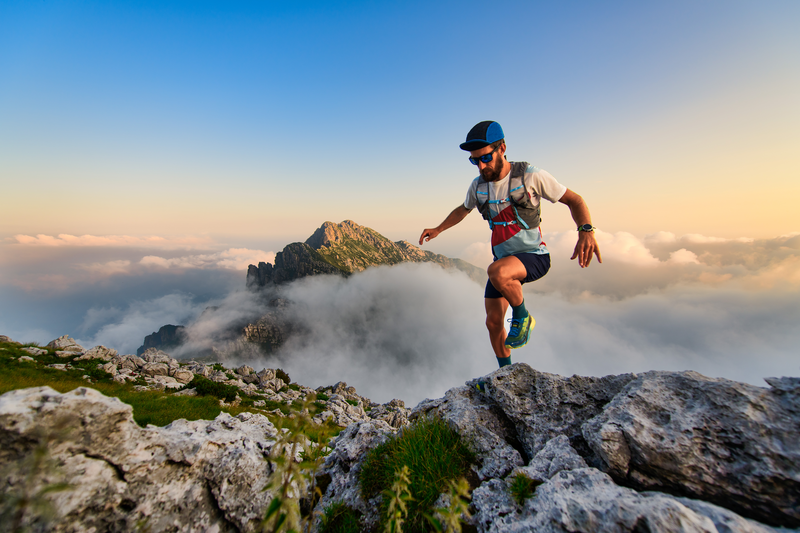
95% of researchers rate our articles as excellent or good
Learn more about the work of our research integrity team to safeguard the quality of each article we publish.
Find out more
ORIGINAL RESEARCH article
Front. Neurol. , 24 July 2019
Sec. Neurorehabilitation
Volume 10 - 2019 | https://doi.org/10.3389/fneur.2019.00776
This article is part of the Research Topic Performance and Participation Outcomes for Individuals with Neurological Conditions View all 17 articles
Background: Recovery from post-stroke aphasia is important for performing the activities of daily life, returning to work, and quality of life. We investigated the association between specific brain lesions and the long-term outcome of four dimensions of aphasia: fluency, comprehension, naming, and repetition 12 months after onset in patients with stroke.
Methods: Our retrospective cross-sectional observational study investigated the relationship between the Korean version of the Western Aphasia Battery scores in 31 stroke patients 1 year after the onset of stroke and stroke lesion location. Brain lesions were assessed using voxel-based lesion symptom mapping (VLSM) in conjunction with magnetic resonance imaging.
Results: Damage to the Rolandic cortex, Heschl's gyrus, the posterior corona radiata, supramarginal cortex, superior longitudinal fasciculus, superior temporal gyrus, and insula was associated with a low total AQ score. Lesions in the inferior triangularis and inferior operculum of the frontal cortex, supramarginal cortex, and insula were associated with a poor fluency outcome. Damage to the parietal cortex, angular cortex, temporal middle cortex, sagittal stratum, and temporal superior cortex was associated with poor recovery of comprehension skills. Lesions in the angular cortex, supramarginal cortex, posterior corona radiata, superior longitudinal fasciculus, internal capsule, temporal superior cortex, and temporal middle cortex were associated with poor recovery of naming in patients with stroke. Damage to the superior temporal cortex, posterior corona radiata, and superior longitudinal fasciculus was associated with poor recovery of repetition component.
Conclusions: We identified specific brain lesions associated with long-term outcomes in four dimensions of aphasia, in patients with post-stroke aphasia. Our findings may be useful for advancing understanding for the pathophysiology of aphasia in stroke patients.
Aphasia is a language disorder typically caused by stroke-related damage to the dominant hemisphere. Post-stroke aphasia is associated with mortality, disability, and the use of health services. The long-term outcomes for post-stroke aphasia vary widely. Recovery from aphasia is important for performing the activities of daily life, returning to work, and quality of life in patients with stroke (1).
