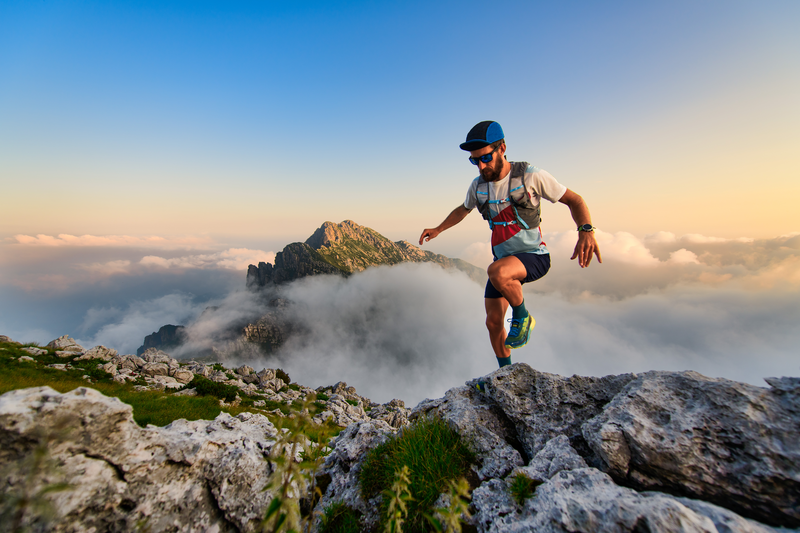
95% of researchers rate our articles as excellent or good
Learn more about the work of our research integrity team to safeguard the quality of each article we publish.
Find out more
REVIEW article
Front. Neurol. , 12 September 2019
Sec. Neurorehabilitation
Volume 10 - 2019 | https://doi.org/10.3389/fneur.2019.00925
This article is part of the Research Topic Concussion Rehabilitation View all 17 articles
Positive effects of methylphenidate (MPH) on attention and cognitive processing speed have been reported in studies of patients with moderate to severe traumatic brain injury (TBI). Studies which have acquired functional brain imaging before and while using MPH have also found alteration of brain activation while performing a cognitive task; in some studies, this alteration of activation in selective brain regions was also related to improved performance on cognitive tests administered outside of the scanning environment. Enhanced cognitive performance has been reported after single doses of MPH and after daily treatment over durations of up to and exceeding 1 month. Preclinical research and both positron emission tomography and single photon emission tomography of humans have shown that MPH increases extracellular dopamine and norepinephrine; the dose effects of MPH have an inverted U-shaped function where high doses may cause insomnia, nervousness, and increased heart rate among other symptoms and impair cognitive performance, whereas too low a dose fails to improve cognitive performance. In the past 5 years, small clinical trials, and experimental pilot studies have found therapeutic effects of single and repeated low doses of MPH in patients with mild TBI who reported cognitive dysfunction. This literature also suggests that MPH may interact with concurrent cognitive interventions to enhance their effects. This focused review will critically evaluate the recent literature on MPH effects on cognitive dysfunction after mild to moderate TBI. To elucidate the neural mechanisms of MPH effects, this review will also include recent imaging research, preclinical, and experimental human studies.
Methylphenidate (MPH) is a dopamine and noradrenaline agonist which has stimulant effects. It is widely prescribed in clinical settings (1) and is used in research. The primary objective of this review is to describe and critique clinical trials of MPH that have focused on improving cognitive performance and cognitive (“mental”) fatigue in persons who sustain mild to moderate traumatic brain injury (TBI). Although other catecholaminergic medications will be briefly considered, we will focus on MPH because it is the most investigated drug in this category, it is widely prescribed in rehabilitation, and in follow-up care for TBI (2, 3). Related objectives include examining the premise for using MPH to treat cognitive dysfunction in TBI; brain imaging and experimental evidence for the neural mechanisms which underpin MPH's effects; methodological issues in clinical trials of MPH; and its potential role as an adjuvant in cognitive rehabilitation. The clinical trials listed in Clinical trials.gov (see https://clinicaltrials.gov; Accessed February 14, 2019) that enrolled adult participants with a spectrum of TBI severity will be summarized. Finally, we will review the subset of published investigations that studied adult participants with mild to moderate TBI.
The positive effects of MPH on cognition in conditions characterized by low dopamine are achieved by reducing reuptake of extracellular dopamine by the dopamine transporter (DAT) which is most densely represented in a group of contiguous subcortical structures in the forebrain, including the caudate, putamen, and nucleus accumbens and, to a lesser extent, in prefrontal cortex (3). Although MPH also affects reuptake of noradrenaline, the literature supports modulation of dopamine levels as the primary mechanism of clinical improvement in studies of TBI (3). MPH has also been the most frequently used dopamine agonist in clinical trials to treat cognitive deficit after TBI (3).
