XZ were responsible for the statistical analysis and interpretation of the data. XW coordinated and supervised the data collection. SZ, CZ, and XW participated in study conception and design. XZ, CZ, and XW critically reviewed and revised the manuscript. All authors contributed to and approved the final version. All authors contributed to the article and approved the submitted version.
Funding
This work was supported by the National Nature Science Foundation of China (U1704281, 31761133015, and 81771418), the Department of Science and Technology of Henan Province, China (171100310200), VINNMER–Marie Curie (VINNOVA, 2015-04780), the Swedish Research Council (2018-02267), and Swedish Governmental grants to scientists working in health care of Gothenburg, Sweden (ALFGBG-717791).
Conflict of Interest
The authors declare that the research was conducted in the absence of any commercial or financial relationships that could be construed as a potential conflict of interest.
Supplementary Material
The Supplementary Material for this article can be found online at: https://www.frontiersin.org/articles/10.3389/fneur.2020.00704/full#supplementary-material
References
1. Graham HK, Rosenbaum P, Paneth N, Dan B, Lin JP, Damiano DL, et al. Cerebral palsy. Nat Rev Dis Primers. (2016) 2:15082. doi: 10.1038/nrdp.2015.82
2. Oskoui M, Coutinho F, Dykeman J, Jette N, Pringsheim T. An update on the prevalence of cerebral palsy: a systematic review and meta-analysis. Dev Med Child Neurol. (2013) 55:509–19. doi: 10.1111/dmcn.12080
3. Paneth N, Hong T, Korzeniewski S. The descriptive epidemiology of cerebral palsy. Clin Perinatol. (2006) 33:251–67. doi: 10.1016/j.clp.2006.03.011
4. Bhushan V, Paneth N, Kiely JL. Impact of improved survival of very low birth weight infants on recent secular trends in the prevalence of cerebral palsy. Pediatrics. (1993) 91:1094–100.
5. MacLennan AH, Lewis S, Moreno-De-Luca A, Fahey M, Leventer RJ, McIntyre S, et al. Genetic or other causation should not change the clinical diagnosis of cerebral palsy. J Child Neurol. (2019) 34:472–6. doi: 10.1177/0883073819840449
6. Nelson KB, Blair E. Prenatal factors in singletons with cerebral palsy born at or near term. N Engl J Med. (2015) 373:946–53. doi: 10.1056/NEJMra1505261
7. Moster D, Lie RT, Markestad T. Long-term medical and social consequences of preterm birth. N Engl J Med. (2008) 359:262–73. doi: 10.1056/NEJMoa0706475
8. Song J, Sun H, Xu F, Kang W, Gao L, Guo J, et al. Recombinant human erythropoietin improves neurological outcomes in very preterm infants. Ann Neurol. (2016) 80:24–34. doi: 10.1002/ana.24677
9. Jacobsson B, Hagberg G. Antenatal risk factors for cerebral palsy. Best Pract Res Clin Obstet Gynaecol. (2004) 18:425–36. doi: 10.1016/j.bpobgyn.2004.02.011
10. Blair E, Stanley FJ. Intrapartum asphyxia: a rare cause of cerebral palsy. J Pediatr. (1988) 112:515–9. doi: 10.1016/S0022-3476(88)80161-6
11. Erkin G, Delialioglu SU, Ozel S, Culha C, Sirzai H. Risk factors and clinical profiles in Turkish children with cerebral palsy: analysis of 625 cases. Int J Rehabil Res. (2008) 31:89–91. doi: 10.1097/MRR.0b013e3282f45225
12. Venkateswaran S, Shevell MI. Etiologic profile of spastic quadriplegia in children. Pediatr Neurol. (2007) 37:203–8. doi: 10.1016/j.pediatrneurol.2007.05.006
13. Anwar S, Chowdhury J, Khatun M, Mollah AH, Begum HA, Rahman Z, et al. Clinical profile and predisposing factors of cerebral palsy. Mymensingh Med J. (2006) 15:142–5. doi: 10.3329/mmj.v15i2.32
