- 1Neurosurgery Unit, University Hospital of Parma, Parma, Italy
- 2Emergency Neurosurgery Unit, Azienda USL-IRCCS di Reggio Emilia, Reggio Emilia, Italy
- 3Division of Neurosurgery, Addenbrooke's Hospital, University of Cambridge, Cambridge, United Kingdom
- 4NIHR Global Health Research Group on Neurotrauma, University of Cambridge, Cambridge, United Kingdom
- 5Department of Neurosurgery, Humanitas University and Research Hospital, Milan, Italy
- 6Department of Neurosurgery, University Hospital of Larissa, University of Thessaly, Larissa, Greece
- 7Division of Neurological Surgery, Department of Surgery, College of Medicine, University College Hospital, University of Ibadan, Ibadan, Nigeria
Cranioplasty (CP) after decompressive craniectomy (DC) for trauma is a neurosurgical procedure that aims to restore esthesis, improve cerebrospinal fluid (CSF) dynamics, and provide cerebral protection. In turn, this can facilitate neurological rehabilitation and potentially enhance neurological recovery. However, CP can be associated with significant morbidity. Multiple aspects of CP must be considered to optimize its outcomes. Those aspects range from the intricacies of the surgical dissection/reconstruction during the procedure of CP, the types of materials used for the reconstruction, as well as the timing of the CP in relation to the DC. This article is a narrative mini-review that discusses the current evidence base and suggests that no consensus has been reached about several issues, such as an agreement on the best material for use in CP, the appropriate timing of CP after DC, and the optimal management of hydrocephalus in patients who need cranial reconstruction. Moreover, the protocol-driven standards of care for traumatic brain injury (TBI) patients in high-resource settings are virtually out of reach for low-income countries, including those pertaining to CP. Thus, there is a need to design appropriate prospective studies to provide context-specific solid recommendations regarding this topic.
Introduction
The operative surgery of therapeutic decompressive craniectomy (DC) for traumatic brain injury (TBI) involves the elevation of a free cranial convexital bone flap that is stored either in vivo (e.g., abdominal or thigh subcutaneous pouches) or in ex vivo mediums (deep freezing and tissue banking) (1, 2). Skull defects can result from direct trauma or postsurgical craniectomy. Varying shapes, sizes, and complexities of the defect can be observed.
Cranioplasty (CP) after DC aims to restore esthesis (3), improve cerebrospinal fluid (CSF) dynamics, and provide cerebral protection. In turn, this can facilitate neurological rehabilitation and potentially enhance neurological recovery (3). Although regarded as a routine neurosurgical procedure, CP can be associated with significant morbidity (4, 5).
This paper is a narrative mini-review rather than a systematic review. Therefore, a full search strategy is not provided; rather, we mainly focused on articles in the English language published in PubMed during the last 15 years.
Surgical Techniques
Although surgically straightforward, CP can lead to intraoperative complications at every operative step (elevation of the scalp flap, dissection of soft tissue from the underlying dura, and filling of the defect with a congruent rigid structure) (6, 7).
Required for flap elevation, the vascular territory of the flap must be kept in mind while performing the incision. Following an inner ellipse of the previous DC-surgery scar could contribute in most cases to the preservation of the vascular perfusion even if an incision outside of the ellipse might be needed in certain settings such as sinking skin flap syndrome (SSFS).
In patients where the skin may not be enough to cover the CP, due to an SSFS or skin lesions or scars, a single- or staged skin expansion procedure should be indicated.
However, most commonly, the operative dissections for CP after DC is performed by just opening the skin incision for the previous DC to develop the scalp flap for the CP.
The primary cranial damage control surgery is executed mainly by means of a unilateral frontotemporoparietal DC. This calls for a thoughtful consideration of the temporalis muscle (8), which is often found shrunken or inferiorly retracted toward its origins and adherent to the overlying scalp flaps and/or the underlying dura. This entails a delicate separation that can result in significant bleeding with a resulting increase in operative time. Other complications are intraoperative dural tears, cortical vascular, and parenchymal injuries, postoperative CSF wound leakage, as well as surgical site infections (SSI).
A number of techniques during the DC can potentially reduce the risks associated with the step of dissection. Some are preemptive techniques, such as the interposition of non-absorbable materials between the dura and the scalp flap during the primary DC and tagging the temporalis muscle with brightly colored, non-absorbable sutures for improved identification (9, 10).
Complications
Routine CP is known to have a higher rate of postoperative complications than other elective cranial procedures (11), which may appear at any point during the clinical course due to various factors both directly and indirectly related to the CP itself.
Walcott et al. reported that previous reoperation, comorbid disease type, presence of a ventriculoperitoneal (VP) shunt, and general cardiovascular risk factors are predictors of complications of CP post-DC after stroke and trauma (12). Additionally, skin flap complications such as dehiscence, ulcers, and necrosis are reported (13) and may be related to the exposure of subjacent tissues, always occur after CP in unilateral craniectomy, and preferentially affect the temporoparietal region. However, no correlation has been found between the biomaterials used and skin complications. Dehiscences occur essentially due to poor preoperative conditions, such as in chronically sunken flaps. Ulcers were always associated with an underlying infection and were rarely observed in craniectomized patients before undergoing CP. Necrosis was ascribed to inadvertent sacrifice of the residual arterial supply after flap reopening or to a venous congestion.
Infection
De Bonis et al. showed a 2.5-fold increased infection risk with a bifrontal CP compared with hemispheric/bihemispheric CP (14), regardless of the bone flap substitution material used. This is due to a longer incision and operative time, less temporalis muscle soft tissue coverage, and possible breaching of frontal sinuses during DC. Polymethylmethacrylate (PMMA) as the CP biomaterial shows significant infection rates when in contact with the nasal sinus mucosa or contaminated material (15). Hydroxyapatite (HA) implants (16) showed the highest incidence of infection (3.8%) in bifrontal defects. Also, a study of patients with titanium CP concluded that bifrontal insertion was one of the most relevant risk factors, with a complication rate of 40% including infections (17).
