- Department of Neurology, University Medical Center Hamburg-Eppendorf (UKE), Hamburg, Germany
Primary angiitis of the central nervous system (PACNS) is an inflammatory brain disease affecting the medium and small vessels of the CNS. Although recent data of patients with PACNS have advanced the understanding of the disease, the diagnosis remains challenging. Clinical presentation of PACNS is broad and unspecific and the majority of the diagnostic approaches are hallmarked by a low specificity. Thus, PACNS is commonly misdiagnosed. In addition, due to its potential aggressive course which may be altered by an adequate immunosuppressive treatment, delineation from other vasculopathies and PACNS mimics is crucial. New diagnostic tools and biomarkers which increase specificity and facilitate the diagnosis for patients with suspected PACNS are highly desirable. This short review summarizes the current procedures within the diagnostic process and aims to illustrate its difficulties and challenges. Furthermore, it highlights emerging biomarkers in the cerebrospinal fluid and peripheral venous blood as well as novel potential imaging tools that may corroborate the diagnosis. With new imaging techniques and a panel of biomarkers the certainty of the diagnosis may be increased and diagnostic processes more accelerated in the future.
Introduction
Primary angiitis of the central nervous system (PACNS) is a poorly understood disease which is restricted to the small- and medium-sized vessels of the brain and spinal cord. It is a rare disease with an estimated incidence of 2.4 cases per 100,000 person-years and an equal frequency in women and men (1). All age groups may be affected, whereas 50% are 37–59 years of age. Of note, PACNS is also a rare cause of stroke and remains an important differential diagnosis in younger (<45 years) adults (2.2%) (2, 3). Diagnosis is extremely hampered by the paucity of specific clinical symptoms and by the low specificity of the diagnostic approaches. Thus, establishing a reliable diagnosis remains challenging and PACNS is often misdiagnosed. In addition, two different subtypes of the disease have been reported, the small (SVV) and medium vessel variant (MVV), with different diagnostic phenotypes which increases the diagnostic challenge. While the MVV shows remarkable vascular irregularities in angiography procedures, current angiography techniques do not have a sufficient resolution to detect vessel abnormalities if the disease is limited to a small-vessel involvement. Moreover, since aggressive immunosuppressive therapy is mandatory to avert the often fatal course of the disease, exclusion from other etiologies is crucial. The aim of this short review is to give an overview of the current diagnostic procedures in PACNS and emphasize its difficulties. It further highlights new potential biomarkers in the cerebrospinal fluid (CSF) and peripheral venous blood as well as imaging markers that may increase the diagnostic accuracy.
Current Diagnostic Approaches
The clinical presentation of PACNS is broad and unspecific. Thus, the average time to diagnosis may be several months (4). The symptoms vary from cognitive dysfunction, subacute, and progressive headache, seizures to focal neurological symptoms (5). Amongst others, the differential diagnosis includes e.g., hemorrhagic or ischemic stroke, neoplasia, encephalitis, dementia, migraine, multiple sclerosis. One of the most important differential diagnosis is the reversible cerebral vasoconstriction syndrome (RCVS) which bears the closest resemblance to PACNS with similar abnormalities in angiography. Recent studies provided new insights in diagnosing and distinguishing RCVS from PACNS by taking into account the patients' variables on admission (clinical symptoms, initial brain imaging etc.) (6, 7). However, diagnosis and distinction from this non-inflammatory vasculopathy is still difficult. In 1988, Calabrese and Mallek (8) proposed diagnostic criteria for PACNS which include a history or presence of a neurological deficit unexplained by any other cause after a thorough examination, evidence of vasculitis either by histopathology or angiography with characteristic changes of vasculitis and exclusion of a systemic vasculitis or any other condition to which the angiographic changes can be secondary. Birnbaum et al. (9) modified these criteria and differentiated between a “probable” and “definite” diagnosis. While definite PACNS is defined by histopathological evidence, all other cases are categorized as suspected PACNS. These revised criteria aimed at preventing inadequate and uncontrolled application of immunosuppressive treatment. To date, the diagnostic workup encompasses extensive laboratory examinations including an immunological screening and CSF analysis, MRI, angiography, and histopathology. Most of the tests are done to rule out differential diagnosis, such as systemic vasculitis (autoimmune or infectious), non-inflammatory vasculopathies (e.g., RCVS), or malignant disorders (e.g., lymphoproliferative diseases). Most of the patients (80–90%) show a pleocytosis (about 10–20 cells/ml) and/or increased protein levels (about 120 mg/ml) (4). However, these abnormalities in CSF are unspecific and, of note, an initial normal CSF does not exclude the diagnosis (10). In addition, there is currently no evidence of differences in CSF findings between the two disease variants. For brain imaging in PACNS, MRI remains the modality of choice. Fluid attenuated inversion recovery (FLAIR)—imaging, T1- and T2-weighted imaging, diffusion-weighted imaging (DWI), susceptibility-weighted imaging (SWI), time–of-flight MR angiography (TOF) and contrast medium-enhanced imaging are required sequences. Most of the patients with PACNS (90–100%) show abnormal MRI findings (9, 11). Typical changes include diffuse white and gray matter changes, contrast medium enhanced parenchymal lesions, leptomeningeal enhancement (12), parenchymal hemorrhages as well as microbleeds (13, 14). Tumor-like mass lesions have also been reported (15). Even a generalized atrophy in the MRI scan can be a sign of PACNS (16). Furthermore, MR angiography (MRA) can be applied. While in patients with SVV brain vessels in MRA are commonly unremarkable, vessel abnormalities are likely to be detected in MVV. Typical irregularities comprise peripheral alternating stenosis/occlusions and dilatation as well as vessel wall thickening in different vascular territories. However, none of these findings are specific, but a normal MRI scan in combination with a normal CSF result rule out the diagnosis of PACNS (17, 18). The current gold standard for vessel imaging in PACNS is digital subtraction angiography (DSA). Its reported overall sensitivity varies between 40 and 90% (4, 19, 20). This high range is most likely explained by the different subtypes of the disease (21, 22). Whereas, DSA may reveal remarkable vascular irregularities in patients with a predominant MVV, it shows unconspicious vessels in SVV. Currently, DSA has only a resolution for vessels >500 μm in diameter (23), thus, the SVV is typically “angiography-negative.” In addition, despite having a higher sensitivity in distal vessels and the posterior circulation (24) compared to MRA, the DSA provides information on changes in vessel contours only and not on the underlying pathological process (25). In particular, distinguishing non-inflammatory vasculopathies (e.g., RCVS or artherosclerosis) remains a challenge. Hence, DSA also has a low specificity. To date, brain biopsy is the only eligible technique to establish a definite diagnosis of PACNS. An optimal biopsy should contain samples from the dura, leptomeninges, cortex and white matter (26). However, the sensitivity of brain biopsy is also low (54–83%) (20, 21, 27). This broad range may be explained by the different case series including SVV and MVV. Notably, about one fourth of all autopsy-documented cases of PACNS were false-negative (28). In particular, in patients with MVV histopathology is frequently false-negative, probably due to abnormalities of medium-sized vessels that are rather detectable by angiography but not covered by brain biopsy (22). The rate of positive results can be increased by targeting MRI positive lesions (18). Three histopathological patterns are known in PACNS so far: granulomatous (58%), lymphocytic (28%) and necrotizing (14%), but they often overlap. The amyloid-ß angiitis (ABRA) with ß-amyloid depositions in the brain vessel walls is considered to be a subset of the granulomatous pattern. To date, no clear difference in clinical symptoms, aggressiveness of the disease, or treatment response between these patterns has been reported.
Due to the variable clinical presentation of PACNS, the lack of specific diagnostics and its differences between SVV and MVV (diverse DSA/MRA results, diverse biopsy results if derived from the right frontal lobe) a definite diagnosis is still a challenge. Additional diagnostic approaches are required to increase certainty. Thus, in the following we focus on potential, novel diagnostic imaging techniques and biomarkers in the CSF and blood to diagnose PACNS.
Emerging New Diagnostic Biomarkers
Interleukin-17 in the Cerebrospinal Fluid
A promising marker for PACNS in the CSF is interleukin-17 (IL-17). IL-17 is a pro-inflammatory cytokine and a potent mediator in cellular immunity. It plays a pivotal role in the pathogenesis of systemic vasculitis, in particular, antineutrophil cytoplasmatic antibodies (ANCA)-associated vasculitis (AAV), or giant cell arteritis (29). Previously, we reported that IL-17 produced by CD4+-T-cells in the CSF was elevated in patients with PACNS (sensitivity 73%, specificity 100%) (Figure 1A) (30). Elevated IL-17 levels were persistent in patients with active PACNS and patients in remission, thus indicating IL-17 being a more specific biomarker of cerebral vasculitis than the cell count or protein elevation in the CSF and being crucial also in the pathogenesis of PACNS. These findings merit the attempt of making IL-17 a target for novel therapeutic interventions. Interestingly, humanized anti-IL-17 antibodies as a treatment approach were already shown to induce clinically relevant responses in patients with psoriasis and rheumatoid arthritis (31). However, the promising results of IL-17 analyses in PACNS need to be verified in larger patient cohorts.
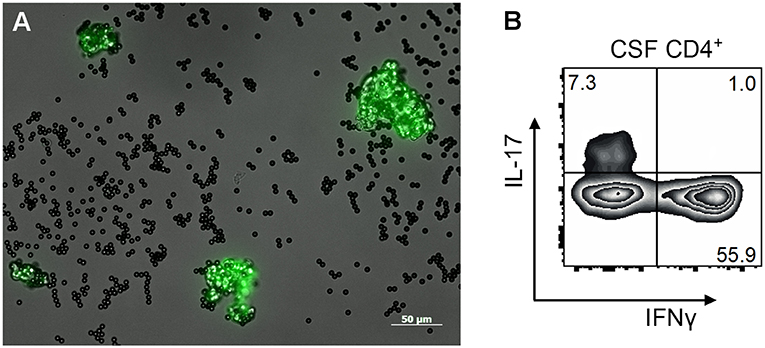
Figure 1. (A) Morphology of circulating endothelial cells (CEC, green) with Dynabeads™ (black) attached after immunomagnetic isolation from peripheral blood of a patient with PACNS displayed under a fluorescence microscope. CECs were isolated by CD 146-coated Dynabeads™ and with a fluorescence-coated (FITC) lectin (Ulex europaeus agglutinin 1, UEA-1) in an endothelial cell specific double-staining method. Afterwards CECs were enumerated in a counting chamber using a fluorescence microscope. (B) FACS plot of expanded T helper cells from a patient with biopsy proven PACNS. CSF cells were expanded in the presence of feeder cells, IL-2 und PHA for 2 weeks and an intracellular cytokine staining for IL-17 and IFNγ was performed.
