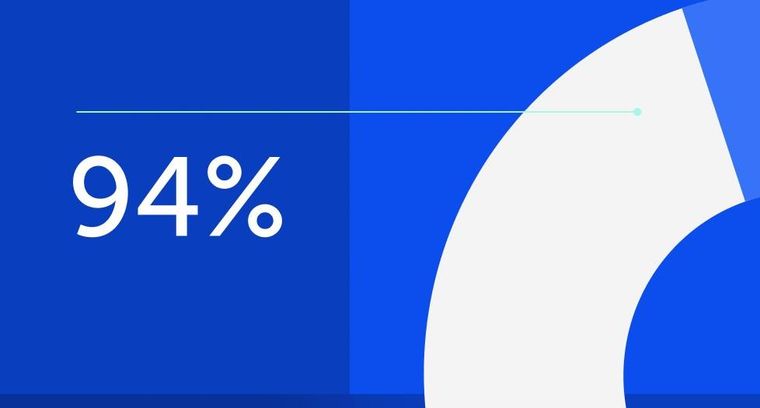
94% of researchers rate our articles as excellent or good
Learn more about the work of our research integrity team to safeguard the quality of each article we publish.
Find out more
ORIGINAL RESEARCH article
Front. Neurol., 10 October 2019
Sec. Neurorehabilitation
Volume 10 - 2019 | https://doi.org/10.3389/fneur.2019.01061
This article is part of the Research TopicEmbodying Tool Use: from Cognition to NeurorehabilitationView all 23 articles
The ability of virtual reality (VR) to recreate controlled, immersive, and interactive environments that provide intensive and customized exercises has motivated its therapeutic use after stroke. Interaction and bodily presence in VR-based interventions is usually mediated through virtual selves, which synchronously represent body movements or responses to events on external input devices. Embodied self-representations in the virtual world not only provide an anchor for visuomotor tasks, but their morphologies can have behavioral implications. While research has focused on the underlying subjective mechanisms of exposure to VR on healthy individuals, the transference of these findings to individuals with stroke is not evident and remains unexplored, which could affect the experience and, ultimately, the clinical effectiveness of neurorehabilitation interventions. This study determined and compared the sense of embodiment and presence elicited by a virtual environment under different perspectives and levels of immersion in healthy subjects and individuals with stroke. Forty-six healthy subjects and 32 individuals with stroke embodied a gender-matched neutral avatar in a virtual environment that was displayed in a first-person perspective with a head-mounted display and in a third-person perspective with a screen, and the participants were asked to interact in a virtual task for 10 min under each condition in counterbalanced order, and to complete two questionnaires about the sense of embodiment and presence experienced during the interaction. The sense of body-ownership, self-location, and presence were more vividly experienced in a first-person than in a third-person perspective by both healthy subjects (p < 0.001, = 0.212; p = 0.005, = 0.101; p = 0.001, = 0.401, respectively) and individuals with stroke (p = 0.019, = 0.070; p = 0.001, = 0.135; p = 0.014, = 0.077, respectively). In contrast, no agency perspective-related differences were found in any group. All measures were consistently higher for healthy controls than for individuals with stroke, but differences between groups only reached statistical significance in presence under the first-person condition (p < 0.010, η = 0.084). In spite of these differences, the participants experienced a vivid sense of embodiment and presence in almost all conditions. These results provide first evidence that, although less intensively, embodiment and presence are similarly experienced by individuals who have suffered a stroke and by healthy individuals, which could support the vividness of their experience and, consequently, the effectiveness of VR-based interventions.
Classical definitions of embodiment have resorted to the concepts of corporeal awareness (1), bodily self-consciousness (2), and the sense of one's own body (3). Embodiment, however, is a complex multi-component phenomenon that could be better described as the representation of an element within the body schema (4), the mental representation of body parts and reachable space, which effectively extends or displaces the normal area of influence of the body parts by real or artificial body parts, habitually used tools, or prostheses (5). Although its neural mechanisms are unclear, it is hypothesized that embodiment operates both via automatic bottom-up and potentially conscious top-down processes to permit the establishment of sensorimotor maps of one's body parts with respect to one's body (5). Previous research has identified different constitutive components of embodiment, including body-ownership, self-location, and agency (5). An understanding of their dissociation is, however, uncertain (6). Body-ownership can be defined as the sense that the body that one inhabits is one's own. Self-location alludes to the sense of being in the place where one's body is. Agency refers to the sense that one can move and control one's own body.
