- 1Department of Neurology, University Hospital of Tuebingen, Tuebingen, Germany
- 2Department of Neurology, University Hospital of Ulm, Ulm, Germany
- 3Department of Neurology, University Hospital of Freiburg, Freiburg, Germany
- 4German Center for Neurodegenerative Diseases (DZNE), Munich, Germany
- 5BioMedical Center (BMC), Ludwig-Maximilians-Universität München, Munich, Germany
- 6Barcelonaβeta Brain Research Center (BBRC), Pasqual Maragall Foundation, Barcelona, Spain
- 7Neuroimmunological Section, Department of Neurology, University Hospital of Rostock, Rostock, Germany
- 8Munich Cluster for Systems Neurology (SyNergy), Munich, Germany
- 9Department of Neurology, University Hospital of Muenster, Münster, Germany
- 10Specialty Hospital Dietenbronn, Schwendi, Germany
Background: In progressive multiple sclerosis (MS), glial activation is thought to be a relevant mechanism of disability progression. Therefore, in vivo assessment of the glial cell activity is, in the emerging treatment era of primary progressive MS (PPMS), more important than ever.
Objectives: To test the association of cerebrospinal fluid (CSF) and serum markers of glial activation in PPMS patients; including glial fibrillary acidic protein (GFAP), chitinase-3-like protein 1 (CHI3L1), soluble variant of triggering receptor expressed on myeloid cells 2 (sTREM2), and marker of neuroaxonal damage (Neurofilament light chain, NfL) as well as clinical severity.
Methods: CSF and serum samples from PPMS patients were collected in the MS-centers at Universities of Freiburg (n = 49), Ulm (n = 27), Muenster (n = 11), and Rostock (n = 6). sTREM2 and CHI3L1 levels were measured using the previously reported ELISA assays, while NfL and GFAP were measured using SIMOA assays. Clinical data included age, gender, disease duration, treatment status, and Expanded Disability Status Scale (EDSS).
Results: 93 CSF samples and 71 matching serum samples were analyzed. The median age of patients was 49 years and disease duration 4.5 years. GFAPserum correlated with EDSS after correction for age (β = 0.3, p = 0.001). Furthermore, EDSS was higher in patients with a GFAPserum level ≥ 151.7 pg/ml compared to patients with GFAPserum below this cut-off (5.5 vs. 4.0, p = 0.009). Other markers did not correlate with the clinical severity. Moreover, we found a correlation between NfLCSF and GFAPCSF, sTREM2 and CHI3L1 (ρ = 0.4 for GFAPCSF and sTREM2, ρ = 0.3 for CHI3L1, p < 0.01 for sTREM2 and CHI3L1 and <0.001 for GFAPCSF). CHI3L1 did not correlate with GFAPCSF but with sTREM2 (ρ = 0.4, p < 0.01).
Discussion: The correlation between the glial activation markers in CSF with the markers of neuroaxonal demise supports the notion of the glial involvement in PPMS. The positive correlation between GFAPCSF with disease duration and GFAPserum with the clinical severity of the disease may highlight a particular role of the astrocytes in PPMS and mark the potential of GFAPserum as a disease severity marker.
Introduction
The pathophysiology of primary progressive multiple sclerosis (PPMS) is complex and involves various mechanisms including inflammatory triggered demyelination, activation of B and T lymphocytes, mitochondrial dysfunction, and iron accumulation (1). However, the glial activation is considered to play a decisive role in the progression of neuroaxonal demise (2–4). While clinical and many radiological parameters can detect the final pathway of those different pathophysiological processes (progression of clinical disability, new MRI-lesions etc.), several other aspects such as role, extent and contribution of the various pathophysiological mechanisms remain widely unexplored. A biomarker-based approach may offer a unique window to assess such disease processes in vivo (5, 6). Over the last years, the level and clinical meaning of different biomarkers in CSF like glial fibrillary acidic protein (GFAP) as a marker for astrocytic activation (7–16), chitinase 3 like 1 protein (CHI3L1) (13, 14, 17–19) and soluble triggering receptor expressed on myeloid cells 2 (sTREM2) for microglial activation (20–23) and neurofilaments light chain (NfL) for neuroaxonal damage were reported. The single molecular assay (SIMOA) enables the detection of the ultra-low concentration of some of those biomarkers in serum (16, 24, 25). We previously showed that GFAP in serum correlates with the Expanded Disability Status Scale (EDSS) specifically in PPMS but not in patients with a relapsing-remitting disease course (16). Similar results were reported later from other groups (26). In this study, we aim to reproduce these findings in a large cohort of PPMS patients and to explore the clinical meaning of the other glial activation markers in PPMS.
Methods
Patient Selection
CSF and serum samples from patients with PPMS were collected from the University Hospitals of Freiburg, Ulm, Muenster, and Rostock. The patients were admitted or seen within the Outpatient Departments between 2010 and 2018. In all patients, the diagnosis has been revised according to the McDonald criteria from 2017 (27) after careful exclusion of relevant differential diagnoses. The lumbar puncture was performed as a part of the diagnostic workup. The clinical severity was measured by assessing the Expanded Disability Status Scale (EDSS), Multiple Sclerosis Severity Score (MSSS) as well as the Age-related Multiple Sclerosis Severity Score (ARMSS) as reported recently (28).