Several studies have investigated the prognosis of speech and language after stroke. Factors predicting post-stroke aphasia recovery include lesion size and location, aphasia severity, and the type of speech deficit (2). Additionally, stroke severity at onset and the Western Aphasia Battery (WAB) score 72 h after onset (3, 4), and the specific brain regions damaged, affect the long-term prognosis of post-stroke aphasia (2, 5–8). The involvement of Broca's area, the superior temporal gyrus, arcuate fasciculus, and superior longitudinal fasciculus are associated with a poor prognosis in patients with chronic post-stroke aphasia at 4–6 months after onset (5, 6, 8). However, the timing of 4–6 months after onset might be less sufficient to reflect full functional recovery, further neurological recovery is thought to be likely (9). Knowledge of the role or specific brain lesions may facilitate the understanding of the pathophysiology of aphasia and could then be used as basis for neuromodulation strategies for aphasia, such as repetitive trans-magnetic stimulation or trans-cranial electric stimulation (10–12).
Thus, we investigated the roles that specific brain lesions play in recovery of deficits in fluency, comprehension, naming and repetition of language, using lesion symptom mapping methods that included analyses of brain magnetic resonance imaging (MRI) scans and clinical language test, in patients with first-ever supratentorial strokes 12 months after stroke onset.
Our retrospective observational study included the data of 31 post-stroke aphasia, right-handed patients with first-stroke patients recruited from a single inpatient/outpatient center between January 2011 and December 2017. The inclusion criteria were: (1) first-ever supratentorial stroke, (2) a single ischemic or hemorrhagic left hemisphere lesion confirmed by magnetic resonance imaging (MRI), (3) right handedness, (4) native Korean speaker, (5) at least 9-years of education, (6) no prior history of psychiatric or neurological disorders, (7) absence of central or peripheral paretic dysarthria, and (8) completion of a language assessment using the Korean version of the Western Aphasia Battery (K-WAB) 1 year after the onset of stroke (6). No restrictions were placed on the type or severity of the aphasia. Subjects were excluded if they had any other functional or structural brain disorder. Similarly, to previous studies for brain lesion analysis, we included the patients with stroke, regardless of type; ischemic and hemorrhagic (13–15). Of the 214 individuals who underwent language assessment for aphasia after stroke, 31 qualified for the study. All subjects received speech therapy and other rehabilitation therapy; physical or occupational therapies as needed. The rehabilitation program of all subjects had started within 5 days after onset. The speech therapy continued up to 12 months after onset, and consisted of with 0.5–2 h per week, respectively.
Demographic characteristics and language assessments were obtained for all subjects. High-resolution 1.5T anatomical MRI scans with 5-mm slice thickness were used to determine the precise location of the brain lesion (16). All participants underwent the same MRI scanning protocol. We used a 1.5-Tesla Philips MR scanner (ACHIEVA, Philips, Netherlands). The MRI protocol included whole-brain, three-dimensional, T1-weight images (TR/TE = 527.4/13, Slice thickness 5 mm, GAP 1.5 mm, flip angle 90', Refocus angle 180', FOV 230*230, Matrix 304*222, Voxel size 0.75*1.02*5 mm, NEX 2), T2-weight images (TR/TE = 4500/100, Slice thickness 5 mm, GAP 1.5 mm, flip angle 90', Refocus angle 160', FOV 230*230, Matrix 384*233, Voxel size 0.6*0.86*5 mm, NEX 2), and fluid-attenuated inversion-recovery (FLAIR) axial images (TR/TE = 6000/100, Slice thickness 5 mm, GAP 1.5 mm, flip angle 90', Refocus angle 150', FOV 230*230, Matrix 304*222, Voxel size 0.75*1.02*5 mm, NEX 2). The study protocol was reviewed and approved by the Institutional Review Board of The Catholic University, College of Medicine (Registry No. VC18RESI0112). The requirement for informed consent was waived by the board.