Experiments on multisensory and/or sensorimotor stimulation have allowed remarkable modulations of the perceived body to be performed, with respect to its natural configuration, which are known as body illusions (7). Body illusions have enabled the investigation of the embodiment subcomponents separately, with a particular emphasis on body-ownership. Because body-ownership should be continuous and omnipresent (8), synchronous visuotactile stimulation has been proven to be sufficient to induce this sense not only over rubber hands (9) or feet (10), but also over mannequins (11), in the absence of agency. In contrast, only experiments involving self-triggered actions, fired by efferent signals, have elicited agency (12). Virtual reality (VR) is a paradigmatic case of the former, as it provides multisensory stimulation while allowing real-time user interaction (13). Previous experimentation employing VR has successfully induced embodiment over virtual body parts (14, 15) and entire virtual bodies (16). Importantly, embodying virtual selves may not only affect the body schema, but could also modulate perception (17) and behavior, according to the physical characteristics of the incarnated avatar (18, 19).
Experience in VR is likewise strongly modulated by the sense of presence, which is the sense of being in the virtual environment (VE) (20) or, in other words, the sense of existing inside it (21). Similarly to embodiment, presence is a multi-component construct (22). Both user characteristics (either demographical, psychological, or clinical) and media characteristics (content or form, also known as immersion) contribute to the experience (22). However, the interaction between presence and immersion, the extent to which VR is capable of delivering an illusion of reality to the human senses (13), is not obvious. Nevertheless, a greater sense of presence is expected for higher levels of immersion, provided that other characteristics of the experience remain unchanged (22).
Although it seems reasonable that being in a specific environment can impact the sense of having, moving, and being in a body, and vice versa, research has focused on each construct individually and, consequently, interactions between embodiment and presence remain underexplored. A preliminary uncontrolled study using consumer HMDs attempted to find interactions between embodiment and presence by modulating the existence of a static avatar in the VE, which was supposed to be embodied by the participants (23). The experiment did not find connections between the investigated constructs because, among other possible reasons, it failed to elicit embodiment over the avatar, which was not able to reproduce the participants' movements. Analogously, a study with an augmented reality-based mirror found no connections between embodiment and presence because, in this case, it failed to promote presence in the VE (24). Another experiment by the same group, however, was successful at promoting high levels of embodiment and presence in a mixed reality environment, but interactions between constructs were not discussed (25). The only true attempt made to disentangle this interrelation suggested that perspective influences body-ownership and self-location over a virtual avatar, but not presence nor agency (26), which contradicts previous reports on presence (27–29).
The ability of VR to recreate controlled, immersive, and interactive environments that engage participants in intensive and customized exercises, has motivated its use in different neurological populations, especially stroke. VR-based exercises provide goal-directed tasks that are accomplished in the virtual world by the actions of virtual selves, which are controlled by the participants (30). Users usually experience the virtual world either from a first-person (egocentric) perspective, using a head-mounted display (HMD), or from a third-person (allocentric) perspective, displayed on a screen. In motor interventions, interaction is usually facilitated by body movements, which are transferred to a virtual avatar that mimics the actions in the virtual world (31–33). Although an increasing number of studies show the potential of VR-based interventions on motor function after stroke (34, 35), with a special emphasis on balance (36) and upper limb (37), little is known about how VR experiences are mediated in this population. On the contrary, all our insights into embodiment and presence have been provided by studies involving healthy subjects, predominantly young adults (38–41). The scant existing literature suggests that the ability to sense presence after stroke may be preserved (42), but there have been no previous reports on the ability to embody virtual selves. A few reports on body illusions in the real world, involving individuals with stroke, have shown contradictory results (43, 44).
While a significant body of research has focused on the underlying subjective mechanisms of exposure to VR on healthy individuals, the transference of these findings to individuals with stroke is not evident and remains unexplored. The importance of investigating such mechanisms in individuals after stroke is that they may influence the experience and performance in the VE, which, ultimately, could affect the clinical effectiveness of neurorehabilitation interventions. Thus, the hypotheses of this study were: first, that healthy subjects can experience a vivid sense of embodiment and presence after interaction with a virtual task that would vary with the level of immersion and spatial representation, in accordance with the findings of previous studies; and second, that the elicited experience and variation would be analogously reproduced in a sample of individuals with stroke. Hence, the objectives of this study were to determine and compare perceived embodiment and presence, under different conditions of immersion and spatial representation, in samples of healthy subjects and individuals with stroke.
A convenience and representative sample of healthy subjects and individuals with stroke was recruited from the staff and outpatient unit of the neurorehabilitation service of Vithas Hospital Valencia al Mar (València, Spain).
Healthy subjects, with no know musculoskeletal or psychological impairment, and matched ages and genders to those of the stroke group, were recruited. Individuals with stroke were included in the study if they had the ability to understand and interact with a VR-based task. Specifically, the exclusion criteria applied to the stroke group included: first, severe cognitive impairment, as defined by scores below 23 in the Mini-Mental State Examination (45); second, an inability to follow instructions, as defined by scores below 45 in the receptive language index of the Mississippi Aphasia Screening Test (46); third, a risk of falling, as defined by scores below 45 in the Berg Balance Scale (47); fourth, visual or hearing impairment that did not allow for interaction; and finally, unilateral spatial neglect.
