- 1Department of Neurosurgery, The Second Affiliated Hospital, Chongqing Medical University, Chongqing, China
- 2Department of Neurology, The Second Affiliated Hospital, Chongqing Medical University, Chongqing, China
- 3Department of Functional Neurosurgery, Nanjing Brain Hospital Affiliated to Nanjing Medical University, Nanjing, China
Background: Deep brain stimulation (DBS) of the subthalamic nucleus (STN) or globus pallidus internus (GPi) have been proven to be equally effective in improving motor-symptoms for advanced Parkinson's disease (PD) patients. However, it is unclear that which target stimulation is more effective in reducing dyskinesia. We conducted the meta-analysis to evaluate the efficacy of STN and GPi-DBS in the dyskinesia.
Methods: A systematic search was performed in PubMed, Embase, and the Cochrane Library databases. Controlled trials about the dyskinesia comparing the efficacy of GPi and STN DBS were included. Clinical data of dyskinesia and levodopa equivalent doses (LED) were collected for the meta-analysis.
Results: Eight eligible trials containing a total of 822 patients were included in this meta-analysis. Our results showed that GPi DBS offered a greater reduction of dyskinesia than STN DBS at 12 months after surgery, with an overall pooled SMD of 0.32 (95% CI = 0.06 to 0.59, P = 0.02). Treatment of STN DBS was associated with a greater reduction of LED compared with GPi DBS, with a change score of −320.55 (95% CI = −401.36 to −239.73, P < 0.00001).
Conclusion: GPi DBS is superior to reduce dyskinesia than STN DBS at 12 months after surgery for advanced PD patients. Further studies should focus on the different mechanism for dyskinesia reduction by GPi or STN DBS.
Introduction
Parkinson's disease (PD) is a chronic and neurodegenerative disorder which affects 1% of the population over 60 years old (1). Dopamine replacement therapy remained the most effective symptomatic treatment of PD since Levodopa was first introduced for the treatment with PD in the 1960s. However, dopaminergic therapies are eventually associated with motor fluctuations and levodopa-induced dyskinesia. In a community-based study, the mean times of onset of dyskinesia were 6.6 years (2). Other studies have reported that 50% of PD patients experienced dyskinesia after 5 years from introduction of L-dopa (3), and this percentage up to 95% after 15 years of therapy (4). The clinical manifestations of the dyskinesia included head, hand, foot, body, and trunk of involuntary movement. General types of dyskinesia could be divided into peak-dosed dyskinesia (PDSK), diphasic dyskinesia (DDSK), and off-period dystonia according to the course of the disease, clinical manifestation, and the relationship with medicine. As the curative effect decreased gradually, off-period dystonia appeared in the early morning or night, resulting in leg and foot cramp (5). Different types of dyskinesia were observed in PD patients. PDSK, off-period dystonia and DDSK were accounted for about 80, 30, and 20%, respectively. Furthermore, different types of dyskinesia could appear or appear alternately in the same patient at the same time (6). Previous studies have shown that incidence of dyskinesia was positively correlated with following factors, including youth, women, long course of levodopa treatment, high dose levodopa treatment and low weight (7). Moreover, some studies demonstrated that PD patients with stiffness had a higher incidence of dyskinesia than tremor (8).
Dyskinesia is unfavorable for quality of life, sometimes being more disabling than PD itself (9, 10). Lower doses and more frequent administration of levodopa may reduce dyskinesia in some patients. However, parkinsonian symptoms and motor fluctuations became worse with the reduction of L-dopa in many cases (11). So patients were encountered with the difficult choice between accepting more serious dyskinesia with better control of PD and less dyskinesia but accompanied by a worsening of PD symptoms. Consequently, it is critical to focus on more effective strategies in order to reduce dyskinesia in the on-state.
Deep brain stimulation (DBS), officially approved by the FDA in 2002, has been proven to improve motor symptoms and dyskinesia. STN and GPi are the two most commonly selected targets. Increasing evidence from randomized clinical trials indicated that the STN DBS and GPi DBS are equally effective in improving motor symptoms and suggests the same in improving dyskinesia (12–15). However, there has been discrepancy as to dyskinesia reduction between two targets. Several randomized controlled trials (RCTs) demonstrated that dyskinesia reduction from GPi DBS was superior to STN DBS (16), whereas other studies indicated there was no significant difference between two targets (17, 18). Up to now, it still remains inconclusive about which target stimulation is more effective in reducing dyskinesia. In the present study, we performed this meta-analysis to evaluate the efficacy of STN and GPi-DBS in the dyskinesia.
