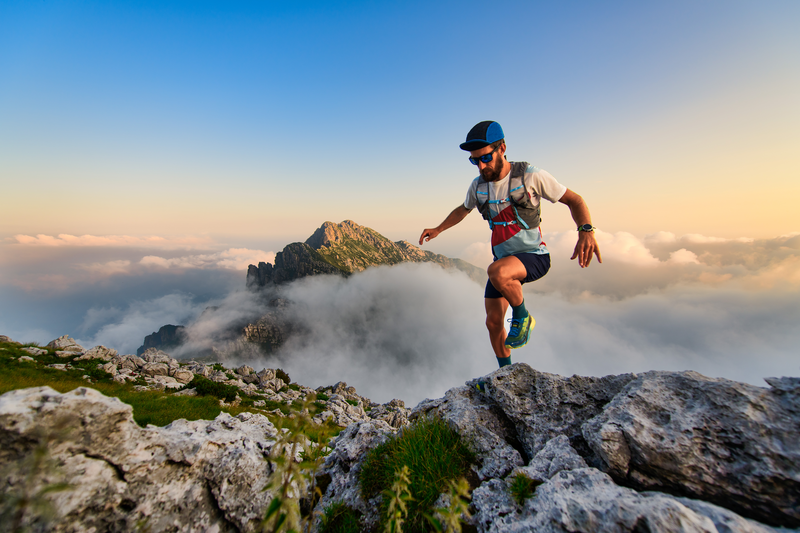
94% of researchers rate our articles as excellent or good
Learn more about the work of our research integrity team to safeguard the quality of each article we publish.
Find out more
ORIGINAL RESEARCH article
Front. Neurol. , 29 November 2019
Sec. Applied Neuroimaging
Volume 10 - 2019 | https://doi.org/10.3389/fneur.2019.01199
This article is part of the Research Topic Brain-image Based Computation for Supporting Clinical Decision in Neurological and Psychiatric Disorders View all 17 articles
Background: This study investigated differences in postural control ability (PCA) and corticoreticulospinal tract (CRT) injury severity according to whiplash in patients with mild traumatic brain injury (mTBI).
Methods: Thirty-one patients with mTBI and 21 healthy control subjects were recruited for this study. The balance error scoring system (BESS) was used for PCA assessment. Based on their whiplash history, the patients were classified into two groups: group A—mTBI with whiplash injury; group B—mTBI without whiplash injury. Fractional anisotropy (FA), apparent diffusion coefficient (ADC), and tract volume (TV) values were estimated for the reconstructed CRTs in all subjects.
Results: Significant differences were observed among the total BESS scores of patient groups A and B and the control group (p < 0.05). The patient group A BESS score was significantly higher than that of patient group B, and that of the patient group B was significantly higher than that of the control group. No significant differences were detected among the FA and ADC values of the CRTs of the two patient groups and the control group (p > 0.05). However, the TV values of the CRT did reveal significant differences; the TV of patient group A was significantly lower than those of patient group B and the control group, and that of patient group B was significantly lower than that of the control group (p < 0.05).
Conclusions: We observed greater CRT injury severity and PCA impairment in mTBI patients with whiplash than in mTBI patients without whiplash. The results indicate that whiplash might lead to a greater level of severity in axonal injuries in mTBI patients.
Traumatic brain injury (TBI) can be caused by a sudden impact or an acceleration–deceleration trauma to the head (1). TBI can be classified as mild, moderate, or severe based on the level of injury severity with mild TBI (mTBI) comprising ~75–90% of all TBI (2, 3). Whiplash is a bony and/or soft tissue injury resulting from acceleration–deceleration energy transfers in the neck (4). Patients with whiplash often complain of cerebral symptoms suggestive of brain injury, and previous studies have reported evidence that indicates the presence of brain injury in patients with whiplash (5–12). Therefore, whiplash has been considered as a pathogenic mechanism of mTBI (13).