The validated K-WAB was administered to all patients on admission by a single speech language pathologist; however, only data from cases of aphasia caused by first-ever stroke were subjected to analysis. The K-WAB assessment consists of four subsets: fluency, comprehension, repetition, and naming (7, 17). The severity of aphasia was quantified using the aphasia quotient (AQ; range, 0–100), which was calculated using the formula developed by Kertesz (fluency score + comprehension score/20 + naming score/10 + repetition score/10) ×2 (17). The data of K-WAB measured at 1 year after onset were used for outcome of language recovery.
Lesion locations and sizes were assessed using MRIcron software (http://www.mricro.com/mricron). T2 images were co-registered with each participant's T1 MRI, and then, the T1 and lesion maps were normalized to the Montreal Neurologic Institute (MNI) template using statistical parametric mapping 8 software (SPM8, http://www.fil.ion.ucl.ac.uk/spm/software/spm8) and non-parametric mapping (NPM) software (18–20). The number of MRI voxels in each stroke lesion was calculated, and the lesions were traced by a trained image analyst and confirmed by an experienced physiatrist (a neurorehabilitation specialist), who was blind to all clinical data. Only voxels indicating that at least 10% (n = 3) of the patients had lesions were included in the final analysis. The non-parametric Brunner–Munzel test for continuous data was used (15). Colored VLSM maps representing the z statistics were generated and overlaid onto the automated anatomical labeling and Johns Hopkins University white matter templates provided with the MRIcron software (18, 21).
The study included 31 patients (mean age, 55.5 ± 11.5 years; 15 females and 16 males). The mean time from the onset of stroke to the language assessment was 725.9 ± 233.4 days, and the mean brain lesion volume was 55482.16 ± 43109.30 voxels. Patient clinical and demographic data are shown in Table 1. The language assessment findings are shown in Supplementary Table 1.
An overlap map of the 31 lesions was created (Figure 1). The VLSM analyses using NPM revealed that lesions of the Rolandic cortex, Heschl's gyrus, posterior corona radiata, supramarginal cortex, superior longitudinal fasciculus (SLF), superior temporal gyrus, and insula were associated with a low total AQ (Table 2, Figure 2). The frontal inferior triangularis, frontal inferior operculum, supramarginal cortex, and insula were associated with fluency; the parietal cortex, angular cortex, temporal middle cortex, sagittal stratum, and temporal superior cortex were associated with comprehension; the angular cortex, supramarginal cortex, posterior corona radiata, SLF, internal capsule, temporal superior cortex, and temporal middle cortex were associated with naming; and the temporal superior cortex, posterior corona radiata, and SLF were associated with repetition (Table 3, Figure 3).
Figure 1. A lesion overlap map of all subjects (n = 31). The color spectrum indicates the frequency of overlap.
Figure 2. Voxel-based lesion-symptom mapping of the total aphasia quotient after application of the non-parametric Brunner–Munzel test. The color scale indicates Brunner–Munzel rank order z statistics. Only voxels significant at p < 0.05 are shown. The statistical map shows voxels with a minimum Z score of 1.89797 and maximum range of 4, which was the maximum brightness.
Figure 3. Voxel-based lesion-symptom mapping (VLSM) of the language deficits after the non-parametric Brunner–Munzel statistical analysis. The color scale indicates Brunner–Munzel rank order z statistics. Only voxels significant at p < 0.05 are shown. The maximum range of the z score was set at 4, which was the maximum brightness. (A) VLSM for fluency with a minimum z score of 2.32076. (B) VLSM for comprehension with a minimum Z score of 2.50055. (C) VLSM for naming with a minimum Z score of 2.38888. (D) VLSM for repetition with a minimum Z score of 3.19465.
The damage of supramarginal cortex affected fluency and naming. The temporal middle cortex and angular cortex related with recovery of comprehension and naming. The SLF and posterior corona radiata may affect the recovery of repetition and naming. The damage of the temporal superior cortex may affect outcome of comprehension, naming and repetition.