Amyloid-beta A4 Protein (APP) in the Cerebrospinal Fluid
Mass-spectrometry-based techniques allow the assessment of a significant fraction of the proteome in biofluids without a pre-selection of target proteins. Ruland et al. reported a significantly lower abundance of proteins in the CSF of patients with PACNS (32). In particular, reduced APP concentrations were detected, a protein indicative for nervous system damage or pathology. Based on these findings the authors speculate that this protein may serve as a surrogate marker of brain injury in cerebral vasculitis. Further studies will have to validate these findings.
Circulating Endothelial Cells in the Peripheral Venous Blood
Circulating endothelial cells (CECs) are known as markers of endothelial damage (33, 34). Inflammatory or non-inflammatory endothelial damage or mechanical injury can lead to the detachment of endothelial cells from the vessel wall. Thereafter, CECs can easily be detected in the blood by immunomagnetic isolation (Figure 1B) or flow cytometry (35). Preliminary studies of AAV showed increased CEC levels in patients with an active disease (36) whereas the number of CECs decreased under successful immunosuppressive treatment. Likewise, lower numbers of CECs were detected in patients with non-inflammatory vascular diseases, such as stroke and myocardial infarction (34, 37, 38). Of note, similar results were observed in patients with PACNS. We demonstrated that CECs were significantly elevated in patients with active PACNS while being decreased under successful immunosuppressive medication, in healthy controls and patients with stroke and cerebrovascular risk factors (39). Hence, CECs may potentially contribute to the diagnosis in biopsy negative cases and monitor the success of immunosuppressive treatment. However, although being a promising biomarker, studies with larger patient numbers are required to verify these results.
High-Resolution Magnetic Resonance Vessel Wall Imaging (HR-MRI)
Commonly used imaging modalities fail to distinguish between inflammatory and non-inflammatory vasculopathies. Preliminary studies demonstrated the possibility of outlining the vessel wall by contrast enhancement (40–42). In enhanced MR imaging of the vessel walls (“dark blood imaging”) the signal of the blood is suppressed and discrimination of the vessel wall from the lumen is increased (Figure 2). This HR-MRI can be performed as T1-weighted, T2-weighted or proton density-weighted sequences (43). This technique has already been used to distinguish PACNS from other vasculopathies (40, 42). In more detail, in PACNS a predominantly smooth, concentric, and long-segment wall thickening with strong enhancement and a perivascular edema were observed (44). Arteriosclerotic plaques exhibit a more eccentric, irregular, and short-segment wall thickening without perivascular edema and only mild enhancement depending on composition and activity of the plaque (25, 42). However, the distinction might still be difficult and this MRI sequence might rather help to differentiate PACNS from vasculopathies of young adults such as RCVS (41, 44). Notably, HR-MRI is merely capable of detecting the MVV with its current capacity of vessel resolution. However, this imaging technique is quickly developing and might soon lead to an improved discrimination between the MVV of PACNS and other intracranial vasculopathies (45). Of note, with higher resolutions it will be able to detect changes even in small vessels. Given that treatment can also affect the imaging findings, e.g., less enhancement under immunosuppressive or antiviral therapy (43, 46), it might also have the potential to monitor disease activity, which would make it extremely valuable for clinical trials (43, 47).
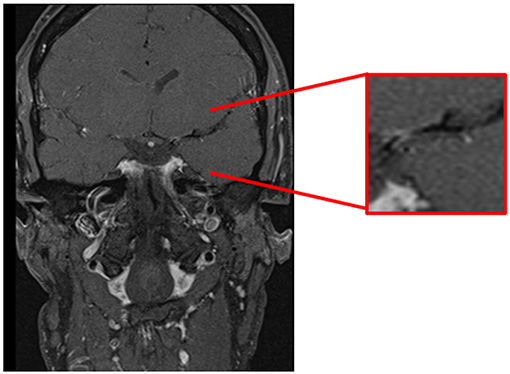
Figure 2. Cranial MRI, coronal T1-weighted-(dark-blood) sequence demonstrating contrast enhancement of the vessel wall in the first segment of the left middle cerebral artery.
Potential New Diagnostic Biomarkers
Endothelial Progenitor Cells in the Peripheral Venous Blood
Another potential biomarker in PACNS are the endothelial progenitor cells (EPCs). In contrast to CECs, EPCs are considered to play a pivotal role in vascular regeneration and endothelial renewal (34). They are bone marrow-derived cells and originate from hematopoietic progenitor cells. EPCs are believed to be mobilized by various factors (e.g., vascular endothelial growth factor, VEGF), migrate to the site of endothelial injury, integrate in the endothelial cell layer and differentiate to mature endothelial cells (48). Interestingly, an inverse relationship of CECs and EPCs in patients with systemic vasculitis was previously reported (49). CEC values were highly elevated immediately after endothelial injury and decreased over time. In contrast, the EPC counts were low at time of endothelial injury and increased in the equal time course. Notably, similar results were observed in patients with Kawasaki disease, acute coronary syndrome and ischemic stroke (50–52). Hence, EPCs are presumed to be a marker for vascular or endothelial repair in inflammatory and non-inflammatory vasculopathies. However, EPCs have heretofore not been investigated, but these findings warrant the analysis of EPCs in PACNS.