Presence of postural control means the subject has the ability to maintain postural orientation in response to perturbations generated from internal or external sources (14). Impairment of postural control ability (PCA) is a common clinical manifestation following mTBI and/or whiplash (14–20). Previous studies have suggested that mTBI due to whiplash might be associated with greater impairment of PCA than that in patients with mTBI due to other causes (16, 17). The corticoreticulospinal tract (CRT), one of the extrapyramiidal motor pathways in the human brain, mainly mediates movements of proximal and axial muscles (21, 22). As a result, the CRT is involved in postural control responses to perturbations generated from internal or external sources (14, 21, 22).
The introduction of diffusion tensor tractography (DTT), a three-dimensional (3D) modeling process that uses diffusion tensor imaging (DTI) data, has enabled 3D reconstruction and estimation of neural tracts, including the CRT (23). The main advantage of DTT over DTI is that the entire neural tract can be evaluated in terms of DTT parameters. Among the various DTT parameters that can be examined, fractional anisotropy (FA), apparent diffusion coefficient (ADC), and tract volume (TV) parameters are the most commonly used (24–26). The FA value, which indicates the degree of directionality of water diffusion, is used to assess the degree of tract directionality whereas the ADC value indicates the magnitude of the water diffusion (24, 25). Therefore, the values of FA and ADC may be used to suggest the microstructural integrity of white matter microstructures, such as axons, myelin, and microtubules (24, 25, 27, 28). In contrast, the TV value indicates the number of voxels (values on a regular grid within a 3D space) that are included in a neural tract (26). A decrement in TV value without a change in the values of FA and ADC of a neural tract indicates a decrement in the number of neural fibers of that tract without a change in the microstructural integrity of the tract (24–26). Therefore, decreases in the values of the FA and TV, as well as increases in the values of the ADC, indicate the presence of neural injury (24–28). A few studies have used DTT to demonstrate CRT injuries in patients with mTBI (29–31). Among these studies, one demonstrated an association between CRT injury and PCA (31). However, no study has reported on the relationship between CRT injury and PCA impairment according to whiplash status in mTBI patients.
For the present study, we hypothesized that PCA impairment and CRT injury would differ according to whiplash presence in mTBI patients. Therefore, we investigated differences in PCA and CRT injuries in mTBI patients with and without a history of whiplash.
A total of 39 consecutive patients with mTBI (18 males, 21 females; mean age 46.20 ± 10.92 years; age range 21–64 years; from January 2016 to December 2018) who visited the rehabilitation department of a university hospital and 20 age- and sex-matched normal control subjects (12 male, 9 female; mean age 45.1 ± 8.93 years; age range 30–64 years) with no history of neurologic/psychiatric disease were recruited for this study (Table 1).
Inclusion criteria for the 39 patients were: (1) more than 2 weeks had elapsed after mTBI onset, (2) at least one of the following: (i) any period of loss of consciousness; (ii) any loss of memory for events immediately before or after the accident; (iii) any alteration in mental state at the time of the accident (e.g., feeling dazed, disoriented, or confused); or (iv) any focal neurological deficit(s) that may or may not be transient but in which the severity of the injury does not exceed the following: loss of consciousness of approximately 30 min or less; after 30 min post-onset, an initial Glasgow Coma Scale of 13–15; and post-traumatic amnesia (PTA) of <24 h (13, 32, 33), (3) no specific lesion observed on brain magnetic resonance imaging (MRI; T1-weighted, T2-weighted, and fluid-attenuated inversion recovery images), (4) age at the time of head trauma >18 years, (5) an abnormal score on the balance error scoring system (BESS) (cut-off score ≥ 14, range: 0–60; a higher value means more severe postural impairment) (34), and (6) no previous history of neurologic/psychiatric disease. Patients were excluded if impairments could have been due to drugs, alcohol, medications, other injuries, treatment for other injuries, other problems, or the result of a penetrating craniocerebral injury.