Following CP infection, the decision to remove a biomaterial is a complex issue and should be addressed in concert with plastic surgeons, especially when poor preoperative conditions of skin flap are observed. Even patients in good clinical conditions are at risk of sudden and/or further deterioration. Although, CP infection is rarely associated with sepsis, it is usually addressed by bone or implant removal until complete healing of the surgical field is achieved.
One possible way to address these issues of infection following CP is the development of new prosthetic biomaterials capable of resisting microbial colonization.
Hydrocephalus (HC)
After DC, the occurrence of ventriculomegaly (VM) or HC is reported with varying incidences (10–45%) mainly due to differences in diagnostic criteria (18–21). The management of HC in patients in need of cranial reconstruction can be challenging and thus is not precisely defined. The debate mainly revolves around the timing of CSF diversion with respect to the CP.
Nasi et al. (22) reported 28.4% occurrence of HC in a series of 130 DC at 6.43 postoperative months. In 91.9% of patients, a ventriculoperitoneal shunt (VPS) was required, 76.4% of which was implanted after CP, 14.7% synchronous with, and only in 8.8% before the cranial reconstruction.
The disappearance of VM after CP is well-documented (23–25), and the postoperative management strategy of an unnecessary VPS placement (26–28) is yet unclear. In patients with a bulging scalp flap and VM, external CSF drainage achieved via ventriculostomy or lumbar drainage could allow an accurate repositioning of CP without brain damage.
The use of programmable shunts for patients dependent on CSF shunt has been effectively proven in various case series (29–31). Nevertheless, in socioeconomic environments with limited resources, a fixed pressure valve remains often the only option.
Material Types
Autologous Bone
With few exceptions, autologous bone remains the most commonly used material to fill cranial defects following DC (7, 32–34). It is biocompatible and quite cost-free. Whenever available, autologous bone thus remains the favored option for filling small- to medium-size defects, as well as even the large cranial defects following DC. It is however more likely to be associated with bone flap resorption (BFR) in the latter. The BFR is a non-linear process, which can result in structural breakdown of the CP requiring reoperation and even bone flap replacement with heterologous materials.
Korhonen et al. reported BFR as a complication occurring at various degrees in up to 90% of patients undergoing autologous CP after DC, in particular in patients younger than 30 years. In any case, it has been observed that postoperative monitoring for BFR required regular clinical follow-up, assessing for mechanical stability rather than routine CT.
Independent risk factors for reoperation were shown to be younger age, shunt dependency, and bone flap fragmentation due to a fracture. Hence, an initial artificial bone substitute implant rather than an autograft could be recommended in all patients with a fragmented flap (35).
Compared with synthetic biomaterials, the use of autologous bone for CP is associated with significantly increased odds of reoperation (36). However, autologous bone does not seem to increase infection rates compared with synthetic material. BFR is the main cause for reoperation overall (35).
Some authors raised the possibility that higher rates of complication in autologous bone graft would be partly explained by bone flap conservation methods. However, a systematic review performed by Corliss et al. found no such statistical evidence (37).
PMMA
PMMA is a very common material for CP, and it can be found used as PMMA liquid or as solid PMMA customized implants. Intraoperatively, liquid PMMA takes from 10 to 20 min to be turned into a moldable viscous paste, which is then applied to the cranial defect (38). This process is an exothermic reaction from which the brain and the meninges need to be shielded. Liquid PMMA is non-absorbable, radiolucent, and inert. Additionally, it can be soaked with antibiotics, making it a good option for patients having failed multiple previous attempts at CP (38) because of SSI. It is both an effective and affordable choice for CP. The abovementioned exothermic reaction, intraoperative preparation, the relative contraindication in pregnancy, toxicity of fumes, as well as the need for artistic skills from the operators are all disadvantages.
On the other hand, solid custom-made PMMA, despite its cost, has a long-standing record, does not require to be prepared intraoperatively, does not cause any exothermic reactions, is easy to contour, is delivered sterile, as well as has a textured surface. To reduce the costs, the use of three-dimensional (3-D) patient-specific customized silicon molds is reported to be filled with less expensive liquid PMMA (39–41).
Polyetheretherketone (PEEK)
PEEK has the advantage of being inert, pliable, and mechanically sound. It requires in-house sterilization and may increase seroma formation.
Punchak et al. (42) showed a trend toward decreased postoperative complication rates of PEEK CPs compared to autologous grafts and showed a stronger trend toward lower failure rates of PEEK grafts compared to titanium grafts. The overall complication rate was shown to be lower with PEEK than with titanium group (43).
Titanium
Titanium can be manufactured as a plate, mesh, or 3-D porous implant and is available with varying stiffness and degrees of openness. Titanium is robust to resist secondary trauma while providing maximal stability of the cranial vault (44).
Titanium CP after DC is associated with better cosmetic and functional outcomes than primary autologous CP without increasing overall healthcare costs (20). Free flap coverage and soft tissue atrophy result in greater risk of titanium mesh exposure (45). The titanium mesh should be well-anchored onto the basi-temporal skull to avoid spontaneous fracture (46).
Most recently, 3-D porous titanium was implemented as a viable alternative. Despite its high cost and limited literature available, 3-D porous titanium shows promising results after a 1-year follow-up (47).
Porous Hydroxyapatite (HA)
Porous HA shows biocompatibility due to its biomimetism and the absence of host immune interactions (48–50) or systemic/local toxicity (51). Composite biomaterials such as scaffolds surface-enriched HA nanoparticle using a poly(trimethylene carbonate) (PTMC) scaffold are shown to have a positive impact on bone generation and repair (45). Bony regeneration rates were reported in two patients having undergone CP at 6 months and 2.5 years, respectively (52). It is an appropriate material for use in large and complex cranial defect reconstruction (53).
A posttraumatic fracture rate of HA prosthesis is reported but, at the same time, HA has the ability to undergo self-repair (16, 53).
A study has tried to address the retention management of infection associated with hydroxyapatite CP (52). The suggestion is that a lower biofilm formation, lower rate of colonization compared to titanium (53), targeted antimicrobial therapy, and a satisfactory area of revascularization allow optimal antibiotic delivery on-site and were all decisive in the possibility of avoiding prosthesis removal.
3-D Prosthesis
Shape is another important factor for a successful CP as an increased congruence between the patient and the implant will lead to a better outcome overall as well as improved aesthetic benefit.
