- 1Department of Neonatology, Ministry of Education Key Laboratory of Child Development and Disorders, National Clinical Research Center for Child Health and Disorders, China International Science and Technology Cooperation Base of Child Development and Critical Disorders, Children's Hospital of Chongqing Medical University, Chongqing, China
- 2Chongqing Key Laboratory of Pediatrics, Children's Hospital of Chongqing Medical University, Chongqing, China
- 3School of Life Sciences, SouthWest University, Chongqing, China
Background and Objective: There remains an unmet clinical need for markers that predict outcomes in the hypothermia-treated (HT) infants with HIE. The aim of this meta-analysis was to investigate the prognostic accuracy of currently available clinical tests performed in the immediate post-natal period for predicting neurological outcomes between 18 months and 3 years of age in HT near-term and term infants with perinatal asphyxia and HIE.
Methods: A comprehensive review of the Embase, Cochrane library, and PubMed databases was performed to identify studies that evaluated the prognostic value of clinical tests for neurological outcomes in HT near-term and term infants with perinatal asphyxia and hypoxic-ischemic encephalopathy. Pooled sensitivity and specificity with corresponding 95% confidence intervals and area under the receiver operating characteristic (ROC) curve (AUC) were calculated.
Results: Of the 1,144 relevant studies, 26 studies describing four clinical tests conducted in 1458 HT near-term or term infants were included. For predicting an unfavorable neurological outcome, of the imaging techniques, MRI within 2 weeks of birth performed best on sensitivity 0.85 (95% CI 0.79–0.89), specificity 0.72 (95% CI 0.66–0.77), and AUC 0.88; among the neurophysiological tests, multichannel EEG (Electroencephalogram) demonstrated the sensitivity 0.63 (95% CI 0.49–0.76), specificity 0.82 (95% CI 0.70–0.91), and AUC 0.88, and for aEEG (amplitude-integrated electroencephalography) background pattern pooled sensitivity, specificity and AUC were 0.90 (95% CI 0.86–0.94), 0.46 (95% CI 0.42–0.51), and 0.78 whereas for SEPs (Somatosensory evoked potentials), pooled sensitivity and specificity were 0.52 (95% CI 0.34–0.69), 0.76 (95% CI 0.63–0.87), and AUC 0.84, respectively.
Conclusions: In the wake of the era of TH, MRI and neurophysiological tests (aEEG or EEG) were promising predictors of adverse outcomes, while SEPs need high-quality studies to confirm the findings. Continued follow-up of the children and well-designed large prospective studies are essential to determine whether these benefits are maintained in later childhood.
Introduction
Hypoxic-ischemic encephalopathy (HIE) after perinatal asphyxia is the primary cause of death or long-term neurological impairment in infants born at term. Early predictive indicators of neurological outcomes in infants with HIE is essential for making rational clinical decisions. Before the era of therapeutic hypothermia, the prognostic value of MRI (first week) in neonates with HIE has been well-validated. aEEG or EEG recorded within the first 7 days of life in term infants may have potential as a neurophysiologic predicting tests. However, the prognostic value of SEP should be interpreted with caution due to small sample sizes (1).
Therapeutic hypothermia for 72 h provides neuroprotection that significantly improves survival and neurological outcomes in term infants with moderate to severe HIE (2). However, the prognostic capability of these parameters may vary under hypothermic conditions, and there remains an unmet clinical need for markers that predict outcomes in hypothermia-treated (HT) infants (3, 4).
To the authors' knowledge, there are no published meta-analyses that investigate the prognostic capabilities of currently available clinical tests for predicting long-term neurological outcomes in HT term-infants with HIE. Therefore, a meta-analysis was conducted to evaluate the prognostic accuracy of currently available clinical tests performed in the immediate post-natal period for predicting neurological outcomes between 18 months and 3 years of age in HT near-term and term infants with perinatal asphyxia and HIE.
Methods
Search Strtegy
Three review authors (W.L, Q.Y and Z.H) independently searched the PubMed, Cochrane Library, and Embase databases from 2009 to February 2019 using the following keywords “hypoxic-ischemic encephalopathy” AND “newborn” AND “hypothermia”; “neonatal Encephalopathy” AND “newborn” AND “hypothermia” Searches were limited to literature published in the English language.
Inclusion and Exclusion Criteria
Inclusion criteria were: (1)observational prognostic studies that included infants ≥35 weeks of gestation with perinatal asphyxia and HIE diagnosed according to clearly described criteria; (2) that were treated with therapeutic hypothermia; and (3) that underwent neurological follow-up longitudinally until ≥18 months of age, with outcomes defined as good or adverse.