We divided the patient group into two groups based on the patient's history of whiplash (indicated by the presence of flexion-hyperextension, lateral flexion, or rotation injury of the head and neck following a motor vehicle collision). Nineteen patients were placed in patient group A (mTBI with whiplash injury; injury causes: motor vehicle accidents, 100%) and 20 patients were assigned to patient group B [mTBI without whiplash injury; injury causes: motor vehicle accidents, 17 (85%); falling, 2 (10%); direct head trauma, 1 (5%)] (Table 1). No significant differences in age or sex compositions were detected between patient groups A and B or between the patient and control groups (p > 05).
This was a retrospective study, and the study was carried out in accordance with the recommendations of “The CARE of guidelines” with written informed consent obtained from all subjects. The patient provided written informed consent in accordance with the Declaration of Helsinki, and the study protocol was approved by the Institutional Review Board of the Yeungnam University hospital.
BESS was used for the evaluation of PCA at the time of DTI scanning. Evaluation using BESS has been shown to provide high validity and reliability in normal subjects (35). In addition, BESS can be used to assess the effects of a mild head injury on PCA in the absence of expensive, sophisticated PCA assessment tools (35, 36). Determination of a BESS score requires the subject to stand unsupported with their eyes closed under six conditions involving a combination of two surface types (firm and balance foam) and three stances (double-limb, single-limb, and tandem). In this study, the balance foam (Airex, Sins, Switzerland) was a 6 cm thick piece of medium-density foam (dimensions 50 × 41 cm, density 55 kg/cm3, tensile strength 260 kPa, elongation to breaking 180%). The non-dominant leg was used as the stance limb during the single-leg trials and it was placed in the rear position during tandem stance trials. The preferred leg to use while kicking a ball was defined as the dominant leg. The order of BESS testing conditions of each individual was randomized by subject and session, with each test lasting 20 s (37). For each test, subjects were asked to assume the required stance by positioning their hands on their iliac crests. A test commenced upon eye closure by the subject. Subjects were instructed to try not to lose their balance but, if needed, make any necessary adjustments and return to the testing position as quickly as possible. Test performance was scored by the addition of one error point for each error committed. An error was assigned when any of the following occurred: (1) lifting hands off the iliac crests; (2) opening the eyes; (3) stepping, stumbling, or falling; (4) moving the hip by more than 30 degrees of flexion or abduction; (5) lifting the forefoot or heel; or (6) remaining out of the testing position for more than 5 s (37). The maximum number of errors for a single stance condition was 10. If the subject could not maintain a stance position for longer than 5 s, subjects were assigned the maximum score for that position. The numbers of errors for each trial were added to obtain a total score (0–60; lower scores indicated better balance) (35).
The DTI data obtained for this study were acquired at an average of 14.0 ± 26.2 months after the onset of TBI by using a 1.5 T Philips Gyroscan Intera scanner (Philips, Best, Netherlands) equipped with a Synergy-L sensitivity encoding (SENSE) head coil in order to obtain single-shot, spin-echo, planar-imaging pulse sequences. For each of the 32 non-colinear diffusion sensitizing gradients, 60 contiguous slices were acquired parallel to the anterior commissure–posterior commissure line. Imaging parameters were as follows: acquisition matrix = 96 × 96, reconstructed to matrix = 192 × 192, field of view = 240 × 240 mm, repetition time = 10,398 ms, time to echo = 72 ms, parallel imaging reduction factor (SENSE factor) = 2, EPI factor = 59, and b = 1,000 s/mm2, number of excitations = 1, and thickness = 2.5 mm. Eddy current-induced image distortions were removed by using affine multiscale two-dimensional registration as provided within the Oxford Centre for Functional Magnetic Resonance Imaging of Brain Software Library (www.fmrib.ox.ac.uk/fsl). DTI-Studio software (CMRM; Johns Hopkins Medical Institute, Baltimore, MD, USA) was used for evaluation of the CRT. The CRT was reconstructed based on fibers passing through two regions of interest (ROIs) on the DTI color map. The first ROI was placed at the reticular formation of the medulla. The second ROI was placed at the tegmentum of the midbrain. Termination criteria used for fiber tracking were a fractional anisotropy (FA) value of <0.2 and an angle of <60 degrees (23). The FA, ADC, and TV values for the DTT-reconstructed CRT were obtained for both hemispheres.