Von Willebrand Factor Antigen in the Peripheral Venous Blood
The von Willebrand factor antigen (vWF-Ag) is a plasma protein which is mainly synthesized from endothelial cells. Its function includes platelet aggregation and adhesion. If endothelium is damaged, e.g., by inflammation, increased levels of vWF-Ag may then be detected in blood. In fact, vWF-Ag was previously suggested as a potential biomarker in patients with systemic vasculitis. In patients suffering from Behçet disease and AAV, vWF-Ag levels were increased compared to controls (53–55). However, these elevated levels were observed in an active stage of disease and remained increased under remission. In contrast, in patients with Granulomatosis with Polyangiitis and Kawasaki disease vWF-Ag levels correlated well with disease activity (56, 57). Likewise, a recent study demonstrated that vWF-Ag may be a marker for disease activity in childhood PACNS (58). Elevated levels were detected in active disease and normalized under successful treatment. These results justify further analyses in adult PACNS.
High Resolution Digital Subtraction Angiography
High resolution digital subtraction angiography including a 3D rotation technique provides a visualization of smaller vessels than with commonly used angiography techniques. A recent study demonstrated that this tool can display small branches (e.g., perforators) of the basilar artery (59). Fukuda et al. (60) described a fusion technique using two three-dimensional DSA images with precise information of the angio-architecture of arterial-venous malformations. The exact structure and location of the fistula, feeders, and drainers with high spatial resolution were obtained. Another novel technique to visualize small vessels beyond the spatial resolution of commonly used imaging modalities is the time-resolved 3D rotational angiography (4D DSA) (61). In particular, for proximal stenosis of the middle cerebral artery local collateral networks can be displayed and microangio-architecture in dural arteriovenous fistulas (dAVF) or arteriovenous malformations (AVM) can be visualized (62). Notably, 4D DSA might reduce the radiation and contrast agent dose and can be less time consuming compared to commonly used methods. In summary, novel imaging techniques in DSA could potentially provide more detailed information on smaller cerebral vessels due to higher spatial resolution. These imaging sequences were applied to studies of AVMs, dAVFs and aneurysms so far. They might also be applied to the SVV of PACNS to further increase the diagnostic specificity.
Positron Emission Tomography (PET)
Fluor-desoxy-glucose (FDG)-PET is a functional imaging technique in nuclear medicine. It uses FDG as the radiopharmaceutical and depicts metabolic activity in the body by visualization of glucose uptake in tissues. Hence, inflamed vessel walls can be uncovered. In fact, in patients with biopsy proven giant cell arteriitis, FDG-PET was successfully applied and showed a high sensitivity and specificity (63). In addition, Novikov et al. demonstrated that PET can be a valuable diagnostic tool in patients with medium vessel involvement (64) and, thus, may contribute to depict inflammation of the vessel walls in patients with inconclusive diagnostic results (65). Of note, FDG-PET was previously applied to follow up and monitor disease activity in vasculitis (66, 67). However, small-vessel involvement cannot be detected by this imaging modality (68).
Conclusion
This short review summarizes current diagnostic procedures applied to primary CNS vasculitis and aims at illustrating the difficulties and challenges in diagnosing PACNS. It further presents novel emerging and potential biomarkers which might facilitate the diagnostic process in the future. New biofluid and imaging markers may increase the certainty of the diagnosis. Biomarkers in the CSF (IL-17, APP) and peripheral venous blood (CEC, EPC, vWF-Ag) seem to be promising approaches, although potential differences in results between SVV and MVV remain to be determined. The ultimate goal would be to identify a panel of markers that might increase the diagnostic accuracy in PACNS in the future. In addition, novel potential imaging techniques, e.g., PET or different high resolution DSA-based techniques, can visualize smaller vessels or inflammatory vessel walls and, therefore, might support the diagnostic work. Data on these diagnostic tools are currently preliminary and, thus, need to be verified in larger patient cohorts or still need to be investigated in adult patients with PACNS. However, they might advance the diagnostic workflow in the future and aid to an accelerated diagnosis in patients lacking histological evidence, hence, in patients with suspected disease. They are promising diagnostic approaches that justify future attempts of investigation.
Author Contributions
MD-C: substantial contribution to the conception and design of the work; acquisition, analysis and interpretation of data for the work; drafting the work and revising it critically for important intellectual content. SS: interpretation of data for the work; revising it critically for important intellectual content. VH: analysis and interpretation of data for the work; revising it critically for important intellectual content. CG: analysis and interpretation of data for the work; drafting of the work and revising it critically for important intellectual content. GT: analysis and interpretation of data for the work; drafting of the work and revising it critically for important intellectual content. TM: conception and design of the work; analysis and interpretation of data for the work; drafting of the work and revising it critically for important intellectual content. All authors provide approval for publication of the content. All authors agree to be accountable for all aspects of the work in ensuring that questions related to the accuracy or integrity of any part of the work are appropriately investigated and resolved.