Exclusion criteria were: (1) reviews, letters, abstracts, or editorials; or (2) studies that reported insufficient data, (3) Non-English language, (4) Uncertain follow-up time and Follow-up time is >18 months.
Study Selection
Three review authors (W.L, Q.Y, and Z.H) independently screened the titles and abstracts identified by the search for potentially relevant studies. Texts were retrieved and reviewed to determine which studies met the inclusion criteria. Disagreements about data extraction were resolved by discussion with a third review author (ZH) until consensus was reached.
Data Extraction
Three review authors (W.D, H.W., and Y.F) independently extracted data from the included studies using a data extraction sheet. The following information was recorded: authors, year of publication, number of study subjects, gestational age, birth weight, Apgar score, blood pH, clinical tests (e.g., imaging, neurophysiological, other), neurological tests, and length of follow-up. If both hypothermic and normothermic infants were included in a study, data about the hypothermic infants were extracted separately. According to the review of the studies, we established the MRI subgroup and the aEEG subgroup.
Statistical Analysis
Statistical analyses were conducted with RevMan v5.3 and Meta-Disc 1.4. Three review authors (W.L, Q.Y, and Z.H) examined the quality of the included studies using Quadas 2, which evaluates four key domains, including patient selection, index test, reference standard, and flow and timing and independently extracted individual patient data from each of the studies into a predefined database. Personal patient data from all studies were pooled to create 2 × 2 tables and pooled sensitivity and specificity with 95% confidence intervals (CIs) and area under the receiver operating characteristic curve (AUC) were calculated. Heterogeneity between studies was tested with the inconsistency (I2) index and χ2- test. A fixed-effect model was used if there was no evidence of heterogeneity between studies (I2 <50%, P > 0.05); otherwise, a random-effects model was used.
Results
Study Identification
The searches identified 1,144 articles. Following the removal of duplicates, the titles and abstracts of 925 studies were screened, and 82 studies were considered potentially eligible for inclusion in this meta-analysis. Full-text articles were retrieved and reviewed; among these, 11 studies were excluded because they were not published in the English language, 27 studies were excluded due to missing data. Fifteen studies were excluded due to uncertain follow-up time and follow-up time is <18 months, and three studies were not analyzed due to small sample size. Finally, 26 reviews were included in the pooled analyses (Figure 1 and Supplementary Table 1).
Characteristics of Included Studies
The characteristics of the included studies are shown in Tables 1, 2. The 26 eligible studies included 1458 HT near-term or term infants with perinatal asphyxia and HIE and follow up available after 18 months of age. Clinical tests performed in the immediate postnatal period included brain magnetic resonance imaging (MRI), EEG, aEEG, SEPs. Neurological examinations were performed between 18 months and 3 years of age.
Methodological Quality of Included Studies
The risks of bias in the index test and patient selection were low (Figures 2, 3). Sixteen studies (5, 8, 10, 12, 14–21, 25, 27, 28, 30) did not indicate whether the reference standard results were interpreted without knowledge of the results of the index test. For flow and timing, 14 studies (5, 7, 8, 10, 13, 14, 18–20, 22, 24, 26, 29, 30) did not include all patients in the analyses. Overall, most of the included studies did not have a high risk of bias.
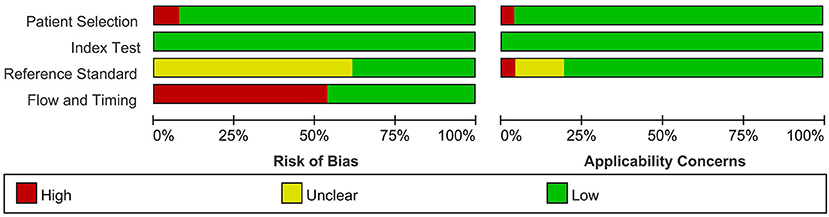
Figure 3. Percentage of the studies with risk of bias and applicability concerns in the different domains of QUADAS-2.
Prognostic Value of Clinical Tests
The results of the meta-analysis are shown in Table 3 (pooled sensitivities and specificities with confidence intervals) and Figure 4 (forest plots of sensitivity and specificity as calculated from the original reports).