Statistical analysis was performed by using SPSS 21.0 for Windows (SPSS, Chicago, IL, USA). One-way analysis of variance with Fisher's least significant difference post hoc test was performed to determine the significance of differences in BESS scores, DTT parameters (FA, ADC, and TV) of the CRT, and age distribution among patient groups A and B and the control group. The chi-squared test was used to examine gender-based differences between patient groups A and B and the control group.
Comparisons of BESS scores and DTT parameter results for the CRTs of the patient and control groups are summarized in Table 2. The mean total BESS score for patient group A was significantly higher than those of patient group B and the control group; furthermore, the mean total BESS score for patient group B was significantly higher than that of the control group (p < 0.05).
Table 2. Clinical data and diffusion tensor imaging parameters for the corticoreticulospinal tracts of patient groups A and B and the control group.
No significant differences were observed in the mean FA and ADC values of the CRTs of patient groups A and B and those of the control group (p > 0.05). However, the mean TV value of the CRT of patient group A was significantly lower than those of patient group B and the control group; moreover, the mean TV value of the CRT of patient group B was significantly lower than that of the control group (p < 0.05) (Figure 1).
Figure 1. Results of diffusion tensor tractography (DTT) of the corticoreticulospinal tract (CRT). (A) T2-weighted brain magnetic resonance images obtained at the time of diffusion tensor imaging in representative subjects of patient group A (51-year-old female), patient group B (49-year-old male), and the control group (56-year-old male); none of the images show an abnormality. (B) Results of DTT of the CRT: the CRTs in the group A patient (green arrows) are narrower on both sides than those of the group B patient (yellow arrows). Moreover, the CRTs of the group B patient (yellow arrows) are narrower on both sides than those of the control group patient.
In this study, we investigated differences in PCA and CRT injury among mTBI patients with and without a history of whiplash injury. The results may be summarized as follows: (1) PCA, as determined by BESS testing, became increasingly worse in the following order: control group, patient group B (mTBI without whiplash), and patient group A (mTBI with whiplash); (2) TV values for the CRTs of the three study groups decreased in the same order, but there were no significant differences in FA and ADC values among the groups.
In the clinical evaluation of a patient's PCA, a high BESS score indicates a high level of impairment of PCA. In this study, the most severe PCA impairment was observed in mTBI patients that experienced whiplash with a lower level of impairment observed in mTBI patients that did not have a history of whiplash. These results indicate that whiplash can produce a more severe effect on the PCA of mTBI patients than that from mTBI alone. This result supports those in previous studies which showed that PCA impairment was greater in mTBI patients with a whiplash injury (16, 17).
In 2011, Nacci et al. assessed shifting of the centers of gravity in whiplash patients with and without mTBI and reported that whiplash patients with mTBI showed greater center of gravity shifting than that of whiplash patients without mTBI (16). In 2016, Gandelman-Marton et al. investigated differences in body weight distribution between mTBI patients with and without whiplash and reported that there was a greater change in body weight distribution in mTBI patients with whiplash than in mTBI patients without whiplash (17).
Among the various DTT parameters that can be examined, FA, ADC, and TV are the most commonly used when evaluating the state of neural tracts in patients with brain injury (24–26). Our results showed a decrement in TV value without a change in the FA and ADC values of the CRT, indicating a decrease in the number of neural fibers in the CRT without a change in its microstructural integrity (24–26). As a result, our TV results indicate the presence of CRT injuries in both patient groups, but the CRT injuries exhibited greater severity in mTBI patients with whiplash than that in those without whiplash. The association of CRT injury with PCA appears to be related to the role of the CRT, which, by mediating movements of proximal and axial muscles, is involved in postural control responses to perturbations generated from internal or external sources (14, 21, 22). Because no brain lesions were detected on conventional brain MRI, traumatic axonal injury was considered the most likely pathogenetic mechanism for the CRT injuries identified in our patient groups (38–40). Based on our results, we conclude that the indirect acceleration–deceleration forces transmitted to the whole brain during whiplash might contribute to increasing the severity of axonal injury to the CRT to a level greater than that in mTBI patients with CRT injuries due to other causes (4, 12).