Conflict of Interest Statement
CG received fees as a consultant or lecture fees from Amgen, Bayer Vital, Bristol-Myers Squibb/Pfizer, Boehringer Ingelheim, Sanofi Aventis. GT received fees as a consultant or lecture fees from Acandis, Bayer Vital, Bristol-Myers Squibb/Pfizer, Boehringer Ingelheim, Daichii Sankyo, GlaxoSmithKline, and Stryker. TM received fees as a consultant or lecture fees from Boehringer Ingelheim, Novartis, Merck-Serono, Grifols, CSL-Behring and he received research fees from BMBF, DFG, EU, Werner-Otto-Stiftung, Schilling-Stiftung, Novartis, Merck-Serono.
The remaining authors declare that the research was conducted in the absence of any commercial or financial relationships that could be construed as a potential conflict of interest.
References
1. Salvarani C, Brown RD Jr., Hunder GG. Adult primary central nervous system vasculitis. Lancet. (2012) 380:767–77. doi: 10.1016/S0140-6736(12)60069-5
2. Marini C, Totaro R, De Santis F, Ciancarelli I, Baldassarre M, Carolei A. Stroke in young adults in the community-based L'Aquila registry: incidence and prognosis. Stroke. (2001) 32:52–6. doi: 10.1161/01.STR.32.1.52
3. Yesilot Barlas N, Putaala J, Waje-Andreassen U, Vassilopoulou S, Nardi K, Odier C, et al. Etiology of first-ever ischaemic stroke in European young adults: the 15 cities young stroke study. Eur J Neurol. (2013) 20:1431–9. doi: 10.1111/ene.12228
4. Salvarani C, Brown RDJr, Calamia KT, Christianson TJ, Weigand SD, Miller DV, et al. Primary central nervous system vasculitis: analysis of 101 patients. Ann Neurol. (2007) 62:442–51. doi: 10.1002/ana.21226
5. Lucke M, Hajj-Ali RA. Advances in primary angiitis of the central nervous system. Curr Cardiol Rep. (2014) 16:533. doi: 10.1007/s11886-014-0533-0
6. Singhal AB, Topcuoglu MA, Fok JW, Kursun O, Nogueira RG, Frosch MP, et al. Reversible cerebral vasoconstriction syndromes and primary angiitis of the central nervous system: clinical, imaging, and angiographic comparison. Ann Neurol. (2016) 79:882–94. doi: 10.1002/ana.24652
7. Rocha EA, Topcuoglu MA, Silva GS, Singhal AB. RCVS2 score and diagnostic approach for reversible cerebral vasoconstriction syndrome. Neurology. (2019) 92:e639–47. doi: 10.1212/WNL.0000000000006917
8. Calabrese LH, Mallek JA. Primary angiitis of the central nervous system. Report of 8 new cases, review of the literature, and proposal for diagnostic criteria. Medicine. (1988) 67:20–39. doi: 10.1097/00005792-198801000-00002
9. Birnbaum J, Hellmann DB. Primary angiitis of the central nervous system. Arch Neurol. (2009) 66:704–9. doi: 10.1001/archneurol.2009.76
10. Schmidley JW. 10 questions on central nervous system vasculitis. Neurologist. (2008) 14:138–9. doi: 10.1097/NRL.0b013e31815bdc2b
11. Salvarani C, Brown RD Jr., Christianson T, Miller DV, Giannini C, Huston J III, et al. An update of the Mayo Clinic cohort of patients with adult primary central nervous system vasculitis: description of 163 patients. Medicine. (2015) 94:e738. doi: 10.1097/MD.0000000000000738
12. Zuccoli G, Pipitone N, Haldipur A, Brown RD Jr., Hunder G, Salvarani C. Imaging findings in primary central nervous system vasculitis. Clin Exp Rheumatol. (2011) 29(1 Suppl 64):S104–9. Available online at: https://www.clinexprheumatol.org/abstract.asp?a=4879
13. Ay H, Sahin G, Saatci I, Soylemezoglu F, Saribas O. Primary angiitis of the central nervous system and silent cortical hemorrhages. AJNR Am J Neuroradiol. (2002) 23:1561–3. Available online at: http://www.ajnr.org/content/23/9/1561.long
14. de Boysson H, Zuber M, Naggara O, Neau JP, Gray F, Bousser MG, et al. Primary angiitis of the central nervous system: description of the first fifty-two adults enrolled in the French cohort of patients with primary vasculitis of the central nervous system. Arthrit Rheumatol. (2014) 66:1315–26. doi: 10.1002/art.38340
15. Kumar RS, Singh A, Rathore C, Kesavadas C. Primary angiitis of central nervous system: tumor-like lesion. Neurol India. (2010) 58:147–9. doi: 10.4103/0028-3886.60417
16. Bonstrup M, Ott K, Glatzel M, Magnus T. Frontal lobe dementia syndrome as a first manifestation of primary angiitis of the central nervous system (PACNS). Clin Neurol Neurosurg. (2016) 141:92–4. doi: 10.1016/j.clineuro.2015.12.016
17. Stone JH, Pomper MG, Roubenoff R, Miller TJ, Hellmann DB. Sensitivities of noninvasive tests for central nervous system vasculitis: a comparison of lumbar puncture, computed tomography, and magnetic resonance imaging. J Rheumatol. (1994) 21:1277–82.