In neurosurgery, 3-D printing can be used to create prosthesis and molds used to reconstruct cranial defects using CT data to obtain the dimension and shape of the repair (54, 55). The cost of equipment, lack of knowledge and training, and introduction of commercial, FDA-approved media for printing are thought to be obstacles to a widespread adoption of neurosurgical 3-D printing usage (55).
CP Following DC in Settings with Limited Resources
Until recently, the low-cost nature of the practice of neurosurgery in resource-limited regions meant that the costly protocol-driven standards of care for TBI in high-resource settings were virtually out of reach for most lower- to middle-income countries (LMICs).
However, it is now being increasingly recognized that when both clinical and radiological signs of a patient are in keeping with raised intracranial pressure (ICP) in TBI, the surgical procedure of DC should no longer be considered a last-tier treatment option. It can, and perhaps should, be performed sooner than later and most pragmatically so in these same low-resource LMICs where the other high-cost means of the non-surgical management of posttraumatic raised ICP are not available (56–59). There is therefore an increasing body of work on the use of DC in damage control surgery TBI from the developing countries.
Additionally, the surgical technique of in situ hinge DC (60–63) instead of the traditional DC has greatly influenced the literature of DC from the LMICs. Hinge DCs, also known as hinge craniotomies, by their nature do not as a rule require salvage CP. This would naturally be expected to be an attractive option as the surgical decompression of choice for raised ICP in the LMICs. There is thus a growing literature on the use of the hinge DC, including modifications of the originally described techniques (64–66) from these regions (Figure 1).
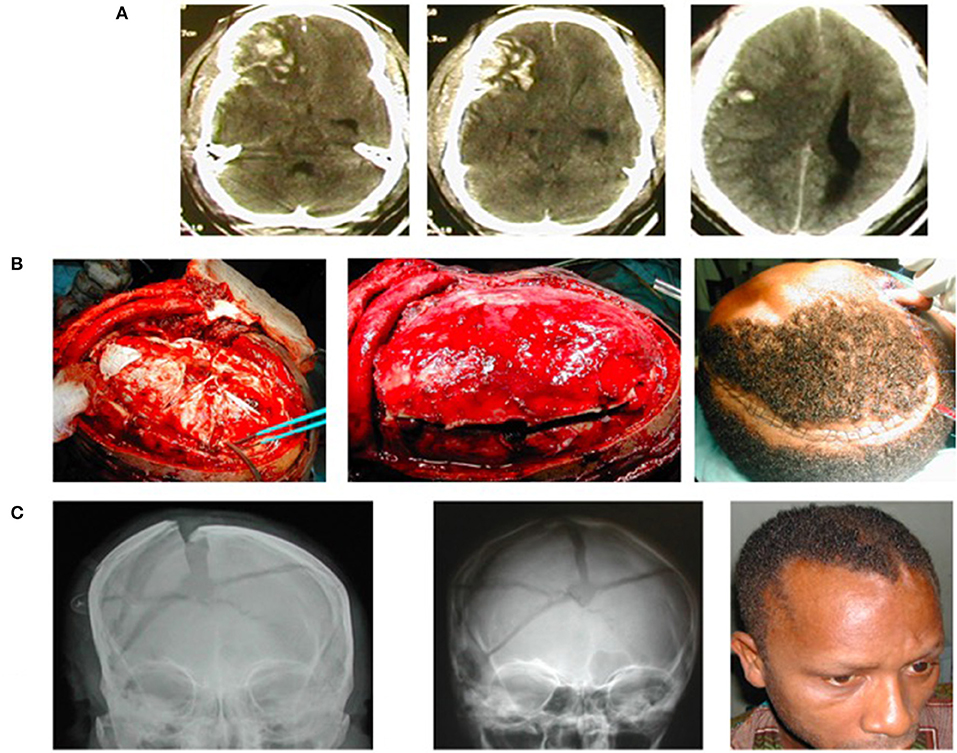
Figure 1. A young man suffered severe traumatic brain injury (TBI), GCS7/15, from road trauma. (A) Cranial CT showed right-sided brain contusions, acute subdural hematoma, subarachnoid hemorrhage, intracerebral hematoma, and bilateral brain swelling that was worse to the right—CT Rotterdam of 6. (B) Intraoperative image of the hinge craniotomy, cruciate durotomy, and evacuation of the extra-axial bleed (to the left); the bone flap returned, floating in situ (middle); and skin closure (to the right). (C) Plain skull X-ray on the first postoperative day showing the elevated/floating bone flap (to the left), bone also revealed comminuted skull fracture; Plain skull X-ray (middle) and clinical picture of the patient (right) 5 weeks postop showing the bone flap spontaneously returned to the rest of the cranium following resolution of the traumatic brain swelling.
CP following DC as yet does not appear to be a major need in the LMICs. But whenever there is need to resort to the traditional DC (e.g., a forbidding massive brain swelling), then the autologous bone flap remains the overwhelming choice, and due to costs, PMMA is the second alternative.
Timing of CP
The appropriate timing of CP after DC in relation to complication rate and outcome has yet to be established. The concept itself of early CP remains ill-defined and refers to time intervals varying from as little as 4 weeks up to as long as 12 weeks (Table 1).
Timing varies according to three pre-CP scenarios encountered, setting the earliest time at which a CP can be performed.
Type 1: The brain is depressed with a significant sinking of the post-DC flap due to a posttraumatic brain atrophy or overdrainage of a ventriculoperitoneal shunt (VPS). Thus, a pre-CP long-lasting CSF diversion should be avoided.
Type 2: The post-craniectomy scalp flap is at the same level as the margins of the cranial vault. The brain should be in the most physiological condition thus at a low risk of observing the development of HC and/or postoperative blood collections.
Type 3: The post-craniectomy scalp flap is over the cranial vault margin due to brain swelling and/or HC/VM. This could well be the worst scenario due to the difficulty of diagnosis and treatment.
Infection was reported to be the highest risk within 14 days of craniectomy, HC within 90 days, and seizure risk after 90 days. Hence, some advocate for an ultra-early CP taking place between 15 and 30 days that would minimize infection, seizure, and autologous flap resorption risks.