CSF and Serum Sample Processing
A standardized protocol for CSF and serum collection was applied as previously recommended (29). Biosamples from patients were stored according to the predefined standard operating procedure (SOPs) at a local biobank at minus 80°C. Later they were transferred for measurement on dry ice to the biobank of the coordinating center in Ulm for further analysis. Hemolytic CSF specimens were excluded. From some patients, only CSF samples were available, with no matching serum samples.
Assessments of the Biomarkers
GFAP and NfL in CSF and serum were measured using Simoa assays (GFAP Discovery kits and NfL Early Access assays, Quanterix Corporation). CHI3L1 was measured using the commercial ELISA-Kits (Human Chitinase 3-like 1 Quantikine ELISA Kit DC3L10, R&D Systems). sTREM2 was measured using the previously reported ELISA using the MSD Platform (21). Samples were diluted, as recommended by the manufacturer, and concentrations were calculated using the corresponding standard curve. The intra-assay coefficient of variation (CV) was assessed by measuring a QC of serum and CSF sample in 5 replicates with a CV below 10% was obtained, whereas a CV of lower than 10% had to be achieved for a valid analysis. We did not find an influence of up to 5 freeze-thaw cycles on the investigated biomarkers, except for GFAP in CSF. Here, the concentration decreased by over 50% after within 2 freeze-thaw cycles. Therefore, GFAP CSF levels between centers were compared, and exceedingly low values were excluded from the analysis.
To compare potentially pathological serum biomarker levels, we determined a cut-off in a group of 20 patients with other non-inflammatory neurological diseases we previously published (16). As the concentration of serum GFAP in a normal or healthy population is not described, we used the 90th percentile to determine a cut-off value for further analysis. This yielded a cut-off value of 151.7 pg/ml for serum GFAP. A cut-off value for serum NfL of 16 pg/ml was suggested recently (30).
Statistical Analysis
All statistical tests were performed using SPSS® Statistics version 25 (IBM Corporation). The Shapiro-Wilk test was used to examine the distribution of the data. Mann-Whitney U test and Kruskal-Wallis test were used to compare medians in skewed distributed parameters. A multiple linear regression model and univariant general linear model was applied to account for a possible confounding bias caused by the strong correlation between GFAP levels and age. The Spearman's rho test was used to test for correlations. A p-value ≤ 0.05 was considered as statistically significant. Figures were made using GraphPad Prism 6 software (GraphPad Software Inc., La Jolla, CA, USA).
Results
Clinical Characteristics
A Total of 93 CSF and 71 matching serum samples were collected. The summary of the clinical characteristics is mentioned in Table 1, and the concentration of the different CSF and serum biomarkers are shown in Table 2. Fifty-five patients did not receive any disease modifying treatment at and before the time of sample collection. Thirty-eight patients were on a treatment: Mitoxantrone (n = 19), three monthly pulse-steroid (n = 11), Rituximab (n = 5), cyclosporine (n = 2), and Interferon beta 1a (n = 1).
Comparison Between Centers
Despite having similar patients characteristics (age, gender distribution, disease duration, and disease severity as assessed by EDSS), GFAPCSF levels differ between the centers; values form Muenster and Rostock were significantly lower than those from Ulm and Freiburg, whereas sTREM2 levels were lower only in the samples form Rostock compared to all other centers (Figure 1). Thus, in the following analysis, we excluded the GFAPCSF from Muenster and Rostock (n = 17), and sTREM2 measurements form Rostock (n = 6). Concentrations of CHI3L1, NfLCSF, NfLserum, and GFAPserum in the samples of Muenster and Rostock were included in the statistical analysis.
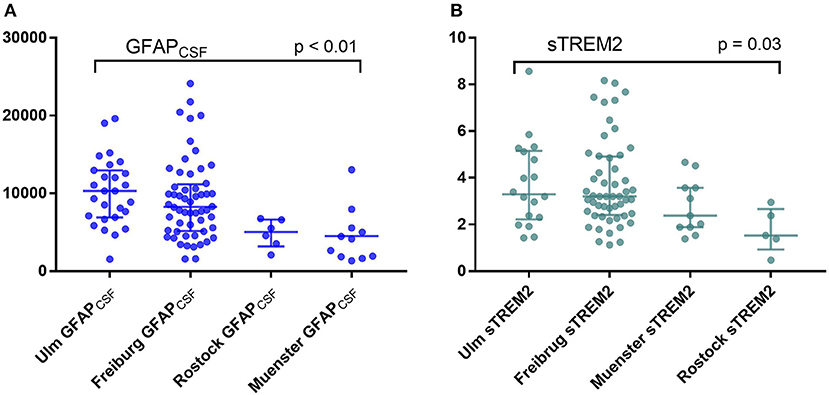
Figure 1. Comparison between the levels of (A) glial fibrillary acidic protein (GFAP) and (B) soluble triggering receptor expressed on myeloid cells 2 (sTREM2) in cerebrospinal fluid (CSF) between the four participating centers (Kruskal-Wallis test).
Clinical Aspects
None of the biomarkers correlated with the age except GFAPserum and NfLserum (ρ = 0.4 and 0.3, p = 0.005 and 0.014, respectively) (Figure 2). Gender and treatment status did not influence the levels any of the tested markers neither in CSF nor in serum (data not shown).