18. Schuster S, Magnus T. [Primary central nervous system vasculitis]. Z Rheumatol. (2015) 74:862. doi: 10.1007/s00393-015-0007-9
19. Harris KG, Tran DD, Sickels WJ, Cornell SH, Yuh WT. Diagnosing intracranial vasculitis: the roles of MR and angiography. AJNR Am J Neuroradiol. (1994) 15:317–30.
20. Marsh EB, Zeiler SR, Levy M, Llinas RH, Urrutia VC. Diagnosing CNS vasculitis: the case against empiric treatment. Neurologist. (2012) 18:233–8. doi: 10.1097/NRL.0b013e31825c6d23
21. Calabrese LH, Furlan AJ, Gragg LA, Ropos TJ. Primary angiitis of the central nervous system: diagnostic criteria and clinical approach. Cleve Clin J Med. (1992) 59:293–306. doi: 10.3949/ccjm.59.3.293
22. Schuster S, Bachmann H, Thom V, Kaufmann-Buehler AK, Matschke J, Siemonsen S, et al. Subtypes of primary angiitis of the CNS identified by MRI patterns reflect the size of affected vessels. J Neurol Neurosurg Psychiatr. (2017) 88:749–55. doi: 10.1136/jnnp-2017-315691
23. Bhattacharyya S, Berkowitz AL. Primary angiitis of the central nervous system: avoiding misdiagnosis and missed diagnosis of a rare disease. Pract Neurol. (2016) 16:195–200. doi: 10.1136/practneurol-2015-001332
24. Eleftheriou D, Cox T, Saunders D, Klein NJ, Brogan PA, Ganesan V. Investigation of childhood central nervous system vasculitis: magnetic resonance angiography versus catheter cerebral angiography. Dev Med Child Neurol. (2010) 52:863–7. doi: 10.1111/j.1469-8749.2009.03591.x
25. Dutra LA, de Souza AW, Grinberg-Dias G, Barsottini OG, Appenzeller S. Central nervous system vasculitis in adults: An update. Autoimmun Rev. (2017) 16:123–31. doi: 10.1016/j.autrev.2016.12.001
26. Parisi JE, Moore PM. The role of biopsy in vasculitis of the central nervous system. Semin Neurol. (1994) 14:341–8. doi: 10.1055/s-2008-1041093
27. Miller DV, Salvarani C, Hunder GG, Brown RD, Parisi JE, Christianson TJ, et al. Biopsy findings in primary angiitis of the central nervous system. Am J Surg Pathol. (2009) 33:35–43. doi: 10.1097/PAS.0b013e318181e097
28. Cupps TR, Moore PM, Fauci AS. Isolated angiitis of the central nervous system. Prospective diagnostic and therapeutic experience. Am J Med. (1983) 74:97–105. doi: 10.1016/0002-9343(83)91125-7
29. Kallenberg CG. Pathophysiology of ANCA-associated small vessel vasculitis. Curr Rheumatol Rep. (2010) 12:399–405. doi: 10.1007/s11926-010-0138-6
30. Thom V, Schmid S, Gelderblom M, Hackbusch R, Kolster M, Schuster S, et al. IL-17 production by CSF lymphocytes as a biomarker for cerebral vasculitis. Neurol Neuroimmunol Neuroinflamm. (2016) 3:e214. doi: 10.1212/NXI.0000000000000214
31. Hueber W, Patel DD, Dryja T, Wright AM, Koroleva I, Bruin G, et al. Effects of AIN457, a fully human antibody to interleukin-17A, on psoriasis, rheumatoid arthritis, and uveitis. Sci Transl Med. (2010) 2:52ra72. doi: 10.1126/scitranslmed.3001107
32. Ruland T, Wolbert J, Gottschalk MG, Konig S, Schulte-Mecklenbeck A, Minnerup J, et al. Cerebrospinal fluid concentrations of neuronal proteins are reduced in primary angiitis of the central nervous system. Front Neurol. (2018) 9:407. doi: 10.3389/fneur.2018.00407
33. Erdbruegger U, Haubitz M, Woywodt A. Circulating endothelial cells: a novel marker of endothelial damage. Clin Chim Acta. (2006) 373:17–26. doi: 10.1016/j.cca.2006.05.016
34. Schmidt DE, Manca M, Hoefer IE. Circulating endothelial cells in coronary artery disease and acute coronary syndrome. Trends Cardiovasc Med. (2015) 25:578–87. doi: 10.1016/j.tcm.2015.01.013
35. Erdbruegger U, Woywodt A, Kirsch T, Haller H, Haubitz M. Circulating endothelial cells as a prognostic marker in thrombotic microangiopathy. Am J Kidney Dis. (2006) 48:564–70. doi: 10.1053/j.ajkd.2006.06.013
36. Woywodt A, Streiber F, de Groot K, Regelsberger H, Haller H, Haubitz M. Circulating endothelial cells as markers for ANCA-associated small-vessel vasculitis. Lancet. (2003) 361:206–10. doi: 10.1016/S0140-6736(03)12269-6
37. Haubitz M, Woywodt A. Circulating endothelial cells and vasculitis. Intern Med. (2004) 43:660–7. doi: 10.2169/internalmedicine.43.660
38. Woywodt A, Gerdes S, Ahl B, Erdbruegger U, Haubitz M, Weissenborn K. Circulating endothelial cells and stroke: influence of stroke subtypes and changes during the course of disease. J Stroke Cerebrovasc Dis. (2012) 21:452–8. doi: 10.1016/j.jstrokecerebrovasdis.2010.11.003
