- 1Department of Otorhinolaryngology and Head & Neck Surgery, Antwerp University Hospital, Edegem, Belgium
- 2Department of Translational Neurosciences, Faculty of Medicine and Health Sciences, University of Antwerp, Antwerp, Belgium
Hearing loss not only has a significant impact on the quality of life of patients and society, but its correlation with cognitive decline in an aging population will also increase the risk of incident dementia. While current management of hearing loss is focused on hearing rehabilitation (and essentially symptomatic), patients are suffering from the burden of progressive hearing loss before hearing aids or cochlear implants are fitted. Although these devices have a significant effect on speech understanding, they do not always lead to normal speech understanding, especially in noisy environments. A significant number of patients suffer from autosomal dominantly inherited disorders that can produce progressive sensorineural hearing loss. This includes DFNA9, a disorder caused by pathologic variants in the COCH gene that leads to post-lingual profound sensorineural hearing loss and bilateral vestibulopathy. Carriers of a pathogenic variant leading to DFNA9 can be diagnosed at the pre-symptomatic or early symptomatic stage which creates a window of opportunity for treatment. Preventing hearing loss from occurring or stabilizing progression would provide the opportunity to avoid hearing aids or cochlear implants and would be able to reduce the increased incidence of dementia. While innovative therapies for restoration of hearing have been studied for restoration of hearing in case of severe-to-profound sensorineural hearing loss and congenital hearing loss, further research is needed to study how we can modify disease progression in late-onset autosomal dominant hereditary sensorineural hearing loss. Recently, gene editing strategies have been explored in autosomal dominant disorders to disrupt dominant mutations selectively without affecting wild-type alleles.
“Why the model of autosomal dominant hereditary hearing loss can be of interest.”
Introduction
Hearing loss has a significant impact on quality of life and society in general. Hearing impairment is the most frequent sensory deficit, affecting 360 million people worldwide and therefore it has been listed by the World Health Organization (WHO) as one of the priority diseases for research into therapeutic interventions to address public health needs (1). The global annual cost of unaddressed hearing loss has been estimated by the WHO to be in the range of $750–790 billion (1). Currently, no disease-modifying therapies are available to slow down or prevent progressive sensorineural hearing loss from happening. Instead, treatment is currently focused on hearing rehabilitation, which means fitting hearing aids that amplify sounds in case of moderate-to-severe sensorineural hearing loss (2, 3). In case of severe-to-profound sensorineural hearing loss, when amplification no longer leads to adequate speech perception, cochlear implantation may provide a solution (4–6). During cochlear implantation a multi-channel electrode is surgically introduced in the cochlea to electrically stimulate the spiral ganglion neurons, replacing mechano-transduction in the hair cells (7).
Rehabilitation of Hearing Loss
Hearing aids and cochlear implants should be regarded as symptomatic treatments that are able to restore functionality (i.e., communication) and quality of life to a certain level. Bilateral cochlear implantation has been reported to restore binaural hearing to a certain level and improve speech comprehension, while reducing tinnitus burden and psychological comorbidities (8–10). However, in most health services, adult cochlear implantation is only reimbursed in a single ear because of its significant cost (10, 11). Despite the availability of these treatments, hearing-impaired patients are often reluctant to adopt any (12, 13). Although many studies have confirmed significant improvement in speech understanding after cochlear implantation, even in aging patients, many potential adult CI candidates are unaware of this treatment option or opt out, with <10% of those with severe-to-profound bilateral sensorineural hearing loss receiving a cochlear implant (14). This low rate is widespread, regardless of geographical location, and is independent of how health services are organized and country-specific economic output (15–18). In contrast, congenital severe-to-profound sensorineural hearing loss is identified at a very young age using universal neonatal hearing screening and the cochlear implantation rate in children is high (19). The obvious disadvantage of hearing rehabilitation is that we are waiting for loss of functionality to occur and act whenever certain hearing thresholds are reached to reimburse hearing aids or cochlear implants (6, 20).
Hearing Loss and Cognitive Decline are Linked
Over the past decade the correlation between hearing loss and cognitive decline in the older population has gained more research interest. Large prospective studies have found an independent relationship between hearing loss on the one hand and age-related cognitive decline and incident dementia on the other hand (21–24). Worldwide, around 50 million people have dementia, with 10 million new cases added every year, and no cure available yet. Therefore, the WHO has also recognized dementia as a public health priority to identify any disease-modifying treatments (25, 26). Because of its close relationship to the cochlea, the vestibular system has also been implicated in cognitive decline (27). However, because earlier studies did not take a potentially concomitant sensorineural hearing loss in to account or were performed in a static condition, further research will be necessary to identify its role (28–32).
The Pathophysiology Behind the Link Between Hearing Loss and Cognitive Decline
The underlying cause of the correlation between hearing and cognition remains unclear, but several hypotheses have been suggested. The common cause hypothesis suggests that hearing impairment and cognitive decline may result from one common mechanism. The cognitive load on perception hypothesis implies that a reduction in cognitive functioning may result in a heavier load on sensory processing. Both hypotheses suggest a top-down correlation. Another hypothesis, the sensory-deprivation hypothesis, implicates peripheral hearing loss as the direct cause of permanent cognitive decline, while the information-degradation hypothesis will point at depression and social isolation resulting from sensorineural hearing loss as the indirect cause. The latter hypotheses rather suggest a bottom-up causality from the periphery. At a pathophysiological level, evidence from rodent studies suggests that sensorineural hearing loss may result in a decreased adult hippocampal neurogenesis, which subsequently leads to impairments of learning and memory (33–35). At a clinical level, brain atrophy has been observed in longitudinal MRI studies in patients with hearing loss when compared with their normally hearing peers (36, 37). This brain atrophy may be a result of ongoing decreased adult hippocampal neurogenesis and would argue in favor of a bottom-up causality, i.e., peripheral sensorineural hearing loss induces changes in the central nervous system. Cognitive decline may lead to mild cognitive impairment, which may subsequently progress to incident dementia. Hearing loss has been identified as the most important modifiable risk factor to dementia onset in middle life (45–65 years). Livingston et al. raised the importance of prevention in dementia and focuses on potentially modifiable risk factors, which totals 35% of known risk factors (38). When specifically looking at these population attributable fractions, hearing loss accounts for 9.1% of these potentially modifiable risk factors, in contrast to hypertension (2%) and obesity (0.8%) (38).