The premise for using MPH to ameliorate cognitive deficits is based on several related lines of research. First, evidence suggests mediation of cognitive function by stimulation of dopaminergic and noradrenergic receptors in prefrontal cortex and in subcortical regions (3, 4). Additionally, the architecture of these neuromodulatory transmitter systems renders them vulnerable to injury as they originate within brainstem nuclei, project widely throughout the brain, and include neurons with long fibers, diffuse arborization, high baseline activity, and little or no myelination (3, 5). This diffuse distribution and fragile neuronal structures make these systems especially susceptible to both mechanical and metabolic injury (e.g., diffuse axonal injury) (3). Third, animal and clinical studies have provided evidence for catecholaminergic disruption following TBI (6, 7). Finally, dopamine levels have been shown to increase during the acute post-injury period within brain areas that include the medial prefrontal cortex, striatum, brainstem, and hypothalamus (3, 8). Such increases are then followed by a hypodopaminergic state characterized by a reduction in dopamine release and other alterations that decrease the overall level of dopaminergic function (3, 9).
Similarly, shortly after TBI there is also an acute increase in noradrenaline which is then followed by a reduction in noradrenergic activity (3). Imaging studies have confirmed these changes; positron emission tomographic imaging (PET) and single photon emission computed tomography (SPECT) have shown reduced dopamine transporter (DAT) binding secondary to lower dopamine levels (3, 4, 10). Jenkins et al. (10) also found that slow cognitive processing speed after moderate to severe TBI was specifically related to reduced DAT binding in the caudate. A recent translational SPECT investigation of moderate to severe TBI patients found that those with low pretreatment DAT level in the caudate showed significant improvement in complex reaction time (RT) after taking 0.3 mg/kg MPH for 2 weeks in a randomized cross-over design (11). In contrast, complex RT did not change in a placebo condition and MPH effects on performance were not significant in patients who had normal baseline levels of DAT. Although self-rated fatigue was reduced in both the low and normal baseline DAT level subgroups, a diminution of self-rated apathy was found only in the low DAT subgroup. In summary, these studies support the premise that MPH-related improvement in cognitive performance is mediated by increased dopamine level.
Task-related fMRI has been used to explore cognitive dysfunction following TBI, including studies employing pharmacological interventions and working memory paradigms [e.g., (12, 13)]. Following mild traumatic brain injury (mTBI), fMRI showed problems with the allocation of neural resources while engaged in working memory tasks. Activation of brain regions such as dorsolateral prefrontal cortex was excessive at low to moderate task difficulty levels, while higher task demands resulted in little or no additional increase in neural processing resources (12, 14). Preliminary research has started to address how modulation of brain activation might be improved through pharmacological manipulations (6). Although most fMRI studies involving pharmacologic agents have focused on moderate to severe TBI, we included them in Table 1 because they provide proof of principle concerning dopaminergic mechanisms in task-related activation and provide a framework for investigation of drug effects to treat persistent cognitive dysfunction after less severe TBI.
Table 1. MPH interventions in adults with a history of TBI ranging from mild to severe (see ClinicalTrials.gov).
MPH is thought to act through mechanisms that block the reuptake of dopamine and noradrenaline, as well as an increase in dopamine release (3). Together, such actions elevate extracellular concentrations of both of these catecholamines to produce stimulatory effects. However, the only pharmacological studies that have used functional neuroimaging to study mTBI have used other catecholaminergic agonists, bromocriptine and guanfacine, and their action differs from that of MPH (13, 15). Bromocriptine is a selective D2 dopamine receptor agonist with complex, dose-dependent effects (3). This agonist binds to presynaptic auto-receptors which inhibit dopamine release, as well as post-synaptic sites. At high doses, the excitatory post-synaptic effect is thought to predominate with a net result that facilitates dopaminergic function. In contrast, guanfacine is a selective α-2A adrenergic agonist which acts on receptors that are concentrated predominantly within the prefrontal cortex and the locus coeruleus, resulting in stimulation of the noradrenergic system (3, 6).
Using a single-dose pharmacological challenge approach with block design fMRI, Mcallister et al. (13) and Mcallister et al. (15) examined the effects of bromocriptine or guanfacine on activation during an auditory letter n-back working memory task. In one study, they administered guanfacine to 13 mTBI patients and 14 healthy control subjects within 1 month of injury as part of a double blind, placebo-controlled crossover design (15). The n-back task had three levels of difficulty and, for mTBI patients, noradrenergic stimulation improved performance at the intermediate level (i.e., 2-back), while the control subjects experienced a decline. Functional neuroimaging with the mTBI patients revealed an activation increase within the right frontal lobe (e.g., middle frontal gyrus) during the guanfacine condition, while the control subjects had activation increases within areas outside of working memory circuitry.