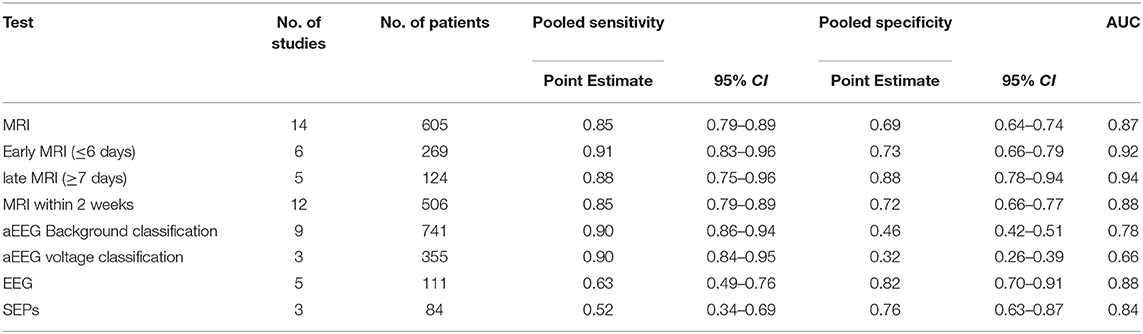
Table 3. Pooled sensitivities and specificities with confidence intervals for tests where pooling was possible.
Imaging: Brain MRI
Fourteen studies (5, 8, 9, 12, 13, 15–17, 19–21, 25, 29, 30) evaluated the prognostic value of brain MRI(I2 = 47.9%, fixed-effect model) for neurological outcomes in HT near-term and term infants with perinatal asphyxia and HIE. Pooled sensitivity and specificity were 0.85 (95% CI 0.79–0.89) and 0.69 (95% CI 0.64–0.74), and AUC was 0.87 for predicting an unfavorable outcome. Early MRI (≤ 6 days from birth) (I2 = 60%, random-effects model) performed best on sensitivity 0.91 (95% CI 0.83–0.96). Late MRI (≥7 days from birth) (I2 = 33%, fixed-effect model) performed best on specificity 0.88 (95% CI 0.78–0.94). MRI within 2 weeks (I2 = 59.5%, random-effects model) of birth performed best on sensitivity 0.85 (95% CI 0.79–0.89), specificity 0.72 (95% CI 0.66–0.77), and AUC 0.88.
Neurophysiological Tests: aEEG, EEG, and SEPs
Nine studies (6, 7, 10, 11, 14, 26–29) evaluated the prognostic value of aEEG tests for neurological outcomes in HT near-term and term infants with perinatal asphyxia and HIE. For aEEG background patterns (I2 = 65.2%, random-effects model), pooled sensitivity and specificity were 0.90 (95% CI 0.86–0.94) and 0.46 (95% CI 0.42–0.51), and AUC was 0.78 for predicting an unfavorable outcome. For aEEG voltage classification (I2 = 36.7, fixed-effect model), pooled sensitivity and specificity were 0.90 (95% CI 0.84–0.95) and 0.32 (95% CI 0.26–0.39), and AUC was 0.66 for predicting an unfavorable outcome.
Multichannel EEG (I2 = 17.7%, fixed-effect model) demonstrated the sensitivity 0.63 (95% CI 0.49–0.76), specificity 0.82 (95% CI 0.70–0.91), and AUC 0.88. Three studies (16, 23, 24) evaluated the prognostic value of SEPs for neurological outcomes in HT near-term and term infants with perinatal asphyxia and HIE. For SEPs (I2 =57.9%, random-effects model), pooled sensitivity and specificity were 0.52 (95% CI 0.34–0.69) and 0.76 (95% CI 0.63–0.87) for predicting an unfavorable outcome, and AUC 0.84.
Discussion
HIE after perinatal asphyxia is a significant cause of mortality and morbidity in newborns, accounting for approximately 20% of cerebral palsy cases (31, 32). Evaluating long-term neurological outcomes based on clinical evaluations in the immediate post-natal period can be challenging. However, a reliable, evidence-based prognosis is essential for parental counseling regarding possible long-term sequelae. Hypothermia is generally accepted as the safest method for reducing neurological morbidity and mortality in infants with perinatal asphyxia and HIE (33, 34). Although the literature is replete with studies evaluating novel but relatively unknown test modalities, to ensure clinical relevance. The present review focused on tests that are widely used in clinical practice and did not attempt to provide an overview of all available procedures (1).