CAN Hearing Rehabilitation Slow Down Cognitive Decline?
The increased risk of cognitive decline in hearing-impaired patients brings the impact of progressive sensorineural hearing loss to another level. In case of mild hearing loss, the risk of incident dementia will increase by x1.89, it will increase by x3 where the patient progresses to moderate hearing loss and will increase by 4.94 if the patient reaches the level of severe-to-profound hearing loss (21). As hearing aids can improve hearing and contribute to reestablishing the individual's participation in society, they could have a positive effect on the expected trajectory of cognition (39). However, the results of studies investigating the effect of hearing aids on cognitive function in older adults are inconclusive (40–47). Recent studies have also studied the impact of unilateral cochlear implantation on the cognitive capabilities of older adults with bilateral severe-to-profound sensorineural hearing loss (28, 48–61). While cochlear implantation leads to a significant improvement of speech understanding in older adults and some improvement in cognition overall when compared to the preoperative performance, even experienced cochlear implant users still underperform when compared with their peers when matched for age, sex and education. It is unclear what the exact reason for some of the improvement that has been observed may be: are hearing aids and cochlear implants improving cognitive abilities or are cognitively healthy individuals more educated about hearing rehabilitation and more prone to seek help (62)?
Challenges and Opportunities for Disease-Modifying Therapies In Progressive Post-Lingual Sensorineural Hearing Loss
Instead of waiting for hearing loss to occur and rehabilitate hearing function to a subpar level, we could aim to develop disease-modifying treatments that are able to slow down or prevent hearing loss progression. Current actions that try to prevent hearing loss are protection from noise exposure and adequate treatment of (or immunization to prevent) upper airway and central nervous infections, such as acute and chronic otitis media, or meningitis (1). A significant challenge in case of age-related hearing loss is the prediction of hearing loss progression. Although the annual deterioration rate of hearing is ~1 dB hearing level (dBHL) per year and patients with mild-to-moderate sensorineural hearing loss may progress to severe-to-profound sensorineural hearing loss, this progression may stabilize spontaneously for an indefinite period at any level (Figure 1) (63–65). For this reason, longitudinal studies evaluating hearing loss over time are essential to enable multi-state modeling and predictions of hearing progression on a population and individual level (66). Several potential therapeutic targets related to age-related hearing loss have been identified, including oxidative phosphorylation dysfunction-related apoptosis and mutations in mitochrondrial DNA. Strategies that have been suggested to influence hearing loss progression are stem cell-based therapy, gene therapy, aspirin, antioxidant defense, antioxidant enhancement, aldosterone modulation, and operant training (67–69). To date, no treatment has emerged to act as a disease-modifier to slow down or prevent sensorineural hearing loss.
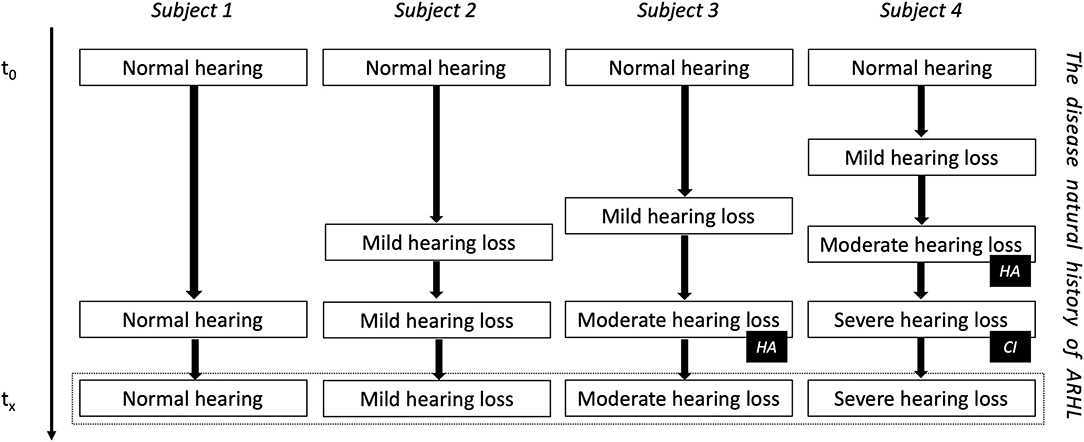
Figure 1. The natural evolution of age-related hearing loss (ARHL). Subject four is the example of DFNA9 in carriers of the P51S pathogenic variant in the COCH gene. HA, indication for a hearing aid; CI, indication for a cochlear implant.