Forty-six healthy subjects (25 men and 21 women), with a mean age of 50.8 ± 10.9 years, agreed to participate in the study (Table 1). Thirty-two individuals with stroke (18 men and 14 women), with a mean age of 48.8 ± 11.8 years, satisfied the participation criteria and agreed to participate in the study (Table 1). These participants presented either ischemic (n = 25) or haemorrhagic stroke (n = 7), with a mean time since onset of 9.2 ± 3.0 months. Both groups were comparable in terms of age and gender.
Ethical approval for the study was granted by the Institutional Review Board of Vithas Hospital Valencia al Mar (TU763198CIS0/1). All participants provided written informed consent before taking part in the study.
An adaptation of an interactive VR-based stepping task, which had been previously administered to individuals with stroke for therapeutic purposes (32, 48), was used as a control task. The VE consisted of an infinite checkered floor, with a central gray circle with a diameter of 50 cm, and a gender-matched mesomorph avatar, which synchronously mimicked the participants' movements (Figure 1). Playdough-colored items (cubes, spheres, and cones), with a bounding box of 20 × 20 × 20 cm, appeared on the floor in front of the central circle. The objective of the task was to step on the items before they disappeared with the closest avatar foot, while keeping the other foot inside the central circle. In between stepping on the items, the foot used had to be moved back into the circle (32, 48). Specific animations and sound effects indicated when an item appeared, disappeared, and was squashed. Extrinsic feedback was provided during the task, with information on the number of items successfully stepped on and the remaining time (Figure 1). At the end of the task, the percentage of items stepped on was shown.
Figure 1. Interaction with the virtual task and virtual environment in first and third-person condition. The figure shows: (A) a participant interacting with the virtual task in first-person perspective (up), and the virtual environment displayed by the HMD (down), and (B) the same participant interacting with the virtual task in third-person perspective (up), and the virtual environment displayed by the screen (B). In both conditions, the participant is squashing a pink playdough item located on the ground with his left foot.
To reproduce two of the most widely used VR configurations, the VE was represented either from the avatar's egocentric point of view (first-person perspective), and displayed with a HMD, the Oculus Rift CV1 (Oculus VR, Irvine, CA), or from an allocentric point of view (third-person perspective), displayed on a 60″ LED Screen (LG, Seoul, South Korea), which was hung on a wall, with its center at ~175 cm from the floor (Figure 1). The center of the VE was defined as being at a distance of 2 m in front of the screen, which was marked on the floor. The HMD had a resolution of 2,160 × 1,200, a refresh rate of 90 Hz, and horizontal and vertical fields of view of 94° and 93°, respectively (49). The screen had a resolution of 1,920 × 1,080, a refresh rate of 60 Hz, and had approximate effective horizontal and vertical fields of view to the participants of 37° and 11°, respectively. Auditory feedback was provided by the integrated headphones in the HMD or the integrated speakers of the TV screen, as appropriate. Interaction was facilitated by a Kinect for Windows v2 (Microsoft, Redmond, WA), which was fixed under the TV at a height of 80 cm from the floor to ensure full body-tracking (50, 51). This device provided the positions of the main joints of the participants at 30 Hz. In the third-person perspective, all joints were used to animate the avatar. By way of contrast, in the first-person perspective, head rotation and acceleration were provided by the HMD (Figure 1).
A high-end computer, including an 8-core Intel® Core™ i7-4790 @3.60 GHz, 8 GB of RAM, and a NVIDIA® Geforce® GTX Titan Xp with 12 GB of GDDR5, was used to run the VE during the experiment.
Two experimenters were in charge of conducting the sessions and ensuring the safety and comfort of the participants. The participants, who were blind to the purpose of the experiment, were briefly introduced to the instrumentation, procedure, and task. They were then situated on the mark on the floor, looking toward the Kinect for Windows v2, and then the experiment started. All participants interacted with the VE for 10 min, in counterbalanced order, under both conditions: first-person perspective with the HMD and third-person perspective with the TV screen. After each condition, the participants were asked to evaluate their perceived sense of embodiment and presence, using two dedicated questionnaires: an adapted version of the Embodiment of Rubber Hand Questionnaire (38) and the Slater-Usoh-Steed Questionnaire (52), respectively. The adapted version of the Embodiment of Rubber Hand Questionnaire contained the same 10 items as the original version but references to a rubber hand were replaced by equivalent references to the virtual avatar. Consequently, this questionnaire assessed the extent to which the participant: can control the avatar with their movements; feels located in the same place as the avatar; and feels the body of the avatar belonged to them. The Slater-Usoh-Steed Questionnaire is a three-item Likert-scale questionnaire that evaluates: the sense of being in a VE; the extent to which a VE feels real; and the extent to which a VE is thought of as a place visited. The scores for both questionnaires ranged from 1 (strongly disagree) to 7 (strongly agree). A speech therapist was in charge of explaining the questions of the questionnaires to the participants with stroke and solving any possible doubt about their meaning.