Current MRI injury scoring systems published in the literature include the Barkovich, the National Institutes for Child Health and Development (NICHD) and Rutherford systems (5, 9, 35). Each scoring system has some limitations. The previous does not explicitly address posterior limb of the internal capsule injury, whereas the latter two systems do not include diffusion-weighted imaging (DWI) (25), and Clinicians often evaluate the neonatal brain MRI as a whole test rather than specific brain MRI components. So, we considered parenchymal (gray or white matter) abnormalities on T1, T2, and diffuse weighted images to define an abnormal MRI. In the systematic review, brain MRI predicted an unfavorable neurological outcome in HT infants with perinatal asphyxia and HIE with a sensitivity of 0.85. Accordingly, in the TOBY trial, the accuracy of prediction of death or disability to 18 months of age by MRI was 0.84 (0.74–0.94) in HT infants and 0.81 (0.71–0.91) in a normothermic group (5). Evidence suggests that therapeutic hypothermia without affecting the overall predictive value of MRI as a marker of neurological impairment (5, 13).
In the era of predates hypothermia treatment, late MRI (8–30 days) had higher sensitivity but lower specificity than early MRI (1–7 days) (36). However, the current literature does not provide detailed individual data on the time of neonatal brain MRI in HT (37). In the present review, late MRI predicted an unfavorable neurological outcome with a sensitivity and specificity of 0.88 and 0.88, respectively, whereas early MRI showed less specificity of 0.73. However, Charon et al. reported that the specificity of MRI for predicting death or disability to 18 months of age in HT infants with HIE was 96.3% in the first week and 89.3% in the second week (18). The discrepancy between our findings and those reported by Charon et al. might be explained by the various abnormal findings and the thresholds for the index tests. Results from the present meta-analysis indicate that within 2 weeks of birth correctly predict neurological outcomes at 18 months of age in HT infants with HIE (pooled sensitivity, 0.85 [95% CI, 0.79–0.89].
Deep gray matter lactate/N-acetyl aspartate (Lac/NAA) peak/area ratio is the most quantitative biomarker for prediction of neurodevelopmental outcomes in magnetic resonance spectroscopy (38). However, the equipment is not widely used in clinical practice, and based on limited available studies, the validity of the results has not been quantified in previous reviews of the literature.
aEEG is the commonly used neurophysiological tests for assessment of HIE severity, for monitoring improvement over time, and for predicting neurological outcomes in infants (39). aEEG can be performed at the bedside, and background patterns and voltage classification have been considered an early predictor of neurological outcomes in HT infants with HIE. In normothermic infants, a persistently abnormal aEEG between 6 and 24 h of age is considered predictive of adverse outcomes (6, 40). However, the predictive value of aEEG for subsequent neurological impairment is altered by hypothermia. In one study, the positive prognostic value of an abnormal aEEG increased from 6 to 48 h of age in HT term infants with HIE. This shift in prognostic accuracy may be explained, at least in part, by the neuroprotective effects of therapeutic hypothermia (41). In the present review, aEEG background pattern predicted an unfavorable neurological outcome with a sensitivity and specificity of 0.90 and 0.46, respectively, and an AUC of 0.78, while aEEG voltage classification was less predictive [sensitivity 0.90 [95% CI 0.84–0.95]; specificity 0.32 [95% CI 0.26–0.39], AUC 0.66]. Possible explanations are that the sedative drugs and anticonvulsants that are commonly administered to HT infants have prolonged half-lives and increased plasma levels compared to normothermic conditions, causing voltage fluctuations on the EEG signal (12). In accordance with our findings, Shany et al. reported that background pattern was more sensitive than voltage classification for predicting neurological outcomes in infants with HIE, although assessment of background pattern may be more subjective (42).
Multichannel EEG is the gold standard for assessment of the severity of HIE and for monitoring improvement over time (39). In our review, EEG predicted subsequent neurological impairment in HT infants with HIE with a sensitivity of 0.63 (95% CI, 0.49–0.76), specificity of 0.82 (95% CI, 0.70–0.91), and an AUC of 0.88, yielding a higher specificity than aEEG. However, EEG is a relatively complex technique. Technicians are required to site EEG leads and specialists are needed to interpret neurophysiology, but these resources may not be readily available (39). High seizure burden and sleep-wake cycling have been independently associated with poor outcomes in HT infants with HIE (41). Unfortunately, lack of data meant that these parameters could not be examined in the present meta-analysis.
SEPs assess the deep brain structures such as the thalami and brainstem, which are known to be selectively vulnerable to hypoxia and ischemia (43). Several studies had shown that normal SEPs were strongly predictive of a favorable outcome, and absent SEPs were strongly predictive of an unfavorable outcome (44, 45). In our review, SEPs predicted an unfavorable neurological outcome in HT infants with HIE with a sensitivity of 0.52 (95% CI, 0.34–0.69) and a specificity of 0.76 (95% CI, 0.63–0.87). Although SEPs may provide complementary prognostic resources to HIE after perinatal asphyxia, the predictive value of SEPs investigated in this review should be interpreted with caution due to small sample sizes.