There is one important cause of postlingual (adult-onset) sensorineural hearing loss where we do know what is happening in the cochlea and what will happen to hearing loss progression in a single adult patient: non-syndromic autosomal dominant hereditary hearing loss. Non-syndromic hearing impairment is a partial or total impairment of hearing not associated with other signs and symptoms. Between 75 and 80 percent of these cases are inherited through an autosomal recessive pattern, while another 20–25 percent of non-syndromic hearing impairment have an autosomal dominant pattern of inheritance (70, 71). The latter means that one copy of the altered gene in each cell is sufficient to cause the phenotype. Consequently, the inheritance rate is 50%. Most reported disorders with postlingual non-syndromic sensorineural demonstrate an autosomal dominant inheritance pattern. One of the first autosomal dominant disorders to be reported is called DFNA9. The DFN is an acronym for DeaFNess, while the A stands for autosomal dominant. DFNA9 is caused by heterozygous gain-of-function mutations or pathogenic variants in the COCH gene. To date, over 25 different variants have been identified worldwide (28). The P51S variant (DFNA9P51S) is the most frequently reported pathogenic variant in Belgium and the Netherlands. The phenotype is characterized by a progressive sensorineural hearing loss, starting from the 3rd−4th decade, followed by a rapid decline to severe-to-profound sensorineural hearing loss by the 6th−7th decade (72–74). Progressive vestibular dysfunction starts at a similar age and evolves toward bilateral vestibular function loss (bilateral vestibulopathy, BVP). BVP causes oscillopsia and imbalance while walking (especially in the dark) (75–83). Currently, no treatment is available to prevent or slow down sensorineural hearing loss or BVP in DFNA9 patients.
Innovative Therapies to Treat Sensorineural Hearing Loss are Emerging
Over 100 clinical trials that evaluate novel inner ear therapies are ongoing worldwide, while only one of these trials involves gene therapy. The latter is the first-in-human phase 1/2 clinical trial (supported by the FDA) to upregulate the atonal gene (ATOH1/MATH1) in supporting cells of the inner ear and to trigger trans-differentiation into functional hair cells (84). For a review on the current state-of-art on gene therapy for human sensorineural hearing loss, please refer to the following papers (85, 86). Recently reported rodent studies on gene editing have generally been aiming to restore hearing in case of congenital sensorineural hearing loss by recovery of gene and protein expression, and subsequent restoration of sensory cell function, e.g., in Usher type 1c (87) or Usher type 1g (88). Clinically, this strategy would imply early treatment (i.e., the intrauterine or neonatal period in humans) in a population with prelingual sensorineural hearing loss, in contrast to adult-onset progressive sensorineural hearing loss. Recently, gene editing strategies have been explored in autosomal dominant disorders (which mainly involve single nucleotide substitutions) to disrupt dominant mutations selectively without affecting wild-type alleles (89). Specifically in DFNA36, Tmc1 point mutations were targeted using an adeno-associated virus-mediated delivery to prevent deafness up to 1 year post-injection in mice (90). A similar strategy may work for other forms of autosomal dominant disorders (91). RNA inhibition using antisense nucleotides is another strategy that has been studied to prevent sensorineural hearing loss in Usher syndrome (92, 93).
Why DFNA9 Can Serve as a Model for Age-Related Hearing Loss
The nature of DFNA9 may present some opportunities for developing a disease-modifying treatment for progressive sensorineural hearing loss and testing this potential treatment in a future clinical trial. Not only do we know the exact cause at a protein level (i.e., a mutated isoform of cochlin), we also know in what area in the inner ear the protein is expressed most abundantly (i.e., fibrocytes of the spiral ligament and spiral limbus) and what its pathophysiology is (primarily fibrocyte degeneration and subsequent spiral ganglion degeneration) (94). Earlier studies have shed light on the annual deterioration rate in DFNA9P51S, i.e., 3 dBHL per year -in contrast to 1 dBHL per year in age-related hearing loss- and has demonstrated high penetrance, i.e., the occurrence of a phenotype in pathogenic variant carriers (74). It is important to notice that each pathogenic variant in DFNA9 may have another phenotype.
In potential pathogenic variant carriers with a known family history, we can establish the genetic diagnosis in pre-symptomatic patients by taking a routine blood sample. If tested at the age of 18 years, early diagnosis in normal hearing carriers will lead to a significant therapeutic interval of up to 25 years to administer any disease-modifying therapy. It also provides opportunity to study if any such therapy will be able to reduce progression speed or (in an optimal scenario) prevent hearing loss from occurring at all. Although restoring hearing up to a certain level has become mainstream because of cochlear implantation, little is known on patient attitudes toward preventing, stabilizing or slowing down progression of sensorineural hearing loss by means of such future potentially disease-modifying treatments. In a survey performed in 53 carriers of pathogenic variants in the COCH gene, various hypothetical scenarios were presented while using a Likert scale to study willingness to participate in clinical trials studying potential treatment strategies (95). Overall, most symptomatic patients would likely consider participation in future innovative inner ear therapy trials, even if it would only slow down the decline of hearing and vestibular function. However, they were more equivocal on high-risk treatments or a placebo-controlled study design. Next to DFNA9, currently over 40 genes are known to be responsible for autosomal dominant non-syndromic hearing loss and may present similar opportunities for innovative treatment. The former data can be used to inform the recruitment and consent process into future innovative treatments to treat these other autosomal dominant disorders. However, the phenotype of different autosomal dominant disorders can vary quite significantly, and therefore may have a significant impact on patient anticipation.
Data Availability Statement
All datasets generated for this study are included in the article/supplementary material.
Author Contributions
VV designed the work, drafted the manuscript, and approves for publication of the content and agrees to be accountable for all aspects of the work in ensuring that questions related to the accuracy or integrity of any part of the work are appropriately investigated and resolved.