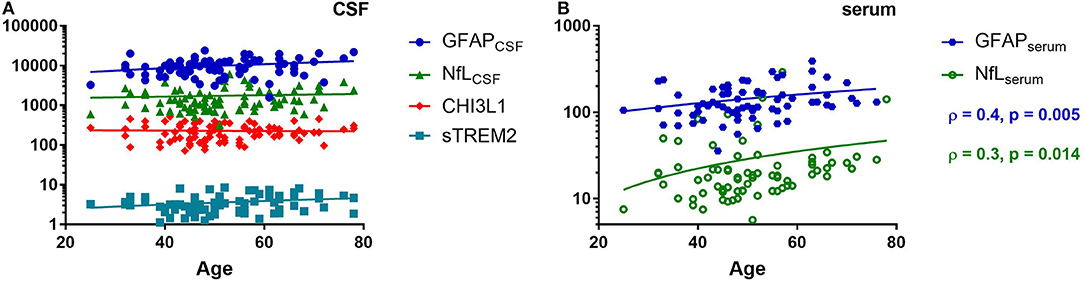
Figure 2. Spearman correlation of various cerebrospinal fluid (CSF) (A) and serum (B) biomarkers with the age of the patients. Glial fibrillary acidic protein (GFAP), neurofilaments light chain (NfL), chitinase 3 like 1 (CHI3L1), soluble triggering receptor expressed on myeloid cells 2 (sTREM2), ρ (Spearman rho).
Of all the assessed markers (in CSF and in serum), only GFAPCSF correlated with disease duration (ρ = 0.3, p = 0.014). None of the CSF markers correlated with disease severity.
Regarding the serum markers, we found a moderate correlation between GFAPserum and EDSS (ρ = 0.4, p = 0.004), which remained significant after adjusting by the effect of age (β = 0.3, p = 0.001) (Figure 3). NfLserum did not correlate with any of the disease severity parameters (Figure 3). No significant correlations were found between GFAPserum and MSSS or ARMSS (data not shown).
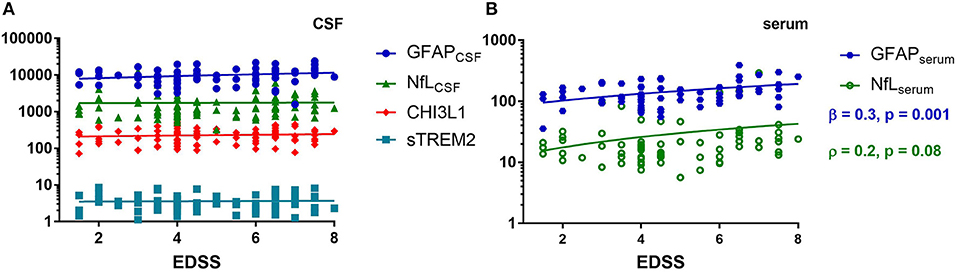
Figure 3. Spearman correlation of cerebrospinal fluid (CSF) (A) and serum (B) biomarkers with the clinical severity as measured by the expanded disability status scale (EDSS). Glial fibrillary acidic protein (GFAP), neurofilaments light chain (NfL), chitinase 3 like 1 (CHI3L1), soluble triggering receptor expressed on myeloid cells 2 (sTREM2), ρ (Spearman rho), β (standardized coefficient) of multiple linear regression, age as a covariant.
To further confirm the association of GFAPserum with the EDSS, we compared the EDSS values with GFAPserum and NfLserum levels higher or lower than the cut-off that was determined as described beforehand. Here, 63% of our PPMS patients had GFAPserum above this cut-off of 151.7 pg/ml (n = 45). Moreover, they had a significantly higher median EDSS than patients below this cut-off (5.5 vs. 4.0, p = 0.009, Figure 4). No differences were found for this comparison for NfLserum (cut-off 16 pg/ml, 4.5 vs. 4.5, p = 0.16, Figure 4). There was a significant age difference for the grouping by NfLserum but not for GFAPserum (p = 0.01 and 0.47, respectively, data not shown).
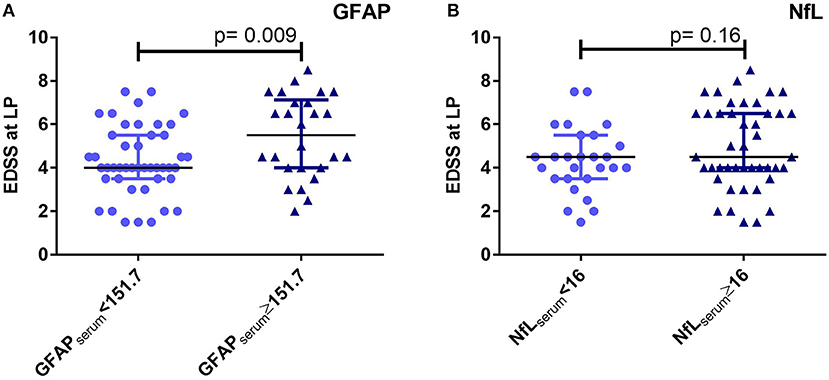
Figure 4. Comparison of expanded disability status scale (EDSS) values for primary progressive multiple sclerosis (PPMS) patients below and above cut-offs of 151.7 pg/ml for glial fibrillary acidic protein (GFAP) (A) and 16 pg/ml for neurofilaments light chain (NfL) (B) in serum (Mann-Whitney U test), respectively. There was a significant age difference for the grouping by NfL but not for GFAP (Mann-Whitney U test, p = 0.01 and 0.47, respectively). LP, lumbar puncture.
Considering NfLserum ≥ 16 pg/ml as a cut-off value for active diseases, PPMS patients with NfLserum ≥ 16 pg/ml (n = 44) have higher median concentration of GFAPserum than those with NfLserum < 16 pg/ml (n = 27) (131.0 vs. 114.5 pg/ml, p = 0.037 after correction for age, Figure 5).
