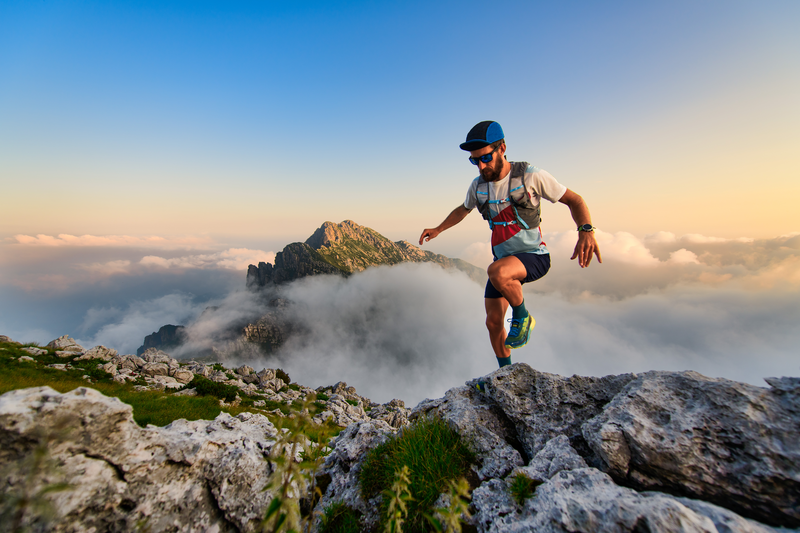
94% of researchers rate our articles as excellent or good
Learn more about the work of our research integrity team to safeguard the quality of each article we publish.
Find out more
MINI REVIEW article
Front. Neurol. , 24 September 2019
Sec. Stroke
Volume 10 - 2019 | https://doi.org/10.3389/fneur.2019.01020
This article is part of the Research Topic Cutting-Edge Approaches for CNS Protection and Repair: Focus on Vascular and Degenerative Disorders View all 83 articles
Small vessel disease (SVD) refers to conditions where damage to arterioles and capillaries is predominant, leading to reduced, or interrupted perfusion of the affected organ. Data suggest that when this condition is evident in any organ, it is already systemic in its occurrence and consequences. SVD affects primarily organs that receive significant portions of cardiac output such as the brain, the kidney, and the retina. Thus, SVD is a major etiologic cause in debilitating conditions such as renal failure, blindness, lacunar infarcts, and dementia. The factors that lead to this devastating condition include all the known vascular risk factors when they are not strictly controlled, but lifestyles that include sedentary existence, obesity, and poor sleep patterns are also recognized drivers of SVD. In addition, depression is now recognized as a vascular risk factor. Inflammation is a mediator of SVD, but it is not known which factor(s) predominate in its etiology. This article emphasizes the need for more investigations to define this link further and suggests clinical and societal responses that might reduce the major impacts of this condition on populations.
Small vessel disease (SVD) refers to any pathologic process that damages small end arteries, arterioles, venules, and brain capillaries (1). Although this condition was described pathologically more than a century ago (2), its pervasive nature and its association with clinically relevant conditions have been increasingly appreciated as imaging technologies advanced and became more accessible (3).
The main target of SVD is the endothelium, the organ that is a barrier between circulating blood and the vessel wall. Healthy endothelial cells control vascular permeability to components in plasma, limit aggregation of platelets and leucocytes, and regulate vascular tone, all of which is essential if blood flow will match metabolic demand (4). The main task of the endothelial cells is therefore to integrate information scanned constantly from the vessel wall, the blood flowing through the arterioles, and from the physiological parameters defining vascular health, and respond by varying vasomotor tone in the arterioles and maintaining an adequate antithrombotic surface.
While the endothelial cells are the main contributors to the properties of the blood brain barrier, their capabilities are induced and maintained by their interactions with mural cells (pericytes and vascular smooth muscle cells), immune cells, and glial cells—predominantly astrocytes (5). As a result, SVD results in lipohyalinosis and arteriolosclerosis.
Several animal models have been studied to shed light on the physiological processes leading to and accompanying SVD, and most involve sustained hypoperfusion of the brain as a result of bilateral stenosis of the common carotid arteries (6). This results in multiple small infarcts and hemosiderin-like regions in subcortical nuclei, with the eventual appearance of cerebral atrophy.
There is a growing appreciation that endothelial cells may become damaged when the environment is inflammatory (7). Inflammatory markers have been associated with periventricular white matter hyperintensities (WMH), the main MRI signature of SVD in the brain (8). Reports have linked a number of abnormalities in the interleukin (IL) pathways to SVD including IL-1beta, tumor necrosis factor, IL-10, IL-21, and IL-23 (9), confirming earlier findings by Flex et al. linking proinflammatory gene polymorphisms to one of the major consequences of SVD, dementia (10). C-reactive protein (CRP), a non-specific marker of inflammation, is considered an endothelial toxin, and CRP is both predictive of SVD and is elevated when the condition is evident (11). A relationship between midlife systemic inflammation and late-life SVD has also been reported (12).