Funding
Parts of the research discussed in the submitted work was funded by the Research Council Flanders (FWO), University of Antwerp (BOFDOCPRO4), Queen Elisabeth Medical Foundation for Neurosciences, Action on Hearing Loss UK and the DFNA9 patient association De negende van.
Conflict of Interest
The author declares that the research was conducted in the absence of any commercial or financial relationships that could be construed as a potential conflict of interest.
Acknowledgments
The author has contributed and approved the final version of this manuscript. The author would like to thank Olivier Vanderveken for reviewing the manuscript.
References
1. WHO. Priority Medicines for Europe and the World Update Report. (2013). Available online at: http://www.who.int/medicines/areas/priority_medicines/Ch6_21Hearing.pdf (accessed January 26, 2017).
2. Hoppe U, Hesse G. Hearing aids: indications, technology, adaptation, and quality control. GMS Curr Top Otorhinolaryngol Head Neck Surg. (2017) 16:Doc08. doi: 10.3205/cto000147
3. Suen JJ, Marrone N, Han HR, Lin FR, Nieman CL. Translating public health practices: community-based approaches for addressing hearing health care disparities. Semin Hear. (2019) 40:37–48. doi: 10.1055/s-0038-1676782
4. Bond M, Mealing S, Anderson R, Elston J, Weiner G, Taylor RS, et al. The effectiveness and cost-effectiveness of cochlear implants for severe to profound deafness in children and adults: a systematic review and economic model. Health Technol Assess. (2009) 13:1–330. doi: 10.3310/hta13440
5. Bond M, Elston J, Mealing S, Anderson R, Weiner G, Taylor R, et al. Systematic reviews of the effectiveness and cost-effectiveness of multi-channel unilateral cochlear implants for adults. Clin Otolaryngol. (2010) 35:87–96. doi: 10.1111/j.1749-4486.2010.02098.x
6. Vickers D, De Raeve L, Graham J. International survey of cochlear implant candidacy. Cochlear Implants Int. (2016) 17 (Suppl. 1):36–41. doi: 10.1080/14670100.2016.1155809
7. Landsberger DM, Vermeire K, Claes A, Van Rompaey V, Van de Heyning P. Qualities of single electrode stimulation as a function of rate and place of stimulation with a cochlear implant. Ear Hear. (2016) 37:e149–59. doi: 10.1097/AUD.0000000000000250
8. Schilder AG, Chong LY, Ftouh S, Burton MJ. Bilateral versus unilateral hearing aids for bilateral hearing impairment in adults. Cochrane Database Syst Rev. (2017) 12:CD012665. doi: 10.1002/14651858.CD012665.pub2
9. McRackan TR, Fabie JE, Bhenswala PN, Nguyen SA, Dubno JR. General health quality of life instruments underestimate the impact of bilateral cochlear implantation. Otol Neurotol. (2019) 40:745–53. doi: 10.1097/MAO.0000000000002225
10. Rana B, Buchholz JM, Morgan C, Sharma M, Weller T, Konganda SA, et al. Bilateral versus unilateral cochlear implantation in adult listeners: speech-on-speech masking and multitalker localization. Trends Hear. (2017) 21:2331216517722106. doi: 10.1177/2331216517722106
11. Health Quality O. Bilateral cochlear implantation: a health technology assessment. Ont Health Technol Assess Ser. (2018) 18:1–139.
12. Anovum. EuroTrak Study in the Netherlands Performed by ANOVUM on Behalf of the European Hearing Impairment Manufacturer's Association and Reported in 2016 (2016). Available online at: https://www.ehima.com/wp-content/uploads/2017/11/EuroTrak_2016_Netherlands.pptx
13. Anovum. EuroTrak Study in Belgium Performed by ANOVUM on Behalf of the European Hearing Impairment Manufacturer's Association and Reported in 2017 (2017). Available online at: https://www.ehima.com/wp-content/uploads/2017/12/EuroTrak-Belgium_2017_Final-Report.pdf
14. Hofkens-Van den Brandt A, Mertens G, Gilles A, Fransen E, Lassaletta L, Gavilan J, et al. Auditory performances in older and younger adult cochlear implant recipients: use of the HEARRING registry. Otol Neurotol. (2019) 40:e787–95. doi: 10.1097/MAO.0000000000002333
15. Sorkin DL, Buchman CA. Cochlear implant access in six developed countries. Otol Neurotol. (2016) 37:e161–4. doi: 10.1097/MAO.0000000000000946
16. D'Haese PSC, De Bodt M, Van Rompaey V, Van de Heyning P. Awareness of hearing loss in older adults: results of a survey conducted in 500 subjects across 5 European countries as a basis for an online awareness campaign. Inquiry. (2018) 55:46958018759421. doi: 10.1177/0046958018759421
17. D'Haese PSC, Van Rompaey V, De Bodt M, Van de Heyning P. The knowledge and beliefs regarding practical aspects of cochlear implants: a study of otorhinolaryngologists in a secondary setting in a multi-country study. Cochlear Implants Int. (2018) 19:14–21. doi: 10.1080/14670100.2017.1385141
18. D'Haese PSC, Van Rompaey V, De Bodt M, Van de Heyning P. Severe hearing loss in the aging population poses a global public health challenge. How can we better realize the benefits of cochlear implantation to mitigate this crisis? Front Public Health. (2019) 7:227. doi: 10.3389/fpubh.2019.00227
19. Van Kerschaver E, Boudewyns AN, Declau F, Van de Heyning PH, Wuyts FL. Socio-demographic determinants of hearing impairment studied in 103,835 term babies. Eur J Public Health. (2013) 23:55–60. doi: 10.1093/eurpub/cks010
20. National Institute for Health and Care Excellence. Hearing Loss in Adults: Assessment and Management. London: NICE (Clinical guideline [NG98]) (2018). Available online at: https://www.nice.org.uk/guidance/ng98 (accessed April 06, 2020).