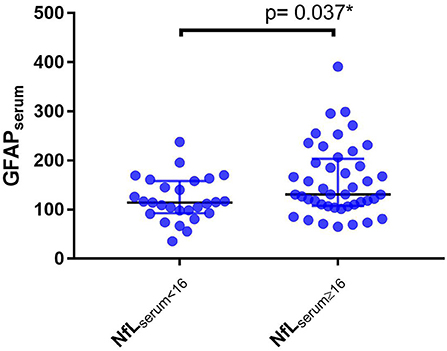
Figure 5. Level of serum glial fibrillary acidic protein (GFAPserum) in PPMS patients with serum neurofilaments light chain levels equal to or higher than 16 pg/ml (NfLserum ≥ 16) or lower the 16 pg/ml (NfLserum < 16), *Univariant general linear model corrected for age.
Correlation Between CSF and Serum Parameters
We found a moderate correlation between GFAP levels in CSF and serum (ρ = 0.4, p = 0.001), and strong correlation between levels of NfL in CSF and serum (ρ = 0.6, p < 0.001).
In CSF, NfL correlated with sTREM2 (ρ = 0.4, p < 0.01,) CHI3L1 (ρ = 0.3, p < 0.01) and GFAP (ρ = 0.4, p < 0.001, Table 3 and Figure 6). Moreover, CHI3L1 correlated with sTREM2 (ρ = 0.4, p < 0.01, Figure 7) but not with GFAP. On the other hand, GFAP did not correlate with sTREM2.
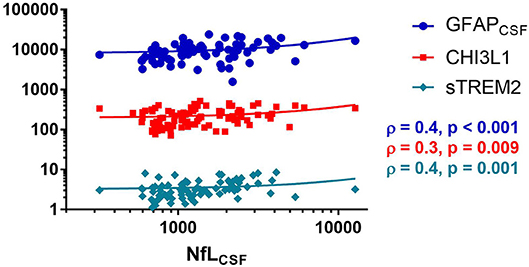
Figure 6. Spearman correlation between neurofilaments light chain (NfL), glial fibrillary acidic protein (GFAP), chitinase 3 like 1 (CHI3L1), and soluble triggering receptor expressed on myeloid cells 2 (sTREM2), ρ (Spearman rho).
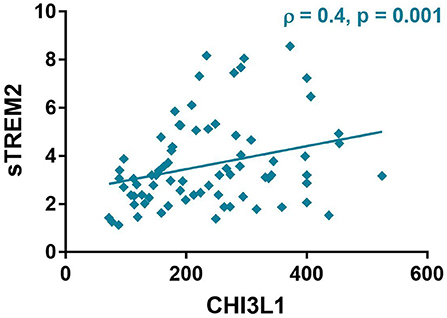
Figure 7. Spearman correlation between chitinase 3 like 1 (CHI3L1) and soluble triggering receptor expressed on myeloid cells 2 (sTREM2), ρ (Spearman rho).
Discussion
Assessment of various biomarkers reflecting different pathophysiological processes involved in the disease progression and the downstream-treatment effect is becoming increasingly important due to the emerging treatment options for progressive MS. Our study evaluates the levels of astroglial activation (GFAP), microglial activation (CHI3L1 and sTREM2) and neuroaxonal damage (NfL) in CSF and serum of a multicentric cohort of PPMS-Patients.
The glial activation is a putative cornerstone in the progression of neurodegeneration in PPMS (1). The astrocytes involvement in MS generally is a double-edged sword; while the glial scar formation may protect the tissue from further damage, it might prevent the remyelination in MS (31). The activation of astrocytes is an early event in the development of MS lesions with the release of cytokines like CCL-2, CXCL-12, MMPs, TGFβ, IL-1, IL-6, IL-10, IL-12, IL-15, IL-23, and IL-27 leading to dysfunction of the blood-brain barrier (BBB) as well as recruitment of innate and adaptive immune cells (32). Furthermore, the A1 subtype of astrocytes is a potent killer of neurons and oligodendrocytes in EAE models and was reported in acute and chronic MS lesions (33).
Nevertheless, the astrocytes in progressive MS play a more specific role in the maintenance of the local inflammation; in models of chronic EAE, levels of lactosylceramide (LacCer) synthesized by β-1,4-galactosyltransferase 6 (B4GALT6) from the activated astrocytes were elevated in the EAE-lesions. Suppression of B4GALT6-activity reduces local inflammation, microglial activation and monocytes recruitment and subsequently the resulting neurodegeneration (34).
Correlation between GFAPCSF and clinical severity were inconsistent among previous studies using the standard ELISA assay (9, 12–15). In a previous study from one of our centers, we reported a moderate correlation between levels of GFAP in serum, but not in CSF, with disease severity scores (16). Our current multicentric cohort validated our results showing a consistent correlation between GFAPserum with EDSS score, even after correction for the age of the patients. In accordance with those results, patients with GFAPserum above the proposed cut-off of 151.7 pg/ml based on our previous results, had more severe disease. Furthermore, levels with GFAPserum were higher in patients having NfLserum ≥ 16, a recently proposed cut-off value for higher activity and worse disease prognosis (30). Nevertheless, this NfL cut-off value was proposed according to pooled results from various studies with relapsing-remitting MS patients. Thus, its value in PPMS has yet to be validated.