Mcallister et al. (15) used the same working memory paradigm and study design with 26 mTBI patients and 31 control subjects to investigate the D2 dopamine receptor agonist bromocriptine. This manipulation did not alter performance for the control subjects, but mTBI patients experienced declines during the 0-back and 3-back task conditions. The activation patterns found with bromocriptine were essentially the opposite of those observed with guanfacine, including increased frontal activation in the control subjects and increases outside of working memory circuitry for subjects with mTBI. When considered in combination, the findings for bromocriptine and guanfacine are consistent with different neural and behavioral responses to different types of catecholaminergic intervention after mTBI.
According to Mcallister et al. (6), alterations in central catecholaminergic sensitivity impair working memory and contribute to cognitive complaints shortly after mTBI, but the effectiveness of different pharmacological interventions for improving cognitive function likely depends upon factors such as the type of catecholaminergic stimulation and the dose. In particular, stimulation of the prefrontal noradrenergic system appears to have the potential to enhance working memory performance following mTBI (15). Although these studies (13, 15) did not examine MPH in patients with mTBI, others have used functional neuroimaging to study activation changes associated with MPH in patients with injuries of greater severity [e.g., (16)].
Newsome et al. (16) examined the effects of a 1-month MPH intervention in patients with moderate to severe TBI. Using a double blind, placebo-controlled design, they administered either a placebo or 15 mg of the drug twice a day for a month with pre-treatment and end-of-treatment scanning performed using a block design visual n-back task with face stimuli. Four TBI patients received MPH and the other five were in the placebo group. In a whole brain analysis examining the 2-back minus 0-back contrast, this MPH treatment, relative to placebo, reduced activation within areas thought to have a role in working memory, such as the anterior cingulate gyrus, cuneus, and cerebellum. An a priori region of interest analysis also found treatment-related reductions within the anterior cingulate gyrus.
Studies of moderate to severe TBI using single-dose pharmacological challenge approaches have also provided evidence for alterations in working memory activation following MPH administration (17, 18). Manktelow et al. (18) used a double blind, placebo-controlled crossover design to study the effects of a single 30 mg. dose in 15 patients with moderate to severe TBI and 15 healthy controls. Using a block design visual letters n-back task, Manktelow et al. reported that the controls performed better than the TBI patients during the placebo condition, but there was no significant between-group difference after administration of the MPH. In TBI patients the drug increased task-related activation within a portion of the left cerebellum to a level comparable to controls and this change was correlated with the improvement in working memory performance. Kim et al. (17) used perfusion fMRI and a block design visual letters n-back task with 21 moderate to severe TBI patients. The pharmacological challenge consisted of a single dose (0.3 mg/kg) of MPH that was delivered as part of a randomized double-blind, placebo-controlled crossover study design. The MPH improved RT, with a trend toward greater task accuracy, and on functional neuroimaging there was also a trend toward a global reduction of cerebral blood flow under all of the task conditions, including the rest blocks. These findings suggest the possibility of a general mechanism of action for cognitive enhancement associated with MPH in patients with moderate to severe TBI.
Visual attention and response inhibition are cognitive functions that have also been studied in moderate to severe TBI patients using MPH (17, 19). In addition to the n-back working memory paradigm that was described above, Kim et al. (17) also employed the Visual Sustained Attention Task (VSAT) block design fMRI paradigm with 18 of their study participants. The administration of a single dose of MPH (0.3 mg/kg) improved both VSAT RT and accuracy. Also, during the MPH condition, there was deactivation within the left posterior superior parietal cortex that was correlated with improved RT. These authors concluded that suppression of activation within this brain region may represent a mechanism through which MPH improves visual attention impairment following TBI. Use of a single 30 mg dose has also been found to be related to activation on event-related fMRI using the stop signal task, which is a challenging measure of response inhibition. Moreno-López et al. (19) used this fMRI paradigm in a randomized double blind, placebo-controlled crossover study with 14 moderate to severe TBI patients and 20 healthy controls. Under the placebo condition the TBI patients had decreased task-related activation, relative to control subjects, within the right inferior frontal gyrus. However, the administration of MPH increased activation within this structure to a level that was similar to that of the control subjects.
In summary, fMRI research addressing neural mechanisms associated with MPH's cognitive effects following TBI has been limited. Although previous fMRI research has often reported the presence of greater and more diffuse activation following TBI (20–22), the more recent task-related fMRI studies of MPH found that the drug altered activation in the direction approximating healthy controls. However, the brain regions most affected by MPH depended on the specific tasks used as represented in Figure 1. These changes in activation were generally correlated with improved cognitive performance.
Figure 1. Brain regions in which methylphenidate (MPH) has modulated activation during performance of cognitive tasks in patients with TBI have varied depending on the specific cognitive task: (A)Visual Working Memory: activation by an n-back task for photos of faces was modulated in anterior cingulate gyrus, cuneus, and cerebellum (16), and for letters in left cerebellum (18); (B) Visual Sustained Reaction Time (RT): MPH modulated deactivation of left posterior superior parietal region (17); (C) Response Inhibition: MPH modulated activation by the stop signal RT task in the right inferior frontal gyrus (19).