14. Lawn JE, Cousens S, Zupan J. 4 million neonatal deaths: when? Where? Why? Lancet. (2005) 365:891–900. doi: 10.1016/S0140-6736(05)71048-5
15. Zhu C, Kang W, Xu F, Cheng X, Zhang Z, Jia L, et al. Erythropoietin improved neurologic outcomes in newborns with hypoxic-ischemic encephalopathy. Pediatrics. (2009) 124:e218–26. doi: 10.1542/peds.2008-3553
16. Perlman JM. Interruption of placental blood flow during labor: potential systemic and cerebral organ consequences. J Pediatr. (2011) 158(Suppl. 2):e1–4. doi: 10.1016/j.jpeds.2010.11.003
17. Rainaldi MA, Perlman JM. Pathophysiology of birth asphyxia. Clin Perinatol. (2016) 43:409–22. doi: 10.1016/j.clp.2016.04.002
18. Ellenberg JH, Nelson KB. The association of cerebral palsy with birth asphyxia: a definitional quagmire. Dev Med Child Neurol. (2013) 55:210–6. doi: 10.1111/dmcn.12016
19. Low JA. The role of blood gas and acid-base assessment in the diagnosis of intrapartum fetal asphyxia. Am J Obstet Gynecol. (1988) 159:1235–40. doi: 10.1016/0002-9378(88)90456-5
20. Goodwin TM, Belai I, Hernandez P, Durand M, Paul RH. Asphyxial complications in the term newborn with severe umbilical acidemia. Am J Obstet Gynecol. (1992) 167:1506–12. doi: 10.1016/0002-9378(92)91728-S
21. Ruth VJ, Raivio KO. Perinatal brain damage: predictive value of metabolic acidosis and the Apgar score. BMJ. (1988) 297:24–7. doi: 10.1136/bmj.297.6640.24
22. Stang A. Critical evaluation of the Newcastle-Ottawa scale for the assessment of the quality of nonrandomized studies in meta-analyses. Eur J Epidemiol. (2010) 25:603–5. doi: 10.1007/s10654-010-9491-z
23. Higgins JP, Altman DG, Gotzsche PC, Juni P, Moher D, Oxman AD, et al. The cochrane collaboration's tool for assessing risk of bias in randomised trials. BMJ. (2011) 343:d5928. doi: 10.1136/bmj.d5928
24. Gluckman PD, Wyatt JS, Azzopardi D, Ballard R, Edwards AD, Ferriero DM, et al. Selective head cooling with mild systemic hypothermia after neonatal encephalopathy: multicentre randomised trial. Lancet. (2005) 365:663–70. doi: 10.1016/S0140-6736(05)17946-X
25. Shankaran S, Laptook AR, Ehrenkranz RA, Tyson JE, McDonald SA, Donovan EF, et al. Whole-body hypothermia for neonates with hypoxic-ischemic encephalopathy. N Engl J Med. (2005) 353:1574–84. doi: 10.1056/NEJMcps050929
26. Azzopardi DV, Strohm B, Edwards AD, Dyet L, Halliday HL, Juszczak E, et al. Moderate hypothermia to treat perinatal asphyxial encephalopathy. N Engl J Med. (2009) 361:1349–58. doi: 10.1056/NEJMoa0900854
27. Simbruner G, Mittal RA, Rohlmann F, Muche R. Systemic hypothermia after neonatal encephalopathy: outcomes of neo.nEURO.network RCT. Pediatrics. (2010) 126:e771–8. doi: 10.1542/peds.2009-2441
28. Jacobs SE, Morley CJ, Inder TE, Stewart MJ, Smith KR, McNamara PJ, et al. Whole-body hypothermia for term and near-term newborns with hypoxic-ischemic encephalopathy: a randomized controlled trial. Arch Pediatr Adolesc Med. (2011) 165:692–700. doi: 10.1001/archpediatrics.2011.43
29. Filippi L, Fiorini P, Catarzi S, Berti E, Padrini L, Landucci E, et al. Safety and efficacy of topiramate in neonates with hypoxic ischemic encephalopathy treated with hypothermia (NeoNATI): a feasibility study. J Matern Fetal Neonatal Med. (2018) 31:973–80. doi: 10.1080/14767058.2017.1304536
30. Malla RR, Asimi R, Teli MA, Shaheen F, Bhat MA. Erythropoietin monotherapy in perinatal asphyxia with moderate to severe encephalopathy: a randomized placebo-controlled trial. J Perinatol. (2017) 37:596–601. doi: 10.1038/jp.2017.17