Why GFAPserum might reflect the disease activity better than GFAPCSF is still not entirely explained. As previously suggested from our group, the enhanced expression of GFAP in the activated astrocytes end feet in the predominantly perivascular MS lesions might be directly drained into the blood compartment and not into the CSF space (16). Supporting data were reported in mouse models of EAE (35). Moreover, the GFAP, as well as other markers, might be transported to blood directly via the glymphatic system as shown recently in murine models of traumatic brain injury (36).
While a recent study suggested lower levels of GFAPserum in relapsing MS patients under treatment (26), the concentration of GFAPserum did not differ according to treatment status in our cohort. This can be explained by the fact, that none of the above-mentioned treatments were proven effective in PPMS. This observation regarding levels of GFAPserum, as well as other markers including the NfLserum, might underscore the ineffectiveness of those treatments in PPMS.
The validation of those results in our larger multicentric cohort might highlight the clinical meaning of levels of GFAP in serum as a possible serum marker in PPMS patients.
Like the astrocytes, the activated microglial release different cytokines (IL-1, Il-6, and TNF-α) as well as NO and ROS leading to exacerbation of inflammatory cascade, to attraction of inflammatory cells from blood and also to mitochondrial dysfunction, a significant mechanism in the disease progression in PMS (2, 3, 37, 38). Moreover, microglial activation in PMS appears to be diffusely prevalent not only in MS lesions but also in normal-appearing white matter (NAWM) forming the so-called microglial nodules. In the less inflammatory cortical lesions, active demyelination can be found in close proximity with the microglia (3).
Data regarding sTREM2 in PPMS are scarce with two studies reported concentration in CSF in twenty-one and in three PPMS patients, respectively (20, 22). In accordance with previous reports, no correlation was found between sTREM2 and EDSS or MSSS. Yet, sTREM2 correlated with NfL in CSF, which might highlight the role of microglia in the neuroaxonal demise in PPMS.
The meaning of CHI3L1 appears to be controversial; some reports consider it as a marker of astrocytic activation (18, 39), whereas other studies count it a marker of active microglial cells (40). The correlation between CHI3L1 and sTREM2 found in our patients is equivocal; it may underscore the microglial origin of CHI3L1 or might reflect the crosstalk between the microglia and astrocytes in PPMS (33) leaving the question regarding the origin of CHI3L1 in MS brains unanswered.
The prognostic value of CHI3L1 is prominent in CIS and RRMS patients (13, 17, 41–44) and to a lesser extent in SPMS (19). CHI3L1 in CSF did not correlate with the clinical parameters in PPMS patients in the above-mentioned studies. Consistent with the various histopathological studies, all measured glial activation markers in CSF correlated with the neuroaxonal demise as assessed by the NfL, but not with the clinical severity scores. This paradox might be due to some methodological limitations of the assays, the need of more specific markers or due to the limited ability of the applied clinical scores to reflect the extent of the neurodegeneration in PPMS. Indeed, the shortness of EDSS to reflect all aspects of the disease progression in PPMS is a lesson learned from the various negative clinical trials in PPMS (45). The upper arm function and the subtle cognitive deficits are underrepresented in the EDSS (46). Furthermore, the EDSS scores were mostly based on the walking distance reported by the patients, which might lead to an incorrect evaluation of the EDSS score (47).
Another limitation of our study is the missing detailed magnetic resonance imaging data (MRI). However, the main aim of our study was to explore the clinical meaning of the glial activation markers.
Although the concentration of most of the measured biomarkers did not vary between centers, GFAP and sTREM2 were notably lower in two of the four centers. As this is a retrospective study, we were not able to completely capture all pre-analytical procedures. To avoid potential bias of pre-analytical procedures, we did the comparison of analyte levels per center and excluded statistically low values. The excluded samples represent a minority in our cohort (17/93 for GFAP and 6/93 for sTREM2), and their exclusion had no statistical impact on the above-mentioned results (data not shown).
A point to be considered while interpreting our results is the protective role of glial cells following neuronal injury. Microglial and astrocytic activation were reported upon axonal degeneration and contributes to tissue repair and limitation of the inflammatory activity (48–50). Our markers reflect the glial activation generally, but not necessarily their pathological role. Complementary data from more specific markers of subsets of microglia or astrocytes could be helpful to understand their pathogenetic role.
In summary, we analyzed in this study various markers of glial activation in CSF and serum and evaluated their correlation with neuroaxonal damage markers and disease severity measures. The correlation between the microglial and astrocytic activation markers in CSF with the markers of neuroaxonal demise (NfL) may underscore the glial involvement in the neurodegeneration in PPMS. The positive correlation between GFAP in serum with the clinical severity of the disease may highlight the potential of GFAPserum as a disease progression marker. It his highly desirable to confirm this finding in a prospectively collected study cohort and compare it to standardized acquired MRI data. Additionally, the determination of GFAP serum levels in a large group of healthy controls might help to further differentiate between age related normal and abnormal GFAP levels.
Data Availability
The datasets generated for this study are available on request to the corresponding author.
Ethics Statement
The study was reviewed by the ethics committee of the University of Ulm, and all experimental protocols were approved (approval number 270/17). Our study was performed in accordance with the ethical standards of the 1964 Declaration of Helsinki. Written informed consent was obtained from all patients participating in this study.
Author Contributions
AA, HT, and AH: study concept. AA and TH: data acquisition, data analysis, and interpretation. AH, EM-R, and MS-C: biomarkers assessment. AA: drafting of the manuscript. HT, SR, MO, CH, UZ, and SM: study supervision and critical revision. All authors critically reviewed and approved the manuscript.