21. Lin FR, Metter EJ, O'Brien RJ, Resnick SM, Zonderman AB, Ferrucci L. Hearing loss and incident dementia. Arch Neurol. (2011) 68:214–20. doi: 10.1001/archneurol.2010.362
22. Gallacher J, Ilubaera V, Ben-Shlomo Y, Bayer A, Fish M, Babisch W, et al. Auditory threshold, phonologic demand, incident dementia. Neurology. (2012) 79:1583–90. doi: 10.1212/WNL.0b013e31826e263d
23. Gurgel RK, Ward PD, Schwartz S, Norton MC, Foster NL, Tschanz JT. Relationship of hearing loss and dementia: a prospective, population-based study. Otol Neurotol. (2014) 35:775–81. doi: 10.1097/MAO.0000000000000313
24. Deal JA, Betz J, Yaffe K, Harris T, Purchase-Helzner E, Satterfield S, et al. Hearing impairment and incident dementia and cognitive decline in older adults: the health ABC study. J Gerontol A Biol Sci Med Sci. (2017) 72:703–9. doi: 10.1093/gerona/glw069
25. Alzheimer's Disease International. The global impact of dementia, an analysis of prevalence, incidence, cost and trends. In: World Alzheimer Report. London: Alzheimer's Disease International (2015).
26. World Health Organization. Dementia: Fact Sheets. (2016). Available online at: http://www.who.int/mediacentre/factsheets/fs362/en/ (accessed March 1, 2017).
27. Dobbels B, Peetermans O, Boon B, Mertens G, Van de Heyning P, Van Rompaey V. Impact of bilateral vestibulopathy on spatial and nonspatial cognition: a systematic review. Ear Hear. (2019) 40:757–65. doi: 10.1097/AUD.0000000000000679
28. De Belder J, Matthysen S, Claes AJ, Mertens G, Van de Heyning P, Van Rompaey V. Does otovestibular loss in the autosomal dominant disorder DFNA9 have an impact of on cognition? A systematic review. Front Neurosci. (2017) 11:735. doi: 10.3389/fnins.2017.00735
29. Dobbels B, Mertens G, Gilles A, Claes A, Moyaert J, van de Berg R, et al. Cognitive function in acquired bilateral vestibulopathy: a cross-sectional study on cognition, hearing, vestibular loss. Front Neurosci. (2019) 13:340. doi: 10.3389/fnins.2019.00340
30. Pineault K, Pearson D, Wei E, Kamil R, Klatt B, Agrawal Y. Association between saccule and semicircular canal impairments and cognitive performance among vestibular patients. Ear Hear. (2019). doi: 10.1097/AUD.0000000000000795. [Epub ahead of print].
31. Zhu RT, Van Rompaey V, Ward BK, Van de Berg R, Van de Heyning P, Sharon JD. The interrelations between different causes of dizziness: a conceptual framework for understanding vestibular disorders. Ann Otol Rhinol Laryngol. (2019) 128:869–78. doi: 10.1177/0003489419845014
32. Danneels M, Van Hecke R, Keppler H, Degeest S, Cambier D, van de Berg R, et al. Psychometric properties of cognitive-motor dual-task studies with the aim of developing a test protocol for persons with vestibular disorders: a systematic review. Ear Hear. (2020) 41:3–16. doi: 10.1097/AUD.0000000000000748
33. Kraus KS, Mitra S, Jimenez Z, Hinduja S, Ding D, Jiang H, et al. Noise trauma impairs neurogenesis in the rat hippocampus. Neuroscience. (2010) 167:1216–26. doi: 10.1016/j.neuroscience.2010.02.071
34. Liu L, Shen P, He T, Chang Y, Shi L, Tao S, et al. Noise induced hearing loss impairs spatial learning/memory and hippocampal neurogenesis in mice. Sci Rep. (2016) 6:20374. doi: 10.1038/srep20374
35. Liu L, Xuan C, Shen P, He T, Chang Y, Shi L, et al. Hippocampal mechanisms underlying impairment in spatial learning long after establishment of noise-induced hearing loss in CBA mice. Front Syst Neurosci. (2018) 12:35. doi: 10.3389/fnsys.2018.00035
36. Lin FR, Ferrucci L, An Y, Goh JO, Doshi J, Metter EJ, et al. Association of hearing impairment with brain volume changes in older adults. Neuroimage. (2014) 90:84–92. doi: 10.1016/j.neuroimage.2013.12.059
37. Alfandari D, Vriend C, Heslenfeld DJ, Versfeld NJ, Kramer SE, Zekveld AA. Brain volume differences associated with hearing impairment in adults. Trends Hear. (2018) 22:2331216518763689. doi: 10.1177/2331216518763689
38. Livingston G, Sommerlad A, Orgeta V, Costafreda SG, Huntley J, Ames D, et al. Dementia prevention, intervention, care. Lancet. (2017) 390:2673–734. doi: 10.1016/S0140-6736(17)31363-6
39. Mamo SK, Reed NS, Price C, Occhipinti D, Pletnikova A, Lin FR, et al. Hearing loss treatment in older adults with cognitive impairment: a systematic review. J Speech Lang Hear Res. (2018) 61:2589–603. doi: 10.1044/2018_JSLHR-H-18-0077
40. van Hooren SA, Anteunis LJ, Valentijn SA, Bosma H, Ponds RW, Jolles J, et al. Does cognitive function in older adults with hearing impairment improve by hearing aid use? Int J Audiol. (2005) 44:265–71. doi: 10.1080/14992020500060370