The damage caused by SVD impacts both deep gray and white matter structures in the brain (13). While CT scans can reveal the presence of SVD, the ideal method for visualizing the full spectrum of SVD is structural MRI. This includes FLAIR, T1 and T2-weighted as well as gradient echo MRI sequences (14). More recently, whole-brain magnetic resonance spectroscopy has also been used to study markers of brain inflammation (15). These brain imaging modalities reveal in SVD recent small subcortical infarcts, hyperintensities in the periventricular and deep white matter, lacunes, microbleeds, and enlarged perivascular spaces, located predominantly in the basal ganglia and the subcortical white matter (16). As well, the blood-brain barrier is impaired in small vessel disease (17). Eventually, brain atrophy becomes evident (18). Combining MRI studies with measurements of cerebral blood flow and metabolism using positron emission tomography is allowing new insights linking the progression of physiological and imaging abnormalities to the development of cognitive deficits (19, 20).
In 2016 a report from experts in the field sponsored by the National Institutes of Neurological Diseases and Stroke titled: “Small Blood Vessels: Big Health Problems,” highlighted the systemic nature of the disease and its neurological consequences (21). The current review unequivocally confirms the conclusion that the presence of SVD in any organ implies that the patient is suffering from a “systemic” condition.
In the brain, SVD is strongly associated with stroke (22), and the presence of SVD in the brain hampers recovery in patients who have suffered a stroke (23). SVD in the brain is also associated with declines in psychiatric (24), and gait functions (25). As might be expected from these statements, and as already noted, SVD is a major etiologic factor in dementia (26).
If brain imaging modalities reveal SVD, there is a high probability that the condition is also affecting in variable combinations the retina (27), the kidneys (28–31), the heart (32), the lungs (33), and the musculoskeletal system (34)—in fact, every organ that is perfused at a high rate of flow. Although it is possible that all these organs are impacted by the same vascular risk factors, there is nonetheless multi-organ involvement with SVD, and the link appears multidirectional. Thus, it was reported recently that retinal neurodegeneration can predict cognitive impairment (35, 36), and small vessel disease as well as retinal microvascular abnormalities are now confirmed to be associated with an increased risk of renal failure (30, 31). Consequently, in addition to damaging the brain, SVD is perhaps the main etiology for blindness, renal dysfunction, and cardiac insufficiency, making it the etiology for major medical, and neurological conditions.
A paper published in 2007 by Vermeer et al. outlined the conditions associated with MRI-defined silent brain infarcts (37). The highest associations were noted with prior stroke, cardiovascular risk factors, and the presence of renal failure (37). This article also identified depression as having a strong association with silent brain infarcts, a link that has been confirmed subsequently (38). A 2014 study that specifically tested the association of total SVD score with vascular risk factors confirmed that smoking, hypertension, male sex, and advancing age were conditions that promoted brain SVD (39). In a separate study, SVD was observed in 3% of 40–49-year-olds but 18.9% of 70-year-olds, highlighting the association of SVD with aging (40).
In terms of the cardiovascular risk factors, hypertension is a major driver of SVD (41), and ambulatory measurements of blood pressure are better predictors of WMH than self-reported measurements (42). In the setting of hypertension, the risk of accumulating WMH rises rapidly above a resting systolic blood pressure of approximately 120 mmHg (43). Systolic blood pressure sustained above this threshold also predicts a growing risk for dementia and strokes (42, 44). Despite this overwhelming evidence in favor of maintaining at-rest systolic blood pressure at 120 mmHg, concern is often expressed that this may lead to increased mortality in the elderly, perhaps contributed to by falls and hip fractures due to orthostatic hypotension and dizziness (45). The implications of these new guidelines and the challenges they represent merited an entire issue of Circulation Research (46). It is important to note that in the SPRINT trial, among patients aged 75 or older, primary outcomes of myocardial infarction, acute coronary syndrome, stroke, congestive heart failure and cardiovascular death were all significantly lower in those whose systolic blood pressures were maintained at or below 120 mmHg (47). Despite this, Canada's hypertension guidelines still define hypertension as systolic blood pressure of more than 135 mm Hg (48).
Obesity's impact on the development of brain small vessel disease is now well-documented (49). A 2017 review concluded that obesity and its comorbidities were associated with impaired cognitive performance, accelerated cognitive decline and dementia in later life (50), confirming the conclusion of a systematic review by Pedditzi et al., published a year earlier (51). In the setting of obesity there is a significant rise in the factors promoting inflammation, leading to the conclusion that accumulated fat, particularly in its abdominal location, is a source for inflammatory cytokines (52). It is perhaps not surprising therefore that obesity has been associated with smaller brain volumes (53).
Another reason for referring to obesity's negative impact on brain function is its high prevalence in our societies. In the United States, in 2010, 35.5% of men and 35.8% of women satisfied the criteria for obesity (Body Mass Index (BMI) of 30 or more) (54), with a clear trend for increasing prevalence of abdominal obesity between 1999 and 2012 in both men and women (55). In 2009, 24% of Canadian men and women satisfied the criteria for obesity (56).