Funding
This study was supported in part by the German Federal Ministry of Education and Research (KKNMS and FTLDc), JPND networks PreFrontAls (01ED1512), the EU (FAIR-PARK II), the German Research Foundation/DFG (SFB1279), the foundation of the state Baden-Württemberg, DFG funded Koselleck Project (HA1737/16-1 to CH), and the FTD Biomarker Award (to CH and MS-C).
Conflict of Interest Statement
The authors declare that the research was conducted in the absence of any commercial or financial relationships that could be construed as a potential conflict of interest.
Acknowledgments
We acknowledge support by Deutsche Forschungsgemeinschaft and Open Access Publishing Fund of University of Tübingen.
References
1. Abdelhak A, Weber MS, Tumani H. Primary progressive multiple sclerosis: putting together the puzzle. Front Neurol. (2017) 8:234. doi: 10.3389/fneur.2017.00234
2. Mahad DH, Trapp BD, Lassmann H. Pathological mechanisms in progressive multiple sclerosis. Lancet Neurol. (2015) 14:183–93. doi: 10.1016/S1474-4422(14)70256-X
3. Lassmann H, van Horssen J, Mahad D. Progressive multiple sclerosis: pathology and pathogenesis. Nat Rev Neurol. (2012) 8:647–56. doi: 10.1038/nrneurol.2012.168
4. Lassmann H. Mechanisms of white matter damage in multiple sclerosis. Glia. (2014) 62:1816–30. doi: 10.1002/glia.22597
5. Abdelhak A, Junker A, Brettschneider J, Kassubek J, Ludolph AC, Otto M, et al. Brain-specific cytoskeletal damage markers in cerebrospinal fluid: is there a common pattern between amyotrophic lateral sclerosis and primary progressive multiple sclerosis? Int J Mol Sci. (2015) 16:17565–88. doi: 10.3390/ijms160817565
6. Tumani H, Teunissen C, Sussmuth S, Otto M, Ludolph AC, Brettschneider J. Cerebrospinal fluid biomarkers of neurodegeneration in chronic neurological diseases. Expert Rev Mol Diagn. (2008) 8:479–94. doi: 10.1586/14737159.8.4.479
7. Eng LF. Glial fibrillary acidic protein (GFAP): the major protein of glial intermediate filaments in differentiated astrocytes. J Neuroimmunol. (1985) 8:203–14. doi: 10.1016/S0165-5728(85)80063-1
8. Rosengren LE, Lycke J, Andersen O. Glial fibrillary acidic protein in CSF of multiple sclerosis patients: relation to neurological deficit. J Neurol Sci. (1995) 133:61–5. doi: 10.1016/0022-510X(95)00152-R
9. Petzold A, Eikelenboom MJ, Gveric D, Keir G, Chapman M, Lazeron RH, et al. Markers for different glial cell responses in multiple sclerosis: clinical and pathological correlations. Brain. (2002) 125 (Pt 7):1462–73. doi: 10.1093/brain/awf165
10. Malmestrom C, Haghighi S, Rosengren L, Andersen O, Lycke J. Neurofilament light protein and glial fibrillary acidic protein as biological markers in MS. Neurology. (2003) 61:1720–5. doi: 10.1212/01.WNL.0000098880.19793.B6
11. Linker RA, Brechlin P, Jesse S, Steinacker P, Lee DH, Asif AR, et al. Proteome profiling in murine models of multiple sclerosis: identification of stage specific markers and culprits for tissue damage. PLoS ONE. (2009) 4:e7624. doi: 10.1371/journal.pone.0007624
12. Axelsson M, Malmestrom C, Nilsson S, Haghighi S, Rosengren L, Lycke J. Glial fibrillary acidic protein: a potential biomarker for progression in multiple sclerosis. J Neurol. (2011) 258:882–8. doi: 10.1007/s00415-010-5863-2
13. Martinez MA, Olsson B, Bau L, Matas E, Cobo Calvo A, Andreasson U, et al. Glial and neuronal markers in cerebrospinal fluid predict progression in multiple sclerosis. Mult Scler. (2015) 21:550–61. doi: 10.1177/1352458514549397
14. Mane-Martinez MA, Olsson B, Bau L, Matas E, Cobo-Calvo A, Andreasson U, et al. Glial and neuronal markers in cerebrospinal fluid in different types of multiple sclerosis. J Neuroimmunol. (2016) 299:112–7. doi: 10.1016/j.jneuroim.2016.08.004
15. Kassubek R, Gorges M, Schocke M, Hagenston VAM, Huss A, Ludolph AC, et al. GFAP in early multiple sclerosis: a biomarker for inflammation. Neurosci Lett. (2017) 657:166–70. doi: 10.1016/j.neulet.2017.07.050