These findings were interpreted as providing evidence for the normalization of working memory activation following the 1-month MPH intervention. To our knowledge, no studies have specifically studied activation changes in patients with mTBI using MPH, but the other catecholaminergic drugs guanfacine and bromocriptine have been examined using a working memory paradigm, and the findings suggest the possibility of different effects associated with the stimulation of dopaminergic and noradrenergic systems (6, 13, 15). Since MPH acts on both of these neuromodulatory systems (i.e., MPH is a dual agonist), there is a justification for further research to determine how this particular drug alters brain function to improve performance following mTBI. However, several studies with moderate to severe TBI have been conducted and, although the details of their findings are inconsistent, there is some preliminary evidence that administration of MPH may normalize the pattern of activation in a way that enhances performance.
High variability in the cognitive response and functional imaging findings may reflect heterogeneity of the neuropathology associated with TBI (3). Current status of fMRI studies using individual differences in the level and type of injury-related cognitive impairment (3, 6), non-linear relationships between catecholamine levels and cognitive function (23), subject factors such as age and genetics (3, 6), and between-study differences in research design and statistical power, also contribute to the variability in findings across studies (22). Differences in the reduction of dopamine among patients sustaining TBI of apparently similar acute severity is another source of variability in response to MPH both in changes of task related activation and improvement in cognitive performance.
Clinical trials of MPH have studied neurologic disorders involving low levels of brain dopamine, especially in the striatum. Currently over 300 clinical trials of MPH have been posted on the ClinicalTrials.gov website with 195 of them on adults (see https://clinicaltrials.gov/ct2/results?term=methylphenidate&cond=brain+injury, accessed February 01, 2019). The majority of these studies involve developmental attention-deficit/hyperactivity disorder (ADHD), followed by narcolepsy and chronic fatigue syndrome due to multiple sclerosis, other autoimmune conditions, or as a result of cancer treatment. Several trials of MPH were designed to enhance gait and balance in Parkinson's disease and to alleviate apathy in patients with Alzheimer's disease or other dementia. In contrast, relatively few trials of MPH were designed to improve cognitive functioning following TBI.
Clinical trials of MPH to treat cognitive deficits after TBI have mostly enrolled moderate to severely injured patients and these studies have been reviewed recently (2, 3, 10). Table 1 summarizes those trials in Clinical Trials.gov that focused on moderate to severe TBI or enrolled patients representing a spectrum of TBI severity. Table 2 summarizes the clinical trials of MPH and related drugs that have enrolled mild to moderate TBI patients. These clinical trials to date have aimed at treating cognitive deficit rather than impacting disability. The clinical trials included in Table 2 were identified by searching PubMed, published meta-analyses, and reviews.
The trials described below used MPH to treat persistent cognitive symptoms, cognitive impairment, and cognitive (or “mental”) fatigue following mild to moderate TBI, including patients who had mild or no impairment of consciousness but sustained brain lesions or other pathology identified by imaging (“complicated mTBI”). The rationale for focusing on mild to moderate TBI is that this range of severity accounts for over 80% of the 2.8 million acute TBI population treated in emergency departments annually in the USA (31). The subgroup of the mild to moderate TBI population who have cognitive impairment persisting for 3 months or longer is estimated to be ~15–30% which represents a large, underserved population (32). However, there is a paucity of high-quality longitudinal follow-up studies using cognitive tests to evaluate recovery in patients with this range of acute TBI severity. This gap in clinical trials of MPH for cognitive deficit after mild to moderate TBI is concerning because cognitive dysfunction impacts return to work and other activities which affect quality of life. In addressing this gap in clinical trials of MPH, it is important to consider the methodological issues in study design and conduct as described below.
There is wide variation in the severity and chronicity of TBI represented in trials that have enrolled a spectrum of TBI severity (Table 1). However, the studies summarized in Table 2 are more homogeneous as they enrolled patients with mild to moderate TBI.
There is also variation across trials in the eligibility criteria for enrollment; some screened for impaired cognitive performance in addition to self-report of cognitive dysfunction, whereas other studies relied on self-report, clinical observations and clinical judgment, and/or report by a collateral source. Studies have also differed in screening for co-morbidities, including depression, anxiety, post-traumatic stress disorder and ADHD; some trials excluded depressed patients to isolate cognitive effects of MPH from MPH- related improvement of mood. Screening for symptom validity and effort is also an issue because patients seeking compensation may exaggerate their cognitive impairment or expend less than full effort in their performance on cognitive tests.
Table 2 shows variation in study design; randomized, clinical trials using placebo-control groups have been limited by small sample sizes, whereas crossover designs have mitigated this problem. An additional advantage of crossover designs is that they are robust to the considerable heterogeneity in TBI pathology even in patients with equivalent TBI severity. The short washout (≈24 h) of MPH is also well-suited for crossover designs as placebo and drug conditions can be scheduled with separation by a brief interval. Administering MPH at the same time each day is also recommended to mitigate confounding by chronobiologic variation. However, practice effects on cognitive tests are a potential confound, arguably more so in crossover designs, especially those that are open label. In addition, patients may experience increased arousal which cues them to the MPH phase of the study.