Several other vascular risk factors have been associated with greater occurrence of SVD. These include prediabetes (57), untreated diabetes (58, 59), and hyperlipidemia (60, 61). In diabetes, microvascular complications are associated with proinflammatory cytokines and with both vascular and intracellular cell adhesion molecules (62). It is now also clear that gut microbiota can impact brain health through immune influences that can lead to cerebral endothelial dysfunction. In this context, new evidence suggests that dietary salt may promote neurovascular and cognitive dysfunction through a gut-initiated TH17 response (63).
A newly emphasized risk factor for SVD is a poor sleep pattern (64). Those who chronically cheat themselves out of sufficient sleep suffer from increased systemic inflammation (65) and are at risk for SVD and eventually develop cortical atrophy (66). The most likely reason for this is that metabolic clearance from the brain occurs preferentially during sleep (67). Unfortunately, it is estimated that a large segment of the population lives daily with inadequate sleep (68), particularly in school aged children (69).
As noted above, depression was identified by Vermeer as a factor promoting silent brain infarcts (37), and depression has been confirmed to be associated specifically with SVD (70, 71). The importance of this association arises from the disturbing statistics about the prevalence of loneliness in society, a state of mind that is highly correlated with depression (72). In the United States, it has been estimated that 40% of Americans felt lonely (73), and a survey by Statistics Canada in 2012 reported that 20% of older people declared feeling lonely (74). Thus, several societal factors associated with loneliness and depression may be promoting SVD.
Since the prevalence of SVD increases with age, it is the consensus that the prevalence of dementia will increase over time as life expectancy lengthens (75). SVD is associated with both a reduction in cerebral blood flow (CBF) (76), and impaired CBF autoregulation (77), making it a key player in the etiologic mix that leads to dementia. Alzheimer's disease, where beta-amyloid and tau proteins accumulate in the brain, does lead to dementia, but the genetically inherited Alzheimer's variant becomes apparent early and impacts patients in their 50's and 60's. When individuals live past that age with normal cognitive function, the physiological profile that leads to dementia is very different. As mentioned above, data from the ADNI cohort (Alzheimer's Disease Neuroimaging Initiative) have clearly shown that when individuals are still cognitively intact, the sequence of pathophysiologic events that eventually leads to a decline in cognitive function starts with a reduction in cerebral blood flow (20), an association that was confirmed in a population-based study (78). This results in both reduced energy supply and reduced ability to clear accumulated waste. The important point to remember is that the reduction in CBF occurs before glucose dysregulation, before the abnormal accumulation of A-beta and other molecular biomarkers in the CSF, and before any impairment in cognition is evident. This new appreciation for the role of cerebral blood flow in delivering energy to the brain and removing the accumulated toxic molecules is perhaps one explanation for the failure of drugs designed to improve symptoms of cognitive decline by reducing the cerebral load of beta-amyloid (79, 80). Consequently, protecting CBF from decline and securing the brain's energy supply are crucial to our ability to defend against dementia.
The powerful message in all of this is that we need to explore the premise that individuals and societies may succeed in lowering their risk for dementia by reducing vascular risk factors and changing potentially harmful lifestyles. A review of advances in stroke research accomplished during 2018 confirms the impact of adverse lifestyle exposures on brain health and on cognition (81). Decades ago the lesson was established that a successful cure is dwarfed in its societal impact by a reduction in risk, and books intended for the public are now available with advice on how to achieve the goal of reducing the risk for dementia (82, 83).
Because SVD represents a major and growing threat to vascular health, it deserves more focused research attention. Addressing the specific pathophysiology of SVD is urgently needed. Several endothelial toxins associated with SVD are already known (84), but if we knew which played predominant roles, then it might be possible to test in SVD therapeutic tools to decrease the concentration of the most harmful inflammatory molecules. Another approach may arise from our knowledge that sustained activation of certain pro-angiogenic signaling factors studied in cancer biology can promote the generation of new blood vessels (85). Could one or more of these factors be tested as repair paradigms in the setting of SVD?
The patient with SVD keeps many subspecialists occupied. He or she sees the neurologist for failing memory function and for other consequences of lacunar infarcts, the ophthalmologist for failing vision, the nephrologist for failing kidney function, the cardiologist for heart failure, and the family physician and geriatrician for control of vascular risk factors. Each specialist is focused on how to protect his or her subspecialty organ from damage. As a result, there is enormous duplication of effort, when a centralized “Vascular Clinic” that focusses on optimizing the function of the small blood vessels may be effective. In addition to monitoring and treating the vascular risk factors, such a clinic would also help patients with the lifestyle changes they need to bring about to reduce further SVD and provide support to accomplish these goals.
SVD puts a very heavy burden on our individual and national health care budgets. Add to that the unpaid labor of caregivers to patients afflicted by the consequences of SVD, and the cost increases further. Society needs to respond with a cohesive and integrated set of actions.