16. Abdelhak A, Huss A, Kassubek J, Tumani H, Otto M. Serum GFAP as a biomarker for disease severity in multiple sclerosis. Sci Rep. (2018) 8:14798. doi: 10.1038/s41598-018-33158-8
17. Hinsinger G, Galeotti N, Nabholz N, Urbach S, Rigau V, Demattei C, et al. Chitinase 3-like proteins as diagnostic and prognostic biomarkers of multiple sclerosis. Mult Scler. (2015) 21:1251–61. doi: 10.1177/1352458514561906
18. Querol-Vilaseca M, Colom-Cadena M, Pegueroles J, San Martin-Paniello C, Clarimon J, Belbin O, et al. YKL-40 (Chitinase 3-like I) is expressed in a subset of astrocytes in Alzheimer's disease and other tauopathies. J Neuroinflammation. (2017) 14:118. doi: 10.1186/s12974-017-0893-7
19. Burman J, Raininko R, Blennow K, Zetterberg H, Axelsson M, Malmestrom C. YKL-40 is a CSF biomarker of intrathecal inflammation in secondary progressive multiple sclerosis. J Neuroimmunol. (2016) 292:52–7. doi: 10.1016/j.jneuroim.2016.01.013
20. Piccio L, Buonsanti C, Cella M, Tassi I, Schmidt RE, Fenoglio C, et al. Identification of soluble TREM-2 in the cerebrospinal fluid and its association with multiple sclerosis and CNS inflammation. Brain. (2008) 131 (Pt 11):3081–91. doi: 10.1093/brain/awn217
21. Kleinberger G, Yamanishi Y, Suarez-Calvet M, Czirr E, Lohmann E, Cuyvers E, et al. TREM2 mutations implicated in neurodegeneration impair cell surface transport and phagocytosis. Sci Transl Med. (2014) 6:243ra86. doi: 10.1126/scitranslmed.3009093
22. Ohrfelt A, Axelsson M, Malmestrom C, Novakova L, Heslegrave A, Blennow K, et al. Soluble TREM-2 in cerebrospinal fluid from patients with multiple sclerosis treated with natalizumab or mitoxantrone. Mult Scler. (2016) 22:1587–95. doi: 10.1177/1352458515624558
23. Suarez-Calvet M, Kleinberger G, Araque Caballero MA, Brendel M, Rominger A, Alcolea D, et al. sTREM2 cerebrospinal fluid levels are a potential biomarker for microglia activity in early-stage Alzheimer's disease and associate with neuronal injury markers. EMBO Mol Med. (2016) 8:466–76. doi: 10.15252/emmm.201506123
24. Kuhle J, Barro C, Disanto G, Mathias A, Soneson C, Bonnier G, et al. Serum neurofilament light chain in early relapsing remitting MS is increased and correlates with CSF levels and with MRI measures of disease severity. Mult Scler. (2016) 22:1550–9. doi: 10.1177/1352458515623365
25. Bogoslovsky T, Wilson D, Chen Y, Hanlon D, Gill J, Jeromin A, et al. Increases of Plasma Levels of Glial Fibrillary Acidic Protein, Tau, and Amyloid beta up to 90 Days after Traumatic Brain Injury. J Neurotrauma. (2017) 34:66–73. doi: 10.1089/neu.2015.4333
26. Hogel H, Rissanen E, Barro C, Matilainen M, Nylund M, Kuhle J, et al. Serum glial fibrillary acidic protein correlates with multiple sclerosis disease severity. Mult Scler. (2018). doi: 10.1177/1352458518819380
27. Thompson AJ, Banwell BL, Barkhof F, Carroll WM, Coetzee T, Comi G, et al. Diagnosis of multiple sclerosis: 2017 revisions of the McDonald criteria. Lancet Neurol. (2017) 17:162–173. doi: 10.1016/S1474-4422(17)30470-2
28. Manouchehrinia A, Westerlind H, Kingwell E, Zhu F, Carruthers R, Ramanujam R, et al. Age related multiple sclerosis severity score: disability ranked by age. Mult Scler. (2017) 23:1938–46. doi: 10.1177/1352458517690618
29. Teunissen CE, Petzold A, Bennett JL, Berven FS, Brundin L, Comabella M, et al. A consensus protocol for the standardization of cerebrospinal fluid collection and biobanking. Neurology. (2009) 73:1914–22. doi: 10.1212/WNL.0b013e3181c47cc2
30. Calabresi P, Kuhle J, Arnold DL, Singh CM, Kinkel RP, Kappos L, et al. Serum Neurofilament Light (NfL) for Disease Prognosis and Treatment Monitoring in Multiple Sclerosis Patients: Is It Ready for Implementation into Clinical Care? Berlin: European Committee for Treatment and Research in Multiple Sclerosis (ECTRIMS) (2018).