Since the introduction of DTI, a few studies have demonstrated CRT injuries in patients with mTBI (29–31). Two studies reported on patients with mTBI who had more severe weakness in their proximal joints than in their distal joints, which was indicated to be a consequence of CRT injury (29, 30). Jang et al. (31) evaluated PCA and CRT status in 25 mTBI patients and demonstrated that PCA impairment is associated with the presence of CRT injury. Nonetheless, to our best knowledge, this is the first original study to demonstrate that whiplash can lead to increased axonal injury severity in the CRT of mTBI patients.
However, there are some limitations to this study that should be considered. First, DTT analysis is operator-dependent, and regions of fiber complexity and crossing can prevent full reflection of the underlying fiber architecture (41). Second, because our patients were recruited from those who visited the rehabilitation department of a university hospital, there was a possibility that patients with a distinct level of severity of clinical manifestations might be included in this study; thus, our results may not be comparable to those from a broader population of mTBI patients with whiplash injury. Third, we did not perform a clinical examination of the impairments to cervical muscle function due to whiplash; variation in which can affect the CRT (42). Hence, we could not rule out the effect of cervical muscles on our results. Fourth, we recruited patients with chronic stage mTBI (i.e., more than 2 weeks after onset) and the average duration to DTI after onset was heterogeneous (14.0 ± 26.2 months) after TBI onset. As a result, recovery or degeneration of the CRT injuries during that interval may have influenced the results (43–45).
In conclusion, we investigated the association of whiplash with PCA and CRT injury in mTBI patients and observed that CRT injuries and PCA impairments were more severe in mTBI patients with whiplash than in those without whiplash. Our results suggest that whiplash might lead to more severe axonal injury in mTBI. Further studies on the effect of whiplash on other neural tracts are warranted.
Financial disclosure statements have been obtained, and no conflicts of interest have been reported by the authors or by any individuals in control of the content of this article.
The datasets generated for this study are available on request to the corresponding author.
This study was carried out in accordance with the recommendations of The CARE of guidelines with written informed consent from all subjects. The patient signed a written informed consent in accordance with the Declaration of Helsinki, and the study protocol was approved by the Institutional Review Board of the Yeungnam University hospital.
SJ and YK were involved in manuscript development, funding, data acquisition, and manuscript writing. SY and JK helped in conceiving, designing the study, manuscript development, and manuscript writing.
The authors declare that the research was conducted in the absence of any commercial or financial relationships that could be construed as a potential conflict of interest.
This work was supported by the Medical Research Center Program (2015R1A5A2009124) through the National Research Foundation of Korea (NRF) funded by the Ministry of Science, ICT, and Future Planning.
1. Vos PE, Battistin L, Birbamer G, Gerstenbrand F, Potapov A, Prevec T, et al. EFNS guideline on mild traumatic brain injury: report of an EFNS task force. Eur J Neurol. (2002) 9:207–19. doi: 10.1046/j.1468-1331.2002.00407.x
2. De Kruijk JR, Twijnstra A, Leffers P. Diagnostic criteria and differential diagnosis of mild traumatic brain injury. Brain Inj. (2001) 15:99–106. doi: 10.1080/026990501458335
3. Vos PE, Alekseenko Y, Battistin L, Ehler E, Gerstenbrand F, Muresanu DF, et al. Mild traumatic brain injury. Eur J Neurol. (2012) 19:191–8. doi: 10.1111/j.1468-1331.2011.03581.x
4. Spitzer WO, Skovron ML, Salmi LR, Cassidy JD, Duranceau J, Suissa S, et al. Scientific monograph of the Quebec task force on whiplash-associated disorders: redefining “whiplash” and its management. Spine. (1995) 20:1S−73.