We found that lesion location was associated with long-term outcomes in fluency, comprehension, naming, and repetition in patients with aphasia at 12 months after stroke onset. Damage to the Rolandic cortex, Heschl's gyrus, posterior corona radiata, supramarginal cortex, superior longitudinal fasciculus, superior temporal gyrus, and insula were associated with overall poor outcomes. Lesions in the inferior triangularis and inferior operculum of the frontal cortex, supramarginal cortex, and insula were associated with poor fluency. Damage to the parietal cortex, angular cortex, temporal middle cortex, sagittal stratum, and temporal superior cortex was associated with poor comprehension skills. Lesions in the angular cortex, supramarginal cortex, posterior corona radiata, superior longitudinal fasciculus, internal capsule, temporal superior cortex, and temporal middle cortex were associated with poor recovery of naming ability, and damage to the superior temporal cortex, posterior corona radiata, and superior longitudinal fasciculus was related to poor recovery of repetition skills. These findings suggest that specific lesion sites are predictors of aphasia recovery in patients with first-ever stroke 12 months after onset.
Nowadays, neuromodulation with repetitive magnetic stimulation or trans-cranial electric stimulation for stroke patients has been widely investigated (12, 22–25). For neuromodulation, determining target specific brain lesion is important (10, 24, 26). Thus, the functions of specific brain lesions for stroke have been uncovered; motor of upper limb, gait, balance, and language (6, 13, 19, 27). These investigations would be useful for understanding of functional deficit of stroke, itself, and also for determining target brain lesion in neuromodulation therapy. Our findings may contribute to the understanding of aphasia itself, and further to usage of neuromodulation therapy of aphasia.
Previous studies have shown that the language network involving Broca's complex, including the inferior prefrontal gyrus, insular cortex, Wernicke's complex, premotor cortex, and superior temporal gyrus play a major role in language (6, 28, 29). Moreover, white matter including the arcuate fascicle, SLF, uncinate fascicle, and the extreme capsule fiber system play a functional role in language processing (5, 7, 29). Another recent study showed that the damage to the arcuate fasciculus related to the recovery of aphasia, and damage to the external capsule also affected the recovery of aphasia (14). Our finding that lesions in the supramarginal cortex, superior temporal gyrus, insula, and SLF were associated with a low AQ score in patients with stroke is consistent with that of previous studies. A previous case report wrote that the Rolandic cortex is the cause of transcortical motor aphasia (30), the Heschl's gyrus is involved in the comprehension of syntax (31), and posterior corona radiata lesions are associated with poor recovery from aphasia (32). These lesions had not been considered as the main cause for aphasia (8). Our findings suggest that the Rolandic cortex, Heschl's gyrus, and posterior corona radiata may be new causative lesions for post-stroke aphasia. Taken together, these findings suggest that the Rolandic cortex, Heschl's gyrus, and posterior corona radiata play roles in the recovery of post-stroke aphasia via the lesion itself, or in disruptions of language network (7).
The role of the Broca's complex in verbal fluency is well-known (28); however, our findings suggest that the inferior triangularis and inferior operculum also play significant roles in mediating fluency. Moreover, the insula cortex has been shown to support fluency as a syntax-specific process (33). A recent lesion-symptom correlational analysis found that the posterior supramarginal gyrus played a role in the processing of concrete and abstract verbs related to fluency (34). We found that damage to the inferior triangularis and inferior operculum of the frontal cortex, supramarginal cortex, and insula were associated with poor fluency. Language fluency is a complex process involving phonation, motor function, syntax synthesis, and semantic meaning; thus, it is not surprising that it is mediated by a network of brain regions. The recent repetitive transcranial magnetic stimulation was consistent with the inhibition of contra-lesional pars triangularis and pars opecularis (24, 35, 36). Our results support the rationale of the target for neuromodulation.
The results that parietal cortex, angular cortex, temporal middle cortex, and temporal superior cortex lesions were associated with poor recovery of comprehension is consistent with previous findings (37–39). Moreover, our finding that the sagittal stratum pathway may mediate language comprehension supports that of a previous study showing that sagittal stratum damage, including the geniculostriate pathway and inferior longitudinal fasciculus, impaired access to orthographic word forms and semantics (40). A recent study of post-stroke aphasia found that the posterior superior temporal gyrus, intraparietal sulcus, and pars triangularis were involved in naming ability (41). We found that the superior temporal cortex, temporal middle cortex, angular cortex, supramarginal cortex, posterior corona radiata, superior longitudinal fasciculus, and internal capsule were associated with poor recovery of naming in stroke patients with aphasia. Naming is higher-order function involving comprehension, semantic, syntax, phonation, and speech. Thus, several lesion sites may affect naming ability.