41. Acar B, Yurekli MF, Babademez MA, Karabulut H, Karasen RM. Effects of hearing aids on cognitive functions and depressive signs in elderly people. Arch Gerontol Geriatr. (2011) 52:250–2. doi: 10.1016/j.archger.2010.04.013
42. Magalhaes R, Iorio MC. Evaluation of participation restriction and cognitive processes in the elderly before and after the audiologic rehabilitation. J Soc Bras Fonoaudiol. (2011) 23:51–6. doi: 10.1590/S2179-64912011000100012
43. Peracino A. Hearing loss and dementia in the aging population. Audiol Neurootol. (2014) 19 (Suppl. 1):6–9. doi: 10.1159/000371595
44. Amieva H, Ouvrard C, Giulioli C, Meillon C, Rullier L, Dartigues JF. Self-reported hearing loss, hearing aids, and cognitive decline in elderly adults: a 25-year study. J Am Geriatr Soc. (2015) 63:2099–104. doi: 10.1111/jgs.13649
45. Meister H, Rahlmann S, Walger M, Margolf-Hackl S, Kiessling J. Hearing aid fitting in older persons with hearing impairment: the influence of cognitive function, age, and hearing loss on hearing aid benefit. Clin Interv Aging. (2015) 10:435–43. doi: 10.2147/CIA.S77096
46. Castiglione A, Benatti A, Velardita C, Favaro D, Padoan E, Severi D, et al. Aging, cognitive decline and hearing loss: effects of auditory rehabilitation and training with hearing aids and cochlear implants on cognitive function and depression among older adults. Audiol Neurootol. (2016) 21 (Suppl. 1):21–8. doi: 10.1159/000448350
47. Rigters SC, van der Schroeff MP, Papageorgiou G, Baatenburg de Jong RJ, Goedegebure A. Progression of hearing loss in the aging population: repeated auditory measurements in the rotterdam study. Audiol Neurootol. (2018) 23:290–7. doi: 10.1159/000492203
48. Jayakody DMP, Friedland PL, Nel E, Martins RN, Atlas MD, Sohrabi HR. Impact of cochlear implantation on cognitive functions of older adults: pilot test results. Otol Neurotol. (2017) 38:e289–95. doi: 10.1097/MAO.0000000000001502
49. Claes AJ, de Backer S, Van de Heyning P, Gilles A, Van Rompaey V, Mertens G. Postoperative cognitive dysfunction after cochlear implantation. Eur Arch Otorhinolaryngol. (2018) 275:1419–27. doi: 10.1007/s00405-018-4976-6
50. Claes AJ, Van de Heyning P, Gilles A, Hofkens-Van den Brandt A, Van Rompaey V, Mertens G. Impaired cognitive functioning in cochlear implant recipients over the age of 55 years: a cross-sectional study using the repeatable battery for the assessment of neuropsychological status for hearing-impaired individuals (RBANS-H). Front Neurosci. (2018) 12:580. doi: 10.3389/fnins.2018.00580
51. Claes AJ, Van de Heyning P, Gilles A, Van Rompaey V, Mertens G. Cognitive outcomes after cochlear implantation in older adults: a systematic review. Cochlear Implants Int. (2018) 19:239–54. doi: 10.1080/14670100.2018.1484328
52. Hillyer J, Elkins E, Hazlewood C, Watson SD, Arenberg JG, Parbery-Clark A. Assessing cognitive abilities in high-performing cochlear implant users. Front Neurosci. (2018) 12:1056. doi: 10.3389/fnins.2018.01056
53. Claes AJ, Van de Heyning P, Gilles A, Van Rompaey V, Mertens G. Cognitive performance of severely hearing-impaired older adults before and after cochlear implantation: preliminary results of a prospective, longitudinal cohort study using the RBANS-H. Otol Neurotol. (2018) 39:e765–73. doi: 10.1097/MAO.0000000000001936
54. Jayakody DMP, Friedland PL, Nel E, Martins RN, Atlas MD, Sohrabi HR. Impact of cochlear implantation on cognitive functions of older adults: pilot test results. Otol Neurotol. (2018) 39:514–5. doi: 10.1097/MAO.0000000000001768
55. Kramer S, Vasil KJ, Adunka OF, Pisoni DB, Moberly AC. Cognitive functions in adult cochlear implant users, cochlear implant candidates, and normal-hearing listeners. Laryngoscope Investig Otolaryngol. (2018) 3:304–10. doi: 10.1002/lio2.172
56. Andries E, Van Rompaey V, Van de Heyning P, Mertens G. Commentary: assessing cognitive abilities in high-performing cochlear implant users. Front Neurosci. (2019) 13:564. doi: 10.3389/fnins.2019.00564
57. Hillyer J, Parada JC, Parbery-Clark A. Assessing performance on the Montreal Cognitive Assessment (MoCA) in experienced cochlear implant users: use of alternative scoring guidelines. Neuropsychol Dev Cogn B Aging Neuropsychol Cogn. (2020) 27:397–411. doi: 10.1080/13825585.2019.1624684
58. Moberly AC, Doerfer K, Harris MS. Does Cochlear Implantation Improve Cognitive Function? Laryngoscope. (2019) 129:2208–9. doi: 10.1002/lary.28140
59. Ohemeng KK, Eisen MD, Kost K, Parham K. Cochlear implantation in the old with cognitive decline. Ear Nose Throat J. (2019) 98:480–1. doi: 10.1177/0145561319846112