In a retrospective cohort study (71), the functional outcome was found to be better for CP performed at the <7 weeks and at 7–12 weeks group compared with the >12 weeks group. Nevertheless, the authors stressed an earlier time to CP should be set as soon as brain edema had normalized so as to have higher chances of a better neurologic outcome and not apparently increased infection rate. At the same time, CP performed at <7 weeks was associated with a significant increase in infection rates when comorbidities, such as diabetes, thromboembolism, and colonization with multidrug resistant (MDR) pathogens, were present (87). Thus, both clinical status and infective status are strong determinants of the outcome of an early CP (<7 weeks) and are of paramount importance in establishing the timing of an early CP.
Conclusions regarding early CP vary widely among different studies (75, 76, 88). Some authors attribute a lower rate of complications to an early CP (67–69, 71, 73, 74), others describe a lower risk of hydrocephalus (34, 70), while no improvement in outcome following early CP was also found (72, 78). Moreover, no impact of timing on outcome (17, 83) or complications (3, 14, 28, 77, 79–82, 84) have been reported. Only two authors (85, 86) associated an early CP with poor outcome, in particular, the highest rate of infection between 0 and 6 months (86). An analysis of literature data suggests a higher rate of complication when CP has been performed between the third and fifth month (Figure 2).
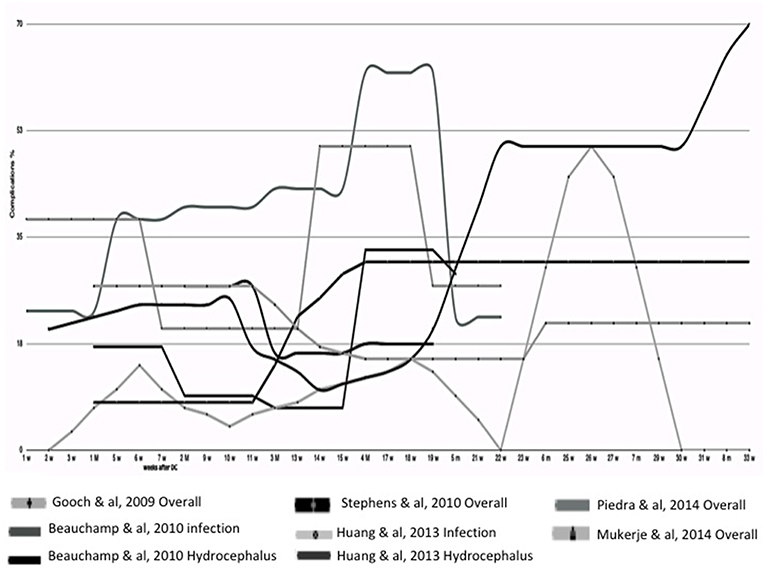
Figure 2. Timing of Cranioplasty. In this graph are compared different clinical courses after cranioplasty analyzed from different papers, where the timing of onset of complication is well-reported. In this partial analysis of literature data, a higher rate of complication is suggested when cranioplasty has been performed between the third and fifth month.
Last reported systematic review and meta-analysis (3) suggested that early CP may lead to even greater improvements. Nevertheless, despite a growing consensus that earlier is better, no more than low-level evidence from retrospective, poorly matched cohort studies (Class IIb, Level C) has been published on this subject.
Conclusion
Despite its therapeutic and cosmetic advantages, CP following DC is not reported to correlate strongly with improved neurologic rehabilitation and outcome.
Different surgical approaches can be used to reduce the surgical complications that may arise at any point of the clinical course among which bifrontal CP is a strong independent risk factor.
Autologous bone is the most commonly used material despite its association with BFR and higher rate of implant failure requiring removal.
A consensus has yet to be reached with regard to the best heterologous material for CP. Porous prostheses may offer promising results despite higher costs.
Standards of care for DC are not applicable in LMICs due to high costs, and thus autologous bone grafts are favored.
Regarding HC, the optimal timing for shunting is yet to be firmly defined. A one-step surgery with CP and CSF shunting and a two-step surgery with or without external CSF drainage are reported as alternatives of management. Finally, CSF shunting without a timely CP should be avoided.
While waiting for results of an ongoing randomized controlled trial (RCT) on early vs. late CP promoted by NIHR Global Health Research Group on Neurotrauma, the timing of reconstructive CP should rather be based on an objective case-by-case assessment of the neurological status of each patient, resolution of brain swelling, and complications associated with large calvarial defects rather than arbitrary time windows (73) and should be performed as soon as brain swelling resolves on CT scan, provided that the patient is not in an infectious state (89).
The authors are aware that the results of future studies may dictate updating many of the recommendations on several aspects of CP after DC contained in this review.
Author Contributions
CI, AK, AA, and L-GR: writing manuscript. CI and AA: analysis of literature. CI and AA: collecting cases. AK and KF: supervision of manuscript.
Funding
The NIHR Global Health Research Group on Neurotrauma was commissioned by the NIHR using Official Development Assistance (ODA) funding (project 16/137/105). The views expressed in this publication are those of the author(s) and not necessarily those of the NHS, National Institute for Health Research or the Department of Health.
Conflict of Interest
CI is a consulent for post market surveillance for Finceramica S.p.A.
The remaining authors declare that the research was conducted in the absence of any commercial or financial relationships that could be construed as a potential conflict of interest.