We found that damages to the superior temporal cortex, posterior corona radiata, and SLF were associated with poor repetition outcomes in post-stroke aphasia patients. A previous study found that the left inferior fronto-occipital fascicle and uncinate fascicle were associated with repetition (42). Another study, which had a small sample (11 aphasic subjects), found that impaired repetition was associated with lesions in the central operculum, angular gyrus, supramarginal gyrus, and Heschl's gyrus in the acute phase of stroke (43). Taken together, these findings indicate that the brain regions involved in repetition deficits were related to those in fluency and comprehension and the white matter connecting them.
The supramarginal cortex, temporal superior and middle cortex, angular cortex, SLF, and posterior corona radiata contributed to two or more functions. Possible explanations were as follows. First, these areas would contribute to several roles, indirectly. Thus, influence from a distinct area might affect our results. Based on the brain network, the studies for a specific brain lesion may be not sufficient to explain all pathogenesis of aphasia. Second, four subsets of aphasia have developed, based on clinical phenotype. The mismatch between the process in brain and clinical phenotype would contribute to our results. However, the diagnosis and treatment should be reliant on clinical phenotype. Our results revealed the specific brain lesion on long-term outcome of aphasia with four subsets; fluency, comprehension, repetition, and naming, in patients with stroke. These results would be useful in the clinical setting. For example, for the planning of non-invasive neuromodulation therapy, our results may have merit for the decision of the target area individually, based on the patient's MRI findings and K-WAB results.
Our study has two major limitations. First, our small sample size and cross-sectional design may limit the interpretation of our findings. Second, our sample may have been affected by selection bias because we excluded the data of subjects who died 12 months post-stroke; thus, the study included relatively well patients who would not normally undergo the K-WAB test 12 months post-stroke. Nonetheless, we identified specific brain areas associated with four language deficits in patients with first-ever stroke 12 months after onset. Our study differs from those conducted previously in that we investigated lesion locations associated with four aphasia deficits using VLSM, and we used data obtained 12 months after stroke onset when further neurological recovery is thought to be unlikely (6, 42, 43).
In conclusion, we identified specific brain lesions associated with long-term outcome with K-WAB and acute MRI data in four language deficits 12 months after onset in stroke patients with aphasia using VLSM. Our findings may be useful for the development of treatment strategies and for advancing understanding of the pathophysiology of aphasia in stroke patients.
All datasets generated for this study are included in the manuscript and/or the Supplementary Files.
The study protocol was reviewed and approved by the Institutional Review Board of The Catholic University, College of Medicine (Registry No. VC18RESI0112). The requirement for informed consent was waived by the board.
BS and KL: making concept, analysis of results, and writing draft. JSK and BH: providing subjects and review of draft. JK: analysis of results and acquisition of data. WH: acquisition of data. SL: making concept, analysis of results, writing draft, review, and finalize of draft.
This research was supported by Basic Science Research Program through the National Research Foundation of Korea (NRF) funded by the Ministry of Science and ICT (grant number: 2017R1E1A1A01074324).
The authors declare that the research was conducted in the absence of any commercial or financial relationships that could be construed as a potential conflict of interest.