60. Sarant J, Harris D, Busby P, Maruff P, Schembri A, Dowell R, et al. The effect of cochlear implants on cognitive function in older adults: initial baseline and 18-month follow up results for a prospective international longitudinal study. Front Neurosci. (2019) 13:789. doi: 10.3389/fnins.2019.00789
61. Sorrentino T, Donati G, Nassif N, Pasini S, Redaelli de Zinis LO. Cognitive function and quality of life in older adult patients with cochlear implants. Int J Audiol. (2019) 59:1–7. doi: 10.1080/14992027.2019.1696993
62. Dawes P, Emsley R, Cruickshanks KJ, Moore DR, Fortnum H, Edmondson-Jones M, et al. Hearing loss and cognition: the role of hearing AIDS, social isolation and depression. PLoS ONE. (2015) 10:e0119616. doi: 10.1371/journal.pone.0119616
63. Gates GA, Cooper JC Jr, Kannel WB, Miller NJ. Hearing in the elderly: the Framingham cohort, 1983-1985. Part I Basic audiometric test results. Ear Hear. (1990) 11:247–56. doi: 10.1097/00003446-199008000-00001
64. Cruickshanks KJ, Wiley TL, Tweed TS, Klein BE, Klein R, Mares-Perlman JA, et al. Prevalence of hearing loss in older adults in Beaver Dam, Wisconsin. The epidemiology of hearing loss study. Am J Epidemiol. (1998) 148:879–86. doi: 10.1093/oxfordjournals.aje.a009713
65. Megighian D, Savastano M, Salvador L, Frigo A, Bolzan M. Audiometric and epidemiological analysis of elderly in the Veneto region. Gerontology. (2000) 46:199–204. doi: 10.1159/000022160
66. Chao TK, Chen TH. Predictive model for progression of hearing loss: meta-analysis of multi-state outcome. J Eval Clin Pract. (2009) 15:32–40. doi: 10.1111/j.1365-2753.2008.00949.x
67. Bielefeld EC, Tanaka C, Chen GD, Henderson D. Age-related hearing loss: is it a preventable condition? Hear Res. (2010) 264:98–107. doi: 10.1016/j.heares.2009.09.001
68. Frisina RD, Frisina DR. Physiological and neurobiological bases of age-related hearing loss: biotherapeutic implications. Am J Audiol. (2013) 22:299–302. doi: 10.1044/1059-0889(2013/13-0003)
69. Ren HM, Ren J, Liu W. Recognition and control of the progression of age-related hearing loss. Rejuvenation Res. (2013) 16:475–86. doi: 10.1089/rej.2013.1435
70. Van Camp G, Smith RJH. Hereditary Hearing Loss Homepage. (1992). Available online at: https://hereditaryhearingloss.org (accessed September 24, 2019).
71. Bommakanti K, Iyer JS, Stankovic KM. Cochlear histopathology in human genetic hearing loss: state of the science and future prospects. Hear Res. (2019) 382:107785. doi: 10.1016/j.heares.2019.107785
72. Bom SJ, Kemperman MH, Huygen PL, Luijendijk MW, Cremers CW. Cross-sectional analysis of hearing threshold in relation to age in a large family with cochleovestibular impairment thoroughly genotyped for DFNA9/COCH. Ann Otol Rhinol Laryngol. (2003) 112:280–6. doi: 10.1177/000348940311200316
73. Chen DY, Chai YC, Yang T, Wu H. Clinical characterization of a novel COCH mutation G87V in a Chinese DFNA9 family. Int J Pediatr Otorhinolaryngol. (2013) 77:1711–5. doi: 10.1016/j.ijporl.2013.07.031
74. JanssensdeVarebeke S, Topsakal V, Van Camp G, Van Rompaey V. A systematic review of hearing and vestibular function in carriers of the Pro51Ser mutation in the COCH gene. Eur Arch Otorhinolaryngol. (2019) 276:1251–62. doi: 10.1007/s00405-019-05322-x
75. Bom SJ, Kunst HP, Huygen PL, Cremers FP, Cremers CW. Non-syndromal autosomal dominant hearing impairment: ongoing phenotypical characterization of genotypes. Br J Audiol. (1999) 33:335–48. doi: 10.3109/03005369909090117
76. Bom SJH, Kemperman MH, De Kok YJM, Huygen PLM, Verhagen WIM, Cremers FPM, et al. Progressive cochleovestibular impairment caused by a point mutation in the COCH gene at DFNA9. Laryngoscope. (1999) 109:1525–30. doi: 10.1097/00005537-199909000-00031
77. Bischoff AM, Huygen PL, Kemperman MH, Pennings RJ, Bom SJ, Verhagen WI, et al. Vestibular deterioration precedes hearing deterioration in the P51S COCH mutation (DFNA9): an analysis in 74 mutation carriers. Otol Neurotol. (2005) 26:918–25. doi: 10.1097/01.mao.0000185048.84641.e3
78. Kemperman MH, De Leenheer EM, Huygen PL, van Duijnhoven G, Morton CC, Robertson NG, et al. Audiometric, vestibular, genetic aspects of a DFNA9 family with a G88E COCH mutation. Otol Neurotol. (2005) 26:926–33. doi: 10.1097/01.mao.0000185062.12458.87
79. Robertson NG, Jones SM, Sivakumaran TA, Giersch ABS, Jurado SA, Call LM, et al. A targeted Coch missense mutation: a knock-in mouse model for DFNA9 late-onset hearing loss and vestibular dysfunction. Hum Mol Genet. (2008) 17:3426–34. doi: 10.1093/hmg/ddn236
80. de Varebeke SP, Termote B, Van Camp G, Govaerts PJ, Schepers S, Cox T, et al. Focal sclerosis of semicircular canals with severe DFNA9 hearing impairment caused by a P51S COCH-mutation: is there a link? Otol Neurotol. (2014) 35:1077–86. doi: 10.1097/MAO.0000000000000283