31. Holley JE, Gveric D, Newcombe J, Cuzner ML, Gutowski NJ. Astrocyte characterization in the multiple sclerosis glial scar. Neuropathol Appl Neurobiol. (2003) 29:434–44. doi: 10.1046/j.1365-2990.2003.00491.x
32. Mayo L, Quintana FJ, Weiner HL. The innate immune system in demyelinating disease. Immunol Rev. (2012) 248:170–87. doi: 10.1111/j.1600-065X.2012.01135.x
33. Liddelow SA, Guttenplan KA, Clarke LE, Bennett FC, Bohlen CJ, Schirmer L, et al. Neurotoxic reactive astrocytes are induced by activated microglia. Nature. (2017) 541:481–7. doi: 10.1038/nature21029
34. Mayo L, Trauger SA, Blain M, Nadeau M, Patel B, Alvarez JI, et al. Regulation of astrocyte activation by glycolipids drives chronic CNS inflammation. Nat Med. (2014) 20:1147–56. doi: 10.1038/nm.3681
35. Sofroniew MV, Vinters HV. Astrocytes: biology and pathology. Acta Neuropathol. (2010) 119:7–35. doi: 10.1007/s00401-009-0619-8
36. Plog BA, Dashnaw ML, Hitomi E, Peng W, Liao Y, Lou N, et al. Biomarkers of traumatic injury are transported from brain to blood via the glymphatic system. J Neurosci. (2015) 35:518–26. doi: 10.1523/JNEUROSCI.3742-14.2015
37. Zrzavy T, Hametner S, Wimmer I, Butovsky O, Weiner HL, Lassmann H. Loss of 'homeostatic' microglia and patterns of their activation in active multiple sclerosis. Brain. (2017) 140:1900–13. doi: 10.1093/brain/awx113
38. Lassmann H. Multiple sclerosis: lessons from molecular neuropathology. Exp Neurol. (2014) 262 (Pt A):2–7. doi: 10.1016/j.expneurol.2013.12.003
39. Bonneh-Barkay D, Wang G, Starkey A, Hamilton RL, Wiley CA. In vivo CHI3L1 (YKL-40) expression in astrocytes in acute and chronic neurological diseases. J Neuroinflammation. (2010) 7:34. doi: 10.1186/1742-2094-7-34
40. Michelucci A, Heurtaux T, Grandbarbe L, Morga E, Heuschling P. Characterization of the microglial phenotype under specific pro-inflammatory and anti-inflammatory conditions: effects of oligomeric and fibrillar amyloid-beta. J Neuroimmunol. (2009) 210:3–12. doi: 10.1016/j.jneuroim.2009.02.003
41. Comabella M, Fernandez M, Martin R, Rivera-Vallve S, Borras E, Chiva C, et al. Cerebrospinal fluid chitinase 3-like 1 levels are associated with conversion to multiple sclerosis. Brain. (2010) 133 (Pt 4):1082–93. doi: 10.1093/brain/awq035
42. Canto E, Tintore M, Villar LM, Costa C, Nurtdinov R, Alvarez-Cermeno JC, et al. Chitinase 3-like 1: prognostic biomarker in clinically isolated syndromes. Brain. (2015) 138 (Pt 4):918–31. doi: 10.1093/brain/awv017
43. Quintana E, Coll C, Salavedra-Pont J, Munoz-San Martin M, Robles-Cedeno R, Tomas-Roig J, et al. Cognitive impairment in early stages of multiple sclerosis is associated with high cerebrospinal fluid levels of chitinase 3-like 1 and neurofilament light chain. Eur J Neurol. (2018) 25:1189–91. doi: 10.1111/ene.13687
44. Sellebjerg F, Royen L, Soelberg Sorensen P, Oturai AB, Jensen PEH. Prognostic value of cerebrospinal fluid neurofilament light chain and chitinase-3-like-1 in newly diagnosed patients with multiple sclerosis. Mult Scler. (2018). doi: 10.1177/1352458518794308
45. Ontaneda D, Fox RJ, Chataway J. Clinical trials in progressive multiple sclerosis: lessons learned and future perspectives. Lancet Neurol. (2015) 14:208–23. doi: 10.1016/S1474-4422(14)70264-9
46. Tur C, Moccia M, Barkhof F, Chataway J, Sastre-Garriga J, Thompson AJ, et al. Assessing treatment outcomes in multiple sclerosis trials and in the clinical setting. Nat Rev Neurol. (2018) 14:75–93. doi: 10.1038/nrneurol.2017.171
47. Skjerbaek AG, Boesen F, Petersen T, Rasmussen PV, Stenager E, Norgaard M, et al. Can we trust self-reported walking distance when determining EDSS scores in patients with multiple sclerosis? The Danish MS hospitals rehabilitation study. Mult Scler. (2018). doi: 10.1177/1352458518795416
48. Belanger M, Magistretti PJ. The role of astroglia in neuroprotection. Dialogues Clin Neurosci. (2009) 11:281–95.
49. Neumann H, Kotter MR, Franklin RJ. Debris clearance by microglia: an essential link between degeneration and regeneration. Brain. (2009) 132 (Pt 2):288–95. doi: 10.1093/brain/awn109
Keywords: SiMoA, GFAP, PPMS, glial activation, progressive multiple sclerosis, neurofilaments, CHI3L1, sTREM2
Citation: Abdelhak A, Hottenrott T, Morenas-Rodríguez E, Suárez-Calvet M, Zettl UK, Haass C, Meuth SG, Rauer S, Otto M, Tumani H and Huss A (2019) Glial Activation Markers in CSF and Serum From Patients With Primary Progressive Multiple Sclerosis: Potential of Serum GFAP as Disease Severity Marker? Front. Neurol. 10:280. doi: 10.3389/fneur.2019.00280
Received: 13 January 2019; Accepted: 04 March 2019;
Published: 26 March 2019.
Edited by:
Thomas Skripuletz, Hannover Medical School, GermanyReviewed by:
Anna Margherita Pietroboni, IRCCS Ca 'Granda Foundation Maggiore Policlinico Hospital (IRCCS), ItalyMassimiliano Castellazzi, University of Ferrara, Italy
Copyright © 2019 Abdelhak, Hottenrott, Morenas-Rodríguez, Suárez-Calvet, Zettl, Haass, Meuth, Rauer, Otto, Tumani and Huss. This is an open-access article distributed under the terms of the Creative Commons Attribution License (CC BY). The use, distribution or reproduction in other forums is permitted, provided the original author(s) and the copyright owner(s) are credited and that the original publication in this journal is cited, in accordance with accepted academic practice. No use, distribution or reproduction is permitted which does not comply with these terms.
*Correspondence: Hayrettin Tumani, aGF5cmV0dGluLnR1bWFuaUB1bmktdWxtLmRl
†These authors have contributed equally to this work