Subcomponents of embodiment were defined, according to the original description on the questionnaire, as the average score of the first five statements (body-ownership), of the sixth to eighth statements (localization), and of the last two statements (agency) (38). Average scores >4 were considered as denoting a meaningful reflection of the vividness of the experience (38, 43).
Mixed ANOVAs were performed to determine the differences between the conditions for the individual groups (healthy and stroke) as between-subject, and perspective as within-subject, factors. The investigators performing the data analysis were blinded. The analyses were computed using SPSS for Windows® v22 (IBM®, Armonk, NY, USA).
Scores for the experienced embodiment and presence, and the statistical differences between them, are provided in Figures 2, 3, respectively.
Figure 2. Reports on embodiment sub-constructs in both conditions. The figure shows box and whisker plots of the sense of (A) body-ownership, (B) self-location, and (C) agency experienced by healthy subjects and individuals with stroke in both conditions. *p < 0.05, **p < 0.01.
Figure 3. Reports on sense of presence in both conditions. The figure shows a box and whisker plot of the sense of presence experienced by healthy subjects and individuals with stroke in both conditions. *p < 0.05, **p < 0.01.
Statistically significant differences were identified between the healthy subjects under the first- and third-person conditions for body-ownership (5.41 ± 0.88 vs. 4.36 ± 1.39; F(1,76) = 20.473, p < 0.001, = 0.212), self-location (5.43 ± 0.84 vs. 4.74 ± 1.47; F(1,76) = 8.553, p = 0.005, = 0.101), and presence (5.49 ± 0.81 vs. 4.03 ± 1.24; F(1,76) = 50.973, p < 0.001, = 0.401). Higher values of these variables were consistently detected for the first-person perspective. Nonetheless, both conditions seemed to successfully induce body-ownership and self-location over the virtual avatar, and presence in the VE, based on the fact that all values exceeded the meaningful threshold. The scores for the sense of presence under the third-person condition were, however, borderline. No differences between conditions were detected for agency (5.98 ± 0.75 vs. 6.04 ± 0.82), which showed the highest values of all the subconstructs of embodiment.
Similar to the healthy participants, statistically significant differences were found between the first- and third-person conditions in the stroke group for body-ownership (4.61 ± 2.02 vs. 3.95 ± 1.92; F(1,76) = 5.753, p = 0.019, = 0.070), self-location (5.10 ± 1.53 vs. 4.13 ± 2.15; F(1,76) = 11.910, p = 0.001, = 0.135), and presence (4.45 ± 1.80 vs. 3.83 ± 1.61; F(1,76) = 6.357, p = 0.014, = 0.077). While these variables were rated as having been vividly experienced in the first-person condition, the third-person condition barely induced self-location over the virtual avatar, and the scores for body-ownership and presence did not (although they almost did) reach the meaningful threshold. As in the healthy group, agency did not show differences between conditions (5.59 ± 1.41 vs. 5.25 ± 1.58), and obtained the highest scores of all the embodiment subconstructs.
A comparison between the groups evidenced statistically significant differences in presence (5.49 ± 0.81 vs. 4.45 ± 1.80; F(1,76) = 6.952, p = 0.010, = 0.084) in the first-person condition. The scores for embodiment and presence in the healthy group were consistently higher than those in the stroke group for all measures and under both conditions.
This study investigated and compared the sense of embodiment and presence experienced by a sample of healthy subjects and individuals with stroke during exposure to an interactive VR task from different perspectives and under different levels of immersion. The results evidenced that the first-person perspective using HMD elicited a greater sense of body-ownership and self-location over a virtual avatar and a greater sense of presence in both populations. Agency, however, remained almost invariable between conditions. The participants with stroke consistently reported less vivid experiences than the healthy participants, while the differences between the groups were only statistically significant for body-ownership and presence under the first-person condition.
The greater sense of body-ownership and self-location under the first-person condition, and the comparable sense of agency under both conditions, reported by the healthy subjects are in line with previous findings (26, 53–55) and also evidence the distinct natures of the different illusions. The contradictory findings of a previous study, which reported similar vividness of body-ownership and agency, regardless of the perspective, might be explained by a lack of sensitivity in the measurement tools used, as only one question was used to assess each illusion (56). Similar to the effect of perspective on body-ownership and self-location, the greater levels of presence reported by the healthy subjects during the high-immersion condition is supported by previous research (27–29). Interestingly, the only previous experiment that simultaneously investigated embodiment and presence varying the point of view in the VE reported an invariable sense of presence between perspectives for an invariable degree of immersion (26). This could indicate that the differences in presence detected under each condition in our study might be better explained by the difference in immersion rather than perspective. Unfortunately, as both concepts were jointly modified for each condition in our study, it is not possible to identify the determining factor for presence. Despite the differences in the sensed embodiment and presence between conditions, their scores may indicate that healthy subjects are able to successfully incarnate virtual avatars and be present in a virtual world, independent of the perspective and the mediating technology.