5. Ettlin TM, Kischka U, Reichmann S, Radii EW, Heim S, Wengen D, et al. Cerebral symptoms after whiplash injury of the neck: a prospective clinical and neuropsychological study of whiplash injury. J Neurol Neurosurg Psychiatry. (1992) 55:943–8. doi: 10.1136/jnnp.55.10.943
6. Otte A, Ettlin T, Fierz L, Mueller-Brand J. Parieto-occipital hypoperfusion in late whiplash syndrome: first quantitative SPET study using technetium-99m bicisate (ECD). Eur J Nucl Med. (1996) 23:72–4. doi: 10.1007/BF01736993
7. Otte A, Ettlin TM, Nitzsche EU, Wachter K, Hoegerle S, Simon GH, et al. PET and SPECT in whiplash syndrome: A new approach to a forgotten brain? J Neurol Neurosurg Psychiatry. (1997) 63:368–72. doi: 10.1136/jnnp.63.3.368
8. Otte A, Mueller-Brand J, Nitzsche EU, Wachter K, Ettlin TM. Functional brain imaging in 200 patients after whiplash injury. J Nucl Med. (1997);38:1002.
9. Obermann M, Nebel K, Schumann C, Holle D, Gizewski ER, Maschke M, et al. Gray matter changes related to chronic posttraumatic headache. Neurology. (2009) 73:978–83. doi: 10.1212/WNL.0b013e3181b8791a
10. Vallez Garcia D, Doorduin J, Willemsen AT, Dierckx RA, Otte A. Altered regional cerebral blood flow in chronic whiplash associated disorders. EBioMedicine. (2016) 10:249–57. doi: 10.1016/j.ebiom.2016.07.008
11. Jang SH, Kim SH, Kwon YH. Excessive daytime sleepiness and injury of the ascending reticular activating system following whiplash injury. Front Neurosci. (2018) 12:348. doi: 10.3389/fnins.2018.00348
12. Jang SH, Kwon YH. A review of traumatic axonal injury following whiplash injury as demonstrated by diffusion tensor tractography. Front Neurol. (2018) 9:57. doi: 10.3389/fneur.2018.00057
13. American Congress of Rehabilitation Medicine. Definition of mild traumatic brain injury. J Head Trauma Rehabil. (1993) 8:86–7. doi: 10.1097/00001199-199309000-00010
14. Cavanaugh JT, Guskiewicz KM, Giuliani C, Marshall S, Mercer V, Stergiou N. Detecting altered postural control after cerebral concussion in athletes with normal postural stability. Br J Sports Med. (2005) 39:805–11. doi: 10.1136/bjsm.2004.015909
15. Rubin AM, Woolley SM, Dailey VM, Goebel JA. Postural stability following mild head or whiplash injuries. Am J Otol. (1995) 16:216–21.
16. Nacci A, Ferrazzi M, Berrettini S, Panicucci E, Matteucci J, Bruschini L, et al. Vestibular and stabilometric findings in whiplash injury and minor head trauma. Acta Otorhinolaryngol Ital. (2011) 31:378–89.
17. Gandelman-Marton R, Arlazoroff A, Dvir Z. Postural stability in patients with different types of head and neck trauma in comparison to healthy subjects. Brain Inj. (2016) 30:1612–6. doi: 10.1080/02699052.2016.1199904
18. Lin LF, Liou TH, Hu CJ, Ma HP, Ou JC, Chiang YH, et al. Balance function and sensory integration after mild traumatic brain injury. Brain Inj. (2015) 29:41–6. doi: 10.3109/02699052.2014.955881
19. Guskiewicz KM, Ross SE, Marshall SW. Postural stability and neuropsychological deficits after concussion in collegiate athletes. J Athl Train. (2001) 36:263–73.