References
1. Bhaskar IP, Zaw NN, Zheng M, Lee GY. Bone flap storage following craniectomy: a survey of practices in major Australian neurosurgical centres. ANZ J Surg. (2011) 81:137–41. doi: 10.1111/j.1445-2197.2010.05584.x
2. Nakaji P, Holland M. Craniectomy: surgical indications and technique. Oper Tech Neurosurg. (2004) 7:10–5. doi: 10.1053/j.otns.2004.04.006
3. Malcolm JG, Rindler RS, Chu JK, Chokshi F, Grossberg JA, Pradilla G, et al. Early cranioplasty is associated with greater neurological improvement: a systematic review and meta-analysis. Neurosurgery. (2018) 82:278–88. doi: 10.1093/neuros/nyx182
4. Robles LA, Cuevas-Solórzano A. Massive brain swelling and death after cranioplasty: a systematic review. World Neurosurg. (2018) 111:99–108. doi: 10.1016/j.wneu.2017.12.061
5. Manfiotto M, Mottolese C, Szathmari A, Beuriat PA, Klein O, Vinchon M, et al. Decompressive craniectomy and CSF disorders in children. Childs Nerv Syst. (2017) 33:1751–7. doi: 10.1007/s00381-017-3542-7
6. Honeybul S, Ho KM. Cranioplasty: morbidity and failure. Br J Neurosurg. (2016) 30:523–8. doi: 10.1080/02688697.2016.1187259
7. Kim JS, Park IS, Kim SK, Park H, Kang DH, Lee CH, et al. Analysis of the risk factors affecting the surgical site infection after cranioplasty following decompressive craniectomy. Korean J Neurotrauma. (2015) 11:100–5. doi: 10.13004/kjnt.2015.11.2.100
8. Honeybul S. Management of the temporal muscle during cranioplasty: technical note. J Neurosurg Pediatr. (2016) 17:701–4. doi: 10.3171/2015.11.PEDS15556
9. Lee CH, Cho DS, Jin SC, Kim SH, Park DB. Usefulness of silicone elastomer sheet as another option of adhesion preventive material during craniectomies. Clin Neurol Neurosurg. (2007) 109:667–71. doi: 10.1016/j.clineuro.2007.05.015
10. Missori P, Polli FM, Peschillo S, D'Avella E, Paolini S, Miscusi M. Double dural patch in decompressive craniectomy to preserve the temporal muscle: technical note. Surg Neurol. (2008) 70:437–9. discussion: 9. doi: 10.1016/j.surneu.2007.03.029
11. Kim JS, Cheong JH, Ryu JI, Kim JM, Kim CH. Bone flap resorption following cranioplasty after decompressive craniectomy: preliminary report. Korean J Neurotrauma. (2015) 11:1–5. doi: 10.13004/kjnt.2015.11.1.1
12. Walcott BP, Kwon CS, Sheth SA, Fehnel CR, Koffie RM, Asaad WF, et al. Predictors of cranioplasty complications in stroke and trauma patients. J Neurosurg. (2013) 118:757–62. doi: 10.3171/2013.1.JNS121626
13. Di Rienzo A, Pangrazi PP, Riccio M, Colasanti R, Ghetti I, Iacoangeli M. Skin flap complications after decompressive craniectomy and cranioplasty: proposal of classification and treatment options. Surg Neurol Int. (2016) 7(Suppl 28):S737–45. doi: 10.4103/2152-7806.193724
14. De Bonis P, Frassanito P, Mangiola P, Nucci CG, Anile C, Pompucci A. Cranial repair: how complicated is filling a “hole”? J Neurotrauma. (2012) 29:1071–6. doi: 10.1089/neu.2011.2116
15. Marchac D, Greensmith A. Long-term experience with methylmethacrylate cranioplasty in craniofacial surgery. J Plast Reconstr Aesthet Surg. (2008) 61:744–52. discussion: 753. doi: 10.1016/j.bjps.2007.10.055
16. Stefini R, Esposito G, Zanotti B, Iaccarino C, Fontanella MM, Servadei F. Use of “custom made” porous hydroxyapatite implants for cranioplasty: postoperative analysis of complications in 1549 patients. Surg Neurol Int. (2013) 4:12. doi: 10.4103/2152-7806.106290
17. Mukherjee S, Thakur B, Haq I, Hettige S, Martin AJ. Complications of titanium cranioplasty–a retrospective analysis of 174 patients. Acta Neurochir. (2014) 156:989–98. discussion: 998. doi: 10.1007/s00701-014-2024-x
18. De Bonis P, Pompucci A, Mangiola A, Rigante L, Anile C. Post-traumatic hydrocephalus after decompressive craniectomy: an underestimated risk factor. J Neurotrauma. (2010) 27:1965–70. doi: 10.1089/neu.2010.1425
19. Cooper DJ, Rosenfeld JV, Murray L, Arabi YM, Davies AR, D'Urso P, et al. Decompressive craniectomy in diffuse traumatic brain injury. N Engl J Med. (2011) 364:1493–502. doi: 10.1056/NEJMoa1102077
20. Honeybul S, Ho KM. Incidence and risk factors for post-traumatic hydrocephalus following decompressive craniectomy for intractable intracranial hypertension and evacuation of mass lesions. J Neurotrauma. (2012) 29:1872–8. doi: 10.1089/neu.2012.2356
21. Takeuchi S, Takasato Y, Masaoka H, Hayakawa T, Yatsushige H, Nagatani K, et al. Hydrocephalus following decompressive craniectomy for ischemic stroke. Acta Neurochir Suppl. (2013) 118:289–91. doi: 10.1007/978-3-7091-1434-6_56
22. Nasi D, Dobran M, Di Rienzo A, Di Somma L, Gladi M, Moriconi E, et al. Decompressive craniectomy for traumatic brain injury: the role of cranioplasty and hydrocephalus on outcome. World Neurosurg. (2018) 116:e543–9. doi: 10.1016/j.wneu.2018.05.028
23. Kakar V, Nagaria J, John Kirkpatrick P. The current status of decompressive craniectomy. Br J Neurosurg. (2009) 23:147–57. doi: 10.1080/02688690902756702
24. Kolias AG, Kirkpatrick PJ, Hutchinson PJ. Decompressive craniectomy: past, present and future. Nat Rev Neurol. (2013) 9:405–15. doi: 10.1038/nrneurol.2013.106
25. Pachatouridis D, Alexiou GA, Zigouris A, Michos E, Drosos D, Fotakopoulos G, et al. Management of hydrocephalus after decompressive craniectomy. Turk Neurosurg. (2014) 24:855–8. doi: 10.5137/1019-5149.JTN.8871-13.1
26. Lee MH, Yang JT, Weng HH, Cheng YK, Lin MH, Su CH, et al. Hydrocephalus following decompressive craniectomy for malignant middle cerebral artery infarction. Clin Neurol Neurosurg. (2012) 114:555–9. doi: 10.1016/j.clineuro.2011.11.027