81. Van Rompaey V, De Belder F, Parizel P, Van de Heyning P. Semicircular canal fibrosis as a biomarker for lateral semicircular canal function loss. Front Neurol. (2016) 7:43. doi: 10.3389/fneur.2016.00043
82. Lucieer F, Duijn S, Van Rompaey V, Perez Fornos A, Guinand N, Guyot JP, et al. Full spectrum of reported symptoms of bilateral vestibulopathy needs further investigation-a systematic review. Front Neurol. (2018) 9:352. doi: 10.3389/fneur.2018.00352
83. Ihtijarevic B, Janssens de Varebeke S, Mertens G, Dekeyzer S, Van de Heyning P, Van Rompaey V. Correlations between vestibular function and imaging of the semicircular canals in DFNA9 patients. Front Neurol. (2019) 10:1341. doi: 10.3389/fneur.2019.01341
84. Praetorius M, Brough DE, Hsu C, Plinkert PK, Pfannenstiel SC, Staecker H. Adenoviral vectors for improved gene delivery to the inner ear. Hear Res. (2009) 248:31–8. doi: 10.1016/j.heares.2008.11.009
85. Omichi R, Shibata SB, Morton CC, Smith RJH. Gene therapy for hearing loss. Hum Mol Genet. (2019) 28:R65–79. doi: 10.1093/hmg/ddz129
86. Ren Y, Landegger LD, Stankovic KM. Gene Therapy for Human Sensorineural Hearing Loss. Front Cell Neurosci. (2019) 13:323. doi: 10.3389/fncel.2019.00323
87. Pan B, Askew C, Galvin A, Heman-Ackah S, Asai Y, Indzhykulian AA, et al. Gene therapy restores auditory and vestibular function in a mouse model of Usher syndrome type 1c. Nat Biotechnol. (2017) 35:264–72. doi: 10.1038/nbt.3801
88. Emptoz A, Michel V, Lelli A, Akil O, Boutet de Monvel J, Lahlou G, et al. Local gene therapy durably restores vestibular function in a mouse model of Usher syndrome type 1G. Proc Natl Acad Sci USA. (2017) 114:9695–700. doi: 10.1073/pnas.1708894114
89. Gao X, Tao Y, Lamas V, Huang M, Yeh WH, Pan B, et al. Treatment of autosomal dominant hearing loss by in vivo delivery of genome editing agents. Nature. (2018) 553:217–21. doi: 10.1038/nature25164
90. Gyorgy B, Nist-Lund C, Pan B, Asai Y, Karavitaki KD, Kleinstiver BP, et al. Allele-specific gene editing prevents deafness in a model of dominant progressive hearing loss. Nat Med. (2019) 25:1123–30. doi: 10.1038/s41591-019-0500-9
91. Urnov F. An ode to gene edits that prevent deafness. Nature. (2018) 553:162–3. doi: 10.1038/d41586-017-08645-z
92. Lentz JJ, Jodelka FM, Hinrich AJ, McCaffrey KE, Farris HE, Spalitta MJ, et al. Rescue of hearing and vestibular function by antisense oligonucleotides in a mouse model of human deafness. Nat Med. (2013) 19:345–50. doi: 10.1038/nm.3106
93. Ponnath A, Depreux FF, Jodelka FM, Rigo F, Farris HE, Hastings ML, et al. Rescue of outer hair cells with antisense oligonucleotides in usher mice is dependent on age of treatment. J Assoc Res Otolaryngol. (2018) 19:1–16. doi: 10.1007/s10162-017-0640-x
94. Calzada AP, Lopez IA, Beltran Parrazal L, Ishiyama A, Ishiyama G. Cochlin expression in vestibular endorgans obtained from patients with Meniere's disease. Cell Tissue Res. (2012) 350:373–84. doi: 10.1007/s00441-012-1481-x
95. Levie C, Moyaert J, JanssensdeVarebeke S, Verdoodt D, Vanderveken OM, Topsakal V, et al. Attitudes of potential participants towards potential gene therapy trials in autosomal dominant progressive sensorineural hearing loss. a hypothetical scenario methodology in DFNA9 patients. In: International Society for Inner Ear Therapies (Hannover) (2019).
Keywords: DFNA9, sensorineural hearing loss, bilateral vestibulopathy, hypothetical scenario, gene therapy
Citation: Van Rompaey V (2020) Making the Case for Research on Disease-Modifying Treatments to Tackle Post-lingual Progressive Sensorineural Hearing Loss. Front. Neurol. 11:290. doi: 10.3389/fneur.2020.00290
Received: 19 February 2020; Accepted: 26 March 2020;
Published: 21 April 2020.
Edited by:
Agnieszka J. Szczepek, Charité—Universitätsmedizin Berlin, GermanyReviewed by:
Heidi Olze, Charité—Universitätsmedizin Berlin, GermanyRichard Charles Dowell, The University of Melbourne, Australia
Copyright © 2020 Van Rompaey. This is an open-access article distributed under the terms of the Creative Commons Attribution License (CC BY). The use, distribution or reproduction in other forums is permitted, provided the original author(s) and the copyright owner(s) are credited and that the original publication in this journal is cited, in accordance with accepted academic practice. No use, distribution or reproduction is permitted which does not comply with these terms.
*Correspondence: Vincent Van Rompaey, dmluY2VudC52YW4ucm9tcGFleSYjeDAwMDQwO3V6YS5iZQ==