20. Guskiewicz KM, Riemann BL, Perrin DH, Nashner LM. Alternative approaches to the assessment of mild head injury in athletes. Med Sci Sports Exerc. (1997) 29:S213–21. doi: 10.1097/00005768-199707001-00003
21. Matsuyama K, Mori F, Nakajima K, Drew T, Aoki M, Mori S. Locomotor role of the corticoreticular-reticulospinal-spinal interneuronal system. Prog Brain Res. (2004) 143:239–49. doi: 10.1016/S0079-6123(03)43024-0
22. Mendoza JE, Foundas AL. Clinical Neuroanatomy: A Neurobehavioral Approach. New York, NY: Springer (2007). 704 p.
23. Yeo SS, Chang MC, Kwon YH, Jung YJ, Jang SH. Corticoreticular pathway in the human brain: diffusion tensor tractography study. Neurosci Lett. (2012) 508:9–12. doi: 10.1016/j.neulet.2011.11.030
24. Mori S, Crain BJ, Chacko VP, van Zijl PC. Three-dimensional tracking of axonal projections in the brain by magnetic resonance imaging. Ann Neurol. (1999) 45:265–9. doi: 10.1002/1531-8249(199902)45:2<265::AID-ANA21>3.0.CO;2-3
25. Assaf Y, Pasternak O. Diffusion tensor imaging (DTI)-based white matter mapping in brain research: a review. J Mol Neurosci. (2008) 34:51–61. doi: 10.1007/s12031-007-0029-0
26. Pagani E, Agosta F, Rocca MA, Caputo D, Filippi M. Voxel-based analysis derived from fractional anisotropy images of white matter volume changes with aging. Neuroimage. (2008) 41:657–67. doi: 10.1016/j.neuroimage.2008.03.021
27. El-Serougy L, Abdel Razek AA, Ezzat A, Eldawoody H, El-Morsy A. Assessment of diffusion tensor imaging metrics in differentiating low-grade from high-grade gliomas. Neuroradiol J. (2016) 29:400–7. doi: 10.1177/1971400916665382
28. Razek A, Batouty N, Fathy W, Bassiouny R. Diffusion tensor imaging of the optic disc in idiopathic intracranial hypertension. Neuroradiology. (2018) 60:1159–66. doi: 10.1007/s00234-018-2078-1
29. Kwon HG, Jang SH. Delayed gait disturbance due to injury of the corticoreticular pathway in a patient with mild traumatic brain injury. Brain Inj. (2014) 28:511–4. doi: 10.3109/02699052.2014.887228
30. Lee HD, Jang SH. Injury of the corticoreticular pathway in patients with mild traumatic brain injury: a diffusion tensor tractography study. Brain Inj. (2015) 29:1219–22. doi: 10.3109/02699052.2015.1045028
31. Jang SH, Kim TH, Kwon YH, Lee MY, Lee HD. Postural instability in patients with injury of corticoreticular pathway following mild traumatic brain injury. Am J Phys Med Rehabil. (2016) 95:580–7. doi: 10.1097/PHM.0000000000000446
32. Alexander MP. Mild traumatic brain injury: pathophysiology, natural history, and clinical management. Neurology. (1995) 45:1253–60. doi: 10.1212/wnl.45.7.1253
33. Ruff RM, Iverson GL, Barth JT, Bush SS, Broshek DK, Policy NAN, et al. Recommendations for diagnosing a mild traumatic brain injury: a national academy of neuropsychology education paper. Arch Clin Neuropsychol. (2009) 24:3–10. doi: 10.1093/arclin/acp006
34. Linens SW, Ross SE, Arnold BL, Gayle R, Pidcoe P. Postural-stability tests that identify individuals with chronic ankle instability. J Athl Train. (2014) 49:15–23. doi: 10.4085/1062-6050-48.6.09
35. Riemann BL, Guskiewicz KM, Shields EW. Relationship between clinical and forceplate measures of postural stability. J Sport Rehabil. (1999) 8:71–82. doi: 10.1123/jsr.8.2.71
36. Lin CC, Roche JL, Steed DP, Musolino MC, Marchetti GF, Furman GR, et al. Test-retest reliability of postural stability on two different foam pads. J Nat Sci. (2015) 1:e43.