The Supplementary Material for this article can be found online at: https://www.frontiersin.org/articles/10.3389/fneur.2019.00776/full#supplementary-material
1. Kim G, Min D, Lee EO, Kang EK. Impact of co-occurring dysarthria and aphasia on functional recovery in post-stroke patients. Ann Rehabil Med. (2016) 40:1010–7. doi: 10.5535/arm.2016.40.6.1010
2. Watila MM, Balarabe SA. Factors predicting post-stroke aphasia recovery. J Neurol Sci. (2015) 352:12–8. doi: 10.1016/j.jns.2015.03.020
3. Lazar RM, Minzer B, Antoniello D, Festa JR, Krakauer JW, Marshall RS. Improvement in aphasia scores after stroke is well predicted by initial severity. Stroke. (2010) 41:1485–8. doi: 10.1161/STROKEAHA.109.577338
4. Harvey RL. Predictors of functional outcome following stroke. Phys Med Rehabil Clin N Am. (2015) 26:583–98. doi: 10.1016/j.pmr.2015.07.002
5. Ivanova MV, Isaev DY, Dragoy OV, Akinina YS, Petrushevskiy AG, Fedina ON, et al. Diffusion-tensor imaging of major white matter tracts and their role in language processing in aphasia. Cortex. (2016) 85:165–81. doi: 10.1016/j.cortex.2016.04.019
6. Sul B, Kim JS, Hong BY, Lee KB, Hwang WS, Kim YK, et al. The prognosis and recovery of aphasia related to stroke lesion. Ann Rehabil Med. (2016) 40:786–93. doi: 10.5535/arm.2016.40.5.786
7. Thiel A, Zumbansen A. The pathophysiology of post-stroke aphasia: a network approach. Restor Neurol Neurosci. (2016) 34:507–18. doi: 10.3233/RNN-150632
8. Hillis AE, Beh YY, Sebastian R, Breining B, Tippett DC, Wright A, et al. Predicting recovery in acute poststroke aphasia. Ann Neurol. (2018) 83:612–22. doi: 10.1002/ana.25184
9. Pedersen PM, Vinter K, Olsen TS. Aphasia after stroke: type, severity and prognosis. Copenhagen Aphasia Study Cerebrovasc Dis. (2004) 17:35–43. doi: 10.1159/000073896
10. Barwood CHS, Murdoch BE, Whelan BM, Lloyd D, Riek S, O'sullivan JD, et al. Improved receptive and expressive language abilities in nonfluent aphasic stroke patients after application of rTMS: an open protocol case series. Brain Stimul. (2012) 5:274–86. doi: 10.1016/j.brs.2011.03.005
11. Li Y, Qu Y, Yuan M, Du T. Low-frequency repetitive transcranial magnetic stimulation for patients with aphasia after stoke: a meta-analysis. J Rehabil Med. (2015) 47:675–81. doi: 10.2340/16501977-1988
12. Seo HY, Kim GW, Won YH, Park SH, Seo JH, Ko MH. Changes in intracortical excitability of affected and unaffected hemispheres after stroke evaluated by paired-pulse transcranial magnetic stimulation. Ann Rehabil Med. (2018) 42:495–501. doi: 10.5535/arm.2018.42.4.495
13. Moon HI, Lee HJ, Yoon SY. Lesion location associated with balance recovery and gait velocity change after rehabilitation in stroke patients. Neuroradiology. (2017) 59:609–18. doi: 10.1007/s00234-017-1840-0
14. Forkel SJ, Catani M. Lesion mapping in acute stroke aphasia and its implications for recovery. Neuropsychologia. (2018) 115:88–100. doi: 10.1016/j.neuropsychologia.2018.03.036
15. Lee KB, Hong BY, Kim JS, Sul B, Yoon SC, Ji EK, et al. Which brain lesions produce spasticity? An observational study on 45 stroke patients. PLoS ONE. (2019) 14:e0210038. doi: 10.1371/journal.pone.0210038
16. Lee KB, Kim JS, Hong BY, Kim YD, Hwang BY, Lim SH. The motor recovery related with brain lesion in patients with intracranial hemorrhage. Behav Neurol. (2015) 2015:6. doi: 10.1155/2015/258161
17. Kertesz A, Poole E. The aphasia quotient: the taxonomic approach to measurement of aphasic disability. Can J Neurol Sci. (1974) 1:7–16. doi: 10.1017/S031716710001951X