Although they did not reach statistical significance, the lower scores for body-ownership and self-location in individuals with stroke in the first-person perspective might suggest a difficulty in these participants to experience the virtual body as their own and being located in it. Although there have been no previous studies that have investigated embodiment in VEs after stroke, experiments involving body illusions in the real world have reported contradictory results that have shown either an increased (43, 57) or decreased (44) predisposition to body-ownership. In our study, post-stroke cognitive disorders, such as a diminished capacity for abstract thinking, which is commonly identified after stroke (58), might have challenged the incarnation of the virtual avatars that, while mimicking the participants' movements, still remained neutral, non-real versions of the participants. In addition, limitations in the body motion-tracking provided by the Kinect v2 (50) and of the mobility of the avatars may have led to some pathological motor patterns in the individuals with stroke to not be exactly reproduced by their virtual selves, which may have reduced their identification of the avatar as their own body and being located in the virtual avatar. This could explain why participants with stroke reported control over the avatar movements, but did not report ownership or self-location. It is important, however, to highlight that the vividness of the embodiment subcomponents was supported by the scores for the different illusions, which exceeded the meaningful threshold under both conditions, but for body-ownership, which were slightly lower than that under the third-person condition. The statistical differences in the sense of presence between the groups were analogous to differences for body-ownership and self-location, with the stroke group showing lower scores. Likewise for these illusions, the cognitive condition of the individuals with stroke might have complicated their interpretations of the VE as real and, consequently, decreased their sense of existing in it; however, also similarly to body-ownership and self-location, scores for this sense support the vividness of the experience.
In summary, the highest scores for sensed body-ownership, self-location, and presence, were provided by the healthy subjects, regardless of condition, and for the first-person perspective with a HMD, regardless of the group. The sense of agency, in contrast, received invariably high scores under all conditions and for both groups. The analogous scores for individuals with stroke and the healthy control group support that the sense of embodiment and presence were similarly experienced in both populations. This suggests that the basic mechanisms that modulate these phenomena could be preserved after a stroke, and may support the effectiveness of VR interventions in this population.
A sense of embodiment and presence were effectively experienced in both healthy subjects and individuals post-stroke, although less intensively in the latter. The feelings were similarly modulated by perspective and level of immersion.
The datasets generated for this study will not be made publicly available confidential data.
Ethical approval for the study was granted by the Institutional Review Board of Vithas Hospital Valencia al Mar (TU763198CIS0/1). All participants provided written informed consent before taking part in the study.
AB and RL designed the study. RL defined the clinical aspects regarding individuals with stroke and JL assessed their condition. AB and JL conducted the experimental sessions. All the authors analyzed and discussed the results of the experiment.
This study was funded by Ministerio de Economía y Competitividad of Spain (Project RTC-2017-6051-7 and Grant BES-2014-068218), Fundació la Marató de la TV3 (Grant 201701-10), and Universitat Politècnica de València (Grant PAID-10-18).
The authors declare that the research was conducted in the absence of any commercial or financial relationships that could be construed as a potential conflict of interest.
We acknowledge the support of NVIDIA Corporation with the donation of the Titan Xp GPU used for this research.
1. Berlucchi G, Aglioti S. The body in the brain: neural bases of corporeal awareness. Trends Neurosci. (1997) 20:560–4. doi: 10.1016/S0166-2236(97)01136-3
2. Legrand D. The bodily self: the sensori-motor roots of pre-reflective self-consciousness. Phenom Cogn Sci. (2006) 5:89–118. doi: 10.1007/s11097-005-9015-6
3. Arzy S, Overney LS, Landis T, Blanke O. Neural mechanisms of embodiment: asomatognosia due to premotor cortex damage. Arch Neurol. (2006) 63:1022–5. doi: 10.1001/archneur.63.7.1022
4. De Vignemont F. Embodiment, ownership and disownership. Conscious Cogn. (2011) 20:82–93. doi: 10.1016/j.concog.2010.09.004
5. Giummarra MJ, Gibson SJ, Georgiou-Karistianis N, Bradshaw JL. Mechanisms underlying embodiment, disembodiment and loss of embodiment. Neurosci Biobehav Rev. (2008) 32:143–60. doi: 10.1016/j.neubiorev.2007.07.001
6. Ma K, Hommel B. The role of agency for perceived ownership in the virtual hand illusion. Conscious Cogn. (2015) 36:277–88. doi: 10.1016/j.concog.2015.07.008
7. Kilteni K, Maselli A, Kording KP, Slater M. Over my fake body: body ownership illusions for studying the multisensory basis of own-body perception. Front Hum Neurosci. (2015) 9:141. doi: 10.3389/fnhum.2015.00141
8. Clark A, Kiverstein J, Vierkant T editors. Decomposing the Will. New York, NY: Oxford University Press (2013). p. 368.