When we became convinced that smoking was the cause of some lung cancers, clinicians, and scientists developed a discourse with our patients and the public emphasizing the serious consequences of smoking. Eventually society took on the industry—and it was not easy. To reduce the incidence of SVD we need to regenerate that energy, educate the public by talking about the damaging consequences of untreated high blood pressure and all the other vascular risk factors we know. Like there was in smoking, there are industries that will oppose us. Focused taxation may be one solution as it is now proven to limit the consumption of items known to contribute to SVD (86).
We now recognize that small vessel disease is a major health threat, aggravated by the current lifestyle trends in our society. We need to understand the disease better and mobilize energies to improve its prevention and treatment.
The author confirms being the sole contributor of this work and has approved it for publication.
The author declares that the research was conducted in the absence of any commercial or financial relationships that could be construed as a potential conflict of interest.
1. Poggesi A, Salvadori E, Pantoni L, Pracucci G, Cesari F, Chiti A, et al. Risk and determinants of dementia in patients with mild cognitive impairment and brain subcortical vascular changes: a study of clinical neuroimaging, and biological markers-the VMCI-tuscany study: rationale, design, and methodology. Int J Alzheimers Dis. (2012) 2012:608013. doi: 10.1155/2012/608013
2. Durand-Fardel CLM. Traité pratique des Maladies des Vieillards. Paris: Baillère (1873) (see also W. Hughes, 'Origin of lacunes', Lancet, 1965, ii, 19–21).
3. Cannistraro RJ, Badi M, Eidelman BH, Dickson DW, Middlebrooks EH, Meschia JF. CNS small vessel disease: a clinical review. Neurology. (2019) 92:1146–56. doi: 10.1212/WNL.0000000000007654
4. Persson PB. The multiple functions of the endothelium: more than just wallpaper. Acta Physiol. (2015) 13:7847–9. doi: 10.1111/apha.12464
5. Daneman R, Prat A. The blood-brain barrier. Cold Spring Harb Perspect Biol. (2015) 7:a020412. doi: 10.1101/cshperspect.a020412
6. Holland PR, Searcy JL, Salvadores N, Scullion G, Chen G, Lawson G, et al. Gliovascular disruption and cognitive deficits in a mouse model with features of small vessel disease. J Cereb Blood Flow Metab. (2015) 35:1005–14. doi: 10.1038/jcbfm.2015.12
7. Steyers CM III, Miller FJ Jr. Endothelial dysfunction in chronic inflammatory diseases. Int J Mol Sci. (2014) 15:11324–49. doi: 10.3390/ijms150711324
8. Rosano C, Marsland AL, Gianaros PJ. Maintaining brain health by monitoring inflammatory processes: a mechanism to promote successful aging. Aging Dis. (2012) 3:16–33.
9. Swardfager W, Yu D, Ramirez J, Cogo-Moreira H, Szilagyi G, Holmes MF, et al. Peripheral inflammatory markers indicate microstructural damage within periventricular white matter hyperintensities in Alzheimer's disease: a preliminary report. Alzheimers Dement. (2017) 7:56–60. doi: 10.1016/j.dadm.2016.12.011
10. Flex A, Giovannini S, Biscetti F, Liperoti R, Spalletta G, Straface G, et al. Effect of proinflammatory gene polymorphisms on the risk of Alzheimer's disease. Neurodegener Dis. (2014) 13:230–6. doi: 10.1159/000353395
11. Mitaki S, Nagai A, Oguro H, Yamaguchi S. C-reactive protein levels are associated with cerebral small vessel-related lesions. Acta Neurol Scand. (2016) 133:68–74. doi: 10.1111/ane.12440
12. Walker KA, Power MC, Hoogeyeen RC, Folsom AR, Ballantyne CM, Knopman DS, et al. Midlife systemic inflammation, late-life white matter integrity, and cerebral small vessel disease: the atherosclerosis risk in communities study. Stroke. (2017) 48:3196–202. doi: 10.1161/STROKEAHA.117.018675
13. Blair GW, Hernandez MV, Thrippleton MJ, Doubal FN, Wardlaw JM. Advanced neuroimaging of cerebral small vessel disease. Curr Treat Options Cardio Med. (2017) 19:56. doi: 10.1007/s11936-017-0555-1
14. Cuadrado-Godia E, Dwivedi P, Sharma S, Ois Santiago A, Roquer Gonzalez J, Balcells M, et al. Cerebral small vessel disease: a review focusing on pathophysiology, biomarkers, and machine learning strategies. J Stroke. (2018) 20:302–20. doi: 10.5853/jos.2017.02922
15. Mueller C, Lin JC, Sheriff S, Maudsley AA, Younger JW. Evidence of widespread metabolite abnormalities in Myalgic encephalomyelitis/chronic fatigue syndrome: assessment with whole-brain magnetic resonance spectroscopy. Brain Imaging Behav. (2019). doi: 10.1007/s11682-018-0029-4. [Epub ahead of print].