Studies have ranged in duration of treatment from single administration of MPH and same day “challenge” testing to the cohort followed by Johansson et al. (30) who maintained MPH responders for 2 years following a 4-months interval without treatment. Table 2 shows that the dose of MPH has ranged from 20 to 30 mg in studies using a fixed dose; other studies have used 0.3 mg/kg with the constraint of a maximum dose. Johansson et al. (30) have used an individualized dose escalation strategy which is atypical in the literature. Johansson et al. (30) maintained MPH responders for 2 years following a 4-months interval without treatment. She reported that MPH effects dissipated during this 4-months drug-free period, but the therapeutic effects of MPH on processing speed, working memory, and cognitive fatigue were reinstated when the patients resumed MPH according to the regimen described below.
In a crossover trial to treat cognitive fatigue, Johansson et al. (30) used a Latin Square design wherein each patient had 4 weeks in each of three different conditions: (1) no medication, (2) low dose MPH, and (3) normal dose MPH. There was no washout period because a short-acting preparation of MPH was used. The low and normal dose conditions included dose escalation during weeks 1–3, which rose to 60 mg/day in the fourth week of the normal dose condition. Of 29 patients (age 18–65 years) who were enrolled in the trial, 5 dropped out including 4 subjects who reported adverse events. Reduction of symptoms as measured by the Mental Fatigue Scale (MFS) (33), was greater in the normal and low dose MPH conditions as compared to no medication and symptom reduction under the normal dose exceeded that of the low dose condition. Conceptually, the MFS has ecological relevance because it queries about variation in fatigue at different times of the day and its effects on psychological health and sleep. In this respect, the MFS compliments measures of cognitive performance which may take an hour or two but possibly overlook the cumulative effects of work or study over hours in the course of the patient's daily schedule (33).
Although dose escalation adds complexity to a trial, it may be more representative of clinical practice and is advisable for older patients. To this point, Jonasson et al. (30) screened for cardiovascular health, including electrocardiography at each visit. The Jonasson et al. study's dose escalation approach is noteworthy because it may have optimized MPH treatment, ostensibly reaching the top of the inverted U-shaped function as represented in Figure 2.
Figure 2. Representation of the inverted U-shaped relation of prefrontal dopamine level to cognitive performance and symptoms. This relation is based in part on animal models including the work by Arnsten et al. (4) and has been described in reports concerning the effects of dopamine agonists on cognition and behavior in humans.
The results of experimental research using animal models and clinical studies are consistent with an inverted U-shaped relation of cognitive performance to dose of MPH. Figure 2 is a hypothetical representation of the results showing that cognitive performance is optimized by a moderate level of dopamine. This relation has been inferred (but not proven) from dose ranging studies of MPH which lacked a measure of dopamine. Suboptimal levels of brain dopamine are associated with fatigue, whereas excessively high levels can increase heart rate and produce symptoms such as nervousness, increased motor activity, and sleep disturbance. Baseline levels of dopamine are lower in older adults and reduced in neurologic conditions such as Parkinson's disease. A recent clinical SPECT investigation indirectly measured dopamine level by evaluating the binding of a radio ligand to DAT which was especially evident in the caudate (10). Consistent with the inverted U-shaped relation, these investigators found that moderate to severe TBI patients responded better to MPH on cognitive tests and reduction of fatigue if their baseline levels of brain dopamine were low, whereas patients who had normal levels of dopamine did not respond as well. Based on the above studies, screening for medical history and substance abuse, serial recording of adverse effects reported by the participant, and repeated measurement of vital signs is good practice for clinical trials of MPH.
Similar to variation in the enrollment criteria, clinical trials have varied in their use of self-report vs. cognitive performance measures. As described in the preceding section, cognitive (“mental”) fatigue is a frequent complaint in the TBI population wherein the individual may be capable of performing the cognitive demands of a task or activity, but finds the process to be effortful and tiring as compared to her/his pre-injury level. Patients also reported that cognitive fatigue was present throughout the day. Interestingly, rating scale and visual analog scale measures of fatigue have been sensitive to MPH effects (30). In a recent translational study in moderate to severe TBI patients, Jenkins et al. (10) found that cognitive fatigue was sensitive to MPH in subgroups of participants who differed in level of pretreatment dopamine in the caudate based on SPECT using a ligand for dopamine.
Of the cognitive performance measures, complex RT, go no-go RT, cognitive processing speed, and set shifting tests have been widely used. Episodic multi-trial recall memory and working memory tests have also been employed, but less frequently than processing speed and RT tests. Tables 1, 2 show that some studies have relied on self-report of cognitive functioning in everyday activities as measured by various scales. From the perspective of ecological validity, a combination of cognitive performance and self-report measures is recommended as used by Jenkins et al. (10).