9. Botvinick M, Cohen J. Rubber hands “feel” touch that eyes see. Nature. (1998) 391:756. doi: 10.1038/35784
10. Crea S, D'Alonzo M, Vitiello N, Cipriani C. The rubber foot illusion. J Neuroeng Rehabil. (2015) 12:77. doi: 10.1186/s12984-015-0069-6
11. Petkova VI, Ehrsson HH. If I were you: perceptual illusion of body swapping. PLoS ONE. (2008) 3:e3832. doi: 10.1371/journal.pone.0003832
12. Frith CD, Blakemore SJ, Wolpert DM. Abnormalities in the awareness and control of action. Philos Trans R Soc B Biol Sci. (2000) 355:1771–88. doi: 10.1098/rstb.2000.0734
13. Bermúdez i Badia S, Fluet GG, Llorens R, Deutsch JE. Virtual reality for sensorimotor rehabilitation post stroke: design principles and evidence. In: Reinkensmeyer D, Dietz V, editors. Neurorehabilitation Technology. Cham: Springer (2016). p. 573–603.
14. Perez-Marcos D, Sanchez-Vives MV, Slater M. Is my hand connected to my body? The impact of body continuity and arm alignment on the virtual hand illusion. Cogn Neurodyn. (2012) 6:295–305. doi: 10.1007/s11571-011-9178-5
15. IJsselsteijn WA, De Kort YAW, Haans A. Is this my hand I see before me? The rubber hand illusion in reality, virtual reality, and mixed reality. Presence Teleoper Virtual Environ. (2006) 15:455–64. doi: 10.1162/pres.15.4.455
16. Slater M, Perez-Marcos D, Ehrsson HH, Sanchez-Vives MV. Inducing illusory ownership of a virtual body. Front Neurosci. (2009) 3:214–20. doi: 10.3389/neuro.01.029.2009
17. Banakou D, Groten R, Slater M. Illusory ownership of a virtual child body causes overestimation of object sizes and implicit attitude changes. Proc Natl Acad Sci USA. (2013) 110:12846–51. doi: 10.1073/pnas.1306779110
18. Yee N, Bailenson J. The proteus effect: the effect of transformed self-representation on behavior. Hum Commun Res. (2007) 33:271–90. doi: 10.1111/j.1468-2958.2007.00299.x
19. Allen MT, Jameson MM, Myers CE. Beyond behavioral inhibition: a computer avatar task designed to assess behavioral inhibition extends to harm avoidance. Front Psychol. (2017) 8:1560. doi: 10.3389/fpsyg.2017.01560
20. Slater M. Place illusion and plausibility can lead to realistic behaviour in immersive virtual environments. Philos Trans R Soc B Biol Sci. (2009) 364:3549–57. doi: 10.1098/rstb.2009.0138
21. Heeter C. Being there: the subjective experience of presence. Presence Teleoper Virtual Environ. (2015) 1:262–71. doi: 10.1162/pres.1992.1.2.262
22. Baños RM, Botella C, Alcañiz M, Liaño V, Guerrero B, Rey B. Immersion and emotion: their impact on the sense of presence. Cyber Psychol Behav. (2004) 7:734–41. doi: 10.1089/cpb.2004.7.734
23. Steed A, Frlston S, Lopez MM, Drummond J, Pan Y, Swapp D. An “In the Wild” experiment on presence and embodiment using consumer virtual reality equipment. IEEE Trans Vis Comput Graph. (2016) 22:1406–14. doi: 10.1109/TVCG.2016.2518135
24. Nimcharoen C, Zollmann S, Collins J, Regenbrecht H. Is that me? - Embodiment and body perception with an augmented reality mirror. In: Adjunct Proceedings - 2018 IEEE International Symposium on Mixed and Augmented Reality, ISMAR-Adjunct 2018. Munich (2019).
25. Regenbrecht H, Meng K, Reepen A, Beck S, Langlotz T. Mixed voxel reality: presence and embodiment in low fidelity, visually coherent, mixed reality environments. In: Proceedings of the 2017 IEEE International Symposium on Mixed and Augmented Reality, ISMAR 2017. Nantes (2017).
26. Gorisse G, Christmann O, Amato EA, Richir S. First- and third-person perspectives in immersive virtual environments: presence and performance analysis of embodied users. Front Robot AI. (2017) 4:33. doi: 10.3389/frobt.2017.00033
27. Anneliene AALFM, Stupar-Rutenfrans S, Bastiaens OSP, Van Gisbergen MMS. Observe or participate: the effect of point-of-view on presence and enjoyment in 360 degree movies for head mounted displays. In: The European Conference on Ambient Intelligence. Athens (2015).