16. Wardlaw JM, Smith C, Dichgans M. Mechanisms underlying sporadic cerebral small vessel disease: insights from neuroimaging. Lancet Neurol. (2013) 12:483–97. doi: 10.1016/S1474-4422(13)70060-7
17. Wong SM, Jansen JFA, Zhang CE, Hoff EI, Staals J, van Oostenbrugge RJ, et al. Blood-brain barrier impairment and hypoperfusion are linked in cerebral small vessel disease. Neurology. (2019) 92:e1669–77. doi: 10.1212/WNL.0000000000007263
18. Nylander R, Fahlstrom M, Rostrup, Kullberrg J, Damangir S, Ahlstrom H, et al. Quantitative and qualitative MRI evaluation of cerebral small vessel disease in an elderly population: a longitudinal study. Acta Radiol. (2018) 59:612–8. doi: 10.1177/0284185117727567
19. Iadecola C, Duering M, Hachinski V, Joutel A, Pendlebury ST, Schneider JA, et al. Vascular cognitive impairment and dementia: JACC scientific expert panel. J Am Coll Cardiol. (2019) 73:3326–44. doi: 10.1016/j.jacc.2019.04.034
20. Iturria-Medina Y, Sotero RC, Tussaint PJ, Mateos-Perez JM, Evans AC. Alzheimer's disease neuroimaging initiative. Nat Commun. (2016) 7:11934. doi: 10.1038/ncomms11934
21. Bosetti F, Galis ZS, Bynoe MS, Charette M, Cipolla MJ, Del Zoppo GJ, et al. “Small blood vessels: big health problems” workshop participants. “small blood vessels: big health problems?” scientific recommendations of the national institutes of health workshop. J AM Heart Assoc. (2016) 5:e004389. doi: 10.1161/JAHA.116.004389
22. Ostergaard L, Engedal TS, Moreton F, Hansen MB, Wardlaw JM, Dalkara T, et al. Cerebral small vessel disease: capillary pathways to stroke and cognitive decline. J Cereb Blood Flow Metab. (2016) 36:302–25. doi: 10.1177/0271678X15606723
23. Liang Y, Chen Y-K, Deng M, Mok VCT, Wang D-F, Ungvari GS, et al. Association of cerebral small vessel disease burden and health-related quality of life after acute ischemic stroke. Front. Aging Neurosci. (2017) 9:372. doi: 10.3389/fnagi.2017.00372
24. Kim HJ, Kang SJ, Kim C, Kim GH, Jeon S, Lee JM, et al. The effects of small vessel disease and amyloid burden on neuropsychiatric symptoms: a study among patients with subcortical vascular cognitive impairments. Neurobiol Aging. (2013) 34:1913–20. doi: 10.1016/j.neurobiolaging.2013.01.002
25. Pinter D, Ritchie SJ, Doubal F, Gattringer T, Morris Z, Bastin ME, et al. Impact of small vessel disease in the brain on gait and balance. Sci Rep. (2017) 7:41637. doi: 10.1038/srep41637
26. van Uden IW, van der Hoist HM, Tuladhar AM, van Norden AG, de Laat KE, Rutten-Jacobs LC, et al. White matter and hippocampal volume predict the risk of dementia in patients with cerebral small vessel disease: the RUN DMC study. J Alzheimers Dis. (2016) 49:863–73. doi: 10.3233/JAD-150573
27. Ahsan H. Diabetic retinopathy—biomolecules and multiple pathophysiology. Diabetes Metab Syndr. (2015) 9:51–4. doi: 10.1016/j.dsx.2014.09.011
28. Makin SD, Cook FA, Dennis MS, Wardlaw JM. Cerebral small vessel disease and renal function: systematic review and meta-analysis. Cerebrovasc Dis. (2015) 39:39–52. doi: 10.1159/000369777
29. Ikram MA, Vernooij MW, Hofman A, Niessen WJ, van der Lugt A, Breteler MMB. Kidney function is related to cerebral small vessel disease. Stroke. (2008) 39:55–61. doi: 10.1161/STROKEAHA.107.493494
30. Vogels SC, Emmelot-Vonk MH, Verhaar HJ, Koek HL. The association of chronic kidney disease with brain lesions on MRI or CT. A systematic review. Maturitas. (2012) 71:331–6. doi: 10.1016/j.maturitas.2012.01.008
31. Yip W, Sabanayagam C, Teo BW, Tay WT, Ikram MK, Tai ES, et al. Retinal microvascular abnormalities and risk of renal failure in Asian populations. PLoS ONE. (2015) 10:e0118076. doi: 10.1371/journal.pone.0118076
32. Kim BJ, Lee SH, Kim CK, Ryu WS, Kwon HM, Choi SY, et al. Advanced coronary artery calcification and cerebral small vessel diseases in the healthy elderly. Cir J. (2011) 75:451–6. doi: 10.1253/circj.CJ-10-0762
33. Seto-Yukimura R, Ogawa E, Hisamatsu T, Torii S, Shiino A, Nozaki K, et al. Reduced lung function and cerebral small vessel disease in japanese men: the shiga epidemiological study of subclinical atherosclerosis (SESSA). J Atheroscler Thromb. (2018) 25:1009–21. doi: 10.5551/jat.42127
34. Schroder JM, Zuchner S, Dichgans M, Nagy Z, Molnar MJ. Peripheral nerve and skeletal muscle involvement in CADASIL. Acta Neuropathol. (2005) 110:587–99. doi: 10.1007/s00401-005-1082-9