Few clinical trials of MPH have used composite measures to assess cognitive performance. Although a composite measure has the advantage of evaluating diverse cognitive operations, specific cognitive tasks such as working memory are supported by preclinical research implicating prefrontal dopamine receptors (4) and measures of cognitive processing speed have been especially sensitive to MPH in clinical trials. As seen in Table 2, timed tests that have been sensitive to MPH include Trail Making, Complex RT, Digit Symbol Substitution and Coding subtests, and Sternberg Memory Scanning, which measures changes in RT depending on the number of items to be held in memory.
In view of the positive effects of MPH on attention, processing speed, and fatigue, it is plausible that MPH may enhance the effects of cognitive training. In a study which enrolled patients representing a wide spectrum of acute TBI severity, Mcdonald et al. (34) found that 0.3 mg/kg of MPH taken over 7 weeks enhanced the effects of memory and attention training in a controlled study.
Despite relatively few postings on ClinicalTrials.gov, there is a considerable body of literature related to using MPH to treat cognitive complaints, cognitive deficits, and mental fatigue following mild to moderate TBI (2). Most of the previous reviews included studies with a wide range of TBI severity (with majority of them on moderate to severe range) (35, 36) and age (from children to older adults) (37). All of the above-mentioned studies have considerable limitations due to small sample sizes, enrollment of individuals with co-morbid depression (24, 27) or use of open-label design (25, 26, 28, 30). Hence, there is an evident need for well-designed and adequately powered, double blind, placebo-controlled clinical trials that will extend our knowledge of neural mechanisms of MPH effects and provide valuable information for clinicians and researchers.
Of considerable note, there is no consensus on whether to include screening measures of cognitive performance to substantiate self-report of cognitive dysfunction in everyday activities. If cognitive performance measures are used to screen for eligibility, they should tap the constructs that patients complain about. Few studies have obtained ratings of cognitive function by collateral sources and measures of symptom validity or effort expended during testing have generally not been used. Based on Mcdonald et al.'s (34) work, trials combining MPH with cognitive training also appear to be justified. Imaging biomarkers of dopamine and/or MPH effects also enhance the rigor of clinical trials.
The extant evidence supports further investigation of MPH for use in treating cognitive dysfunction and mental fatigue following mild to moderate TBI. However, there is a need for phase 3 clinical trials to evaluate the effectiveness of MPH and identifying the specific context of use in which it is most strongly indicated.
HL, RS, and MT wrote the main manuscript text. EW, TA, and JP edited the paper. TA designed and produced the figures. JH reviewed and verified manuscript and figures. JP revised and formatted the manuscript according to the journal's guidelines.
This work was supported by the National Institutes of Health (NIH) (Grant NS42772; PI: HL); and the VA Merit Review Award Number I01 CX001820 (PI: RS).
The authors declare that the research was conducted in the absence of any commercial or financial relationships that could be construed as a potential conflict of interest.
1. Challman TD, Lipsky JJ. Methylphenidate: its pharmacology and uses. Mayo Clin Proc. (2000) 75:711–21. doi: 10.1016/S0025-6196(11)64618-1
2. Huang CH, Huang CC, Sun CK, Lin GH, Hou WH. Methylphenidate on cognitive improvement in patients with traumatic braininjury: a meta-analysis. Curr Neuropharmacol. (2016) 14:272–81. doi: 10.2174/1570159X13666150514233033
3. Jenkins PO, Mehta MA, Sharp DJ. Catecholamines and cognition after traumatic brain injury. Brain. (2016) 139:2345–71. doi: 10.1093/brain/aww128
4. Arnsten AF, Wang MJ, Paspalas CD. Neuromodulation of thought: flexibilities and vulnerabilities in prefrontal cortical network synapses. Neuron. (2012) 76:223–39. doi: 10.1016/j.neuron.2012.08.038
5. Nieuwenhuys R. Chemoarchitecture of the Brain. Berlin: Springer-Verlag. (1985). doi: 10.1007/978-3-642-70426-0
6. Mcallister TW, Flashman LA, Mcdonald BC, Saykin AJ. Mechanisms of working memory dysfunction after mild and moderate TBI: evidence from functional MRI and neurogenetics. J Neurotrauma. (2006) 23:1450–67. doi: 10.1089/neu.2006.23.1450