28. Salamin P, Thalmann D, Vexo F. The benefits of third-person perspective in virtual and augmented reality? In: Proceedings of the ACM Symposium on Virtual Reality Software and Technology, VRST 2006. Limassol (2006).
29. Salamin P, Tadi T, Blanke O, Vexo F, Thalmann D. Quantifying effects of exposure to the third and first-person perspectives in virtual-reality-based training. IEEE Trans Learn Technol. (2010) 3:272–6. doi: 10.1109/TLT.2010.13
30. Lohse KR, Hilderman CGE, Cheung KL, Tatla S, Van der Loos HFM. Virtual reality therapy for adults post-stroke: a systematic review and meta-analysis exploring virtual environments and commercial games in therapy. PLoS ONE. (2014) 9:e93318. doi: 10.1371/journal.pone.0093318
31. Gil-Gómez JA, Lloréns R, Alcñiz M, Colomer C. Effectiveness of a Wii balance board-based system (eBaViR) for balance rehabilitation: a pilot randomized clinical trial in patients with acquired brain injury. J Neuroeng Rehabil. (2011) 8:30. doi: 10.1186/1743-0003-8-30
32. Lloréns R, Gil-Gómez JA, Alcañiz M, Colomer C, Noé E. Improvement in balance using a virtual reality-based stepping exercise: a randomized controlled trial involving individuals with chronic stroke. Clin Rehabil. (2015) 29:261–8. doi: 10.1177/0269215514543333
33. Colomer C, Llorens R, Noé E, Alcañiz M, Fregni F, Pascual-Leone A, et al. Effect of a mixed reality-based intervention on arm, hand, and finger function on chronic stroke. J Neuroeng Rehabil. (2016) 13:45. doi: 10.1186/s12984-016-0153-6
34. Laver KE, Lange B, George S, Deutsch JE, Saposnik G, Crotty M. Virtual reality for stroke rehabilitation. Cochrane Database Syst Rev. (2017) 11:CD008349. doi: 10.1002/14651858.CD008349.pub4
35. Saposnik G, Levin M. Virtual reality in stroke rehabilitation: a meta-analysis and implications for clinicians. Stroke. (2011) 42:1380–6. doi: 10.1161/STROKEAHA.110.605451
36. Li Z, Han XG, Sheng J, Ma SJ. Virtual reality for improving balance in patients after stroke: a systematic review and meta-analysis. Clin Rehabil. (2016) 30:432–40. doi: 10.1177/0269215515593611
37. Aminov A, Rogers JM, Middleton S, Caeyenberghs K, Wilson PH. What do randomized controlled trials say about virtual rehabilitation in stroke? A systematic literature review and meta-analysis of upper-limb and cognitive outcomes. J NeuroEng Rehabil. (2018) 15:29. doi: 10.1186/s12984-018-0370-2
38. Longo MR, Schüür F, Kammers MPM, Tsakiris M, Haggard P. What is embodiment? A psychometric approach. Cognition. (2008) 107:978–98. doi: 10.1016/j.cognition.2007.12.004
39. Dummer T, Picot-Annand A, Neal T, Moore C. Movement and the rubber hand illusion. Perception. (2009) 38:271–80. doi: 10.1068/p5921
40. Riva G, Mantovani F, Capideville CS, Preziosa A, Morganti F, Villani D, et al. Affective interactions using virtual reality: the link between presence and emotions. CyberPsychol Behav. (2007) 10:45–56. doi: 10.1089/cpb.2006.9993
41. Bulu ST. Place presence, social presence, co-presence, and satisfaction in virtual worlds. Comput Educ. (2012) 58:154–61. doi: 10.1016/j.compedu.2011.08.024
42. Rand D, Katz N, Weiss PL. Evaluation of virtual shopping in the VMALL: comparison of post-stroke participants to healthy control groups. Disabil Rehabil. (2007) 29:1710–9. doi: 10.1080/09638280601107450
43. Llorens R, Borrego A, Palomo P, Cebolla A, Noé E, I Badia SB, et al. Body schema plasticity after stroke: subjective and neurophysiological correlates of the rubber hand illusion. Neuropsychologia. (2017) 96:61–9. doi: 10.1016/j.neuropsychologia.2017.01.007
44. Zeller D, Gross C, Bartsch A, Johansen-Berg H, Classen J. Ventral premotor cortex may be required for dynamic changes in the feeling of limb ownership: a lesion study. J Neurosci. (2011) 31:4852–7. doi: 10.1523/JNEUROSCI.5154-10.2011
45. Folstein MF, Folstein SE, McHugh PR. “Mini-mental state.” A practical method for grading the cognitive state of patients for the clinician. J Psychiatr Res. (1975) 12:189–98. doi: 10.1016/0022-3956(75)90026-6
46. Romero M, Sánchez A, Marín C, Navarro MD, Ferri J, Noé E. Clinical usefulness of the Spanish version of the Mississippi Aphasia Screening Test (MASTsp): validation in stroke patients. Neurol Eng Ed. (2012) 27:216–24. doi: 10.1016/j.nrleng.2011.06.001
47. Berg KO, Wood-Dauphinee SL, Williams JI, Maki B. Measuring balance in the elderly: validation of an instrument. Can J Public Health. (1992) 83(Suppl. 2):S7–11.