35. Mutlu U, Colijn JM, Ikram MA, Bonnemaijer PWM, Licher S, Wolters FJ, et al. Association of retinal neurodegeneration on optical coherence tomography with dementia: a population-based study. JAMA Neurol. (2018) 75:1256–63. doi: 10.1001/jamaneurol.2018.1563
36. O'Bryhim BE, Apte RS, Kung N, Coble D, Van Stavern G. Association of preclinical Alzheimer disease with optical coherence tomographic angiography findings. JAMA Ophthalmol. (2018) 136:1242–8. doi: 10.1001/jamaophthalmol.2018.3556
37. Vermeer SE, Longstreth WT, Koudstaal PJ. Silent brain infarcts: a systematic review. Lancet Neurol. (2007) 6:611–9. doi: 10.1016/S1474-4422(07)70170-9
38. Brookes RL, Herbert V, Lawrence AJ, Morris RG, Markus HS. Depression in small-vessel disease relates to white matter ultrastructural damage, not disability. Neurology. (2014) 83:1417–23. doi: 10.1212/WNL.0000000000000882
39. Staals J, Makin SDJ, Doubal FN, Dennis MS, Wardlaw JM. Stroke subtype, vascular risk factors, and total MRI brain small-vessel disease burden. Neurology. (2014) 83:1228–34. doi: 10.1212/WNL.0000000000000837
40. Smith EE, O'Donnell M, Dagenais G, Lear SA, Wielgosz A, Sharma M, et al. Early cerebral small vessel disease and brain volume, cognition, and gait. Ann Neurol. (2015) 77:251–61. doi: 10.1002/ana.24320
41. Abraham HMA, Wolfson L, Moscufo N, Guttmann CRG, Kaplan RF, White WB. Cardiovascular risk factors and small vessel disease of the brain: blood pressure, white matter lesions, and functional decline in older persons. J Cereb Blood Flow Metab. (2016) 36:132–42. doi: 10.1038/jcbfm.2015.121
42. Abell JG, Kivimaki M, Dugravot A, Tabak AG, Fayosse A, Shipley M, et al. Association between systolic blood pressure and dementia in the Whitehall II cohort study: role of age, duration, and threshold used to define hypertension. Eur Heart J. (2018) 39:3119–25. doi: 10.1093/eurheartj/ehy288
43. The SPRINT MIND Investigators for the SPRINT Research Group, Nasrallah IM, Pajewski NM, Auchus AP, Chelune G, Cheung AK, et al. Association of intensive vs. standard blood pressure control with cerebral white matter lesions. JAMA. (2019) 322:524–34. doi: 10.1001/jama.2019.10551
44. Egan BM, Li J, Wagner CS. Systolic blood pressure intervention trial (SPRINT) and target systolic blood pressure in future hypertension guidelines. Hypertension. (2016) 68:318–23. doi: 10.1161/HYPERTENSIONAHA.116.07575
45. Birns J, Markus H, Kaira L. Blood pressure reduction for vascular risk: is there a price to be paid? Stroke. (2005) 36:1308–13. doi: 10.1161/01.STR.0000165901.38039.5f
46. Iadecola Gottesman RF. Neurovascular and cognitive dysfunction in hypertension: epidemiology, pathobiology, and treatment. Circul Res. (2019) 124:1025–44. doi: 10.1161/CIRCRESAHA.118.313260
47. Wright JT Jr, Williamson JD, Whelton PK, Snyder JK, Sink KM, Rocco MV, et al. A randomized trial of intensive versus standard blood pressure control. SPRINT research group. N Engl J Med. Nov. (2015) 373:2103–216. doi: 10.1056/NEJMoa1511939
48. Nerenberg KA, Zarnke KB, Leung AA, Dasgupta K, Butalia S, McBrien K, et al. Hypertension Canada's 2018 guidelines for diagnosis, risk assessment, prevention, and treatment of hypertension in adults and children. Can J Cardiol. (2018) 34:506–25. doi: 10.1016/j.cjca.2018.02.022
49. Yamashiro K, Tanaka R, Tanaka Y, Miyamoto N, Shimada Y, Ueno Y, et al. Visceral fat accumulation is associated with cerebral small vessel disease. Eur J Neurol. (2014) 21:667–73. doi: 10.1111/ene.12374
50. Dye L, Boyle NB, Champ C, Lawton C. The relationship between obesity and cognitive health and decline. Proc Nutr Soc. (2017) 76:443–54. doi: 10.1017/S0029665117002014
51. Pedditzi E, Peters R, Beckett N. The risk of overweight/obesity in mid-life and late life for the development of dementia: a systematic review and meta-analysis of longitudinal studies. Age Ageing. (2016) 45:14–21. doi: 10.1093/ageing/afv151
52. Shaley D, Arbuckle MR. Metabolism and memory: obesity, diabetes, and dementia. Biol Psychiatry. (2017) 82:e81–3. doi: 10.1016/j.biopsych.2017.09.025
53. Brey RL. Long-term obesity is linked to loss of brain tissue. Neurology. (2004) 63:E19–20. doi: 10.1212/01.wnl.0000148952.71557.bf
54. Flegal KM, Carroll MD, Kit BK, Ogden CL. Prevalence of obesity and trends in the distribution of body mass index among US adults, 1999–2010. JAMA. (2012) 307:491–7. doi: 10.1001/jama.2012.39