39. Deb M, Gerdes S, Heeren M, Lambrecht J, Worthmann H, Goldbecker A, et al. Circulating endothelial cells as potential diagnostic biomarkers in primary central nervous system vasculitis. J Neurol Neurosurg Psychiatr. (2013) 84:732–4. doi: 10.1136/jnnp-2012-303335
40. Kuker W, Gaertner S, Nagele T, Dopfer C, Schoning M, Fiehler J, et al. Vessel wall contrast enhancement: a diagnostic sign of cerebral vasculitis. Cerebrovasc Dis. (2008) 26:23–9. doi: 10.1159/000135649
41. Swartz RH, Bhuta SS, Farb RI, Agid R, Willinsky RA, Terbrugge KG, et al. Intracranial arterial wall imaging using high-resolution 3-tesla contrast-enhanced MRI. Neurology. (2009) 72:627–34. doi: 10.1212/01.wnl.0000342470.69739.b3
42. Saam T, Habs M, Cyran CC, Grimm J, Pfefferkorn T, Schuller U, et al. [New aspects of MRI for diagnostics of large vessel vasculitis and primary angiitis of the central nervous system]. Radiologe. (2010) 50:861–71. doi: 10.1007/s00117-010-2004-y
43. Lehman VT, Brinjikji W, Kallmes DF, Huston JR, Lanzino G, Rabinstein AA, et al. Clinical interpretation of high-resolution vessel wall MRI of intracranial arterial diseases. Br J Radiol. (2016) 89:20160496. doi: 10.1259/bjr.20160496
44. Obusez EC, Hui F, Hajj-Ali RA, Cerejo R, Calabrese LH, Hammad T, et al. High-resolution MRI vessel wall imaging: spatial and temporal patterns of reversible cerebral vasoconstriction syndrome and central nervous system vasculitis. AJNR Am J Neuroradiol. (2014) 35:1527–32. doi: 10.3174/ajnr.A3909
45. Mossa-Basha M, Hwang WD, De Havenon A, Hippe D, Balu N, Becker KJ, et al. Multicontrast high-resolution vessel wall magnetic resonance imaging and its value in differentiating intracranial vasculopathic processes. Stroke. (2015) 46:1567–73. doi: 10.1161/STROKEAHA.115.009037
46. Noh HJ, Choi JW, Kim JP, Moon GJ, Bang OY. Role of high-resolution magnetic resonance imaging in the diagnosis of primary angiitis of the central nervous system. J Clin Neurol. (2014) 10:267–71. doi: 10.3988/jcn.2014.10.3.267
47. Kissin EY, Merkel PA. Diagnostic imaging in Takayasu arteritis. Curr Opin Rheumatol. (2004) 16:31–7. doi: 10.1097/00002281-200401000-00007
48. Liao S, Luo C, Cao B, Hu H, Wang S, Yue H, et al. Endothelial progenitor cells for ischemic stroke: update on basic research and application. Stem Cells Int. (2017) 2017:2193432. doi: 10.1155/2017/2193432
49. de Groot K, Goldberg C, Bahlmann FH, Woywodt A, Haller H, Fliser D, et al. Vascular endothelial damage and repair in antineutrophil cytoplasmic antibody-associated vasculitis. Arthr Rheum. (2007) 56:3847–53. doi: 10.1002/art.23070
50. Nakatani K, Takeshita S, Tsujimoto H, Kawamura Y, Tokutomi T, Sekine I. Circulating endothelial cells in Kawasaki disease. Clin Exp Immunol. (2003) 131:536–40. doi: 10.1046/j.1365-2249.2003.02091.x
51. Massa M, Rosti V, Ferrario M, Campanelli R, Ramajoli I, Rosso R, et al. Increased circulating hematopoietic and endothelial progenitor cells in the early phase of acute myocardial infarction. Blood. (2005) 105:199–206. doi: 10.1182/blood-2004-05-1831
52. Bogoslovsky T, Chaudhry A, Latour L, Maric D, Luby M, Spatz M, et al. Endothelial progenitor cells correlate with lesion volume and growth in acute stroke. Neurology. (2010) 75:2059–62. doi: 10.1212/WNL.0b013e318200d741
53. Direskeneli H, Keser G, D'Cruz D, Khamashta MA, Akoglu T, Yazici H, et al. Anti-endothelial cell antibodies, endothelial proliferation and von Willebrand factor antigen in Behcet's disease. Clin Rheumatol. (1995) 14:55–61. doi: 10.1007/BF02208085
54. Beyan E, Sadikoglu B, Ertugrul E, Beyan C. Von Willebrand factor antigen levels in Behcet disease. Am J Hematol. (2005) 79:70–2. doi: 10.1002/ajh.20317
55. Salmela A, Ekstrand A, Joutsi-Korhonen L, Raisanen-Sokolowski A, Lassila R. Activation of endothelium, coagulation and fibrinolysis is enhanced and associates with renal anti-neutrophil cytoplasmic antibody-associated vasculitis. Nephrol Dial Transplant. (2015) 30(Suppl 1):i53–9. doi: 10.1093/ndt/gfu379
56. D'Cruz D, Direskeneli H, Khamashta M, Hughes GR. Lymphocyte activation markers and von Willebrand factor antigen in Wegener's granulomatosis: potential markers for disease activity. J Rheumatol. (1999) 26:103–9.