Although not explored in this review, we propose that a combination of the above prognostic tests performed would provide the greater prognostic accuracy for predicting long-term neurological outcomes in the HIE infants undergoing HT.
Strengths and Weaknesses
To the authors' knowledge, this is the first meta-analysis to investigate the prognostic value of clinical tests performed in the immediate post-natal period as predictors of adverse neurological outcomes in HT near-term and term infants with perinatal asphyxia and HIE. Importantly, to investigate which HIE infants would have neurological sequelae, this review only included studies with long-term follow-up, whereby infants were followed until ≥18 months of age. In our opinion, outcomes couldn't be accurately assessed in infants younger than 18 months as neurological sequelae usually manifest at ≥12 months of age, and mental and behavioral disabilities might appear even later (46).
This meta-analysis has several limitations. First, the sample size was small. Second, this review was restricted to articles published in the English language, which may have led to an overestimation or underestimation of prognostic reliability. Finally, heterogeneity was low to moderate, possibly due to differences in thresholds for the index test, the severity of HIE and the design of the studies. Subgroup analyses were not performed to investigate the source of this heterogeneity. Well-designed more extensive studies are required to determine an accurate estimate of the value of clinical tests performed in the immediate post-natal period for predicting in HT infants with HIE.
Conclusions
This systematic review and meta-analysis provided insight into the prognostic value of clinical tests performed in the immediate post-natal period as predictors of adverse neurological outcomes in HT near-term and term infants with perinatal asphyxia. MRI and neurophysiological tests (aEEG or EEG) were promising predictors of the adverse outcomes, whereas SEPs need high-quality studies to confirm the findings. Given the heterogeneity in the tests' performance, continued follow-up of the children and well-designed large prospective studies are essential to determine whether these benefits are maintained in later childhood.
Data Availability Statement
All datasets generated for this study are included in the article/Supplementary Material.
Author Contributions
WL, QY, and ZH performed the screening, extraction of data for included studies, and assessed the quality of study. WD, HW, and YF conducted data extraction of included studies. WL supervises the development of concepts, execution of methodology, analysis, and manuscript writing. All authors reviewed and commented the manuscript.
Funding
This study was supported by grants from the Clinical Research Project of Children's Hospital of Chongqing Medical University (YBXM 2019–007).
Conflict of Interest
The authors declare that the research was conducted in the absence of any commercial or financial relationships that could be construed as a potential conflict of interest.
Supplementary Material
The Supplementary Material for this article can be found online at: https://www.frontiersin.org/articles/10.3389/fneur.2020.00133/full#supplementary-material
References
1. van Laerhoven H, de Haan TR, Offringa M, Post B, van der Lee JH. Prognostic tests in term neonates with hypoxic-ischemic encephalopathy: a systematic review. Pediatrics. (2013) 131:88–98. doi: 10.1542/peds.2012-1297
2. Tagin MA, Woolcott CG, Vincer MJ, Whyte RK, Stinson DA. Hypothermia for neonatal hypoxic ischemic encephalopathy: an updated systematic review and meta-analysis. Arch Pediatr Adolesc Med. (2012) 166:558–66. doi: 10.1001/archpediatrics.2011.1772
3. Del Río R, Ochoa C, Alarcon A, Arnáez J, Blanco D, García-Alix A. Amplitude integrated electroencephalogram as a prognostic tool in neonates with hypoxic-ischemic encephalopathy: a systematic review. PLoS ONE. (2016) 11:e0165744. doi: 10.1371/journal.pone.0165744