18. Bates E, Wilson SM, Saygin AP, Dick F, Sereno MI, Knight RT, et al. Voxel-based lesion-symptom mapping. Nat Neurosci. (2003) 6:448–50. doi: 10.1038/nn1050
19. Lee KB, Kim JS, Hong BY, Sul B, Song S, Sung WJ, et al. Brain lesions affecting gait recovery in stroke patients. Brain Behav. (2017) 7:e00868. doi: 10.1002/brb3.868
20. Lee KB, Lim SH. Prognosis and recovery of motor function with lesion–symptom mapping in patients with stroke. Brain Neurorehabil. (2017) 10:e5. doi: 10.12786/bn.2017.10.e5
21. Rorden C, Karnath HO, Bonilha L. Improving lesion-symptom mapping. J Cogn Neurosci. (2007) 19:1081–8. doi: 10.1162/jocn.2007.19.7.1081
22. Louise-Bender Pape T, Rosenow J, Lewis G, Ahmed G, Walker M, Guernon A, et al. Repetitive transcranial magnetic stimulation-associated neurobehavioral gains during coma recovery. Brain Stimul. (2009) 2:22–35. doi: 10.1016/j.brs.2008.09.004
23. Chang WH, Kim YH, Bang OY, Kim ST, Park YH, Lee PK. Long-term effects of rTMS on motor recovery in patients after subacute stroke. J Rehabil Med. (2010) 42:758–64. doi: 10.2340/16501977-0590
24. Ren CL, Zhang GF, Xia N, Jin CH, Zhang XH, Hao JF, et al. Effect of low-frequency rTMS on aphasia in stroke patients: a meta-analysis of randomized controlled trials. PLoS ONE. (2014) 9:e102557. doi: 10.1371/journal.pone.0102557
25. Simonetta-Moreau M. Non-invasive brain stimulation (NIBS) and motor recovery after stroke. Ann Phys Rehabil Med. (2014) 57:530–42. doi: 10.1016/j.rehab.2014.08.003
26. Shon YM, Lim SC, Lim SH. Therapeutic effect of repetitive transcranial magnetic stimulation on non-lesional focal refractory epilepsy. J Clin Neurosci. (2019) 63:130–3. doi: 10.1016/j.jocn.2019.01.025
27. Moon HI, Pyun SB, Tae WS, Kwon HK. Neural substrates of lower extremity motor, balance, and gait function after supratentorial stroke using voxel-based lesion symptom mapping. Neuroradiology. (2016) 58:723–31. doi: 10.1007/s00234-016-1672-3
28. Friederici AD, Gierhan SM. The language network. Curr Opin Neurobiol. (2013) 23:250–4. doi: 10.1016/j.conb.2012.10.002
29. Poeppel D. The neuroanatomic and neurophysiological infrastructure for speech and language. Curr Opin Neurobiol. (2014) 28:142–9. doi: 10.1016/j.conb.2014.07.005
30. Racy A, Jannotta FS, Lehner LH. Aphasia resulting from occlusion of the left anterior cerebral artery. Report of a case with an old infarct in the left Rolandic region. Arch Neurol. (1979) 36:221–4. doi: 10.1001/archneur.1979.00500400075012
31. Chu R, Meltzer JA, Bitan T. Interhemispheric interactions during sentence comprehension in patients with aphasia. Cortex. (2018) 109:74–91. doi: 10.1016/j.cortex.2018.08.022
32. Komiya K, Sakai Y, Horikoshi T, Naganuma H. Recovery process and prognosis of aphasic patients with left putaminal hemorrhage: relationship between hematoma type and language modalities. J Stroke Cerebrovasc Dis. (2013) 22:132–42. doi: 10.1016/j.jstrokecerebrovasdis.2011.07.007
33. Friederici AD, Ruschemeyer SA, Hahne A, Fiebach CJ. The role of left inferior frontal and superior temporal cortex in sentence comprehension: localizing syntactic and semantic processes. Cereb Cortex. (2003) 13:170–7. doi: 10.1093/cercor/13.2.170
34. Alyahya RSW, Halai AD, Conroy P, Lambon Ralph MA. The behavioural patterns and neural correlates of concrete and abstract verb processing in aphasia: A novel verb semantic battery. Neuroimage Clin. (2018) 17:811–25. doi: 10.1016/j.nicl.2017.12.009