7. Kobori N, Hu B, Dash PK. Altered adrenergic receptor signaling following traumatic brain injury contributes to working memory dysfunction. Neuroscience. (2011) 172:293–302. doi: 10.1016/j.neuroscience.2010.10.048
8. Kobori N, Clifton GL, Dash PK. Enhanced catecholamine synthesis in the prefrontal cortex after traumatic brain injury: implications for prefrontal dysfunction. J Neurotrauma. (2006) 23:1094–102. doi: 10.1089/neu.2006.23.1094
9. Wagner AK, Sokoloski JE, Ren D, Chen X, Khan AS, Zafonte RD, et al. Controlled cortical impact injury affects dopaminergic transmission in the rat striatum. J Neurochem. (2005) 95:457–65. doi: 10.1111/j.1471-4159.2005.03382.x
10. Jenkins PO, De Simoni S, Bourke NJ, Fleminger J, Scott G, Towey DJ, et al. Dopaminergic abnormalities following traumatic brain injury. Brain. (2018) 141:797–810. doi: 10.1093/brain/awx357
11. Jenkins PO, De Simoni S, Bourke NJ, Fleminger J, Scott G, Towey DJ, et al. Stratifying drug treatment of cognitive impairments after traumatic brain injury using neuroimaging. Brain. (2019) 142:2367–79. doi: 10.1093/brain/awz149
12. Mcallister TW, Saykin AJ, Flashman LA, Sparling MB, Johnson SC, Guerin SJ, et al. Brain activation during working memory 1 month after mild traumatic brain injury: a functional MRI study. Neurology. (1999) 53:1300–8. doi: 10.1212/WNL.53.6.1300
13. Mcallister TW, Flashman LA, Mcdonald BC, Ferrell RB, Tosteson TD, Yanofsky NN, et al. Dopaminergic challenge with bromocriptine one month after mild traumatic brain injury: altered working memory and BOLD response. J Neuropsychiatry Clin Neurosci. (2011) 23:277–86. doi: 10.1176/appi.neuropsych.23.3.277
14. Mcallister TW, Sparling MB, Flashman LA, Guerin SJ, Mamourian AC, Saykin AJ. Differential working memory load effects after mild traumatic brain injury. Neuroimage. (2001) 14:1004–12. doi: 10.1006/nimg.2001.0899
15. Mcallister TW, Mcdonald BC, Flashman LA, Ferrell RB, Tosteson TD, Yanofsky NN, et al. Alpha-2 adrenergic challenge with guanfacine one month after mild traumatic brain injury: altered working memory and BOLD response. Int J Psychophysiol. (2011) 82:107–14. doi: 10.1016/j.ijpsycho.2011.06.022
16. Newsome MR, Scheibel RS, Seignourel PJ, Steinberg JL, Troyanskaya M, Li X, et al. Effects of methylphenidate on working memory in traumatic brain injury: a preliminary FMRI investigation. Brain Imaging Behav. (2009) 3:298–305. doi: 10.1007/s11682-009-9072-5
17. Kim J, Whyte J, Patel S, Europa E, Wang J, Coslett HB, et al. Methylphenidate modulates sustained attention and cortical activation in survivors of traumatic brain injury: a perfusion fMRI study. Psychopharmacology. (2012) 222:47–57. doi: 10.1007/s00213-011-2622-8
18. Manktelow AE, Menon DK, Sahakian BJ, Stamatakis EA. Working memory after traumatic brain injury: the neural basis of improved performance with methylphenidate. Front Behav Neurosci. (2017) 11:58. doi: 10.3389/fnbeh.2017.00058
19. Moreno-Lopez L, Manktelow AE, Sahakian BJ, Menon DK, Stamatakis EA. Anything goes? Regulation of the neural processes underlying response inhibition in TBI patients. Eur Neuropsychopharmacol. (2017) 27:159–69. doi: 10.1016/j.euroneuro.2016.12.002
20. Hillary FG. Neuroimaging of working memory dysfunction and the dilemma with brain reorganization hypotheses. J Int Neuropsychol Soc. (2008) 14:526–34. doi: 10.1017/S1355617708080788
21. Hillary FG, Roman CA, Venkatesan U, Rajtmajer SM, Bajo R, Castellanos ND. Hyperconnectivity is a fundamental response to neurological disruption. Neuropsychology. (2015) 29:59–75. doi: 10.1037/neu0000110
22. Scheibel RS. Functional magnetic resonance imaging of cognitive control following traumatic brain injury. Front Neurol. (2017) 8:352. doi: 10.3389/fneur.2017.00352
23. Cools R, D'esposito M. Inverted-U-shaped dopamine actions on human working memory and cognitive control. Biol Psychiatry(2011) 69:e113–25. doi: 10.1016/j.biopsych.2011.03.028
24. Lee H, Kim SW, Kim JM, Shin IS, Yang SJ, Yoon JS. Comparing effects of methylphenidate, sertraline and placebo on neuropsychiatric sequelae in patients with traumatic brain injury. Hum Psychopharmacol. (2005) 20:97–104. doi: 10.1002/hup.668
25. Johansson B, Wentzel AP, Andrell P, Odenstedt J, Mannheimer C, Ronnback L. Evaluation of dosage, safety and effects of methylphenidate on post-traumatic brain injury symptoms with a focus on mental fatigue and pain. Brain Inj. (2014) 28:304–10. doi: 10.3109/02699052.2013.865267