55. Ford ES, Maynard LM, Li C. Trends in mean waist circumference and abdominal obesity among US adults, 1999–2012. JAMA. (2014) 312:1073–5. doi: 10.1001/jama.2014.8362
56. Shields M, Carroll MD, Ogden CL. Adult obesity prevalence in Canada and the United States. NCHS Data Brief . (2011) 56:1–8. doi: 10.3945/an.111.000497
57. Sorensen BM, Houben AJ, Berendschot TT, Schouten JS, Kroon AA, van der Kallen CJ, et al. Prediabetes and type 2 diabetes are associated with generalized microvascular dysfunction: the maastricht study. Circulation. (2016) 134:1339–52. doi: 10.1161/CIRCULATIONAHA.116.023446
58. Sanahuja J, Alonso N, Diez J, Ortega E, Rubinat E, Traveset A, et al. Increased burden of cerebral small vessel disease in patients with type 2 diabetes and retinopathy. Diabetes Care. (2016) 39:1614–20. doi: 10.2337/dc15-2671
59. Karapanayiotides T, Piechowski-Jozwiak B, van Melle G, Bogousslavsky J, Devuyst G. Stroke patterns, etiology, and prognosis in patients with diabetes mellitus. Neurology. (2004) 62:1558–62. doi: 10.1212/01.WNL.0000123252.55688.05
60. Staszewski J, Piusinska-Macoch R, Brodacki B, Skrobowska E, Stepien A. Association between hemostatic markers, serum lipid fractions and progression of cerebral small vessel disease: a 2-years follow-up study. Neurol Neurochir Pol. (2018) 52:54–63. doi: 10.1016/j.pjnns.2017.11.005
61. Kraft P, Schuhmann MK, Garz C, Jandke S, Urlaub D, Menci S, et al. Hypercholesterolemia induced cerebral small vessel disease. PLoS ONE. (2017) 12:e0182822. doi: 10.1371/journal.pone.0182822
62. Kaul K, Hodgkinson A, Tarr JM, Kohner EM, Chibber R. Is inflammation a common retinal-renal-nerve pathogenic link in diabetes? Curr Diabetes Rev. (2010) 6:294–303. doi: 10.2174/157339910793360851
63. Faraco G, Brea D, Garcia-Bonilla L, Wang G, Racchumi G, Chang H, et al. Dietary salt promotes neurovascular and cognitive dysfunction through a gut-initiage TH17 response. Nat Neurosci. (2018) 21:240–9. doi: 10.1038/s41593-017-0059-z
64. Gelber RP, Redline S, Ross GW, Petrovitch H, Sonnen JA, Zarow C, et al. Associations of brain lesions at autopsy with polysomnography features before death. Neurology. (2015) 84:296–303. doi: 10.1212/WNL.0000000000001163
65. Irwin MR, Olmstead R, Carroll JE. Sleep disturbance, sleep duration, and inflammation: a systematic review and meta-analysis of cohort studies and experimental sleep deprivation. Biol Psychiatry. (2016) 80:40–52. doi: 10.1016/j.biopsych.2015.05.014
66. Sexton CE, Storsve AB, Walhovd KB, Johansen-Berg H, Fjell AM. Poor sleep quality is associated with increased cortical atrophy in community-dwelling adults. Neurology. (2014) 83:967–73. doi: 10.1212/WNL.0000000000000774
67. Xie L, Kang H, Xu Q, Chen MJ, Liao Y, Thiyagarajan M, et al. Sleep drives metabolite clearance from the adult brain. Science. (2013) 342:373.7. doi: 10.1126/science.1241224
68. Jean-Louis G, Grandner MA, Youngstedt SD, Williams NJ, Zizi F, Sarpong DF, et al. Differential increase in prevalence estimates of indadequate sleep among black and white Americans. BMC Public Health. (2015) 15:1185. doi: 10.1186/s12889-015-2500-0
69. Owens JA, Spirito A, McGuinn M, Nobile C. Sleep habits and sleep disturbance in elementary school-aged children. J Dev Behav Pediatr. (2000) 21:27–36. doi: 10.1097/00004703-200002000-00005
70. Rensma SP, van Sloten TT, Launer LJ, Stehouwer CDA. Cerebral small vessel disease and risk of incident stroke, dementia and depression, and all-cause mortality: a systematic review and meta-analysis. Neurosci Biobehav Rev. (2018) 90:164–73. doi: 10.1016/j.neubiorev.2018.04.003
71. van Sloten TT, Mitchell GF, Sigurdsson S, van Buchem MA, Jonsson PV, Garcia ME, et al. Associations between arterial stiffness, depressive symptoms and cerebral small vessel disease: cross-sectional findings from the AGES-Reykjavik Study. J Psychiatry Neurosci. (2016) 41:162–8. doi: 10.1503/jpn.140334
72. Cacioppo JT, Hawkley LC, Thisted RA. Perceived social isolation makes me sad: 5-year cross-lagged analyses of loneliness and depressive symptomatology in the Chicago Health Aging, and Social Relations Study. Psychol Aging. (2010) 25:453–63. doi: 10.1037/a0017216
73. Hawkley LC, Cacioppo JT. Loneliness matters: a theoretical and empirical review of consequences and mechanisms. Ann Behav Med. (2010) 40:218–27. doi: 10.1007/s12160-010-9210-8