35. Tsai PY, Wang CP, Ko JS, Chung YM, Chang YW, Wang JX. The persistent and broadly modulating effect of inhibitory rTMS in nonfluent aphasic patients: a sham-controlled, double-blind study. Neurorehabil Neural Repair. (2014) 28:779–87. doi: 10.1177/1545968314522710
36. Kapoor A. Repetitive transcranial magnetic stimulation therapy for post-stroke non-fluent aphasia: a critical review. Top Stroke Rehabil. (2017) 24:547–53. doi: 10.1080/10749357.2017.1331417
37. Bonner MF, Peelle JE, Cook PA, Grossman M. Heteromodal conceptual processing in the angular gyrus. Neuroimage. (2013) 71:175–86. doi: 10.1016/j.neuroimage.2013.01.006
38. Golestani N, Hervais-Adelman A, Obleser J, Scott SK. Semantic versus perceptual interactions in neural processing of speech-in-noise. Neuroimage. (2013) 79:52–61. doi: 10.1016/j.neuroimage.2013.04.049
39. Hartwigsen G, Golombek T, Obleser J. Repetitive transcranial magnetic stimulation over left angular gyrus modulates the predictability gain in degraded speech comprehension. Cortex. (2015) 68:100–10. doi: 10.1016/j.cortex.2014.08.027
40. Faria AV, Crinion J, Tsapkini K, Newhart M, Davis C, Cooley S, et al. Patterns of dysgraphia in primary progressive aphasia compared to post-stroke aphasia. Behav Neurol. (2013) 26:21–34. doi: 10.1155/2013/561692
41. Skipper-Kallal LM, Lacey EH, Xing S, Turkeltaub PE. Functional activation independently contributes to naming ability and relates to lesion site in post-stroke aphasia. Hum Brain Mapp. (2017) 38:2051–66. doi: 10.1002/hbm.23504
42. Zhang J, Wei X, Xie S, Zhou Z, Shang D, Ji R, et al. Multifunctional roles of the ventral stream in language models: advanced segmental quantification in post-stroke aphasic patients. Front Neurol. (2018) 9:89. doi: 10.3389/fneur.2018.00089
Keywords: stroke, aphasia, K-WAB, prognosis, outcome, brain imaging, VLSM
Citation: Sul B, Lee KB, Hong BY, Kim JS, Kim J, Hwang WS and Lim SH (2019) Association of Lesion Location With Long-Term Recovery in Post-stroke Aphasia and Language Deficits. Front. Neurol. 10:776. doi: 10.3389/fneur.2019.00776
Received: 17 April 2019; Accepted: 03 July 2019;
Published: 24 July 2019.
Edited by:
Lisa Tabor Connor, Washington University in St. Louis, United StatesReviewed by:
Alessandro Giustini, Consultant, Arezzo, ItalyCopyright © 2019 Sul, Lee, Hong, Kim, Kim, Hwang and Lim. This is an open-access article distributed under the terms of the Creative Commons Attribution License (CC BY). The use, distribution or reproduction in other forums is permitted, provided the original author(s) and the copyright owner(s) are credited and that the original publication in this journal is cited, in accordance with accepted academic practice. No use, distribution or reproduction is permitted which does not comply with these terms.
*Correspondence: Seong Hoon Lim, c2Vvbmdob29uQGNhdGhvbGljLmFjLmty
†These authors have contributed equally to this work
Disclaimer: All claims expressed in this article are solely those of the authors and do not necessarily represent those of their affiliated organizations, or those of the publisher, the editors and the reviewers. Any product that may be evaluated in this article or claim that may be made by its manufacturer is not guaranteed or endorsed by the publisher.
Research integrity at Frontiers
Learn more about the work of our research integrity team to safeguard the quality of each article we publish.