4. Edwards AD, Brocklehurst P, Gunn AJ, Halliday H, Juszczak E, Levene M, et al. Neurological outcomes at 18 months of age after moderate hypothermia for perinatal hypoxic ischaemic encephalopathy: synthesis and meta-analysis of trial data. BMJ. (2010) 340:c363. doi: 10.1136/bmj.c363
5. Rutherford M, Ramenghi LA, Edwards AD, Brocklehurst P, Halliday H, Levene M, et al. Assessment of brain tissue injury after moderate hypothermia in neonates with hypoxic-ischaemic encephalopathy: a nested substudy of a randomised controlled trial. Lancet Neurol. (2010) 9:39–45. doi: 10.1016/S1474-4422(09)70295-9
6. Thoresen M, Hellström-Westas L, Liu X, de Vries LS. Effect of hypothermia on amplitude-integrated electroencephalogram in infants with asphyxia. Pediatrics. (2010) 126:e131–9. doi: 10.1542/peds.2009-2938
7. Shankaran S, Pappas A, McDonald SA, Laptook AR, Bara R, Ehrenkranz RA, et al. Predictive value of an early amplitude integrated electroencephalogram and neurologic examination. Pediatrics. (2011) 128:e112–20. doi: 10.1542/peds.2010-2036
8. Takenouchi T, Rubens EO, Yap VL, Ross G, Engel M, Perlman JM. Delayed onset of sleep-wake cycling with favorable outcome in hypothermic-treated neonates with encephalopathy. J Pediatr. (2011) 159:232–7. doi: 10.1016/j.jpeds.2011.01.006
9. Shankaran S, Barnes PD, Hintz SR, Laptook AR, Zaterka-Baxter KM, McDonald SA, et al. Brain injury following trial of hypothermia for neonatal hypoxic-ischaemic encephalopathy. Arch Dis Child Fetal Neonatal Ed. (2012) 97:F398–404. doi: 10.1136/archdischild-2011-301524
10. Cseko AJ, Bangó M, Lakatos P, Kárdási J, Pusztai L, Szabó M. Accuracy of amplitude-integrated electroencephalography in the prediction of neurodevelopmental outcome in asphyxiated infants receiving hypothermia treatment. Acta Paediatr. (2013) 102:707–11. doi: 10.1111/apa.12226
11. Lemmers PM, Zwanenburg RJ, Benders MJ, de Vries LS, Groenendaal F, van Bel F, et al. Cerebral oxygenation and brain activity after perinatal asphyxia: does hypothermia change their prognostic value. Pediatr Res. (2013) 74:180–5. doi: 10.1038/pr.2013.84
12. Li J, Funato M, Tamai H, Wada H, Nishihara M, Iwamoto H, et al. Predictors of neurological outcome in cooled neonates. Pediatr Int. (2013) 55:169–76. doi: 10.1111/ped.12008
13. Chalak LF, DuPont TL, Sánchez PJ, Lucke A, Heyne RJ, Morriss MC, et al. Neurodevelopmental outcomes after hypothermia therapy in the era of Bayley-III. J Perinatol. (2014) 34:629–33. doi: 10.1038/jp.2014.67
14. Azzopardi D. Predictive value of the amplitude integrated EEG in infants with hypoxic ischaemic encephalopathy: data from a randomised trial of therapeutic hypothermia. Arch Dis Child Fetal Neonatal Ed. (2014) 99:F80–2. doi: 10.1136/archdischild-2013-303710
15. Del Balzo F, Maiolo S, Papoff P, Giannini L, Moretti C, Properzi E, et al. Electroencephalogram and magnetic resonance imaging comparison as a predicting factor for neurodevelopmental outcome in hypoxic ischemic encephalopathy infant treated with hypothermia. Pediatr Rep. (2014) 6:5532. doi: 10.4081/pr.2014.5532
16. Garfinkle J, Sant'Anna GM, Rosenblatt B, Majnemer A, Wintermark P, Shevell MI. Somatosensory evoked potentials in neonates with hypoxic-ischemic encephalopathy treated with hypothermia. Eur J Paediatr Neurol. (2015) 19:423–8. doi: 10.1016/j.ejpn.2015.03.001
17. Alderliesten T, de Vries LS, Khalil Y, van Haastert IC, Benders MJ, Koopman-Esseboom C, et al. Therapeutic hypothermia modifies perinatal asphyxia-induced changes of the corpus callosum and outcome in neonates. PLoS ONE. (2015) 10:e0123230. doi: 10.1371/journal.pone.0123230
18. Charon V, Proisy M, Bretaudeau G, Bruneau B, Pladys P, Beuchée A, et al. Early MRI in neonatal hypoxic-ischaemic encephalopathy treated with hypothermia: prognostic role at 2-year follow-up. Eur J Radiol. (2016) 85:1366–74. doi: 10.1016/j.ejrad.2016.05.005
19. Weeke LC, Boylan GB, Pressler RM, Hallberg B, Blennow M, Toet MC, et al. Role of EEG background activity, seizure burden and MRI in predicting neurodevelopmental outcome in full-term infants with hypoxic-ischaemic encephalopathy in the era of therapeutic hypothermia. Eur J Paediatr Neurol. (2016) 20:855–64. doi: 10.1016/j.ejpn.2016.06.003