27. Lin BJ, Chou KN, Feng SW, Ju DT, Ma HI, Hueng DY, et al. Role of post-decompressive hydrocephalus in patients with malignant cerebral infarction. Turk Neurosurg. (2015) 25:742–8. doi: 10.5137/1019-5149.JTN.11345-14.1
28. Tsang AC, Hui VK, Lui WM, Leung GK. Complications of post-craniectomy cranioplasty: risk factor analysis and implications for treatment planning. J Clin Neurosci. (2015) 22:834–7. doi: 10.1016/j.jocn.2014.11.021
29. Oh CH, Park CO, Hyun DK, Park HC, Yoon SH. Comparative study of outcomes between shunting after cranioplasty and in cranioplasty after shunting in large concave flaccid cranial defect with hydrocephalus. J Korean Neurosurg Soc. (2008) 44:211–6. doi: 10.3340/jkns.2008.44.4.211
30. Jung YT, Lee SP, Cho JI. An improved one-stage operation of cranioplasty and ventriculoperitoneal shunt in patient with hydrocephalus and large cranial defect. Korean J Neurotrauma. (2015) 11:93–9. doi: 10.13004/kjnt.2015.11.2.93
31. Li G, Wen L, Zhan RY, Shen F, Yang XF, Fu WM, et al. Cranioplasty for patients developing large cranial defects combined with post-traumatic hydrocephalus after head trauma. Brain Inj. (2008) 22:333–7. doi: 10.1080/02699050801958353
32. Goldstein JA, Paliga JT, Bartlett SP. Cranioplasty: indications and advances. Curr Opin Otolaryngol Head Neck Surg. (2013) 21:400–9. doi: 10.1097/MOO.0b013e328363003e
33. Kim SH, Kang DS, Cheong JH, Kim JH, Song KY, Kong MH. Comparison of complications following cranioplasty using a sterilized autologous bone flap or polymethyl methacrylate. Korean J Neurotrauma. (2017) 13:15–23. doi: 10.13004/kjnt.2017.13.1.15
34. Morton RP, Abecassis IJ, Hanson JF, Barber JK, Chen M, Kelly CM, et al. Timing of cranioplasty: a 10.75-year single-center analysis of 754 patients. J Neurosurg. (2018) 128:1648–52. doi: 10.3171/2016.11.JNS161917
35. Schwarz F, Dünisch P, Walter J, Sakr Y, Kalff R, Ewald C. Cranioplasty after decompressive craniectomy: is there a rationale for an initial artificial bone-substitute implant? A single-center experience after 631 procedures. J Neurosurg. (2016) 124:710–5. doi: 10.3171/2015.4.JNS159
36. Malcolm JG, Rima ZM, Rindler S, Allen JW, Grossberg JA, Faiz GP, et al. Autologous cranioplasty is associated with increased reoperation rate: a systematic review and meta-analysis. World Neurosurg. (2018) 116:80–68. doi: 10.1016/j.wneu.2018.05.009
37. Corliss B, Gooldy T, Vaziri S, Kubilis P, Murad G, Fargen K. Complications after in vivo and ex vivo autologous bone flap storage for cranioplasty: a comparative analysis of the literature. World Neurosurg. (2016) 96:510–5. doi: 10.1016/j.wneu.2016.09.025
38. Hsu VM, Tahiri Y, Wilson AJ, Grady MS, Taylor JA. A preliminary report on the use of antibiotic-impregnated methyl methacrylate in salvage cranioplasty. J Craniofac Surg. (2014) 25:393–6. doi: 10.1097/SCS.0000000000000655
39. Guerrini F, Dallolio V, Grimod G, Cesana C, Vismara D, Franzin AB. It is time to reduce free-hand manipulation: case report of our proposal for an innovative 1-step cranioplasty. World Neurosurg. (2017) 107:1052.e7–10. doi: 10.1016/j.wneu.2017.08.111
40. Cheng CH, Chuang HY, Lin HL, Liu CL, Yao CH. Surgical results of cranioplasty using three-dimensional printing technology. Clin Neurol Neurosurg. (2018) 168:118–23. doi: 10.1016/j.clineuro.2018.03.004
41. Abdel Hay J, Smayra T, Moussa R. Customized polymethylmethacrylate cranioplasty implants using 3-dimensional printed polylactic acid molds: technical note with 2 illustrative cases. World Neurosurg. (2017) 105:971–9 e1. doi: 10.1016/j.wneu.2017.05.007
42. Punchak M, Chung LK, Lagman C, Bui TT, Lazareff J, Rezzadeh K, et al. Outcomes following polyetheretherketone (PEEK) cranioplasty: systematic review and meta-analysis. J Clin Neurosci. (2017) 41:30–5. doi: 10.1016/j.jocn.2017.03.028
43. Zhang Q, Yuan Y, Li X, Sun T, Zhou Y, Yu H, et al. A large multicenter retrospective research on embedded cranioplasty and covered cranioplasty. World Neurosurg. (2018) 112:645–51. doi: 10.1016/j.wneu.2018.01.114
44. Cho HR, Roh TS, Shim KW, Kim YO, Lew DH, Yun IS. Skull reconstruction with custom made three-dimensional titanium implant. Arch Craniofac Surg. (2015) 16:11–6. doi: 10.7181/acfs.2015.16.1.11
45. Maqbool T, Binhammer A, Antonyshyn OM. Risk factors for titanium mesh implant exposure following cranioplasty. J Craniofac Surg. (2018) 29:1181–6. doi: 10.1097/SCS.0000000000004479
46. Jiang Y, Wang YK, Yu M-K. Spontaneous fracture of cranioplastic titanium implants without head trauma in an adult: a case report. Int J Surg Case Rep. (2016) 24:50–3. doi: 10.1016/j.ijscr.2016.04.039
47. Francaviglia N, Maugeri R, Odierna Contino A, Meli F, Fiorenza V, Costantino G, et al. Skull bone defects reconstruction with custom-made titanium graft shaped with electron beam melting technology: preliminary experience in a series of ten patients. Acta Neurochir Suppl. (2017) 124:137–41. doi: 10.1007/978-3-319-39546-3_21
48. Boyde A, Corsi A, Quarto R, Cancedda R, Bianco P. Osteoconduction in large macroporous hydroxyapatite ceramic implants: evidence for a complementary integration and disintegration mechanism. Bone. (1999) 24:579–89. doi: 10.1016/S8756-3282(99)00083-6
49. Marcacci M, Kon E, Zaffagnini S, Giardino R, Rocca M, Corsi A. Reconstruction of extensive long-bone defects in sheep using porous hydroxyapatite sponges. Calcif Tissue Int. (1999) 64:83–90. doi: 10.1007/s002239900583
50. Olmi RM, Ravaglioni A, Krajewski A, Pizzoferrato A. Impianti di idrossiapatite in femori di conigli. Osservazioni istologiche e microradiografiche. Chir Organi Mov. (1984) 69:383–90.