26. Johansson B, Wentzel AP, Andrell P, Mannheimer C, Ronnback L. Methylphenidate reduces mental fatigue and improves processing speed in persons suffered a traumatic brain injury. Brain Inj. (2015) 29:758–65. doi: 10.3109/02699052.2015.1004747
27. Mcallister TW, Zafonte R, Jain S, Flashman LA, George MS, Grant GA, et al. Randomized placebo-controlled trial of methylphenidate or galantamine for persistent emotional and cognitive symptoms associated with PTSD and/or traumatic brain injury. Neuropsychopharmacology. (2016) 41:1191–8. doi: 10.1038/npp.2015.282
28. Johansson B, Wentzel AP, Andrell P, Ronnback L, Mannheimer C. Long-term treatment with methylphenidate for fatigue after traumatic brain injury. Acta Neurol Scand. (2017) 135:100–7. doi: 10.1111/ane.12587
29. Zhang WT, Wang YF. Efficacy of methylphenidate for the treatment of mental sequelae after traumatic brain injury. Medicine. (2017) 96:e6960. doi: 10.1097/MD.0000000000006960
30. Johansson B, Wentzel AP, Andrell P, Ronnback L, Mannheimer C. Two-year methylphenidate treatment of mental fatigue and cognitive function after a traumatic brain injury: a clinical prospective study. J Clin Psychopharmacol. (2018) 38:164–5. doi: 10.1097/JCP.0000000000000854
31. Taylor CA, Bell JM, Breiding MJ, Xu L. Traumatic brain injury-related emergency department visits, hospitalizations, and deaths - United States, 2007 and 2013. MMWR Surveill Summ. (2017) 66:1–16. doi: 10.15585/mmwr.ss6609a1
32. Carroll LJ, Cassidy JD, Cancelliere C, Cote P, Hincapie CA, Kristman VL, et al. Systematic review of the prognosis after mild traumatic brain injury in adults: cognitive, psychiatric, and mortality outcomes: results of the International Collaboration on Mild Traumatic Brain Injury Prognosis. Arch Phys Med Rehabil. (2014) 95:S152–73. doi: 10.1016/j.apmr.2013.08.300
33. Jonasson A, Levin C, Renfors M, Strandberg S, Johansson B. Mental fatigue and impaired cognitive function after an acquired brain injury. Brain Behav. (2018) 8:e01056. doi: 10.1002/brb3.1056
34. Mcdonald BC, Flashman LA, Arciniegas DB, Ferguson RJ, Xing L, Harezlak J, et al. Methylphenidate and memory and attention adaptation training for persistent cognitive symptoms after traumatic brain injury: a randomized, placebo-controlled trial. Neuropsychopharmacology. (2017) 42:1766–75. doi: 10.1038/npp.2016.261
35. Sivan M, Neumann V, Kent R, Stroud A, Bhakta BB. Pharmacotherapy for treatment of attention deficits after non-progressive acquired brain injury. A systematic review. Clin Rehabil. (2010) 24:110–21. doi: 10.1177/0269215509343234
36. Frenette AJ, Kanji S, Rees L, Williamson DR, Perreault MM, Turgeon AF, et al. Efficacy and safety of dopamine agonists in traumatic brain injury: a systematic review of randomized controlled trials. J Neurotrauma. (2012) 29:1–18. doi: 10.1089/neu.2011.1812
Keywords: traumatic brain injury, methylphenidate, clinical trials, imaging, dopamine, cognition
Citation: Levin H, Troyanskaya M, Petrie J, Wilde EA, Hunter JV, Abildskov TJ and Scheibel RS (2019) Methylphenidate Treatment of Cognitive Dysfunction in Adults After Mild to Moderate Traumatic Brain Injury: Rationale, Efficacy, and Neural Mechanisms. Front. Neurol. 10:925. doi: 10.3389/fneur.2019.00925
Received: 20 February 2019; Accepted: 09 August 2019;
Published: 12 September 2019.
Edited by:
Karen M. Barlow, University of Queensland, AustraliaReviewed by:
Owen Thomas Lloyd, Children's Health Queensland, AustraliaCopyright © 2019 Levin, Troyanskaya, Petrie, Wilde, Hunter, Abildskov and Scheibel. This is an open-access article distributed under the terms of the Creative Commons Attribution License (CC BY). The use, distribution or reproduction in other forums is permitted, provided the original author(s) and the copyright owner(s) are credited and that the original publication in this journal is cited, in accordance with accepted academic practice. No use, distribution or reproduction is permitted which does not comply with these terms.
*Correspondence: Harvey Levin, aGxldmluQGJjbS5lZHU=
Disclaimer: All claims expressed in this article are solely those of the authors and do not necessarily represent those of their affiliated organizations, or those of the publisher, the editors and the reviewers. Any product that may be evaluated in this article or claim that may be made by its manufacturer is not guaranteed or endorsed by the publisher.
Research integrity at Frontiers
Learn more about the work of our research integrity team to safeguard the quality of each article we publish.