31. Shankaran S, Laptook AR, Pappas A, McDonald SA, Das A, Tyson JE, et al. Effect of depth and duration of cooling on death or disability at age 18 months among neonates with hypoxic-ischemic encephalopathy: a randomized clinical trial. JAMA. (2017) 318:57–67. doi: 10.1001/jama.2017.7218
32. Carli G, Reiger I, Evans N. One-year neurodevelopmental outcome after moderate newborn hypoxic ischaemic encephalopathy. J Paediatr Child Health. (2004) 40:217–20. doi: 10.1111/j.1440-1754.2004.00341.x
33. Perez A, Ritter S, Brotschi B, Werner H, Caflisch J, Martin E, et al. Long-term neurodevelopmental outcome with hypoxic-ischemic encephalopathy. J Pediatr. (2013) 163:454–9. doi: 10.1016/j.jpeds.2013.02.003
34. Wassink G, Gunn ER, Drury PP, Bennet L, Gunn AJ. The mechanisms and treatment of asphyxial encephalopathy. Front Neurosci. (2014) 8:40. doi: 10.3389/fnins.2014.00040
35. Johnston MV, Fatemi A, Wilson MA, Northington F. Treatment advances in neonatal neuroprotection and neurointensive care. Lancet Neurol. (2011) 10:372–82. doi: 10.1016/S1474-4422(11)70016-3
36. Sanderson TH, Reynolds CA, Kumar R, Przyklenk K, Huttemann M. Molecular mechanisms of ischemia-reperfusion injury in brain: pivotal role of the mitochondrial membrane potential in reactive oxygen species generation. Mol Neurobiol. (2013) 47:9–23. doi: 10.1007/s12035-012-8344-z
37. Circu ML, Aw TY. Reactive oxygen species, cellular redox systems, and apoptosis. Free Radic Biol Med. (2010) 48:749–62. doi: 10.1016/j.freeradbiomed.2009.12.022
38. Redza-Dutordoir M, Averill-Bates DA. Activation of apoptosis signalling pathways by reactive oxygen species. Biochim Biophys Acta. (2016) 1863:2977–92. doi: 10.1016/j.bbamcr.2016.09.012
39. Wu Y, Song J, Wang Y, Wang X, Culmsee C, Zhu C. The potential role of ferroptosis in neonatal brain injury. Front Neurosci. (2019) 13:115. doi: 10.3389/fnins.2019.00115
40. Ahearne CE, Denihan NM, Walsh BH, Reinke SN, Kenny LC, Boylan GB, et al. Early cord metabolite index and outcome in perinatal asphyxia and hypoxic-ischaemic encephalopathy. Neonatology. (2016) 110:296–302. doi: 10.1159/000446556
41. Denihan NM, Boylan GB, Murray DM. Metabolomic profiling in perinatal asphyxia: a promising new field. Biomed Res Int. (2015) 2015:254076. doi: 10.1155/2015/254076
42. Sun Y, Li T, Xie C, Zhang Y, Zhou K, Wang X, et al. Dichloroacetate treatment improves mitochondrial metabolism and reduces brain injury in neonatal mice. Oncotarget. (2016) 7:31708–22. doi: 10.18632/oncotarget.9150
43. Xie C, Ginet V, Sun Y, Koike M, Zhou K, Li T, et al. Neuroprotection by selective neuronal deletion of Atg7 in neonatal brain injury. Autophagy. (2016) 12:410–23. doi: 10.1080/15548627.2015.1132134
44. Li K, Li T, Wang Y, Xu Y, Zhang S, Culmsee C, et al. Sex differences in neonatal mouse brain injury after hypoxia-ischemia and adaptaquin treatment. J Neurochem. (2019) 150:759–75. doi: 10.1111/jnc.14790
45. Fahey J, King TL. Intrauterine asphyxia: clinical implications for providers of intrapartum care. J Midwifery Womens Health. (2005) 50:498–506. doi: 10.1016/j.jmwh.2005.08.007
46. Malin GL, Morris RK, Khan KS. Strength of association between umbilical cord pH and perinatal and long term outcomes: systematic review and meta-analysis. BMJ. (2010) 340:c1471. doi: 10.1136/bmj.c1471
47. Sehdev HM, Stamilio DM, Macones GA, Graham E, Morgan MA. Predictive factors for neonatal morbidity in neonates with an umbilical arterial cord pH less than 7.00. Am J Obstet Gynecol. (1997) 177:1030–4. doi: 10.1016/S0002-9378(97)70008-5
48. Goldaber KG, Gilstrap LC III, Leveno KJ, Dax JS, McIntire DD. Pathologic fetal acidemia. Obstet Gynecol. (1991) 78:1103–7.