20. Chiang MC, Lien R, Chu SM, Yang PH, Lin JJ, Hsu JF, et al. Serum lactate, brain magnetic resonance imaging and outcome of neonatal hypoxic ischemic encephalopathy after therapeutic hypothermia. Pediatr Neonatol. (2016) 57:35–40. doi: 10.1016/j.pedneo.2015.04.008
21. Heursen EM, Zuazo Ojeda A, Benavente Fernández I, Jimenez Gómez G, Campuzano Fernández-Colima R, Paz-Expósito J, et al. Prognostic value of the apparent diffusion coefficient in newborns with hypoxic-ischaemic encephalopathy treated with therapeutic hypothermia. Neonatology. (2017) 112:67–72. doi: 10.1159/000456707
22. Ahearne CE, Chang RY, Walsh BH, Boylan GB, Murray DM. Cord blood IL-16 is associated with 3-year neurodevelopmental outcomes in perinatal asphyxia and hypoxic-ischaemic encephalopathy. Dev Neurosci. (2017) 39:59–65. doi: 10.1159/000471508
23. Cainelli E, Trevisanuto D, Cavallin F, Manara R, Suppiej A. Evoked potentials predict psychomotor development in neonates with normal MRI after hypothermia for hypoxic-ischemic encephalopathy. Clin Neurophysiol. (2018) 129:1300–6. doi: 10.1016/j.clinph.2018.03.043
24. Nevalainen P, Lauronen L, Metsäranta M, Lönnqvist T, Ahtola E, Vanhatalo S. Neonatal somatosensory evoked potentials persist during hypothermia. Acta Paediatr. (2017) 106:912–7. doi: 10.1111/apa.13813
25. Trivedi SB, Vesoulis ZA, Rao R, Liao SM, Shimony JS, McKinstry RC, et al. A validated clinical MRI injury scoring system in neonatal hypoxic-ischemic encephalopathy. Pediatr Radiol. (2017) 47:1491–9. doi: 10.1007/s00247-017-3893-y
26. Skranes JH, Løhaugen G, Schumacher EM, Osredkar D, Server A, Cowan FM, et al. Amplitude-integrated electroencephalography improves the identification of infants with encephalopathy for therapeutic hypothermia and predicts neurodevelopmental outcomes at 2 years of age. J Pediatr. (2017) 187:34–42. doi: 10.1016/j.jpeds.2017.04.041
27. Weeke LC, Vilan A, Toet MC, van Haastert IC, de Vries LS, Groenendaal F. A comparison of the thompson encephalopathy score and amplitude-integrated electroencephalography in infants with perinatal asphyxia and therapeutic hypothermia. Neonatology. (2017) 112:24–9. doi: 10.1159/000455819
28. Liu X, Jary S, Cowan F, Thoresen M. Reduced infancy and childhood epilepsy following hypothermia-treated neonatal encephalopathy. Epilepsia. (2017) 58:1902–11. doi: 10.1111/epi.13914
29. Barta H, Jermendy A, Kolossvary M, Kozak LR, Lakatos A, Meder U, et al. Prognostic value of early, conventional proton magnetic resonance spectroscopy in cooled asphyxiated infants. BMC Pediatr. (2018) 18:302. doi: 10.1186/s12887-018-1269-6
30. De Wispelaere LA, Ouwehand S, Olsthoorn M, Govaert P, Smit LS, de Jonge RC, et al. Electroencephalography and brain magnetic resonance imaging in asphyxia comparing cooled and non-cooled infants. Eur J Paediatr Neurol. (2019) 23:181–90. doi: 10.1016/j.ejpn.2018.09.001
31. Nelson KB. Neonatal encephalopathy: etiology and outcome. Dev Med Child Neurol. (2005) 47:292. doi: 10.1017/S0012162205000563
32. Badawi N, Felix JF, Kurinczuk JJ, Dixon G, Watson L, Keogh JM, et al. Cerebral palsy following term newborn encephalopathy: a population-based study. Dev Med Child Neurol. (2005) 47:293–8. doi: 10.1017/S0012162205000575
33. Gunn AJ, Gluckman PD, Gunn TR. Selective head cooling in newborn infants after perinatal asphyxia: a safety study. Pediatrics. (1998) 102(4 Pt 1):885–92. doi: 10.1542/peds.102.4.885
34. Shankaran S, Laptook A, Wright LL, Ehrenkranz RA, Donovan EF, Fanaroff AA, et al. Whole-body hypothermia for neonatal encephalopathy: animal observations as a basis for a randomized, controlled pilot study in term infants. Pediatrics. (2002) 110(2 Pt 1):377–85. doi: 10.1542/peds.110.2.377
35. Barkovich AJ, Hajnal BL, Vigneron D, Sola A, Partridge JC, Allen F, et al. Prediction of neuromotor outcome in perinatal asphyxia: evaluation of MR scoring systems. AJNR Am J Neuroradiol. (1998) 19:143–9.