51. Chistolini P, Ruspantini I, Bianco P, Corsi A, Cancedda R, Quarto R. Biomechanical evaluation of cell-loaded and cell-free hydroxyapatite implants for the reconstruction of segmental bone defects. J Mater Sci Mater Med. (1999) 10:739–42. doi: 10.1023/A:1008939524807
52. Ono H, Sase T, Tanaka Y, Takasuna H. Histological assessment of porous custom-made hydroxyapatite implants 6 months and 2.5 years after cranioplasty. Surg Neurol Int. (2017) 8:8. doi: 10.4103/2152-7806.198735
53. Staffa G, Barbanera A, Faiola A, Fricia M, Limoni P, Mottaran R, et al. Custom made bioceramic implants in complex and large cranial reconstruction: a two-year follow-up. J Craniomaxillofac Surg. (2012) 40:65–70. doi: 10.1016/j.jcms.2011.04.014
54. Panesar SS, Belo JTA, D'Souza RN. Feasibility of clinician-facilitated three-dimensional printing of synthetic cranioplasty flaps. World Neurosurg. (2018) 113:628–37. doi: 10.1016/j.wneu.2018.02.111
55. Baskaran V, Strkalj G, Strkalj M, Di Ieva A. Current applications and future perspectives of the use of 3D printing in anatomical training and neurosurgery. Front Neuroanat. (2016) 10:69. doi: 10.3389/fnana.2016.00069
56. Piek J. Decompressive surgery in the treatment of traumatic brain injury. Curr Opin Crit Care. (2002) 8:134–8. doi: 10.1097/00075198-200204000-00008
57. Adeleye AO. Decompressive craniectomy for traumatic brain injury in a developing country: an initial observational study. Indian J Neurotrauma. (2010) 7:41–6. doi: 10.1016/S0973-0508(10)80010-3
58. Sinha S, Raheja A, Garg M, Moorthy S, Agrawal D, Gupta DK, et al. Decompressive craniectomy in traumatic brain injury: a single-center, multivariate analysis of 1,236 patients at a tertiary care hospital in India. Neurol India. (2015) 63:175–83. doi: 10.4103/0028-3886.156277
59. Charry JD, Rubiano AM, Nikas CV, Ortíz JC, Puyana JC, Carney N, et al. Results of early cranial decompression as an initial approach for damage control therapy in severe traumatic brain injury in a hospital with limited resources. J Neurosci Rural Pract. (2016) 7:7–12. doi: 10.4103/0976-3147.172151
60. Goettler CE, Tucci KA. Decreasing the morbidity of decompressive craniectomy: the Tucci flap. J Trauma. (2007) 62:777–8. doi: 10.1097/TA.0b013e31802ee55e
61. Kersh AM, El-Gendye HH. Unilateral Decompressive Craniectomy in Traumatic Brain Injury Patients With Poor Glasgow Coma Scale as an Intraoperative Decision after Evacuation of Focal Lesion; Outcome in 30 Consecutive Cases. (2012). p. 143. Available online at: https://www.medicaljournalofcairouniversity.com
62. Ko K, Segan S. In situ hinge craniectomy. Neurosurgery. (2007) 60:255–8. discussion: 8–9. doi: 10.1227/01.NEU.0000255380.64969.81
63. Schmidt JH, Reyes BJ, Fischer R, Flaherty SK. Use of hinge craniotomy for cerebral decompression. Technical note. J Neurosurg. (2007) 107:678–82. doi: 10.3171/JNS-07/09/0678
64. Adeleye AO, Azeez AL. Decompressive craniectomy bone flap hinged on the temporalis muscle: a new inexpensive use for an old neurosurgical technique. Surg Neurol Int. (2011) 2:150. doi: 10.4103/2152-7806.86227
65. Mracek J, Choc M, Mork J, Vacek P, Mracek Z. Osteoplastic decompressive craniotomy-an alternative to decompressive craniectomy. Acta Neurochir. (2011) 153:2259–63. doi: 10.1007/s00701-011-1132-0
66. Adeleye AO. Clinical and radiologic outcome of a less invasive, low-cost surgical technique of osteoplastic decompressive craniectomy. J Neurol Surg A Cent Eur Neurosurg. (2016) 77:167–75. doi: 10.1055/s-0035-1566115
67. Chun HJ, Yi HJ. Efficacy and safety of early cranioplasty, at least within 1 month. J Craniofac Surg. (2011) 22:203–7. doi: 10.1097/SCS.0b013e3181f753bd
68. Chang V, Hartzfeld P, Langlois M, Mahmood A, Seyfried D. Outcomes of cranial repair after craniectomy. J Neurosurg. (2010) 112:1120–4. doi: 10.3171/2009.6.JNS09133
69. Matsuno A, Tanaka H, Iwamuro H, Takanashi S, Miyawaki S, Nakashima M, et al. Analyses of the factors influencing bone graft infection after delayed cranioplasty. Acta Neurochir. (2006) 148:535–40. discussion: 540. doi: 10.1007/s00701-006-0740-6
70. Waziri A, Fusco D, Mayer SA, McKhann GM 2nd, Conolly ES Jr. Postoperative hydrocephalus in patients undergoing decompressive hemicraniectomy for ischemic or hemorrhagic stroke. Neurosurgery. (2007) 61:489–93. discussion: 493–4. doi: 10.1227/01.NEU.0000290894.85072.37
71. Archavlis E, Carvi Y, Nievas M. The impact of timing of cranioplasty in patients with large cranial defects after decompressive hemicraniectomy. Acta Neurochir. (2012) 154:1055–62. doi: 10.1007/s00701-012-1333-1
72. Schoekler B, Trummer M. Prediction parameters of bone flap resorption following cranioplasty with autologous bone. Clin Neurol Neurosurg. (2014) 120:64–7. doi: 10.1016/j.clineuro.2014.02.014
73. Tasiou A, Vagkopoulos K, Georgiadis I, Brotis AG, Gatos H, Fountas KN. Cranioplasty optimal timing in cases of decompressive craniectomy after severe head injury: a systematic literature review. Interdiscipl Neurosurg. (2014) 1:107–11. doi: 10.1016/j.inat.2014.06.005
74. Qasmi SA, Ghaffar A, Hussain Z, Mushtaq J. Early autologous cranioplasty after decompressive hemi-craniectomy for severe traumatic brain injury. J Pak Med Assoc. (2015) 65:13259.