49. MacLennan A. A template for defining a causal relation between acute intrapartum events and cerebral palsy: international consensus statement. BMJ. (1999) 319:1054–9. doi: 10.1136/bmj.319.7216.1054
50. Hankins GD, Speer M. Defining the pathogenesis and pathophysiology of neonatal encephalopathy and cerebral palsy. Obstet Gynecol. (2003) 102:628–36. doi: 10.1016/S0029-7844(03)00574-X
51. Committee on Fetus and Newborn, American Academy of Pediatrics, Committee on Obstetric Practice, American College of Obstetricians and Gynecologists. Use and abuse of the Apgar score. Pediatrics. (1996) 98:141–2.
52. Perlman JM. Pathogenesis of hypoxic-ischemic brain injury. J Perinatol. (2007) 27:S39–46. doi: 10.1038/sj.jp.7211716
53. Graham EM, Ruis KA, Hartman AL, Northington FJ, Fox HE. A systematic review of the role of intrapartum hypoxia-ischemia in the causation of neonatal encephalopathy. Am J Obstet Gynecol. (2008) 199:587–95. doi: 10.1016/j.ajog.2008.06.094
54. Armstrong L, Stenson BJ. Use of umbilical cord blood gas analysis in the assessment of the newborn. Arch Dis Child Fetal Neonatal Ed. (2007) 92:F430–4. doi: 10.1136/adc.2006.099846
55. Edwards AD, Brocklehurst P, Gunn AJ, Halliday H, Juszczak E, Levene M, et al. Neurological outcomes at 18 months of age after moderate hypothermia for perinatal hypoxic ischaemic encephalopathy: synthesis and meta-analysis of trial data. BMJ. (2010) 340:c363. doi: 10.1136/bmj.c363
56. Fleiss B, Gressens P. Tertiary mechanisms of brain damage: a new hope for treatment of cerebral palsy? Lancet Neurol. (2012) 11:556–66. doi: 10.1016/S1474-4422(12)70058-3
57. Pappas A, Korzeniewski SJ. Long-term cognitive outcomes of birth asphyxia and the contribution of identified perinatal asphyxia to cerebral palsy. Clin Perinatol. (2016) 43:559–72. doi: 10.1016/j.clp.2016.04.012
58. Nelson KB, Ellenberg JH. Apgar scores as predictors of chronic neurologic disability. Pediatrics. (1981) 68:36–44.
59. Nelson KB, Ellenberg JH. Antecedents of cerebral palsy. Multivariate analysis of risk. N Engl J Med. (1986) 315:81–6. doi: 10.1056/NEJM198607103150202
Keywords: birth asphyxia, cerebral palsy, erythropoietin, hypothermia, meta-analysis
Citation: Zhang S, Li B, Zhang X, Zhu C and Wang X (2020) Birth Asphyxia Is Associated With Increased Risk of Cerebral Palsy: A Meta-Analysis. Front. Neurol. 11:704. doi: 10.3389/fneur.2020.00704
Received: 11 April 2020; Accepted: 09 June 2020;
Published: 16 July 2020.
Edited by:
Bobbi Fleiss, RMIT University, AustraliaReviewed by:
Sandra E. Juul, University of Washington, United StatesThalia Harmony, National Autonomous University of Mexico, Mexico
Copyright © 2020 Zhang, Li, Zhang, Zhu and Wang. This is an open-access article distributed under the terms of the Creative Commons Attribution License (CC BY). The use, distribution or reproduction in other forums is permitted, provided the original author(s) and the copyright owner(s) are credited and that the original publication in this journal is cited, in accordance with accepted academic practice. No use, distribution or reproduction is permitted which does not comply with these terms.
*Correspondence: Xiaoyang Wang, xiaoyang.wang@fysiologi.gu.se