36. Thayyil S, Chandrasekaran M, Taylor A, Bainbridge A, Cady EB, Chong WK, et al. Cerebral magnetic resonance biomarkers in neonatal encephalopathy: a meta-analysis. Pediatrics. (2010) 125:e382–95. doi: 10.1542/peds.2009-1046
37. Sánchez Fernández I, Morales-Quezada JL, Law S, Kim P. Prognostic value of brain magnetic resonance imaging in neonatal hypoxic-ischemic encephalopathy: a meta-analysis. J Child Neurol. (2017) 32:1065–73. doi: 10.1177/0883073817726681
38. Degraeuwe PLj, Jaspers GJ, Robertson NJ, Kessels AG. Magnetic resonance spectroscopy as a prognostic marker in neonatal hypoxic-ischemic encephalopathy: a study protocol for an individual patient data meta-analysis. Syst Rev. (2013) 2:96. doi: 10.1186/2046-4053-2-96
39. Martinello K, Hart AR, Yap S, Mitra S, Robertson NJ. Management and investigation of neonatal encephalopathy: 2017 update. Arch Dis Child Fetal Neonatal Ed. (2017) 102:F346–58. doi: 10.1136/archdischild-2015-309639
40. Hellström-Westas L, Rosén I, Svenningsen NW. Predictive value of early continuous amplitude integrated EEG recordings on outcome after severe birth asphyxia in full term infants. Arch Dis Child Fetal Neonatal Ed. (1995) 72:F34–8. doi: 10.1136/fn.72.1.F34
41. Chandrasekaran M, Chaban B, Montaldo P, Thayyil S. Predictive value of amplitude-integrated EEG (aEEG) after rescue hypothermic neuroprotection for hypoxic ischemic encephalopathy: a meta-analysis. J Perinatol. (2017) 37:684–9. doi: 10.1038/jp.2017.14
42. Shany E, Goldstein E, Khvatskin S, Friger MD, Heiman N, Goldstein M, et al. Predictive value of amplitude-integrated electroencephalography pattern and voltage in asphyxiated term infants. Pediatr Neurol. (2006) 35:335–42. doi: 10.1016/j.pediatrneurol.2006.06.007
43. Gunn AJ, Bennet L. Fetal hypoxia insults and patterns of brain injury: insights from animal models. Clin Perinatol. (2009) 36:579–93. doi: 10.1016/j.clp.2009.06.007
44. Swarte RM, Cherian PJ, Lequin M, Visser GH, Govaert P. Somatosensory evoked potentials are of additional prognostic value in certain patterns of brain injury in term birth asphyxia. Clin Neurophysiol. (2012) 123:1631–8. doi: 10.1016/j.clinph.2011.12.009
45. Suppiej A, Cappellari A, Franzoi M, Traverso A, Ermani M, Zanardo V. Bilateral loss of cortical somatosensory evoked potential at birth predicts cerebral palsy in term and near-term newborns. Early Hum Dev. (2010) 86:93–8. doi: 10.1016/j.earlhumdev.2010.01.024
Keywords: therapeutic hypothermia, hypoxic-ischemic encephalopathy, neonates, clinical test, prognosis
Citation: Liu W, Yang Q, Wei H, Dong W, Fan Y and Hua Z (2020) Prognostic Value of Clinical Tests in Neonates With Hypoxic-Ischemic Encephalopathy Treated With Therapeutic Hypothermia: A Systematic Review and Meta-Analysis. Front. Neurol. 11:133. doi: 10.3389/fneur.2020.00133
Received: 21 August 2019; Accepted: 05 February 2020;
Published: 25 February 2020.
Edited by:
Deirdre M. Murray, University College Cork, IrelandReviewed by:
Maurizio Elia, Oasi Research Institute (IRCCS), ItalyRyan J. Felling, Johns Hopkins University, United States
Copyright © 2020 Liu, Yang, Wei, Dong, Fan and Hua. This is an open-access article distributed under the terms of the Creative Commons Attribution License (CC BY). The use, distribution or reproduction in other forums is permitted, provided the original author(s) and the copyright owner(s) are credited and that the original publication in this journal is cited, in accordance with accepted academic practice. No use, distribution or reproduction is permitted which does not comply with these terms.
*Correspondence: Ziyu Hua, h_ziyu@163.com
†These authors have contributed equally to this work and share first authorship