37. Riemann BL, Guskiewicz KM. Effects of mild head injury on postural stability as measured through clinical balance testing. J Athl Train. (2000) 35:19–25.
38. Povlishock JT, Christman CW. The pathobiology of traumatically induced axonal injury in animals and humans: a review of current thoughts. J Neurotrauma. (1995) 12:555–64. doi: 10.1089/neu.1995.12.555
39. Shenton ME, Hamoda HM, Schneiderman JS, Bouix S, Pasternak O, Rathi Y, et al. A review of magnetic resonance imaging and diffusion tensor imaging findings in mild traumatic brain injury. Brain Imaging Behav. (2012) 6:137–92. doi: 10.1007/s11682-012-9156-5
40. Jang SH. Traumatic axonal injury in mild traumatic brain injury. In: Gorbunoy N, editor. Traumatic Brain Injury. 1st ed. London: InTech (2018). p. 137–54.
41. Fillard P, Descoteaux M, Goh A, Gouttard S, Jeurissen B, Malcolm J, et al. Quantitative evaluation of 10 tractography algorithms on a realistic diffusion MR phantom. Neuroimage. (2011) 56:220–34. doi: 10.1016/j.neuroimage.2011.01.032
42. Krogh S, Kasch H. Whiplash injury results in sustained impairments of cervical muscle function: a one-year prospective, controlled study. J Rehabil Med. (2018) 50:548–55. doi: 10.2340/16501977-2348
43. Murugavel M, Cubon V, Putukian M, Echemendia R, Cabrera J, Osherson D, et al. A longitudinal diffusion tensor imaging study assessing white matter fiber tracts after sports-related concussion. J Neurotrauma. (2014) 31:1860–71. doi: 10.1089/neu.2014.3368
44. Jang SH, Seo JP. Delayed degeneration of the left fornical crus with verbal memory impairment in a patient with mild traumatic brain injury: a case report. Medicine. (2017) 96:e9219. doi: 10.1097/MD.0000000000009219
Keywords: corticoreticulospinal tract, diffusion tensor tractography, balance error scoring system, postural control ability, whiplash injury, mild traumatic brain injury
Citation: Jang SH, Yeo SS, Kwon JW and Kwon YH (2019) Differences in Corticoreticulospinal Tract Injuries According to Whiplash in Mild Traumatic Brain Injury Patients. Front. Neurol. 10:1199. doi: 10.3389/fneur.2019.01199
Received: 12 March 2019; Accepted: 28 October 2019;
Published: 29 November 2019.
Edited by:
Feng Feng, Peking Union Medical College Hospital (CAMS), ChinaReviewed by:
Gunnel Peterson, Uppsala University, SwedenCopyright © 2019 Jang, Yeo, Kwon and Kwon. This is an open-access article distributed under the terms of the Creative Commons Attribution License (CC BY). The use, distribution or reproduction in other forums is permitted, provided the original author(s) and the copyright owner(s) are credited and that the original publication in this journal is cited, in accordance with accepted academic practice. No use, distribution or reproduction is permitted which does not comply with these terms.
*Correspondence: Young Hyeon Kwon, a3loNzY0ODc2NEBkYXVtLm5ldA==
Disclaimer: All claims expressed in this article are solely those of the authors and do not necessarily represent those of their affiliated organizations, or those of the publisher, the editors and the reviewers. Any product that may be evaluated in this article or claim that may be made by its manufacturer is not guaranteed or endorsed by the publisher.
Research integrity at Frontiers
Learn more about the work of our research integrity team to safeguard the quality of each article we publish.