48. Lloréns R, Noé E, Colomer C, Alcañiz M. Effectiveness, usability, and cost-benefit of a virtual reality-based telerehabilitation program for balance recovery after stroke: a randomized controlled trial. Arch Phys Med Rehabil. (2015) 96:418–25 e2. doi: 10.1016/j.apmr.2014.10.019
49. Borrego A, Latorre J, Alcañiz M, Llorens R. Comparison of oculus rift and HTC vive: feasibility for virtual reality-based exploration, navigation, exergaming, and rehabilitation. Games Health J. (2018) 7:151–6. doi: 10.1089/g4h.2017.0114
50. Latorre J, Llorens R, Colomer C, Alcañiz M. Reliability and comparison of Kinect-based methods for estimating spatiotemporal gait parameters of healthy and post-stroke individuals. J Biomech. (2018) 72:268–73. doi: 10.1016/j.jbiomech.2018.03.008
51. Lloréns R, Noé E, Naranjo V, Borrego A, Latorre J, Alcañiz M. Tracking systems for virtual rehabilitation: objective performance vs. subjective experience. a practical scenario. Sensors. (2015) 15:6586–606. doi: 10.3390/s150306586
52. Slater M, Steed A. A virtual presence counter. Presence Teleoper Virtual Environ. (2000) 9:413–34. doi: 10.1162/105474600566925
53. Slater M, Spanlang B, Sanchez-Vives MV, Blanke O. First person experience of body transfer in virtual reality. PLoS ONE. (2010) 5:e10564. doi: 10.1371/journal.pone.0010564
54. Petkova VI, Khoshnevis M, Ehrsson HH. The perspective matters! Multisensory integration in egocentric reference frames determines full-body ownership. Front Psychol. (2011) 2:35. doi: 10.3389/fpsyg.2011.00035
55. Maselli A, Slater M. The building blocks of the full body ownership illusion. Front Hum Neurosci. (2013) 7:83. doi: 10.3389/fnhum.2013.00083
56. Debarba HG, Molla E, Herbelin B, Boulic R. Characterizing embodied interaction in first and third person perspective viewpoints. In: 2015 IEEE Symposium on 3D User Interfaces, 3DUI 2015 - Proceedings. Arles (2015).
57. Burin D, Livelli A, Garbarini F, Fossataro C, Folegatti A, Gindri P, et al. Are movements necessary for the sense of body ownership? Evidence from the rubber hand illusion in pure hemiplegic patients. PLoS ONE. (2015) 10:e0117155. doi: 10.1371/journal.pone.0117155
58. Teasell R, Salter K, Faltynek P, Cotoi A, Eskes G. Post-stroke cognitive disorders. In: Evidence-Based Review of Stroke Rehabilitation. Available online at: http://www.ebrsr.com/evidence-review/12-post-stroke-cognitive-disorders
Keywords: embodiment, body-ownership, self-location, agency, presence, stroke, virtual reality, immersion
Citation: Borrego A, Latorre J, Alcañiz M and Llorens R (2019) Embodiment and Presence in Virtual Reality After Stroke. A Comparative Study With Healthy Subjects. Front. Neurol. 10:1061. doi: 10.3389/fneur.2019.01061
Received: 04 June 2019; Accepted: 20 September 2019;
Published: 10 October 2019.
Edited by:
Yusuf Ozgur Cakmak, University of Otago, New ZealandReviewed by:
Holger Thomas Regenbrecht, University of Otago, New ZealandCopyright © 2019 Borrego, Latorre, Alcañiz and Llorens. This is an open-access article distributed under the terms of the Creative Commons Attribution License (CC BY). The use, distribution or reproduction in other forums is permitted, provided the original author(s) and the copyright owner(s) are credited and that the original publication in this journal is cited, in accordance with accepted academic practice. No use, distribution or reproduction is permitted which does not comply with these terms.
*Correspondence: Roberto Llorens, cmxsb3JlbnNAaTNiLnVwdi5lcw==
Disclaimer: All claims expressed in this article are solely those of the authors and do not necessarily represent those of their affiliated organizations, or those of the publisher, the editors and the reviewers. Any product that may be evaluated in this article or claim that may be made by its manufacturer is not guaranteed or endorsed by the publisher.
Research integrity at Frontiers
Learn more about the work of our research integrity team to safeguard the quality of each article we publish.