57. Falcini F, Generini S, Pignone A, Leoncini G, Cimaz R, Partsch G, et al. Are angiotensin converting enzyme and von Willebrand factor circulating levels useful surrogate parameters to monitor disease activity in Kawasaki disease? Endothelium. (1999) 6:209–15. doi: 10.3109/10623329909053411
58. Cellucci T, Tyrrell PN, Pullenayegum E, Benseler SM. von Willebrand factor antigen–a possible biomarker of disease activity in childhood central nervous system vasculitis? Rheumatology. (2012) 51:1838–45. doi: 10.1093/rheumatology/kes156
59. Lescher S, Samaan T, Berkefeld J. Evaluation of the pontine perforators of the basilar artery using digital subtraction angiography in high resolution and 3D rotation technique. AJNR Am J Neuroradiol. (2014) 35:1942–7. doi: 10.3174/ajnr.A3981
60. Fukuda K, Higashi T, Okawa M, Iwaasa M, Abe H, Inoue T. Fusion technique using three-dimensional digital subtraction angiography in the evaluation of complex cerebral and spinal vascular malformations. World Neurosurg. (2016) 85:353–8. doi: 10.1016/j.wneu.2015.08.075
61. Kammerer S, Mueller-Eschner M, Berkefeld J, Tritt S. Time-resolved 3D rotational angiography (4D DSA) of the lenticulostriate arteries: display of normal anatomic variants and collaterals in cases with chronic obstruction of the MCA. Clin Neuroradiol. (2017) 27:451–7. doi: 10.1007/s00062-017-0578-8
62. Lescher S, Gehrisch S, Klein S, Berkefeld J. Time-resolved 3D rotational angiography: display of detailed neurovascular anatomy in patients with intracranial vascular malformations. J Neurointerv Surg. (2017) 9:887–94. doi: 10.1136/neurintsurg-2016-012462
63. Prieto-Gonzalez S, Depetris M, Garcia-Martinez A, Espigol-Frigole G, Tavera-Bahillo I, Corbera-Bellata M, et al. Positron emission tomography assessment of large vessel inflammation in patients with newly diagnosed, biopsy-proven giant cell arteritis: a prospective, case-control study. Ann Rheum Dis. (2014) 73:1388–92. doi: 10.1136/annrheumdis-2013-204572
64. Novikov PI, Meshkov AD, Moiseev SV. Positron emission tomography in giant cell arteritis: a new diagnostic tool? Ann Rheum Dis. (2014) 73:e70. doi: 10.1136/annrheumdis-2014-206227
65. Abdel Razek AA, Alvarez H, Bagg S, Refaat S, Castillo M. Imaging spectrum of CNS vasculitis. Radiographics. (2014) 34:873–94. doi: 10.1148/rg.344135028
66. Berlit P. Diagnosis and treatment of cerebral vasculitis. Ther Adv Neurol Disord. (2010) 3:29–42. doi: 10.1177/1756285609347123
67. Pacheco Castellanos Mdel C, Minguez Vega M, Martinez Caballero A, Bernabeu Gonzalvez MP. Early diagnosis of large vessel vasculitis: usefulness of positron emission tomography with computed tomography. Reumatol Clin. (2013) 9:65–8. doi: 10.1016/j.reumae.2013.01.005
Keywords: PACNS, biomarker, vasculitis, cerebrospinal fluid, peripheral venous blood, imaging, circulating endothelial cells
Citation: Deb-Chatterji M, Schuster S, Haeussler V, Gerloff C, Thomalla G and Magnus T (2019) Primary Angiitis of the Central Nervous System: New Potential Imaging Techniques and Biomarkers in Blood and Cerebrospinal Fluid. Front. Neurol. 10:568. doi: 10.3389/fneur.2019.00568
Received: 25 February 2019; Accepted: 15 May 2019;
Published: 06 June 2019.
Edited by:
Thomas Skripuletz, Hanover Medical School, GermanyReviewed by:
Anastasia Bougea, National and Kapodistrian University of Athens, GreeceMehmet Akif Topçuoǧlu, Hacettepe University, Turkey
Copyright © 2019 Deb-Chatterji, Schuster, Haeussler, Gerloff, Thomalla and Magnus. This is an open-access article distributed under the terms of the Creative Commons Attribution License (CC BY). The use, distribution or reproduction in other forums is permitted, provided the original author(s) and the copyright owner(s) are credited and that the original publication in this journal is cited, in accordance with accepted academic practice. No use, distribution or reproduction is permitted which does not comply with these terms.
*Correspondence: Milani Deb-Chatterji, bS5kZWItY2hhdHRlcmppQHVrZS5kZQ==