74. Gilmour H. Social participation and the health and well-being of Canadian seniors. Health Rep. (2012) 23:23−32.
75. Grammas P. Neurovascular dysfunction inflammation and endothelial activation: implications for the pathogenesis of Alzheimer's disease. J Neuroinf. (2011) 8:26. doi: 10.1186/1742-2094-8-26
76. Shi Y, Thrippleton MJ, Makin SD, Marshall L, Geerlings MI, de Craen AJM, et al. Cerebral blood flow in small vessel disease: a systematic review and meta-analysis. J Cereb Blood Flow Metab. (2016) 36:1653–67. doi: 10.1177/0271678X16662891
77. Brickman AM, Guzman VA, Gonzalez-Castellon M, Razlighi Q, Gu Y, Narkhede A, et al. Cerebral autoregulation, beta amyloid, and white matter hyperintensities are interrelated. Neurosci Lett. (2015) 592:54–8. doi: 10.1016/j.neulet.2015.03.005
78. Wolters FJ, Zonneveld HI, Hofman A, van der Lugt A, Koudstaal PJ, Vernooij MV, et al. Cerebral perfusion and the risk of dementia: a population-based study. Circulation. (2017) 136:719–28. doi: 10.1161/CIRCULATIONAHA.117.027448
79. Mehta D, Jackson R, Paul G, Shi J, Sabbagh M. Why do trials for Alzheimer's disease drugs keep failing? A discontinued drug perspective for 2010-2015. Expert Opin Investig Drugs. (2017) 26:735–9. doi: 10.1080/13543784.2017.1323868
80. Ricciarelli R, Fedele E. The amyloid cascade hypothesis in Alzheimer's disease: it's time to change our mind. Curr Neuropharmacol. (2017) 15:926–35. doi: 10.2174/1570159X15666170116143743
81. Wardlaw JM, Bath PM. Stroke research in 2018: extended time windows, refined benefit, and lifestyle prevention targets. Lancet Neurol. (2019) 18:2–3. doi: 10.1016/S1474-4422(18)30457-5
83. Sherzai D, Sherzai A. The Alzheimer's Solution: A Breakthrough Program to Prevent and Reverse the Symptoms of Cognitive Decline at Every Age. New York, NY: Harper Collins Publishers (2017).
84. Poggesi A, Pasi M, Pescini F, Pantoni L, Inzitari D. Circulating biologic markers of endothelial dysfunction in cerebral small vessel disease: a review. J Cereb Blood Flow Metab. (2016) 36:72–94. doi: 10.1038/jcbfm.2015.116
85. Fraineau S, PalIi CG, McNeil B, Ritso M, Prasain SN, Chu A, et al. Epigenetic activation of pro-angiogenic signaling pathways in human endothelial progenitors increases vasculogenesis. Stem Cell Rep. (2017) 9:1573–87. doi: 10.1016/j.stemcr.2017.09.009
Keywords: SVD, vascular risk factors, inflammation, dementia, lifestyle
Citation: Hakim AM (2019) Small Vessel Disease. Front. Neurol. 10:1020. doi: 10.3389/fneur.2019.01020
Received: 15 February 2019; Accepted: 09 September 2019;
Published: 24 September 2019.
Edited by:
Gregory Jaye Bix, University of Kentucky, United StatesReviewed by:
Costantino Iadecola, Cornell University, United StatesCopyright © 2019 Hakim. This is an open-access article distributed under the terms of the Creative Commons Attribution License (CC BY). The use, distribution or reproduction in other forums is permitted, provided the original author(s) and the copyright owner(s) are credited and that the original publication in this journal is cited, in accordance with accepted academic practice. No use, distribution or reproduction is permitted which does not comply with these terms.
*Correspondence: Antoine M. Hakim, YWhha2ltQG9ocmkuY2E=
Disclaimer: All claims expressed in this article are solely those of the authors and do not necessarily represent those of their affiliated organizations, or those of the publisher, the editors and the reviewers. Any product that may be evaluated in this article or claim that may be made by its manufacturer is not guaranteed or endorsed by the publisher.
Research integrity at Frontiers
Learn more about the work of our research integrity team to safeguard the quality of each article we publish.