75. Beauchamp KM, Kashuk J, Moore EE, Bolles G, Rabb C, Seinfeld J, et al. Cranioplasty after post- injury decompressive craniectomy: is timing of the essence? J Trauma. (2010) 69:270–4. doi: 10.1097/TA.0b013e3181e491c2
76. Gooch MR, Gin GE, Kenning TJ, German JW. Complications of cranioplasty following decompressive craniectomy: analysis of 62 cases. Neurosurg Focus. (2009) 26:E9. doi: 10.3171/2009.3.FOCUS0962
77. Song J, Liu M, Mo X, Du H, Huang H, Xu GZ. Beneficial impact of early cranioplasty in patients with decompressive craniectomy: evidence from transcranial Doppler ultrasonography. Acta Neurochir. (2014) 156:193–8. doi: 10.1007/s00701-013-1908-5
78. Huang YH, Lee TC, Yang KY, Liao CC. Is timing of cranioplasty following post traumatic craniectomy related to neurological outcome? Int J Surg. (2013) 11:886–90. doi: 10.1016/j.ijsu.2013.07.013
79. Piedra MP, Ragel BT, Dogan A, Coppa ND, Delashaw JB. Timing of cranioplasty after decompressive craniectomy for ischemic or hemorrhagic stroke. J Neurosurg. (2013) 118:109–14. doi: 10.3171/2012.10.JNS121037
80. Piedra MP, Nemecek AN, Ragel BT. Timing of cranioplasty after decompressive craniectomy for trauma. Surg Neurol Int. (2015) 5:25. doi: 10.4103/2152-7806.127762
81. Sundseth J, Sundseth A, Berg-Johnson J, Sorteberg W, Lindegaard KF. Cranioplasty with autologous cryopreserved bone after decompressive craniectomy: complications and risk factors for developing surgical site infection. Acta Neurochir. (2014) 156:805–11. doi: 10.1007/s00701-013-1992-6
82. Kim H, Sung SO, Kim SJ, Kim SR, Park IS, Jo KW. Analysis of the factors affecting graft infection after cranioplasty. Acta Neurochir. (2013) 155:2171–6. doi: 10.1007/s00701-013-1877-8
83. Coulter IC, Pesic-Smith JD, Cato-Addison WB, Khan SA, Thompson D, Jenkins AJ, et al. Routine but risky: a multi-centre analysis of the outcomes of cranioplasty in the Northeast of England. Acta Neurochir. (2014) 156:1361. doi: 10.1007/s00701-014-2081-1
84. Krause-Titz UR, Warneke N, Freitag-Wolf S, Barth H, Mehdorn HM. Factors influencing the outcome (GOS) in reconstructive cranioplasty. Neurosurg Rev. (2016) 39:133–9. doi: 10.1007/s10143-015-0678-3
85. Schuss P, Vatter H, Marquardt G, Imöhl L, Ulrich CT, Seifert V, et al. Cranioplasty after decompressive craniectomy: the effect of timing on postoperative complications. J Neurotrauma. (2012) 29:1090–5. doi: 10.1089/neu.2011.2176
86. Thavarajah D, De Lacy P, Hussien A, Sugar A. The minimum time for cranioplasty insertion from craniectomy is six months to reduce risk of infection—a case series of 82 patients. Br J Neurosurg. (2012) 26:78–80. doi: 10.3109/02688697.2011.603850
87. Winkler PA, Stummer W, Linke R, Krishnan KG, Tatsch K. Influence of cranioplasty on postural blood flow regulation, cerebrovascular reserve capacity, and cerebral glucose metabolism. J Neurosurg. (2000) 93:53–61. doi: 10.3171/jns.2000.93.1.0053
88. Stephens FL, Mossop CM, Bell RS, Tigno T, Rosner MK, Kumar A, et al. Cranioplasty complications following wartime decompressive craniectomy. Neurosurg Focus. (2010) 28:E3. doi: 10.3171/2010.2.FOCUS1026
Keywords: cranioplasty, decompressive craniectomy, traumatic brain injury, cranial reconstruction, bone flap, posttraumatic hydrocephalus
Citation: Iaccarino C, Kolias AG, Roumy L-G, Fountas K and Adeleye AO (2020) Cranioplasty Following Decompressive Craniectomy. Front. Neurol. 10:1357. doi: 10.3389/fneur.2019.01357
Received: 04 July 2019; Accepted: 09 December 2019;
Published: 29 January 2020.
Edited by:
Elham Rostami, Academic Hospital, SwedenReviewed by:
Maurizio Iacoangeli, Marche Polytechnic University, ItalyDomenico D'Avella, University of Padova, Italy
Copyright © 2020 Iaccarino, Kolias, Roumy, Fountas and Adeleye. This is an open-access article distributed under the terms of the Creative Commons Attribution License (CC BY). The use, distribution or reproduction in other forums is permitted, provided the original author(s) and the copyright owner(s) are credited and that the original publication in this journal is cited, in accordance with accepted academic practice. No use, distribution or reproduction is permitted which does not comply with these terms.
*Correspondence: Corrado Iaccarino, iaccarino.corrado@gmail.com