Methods
Search Strategy and Selection Criteria
A systematic search for articles written in English was performed in PubMed, Cochrane library, and Embase databases according to PRISMA guidelines (19). Databases were searched from inception to January 2018. Medical Subject Headings (MeSH) terms and corresponding keywords were exploded in the electronic search process. The search terms were (MeSH exp Parkinson Disease, and keywords Idiopathic Parkinson's disease, Primary Parkinsonism), (MeSH exp Deep Brain Stimulation and keywords Electrical Stimulation of the Brain and Deep Brain Stimulations), and (MeSH exp Dyskinesias and keywords Dyskinesia). We also examined reference lists of all eligible studies and reviews in the field for further possible titles. The process was repeated until no new titles were found.
The initial search was conducted by two reviewers independently (YL and FL). Retrieved literatures were imported into endnote, with duplication discarded. Unrelated literatures were excluded after scanning of titles and abstracts carefully. Full-text articles of the remaining literatures were acquired to identify eligibility. Any discrepancy was resolved by discussion or decided by a third reviewer (HL). The PRISMA statement flow diagram displayed the process of literature search and selection, as shown in Figure 1. Published studies were included by meeting the following criteria: (1) population: patients with PD were responsive to levodopa; (2) intervention: GPi DBS or STN DBS (either bilateral or unilateral); (3) comparison: STN DBS or GPi DBS (either bilateral or unilateral); (4) reporting clinical data of dyskinesia before and after surgery. Literatures were excluded for the following reasons: (1) maximum follow-up time < 6 months; (2) data from conference abstracts or literatures that could not be extracted.
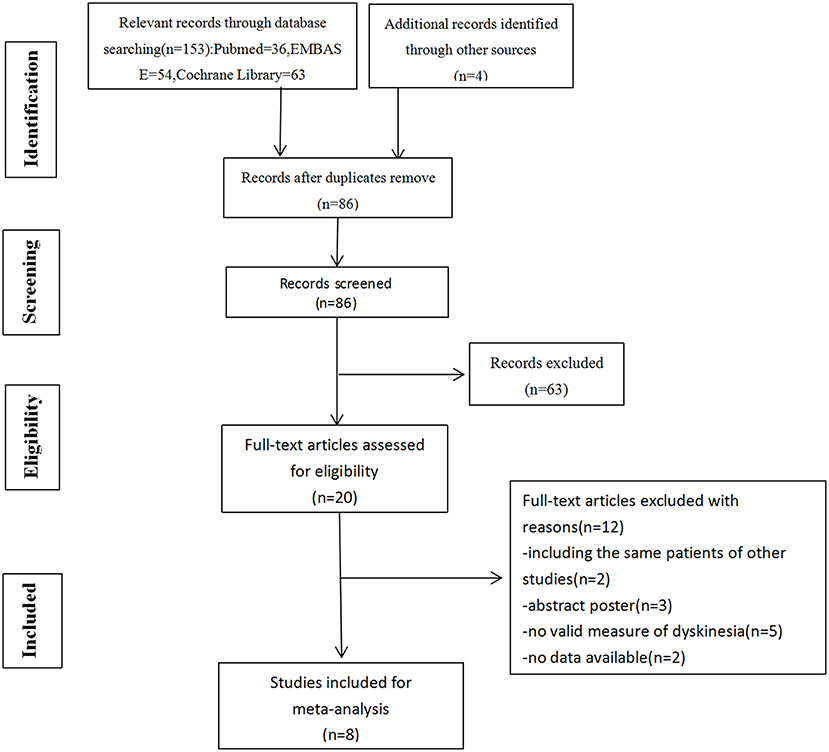
Figure 1. Flow diagram of selection of studies. Among 157 articles screened, 5 randomized controlled studies and 3 non-randomized controlled trials were included in our meta-analysis.
Data Extraction
Key characteristics of studies were extracted independently by two authors (QH and LC), ready for comparative analysis. All data were tabulated onto a predefined spreadsheet. For each included study, the following were extracted: authors, title, journal, year of publication, participant characteristics, dyskinesia scores, LED scores, and assessment time points in relation to DBS.
Data Analysis
Data analysis was performed by the RevMan 5.3 (The Cochrane Collaboration, London, UK). All the outcomes were displayed in consistent data. Standardized mean differences (SMD) with 95% confidence intervals (CI) were calculated for dyskinesia, since the analyzed domains involved multiple testing instruments. Mean differences (MD) with 95% CI were reported for the LED. The heterogeneity across studies was calculated using I-square and chi-square. Once the heterogeneity was small (I2 < 50%), the fixed-effects model was used; otherwise, the random effects model was used. P < 0.05 was considered statistically significant.
Quality Assessment
The methodological quality of the selected studies was assessed using Cochrane collaboration's tool. The risk of bias tool included six domains: selection, performance, detection, attrition, reporting and other bias (20). Methodological Index for Non-randomized Studies (MINORS) was used for assessing the quality of non-randomized controlled studies. MINORS involved 12 items for comparative studies, subsequently each item was scored from 0 to 2; 0 indicating that it was not reported in the article, 1 indicating that it was reported but inadequately, and 2 indicating that it was reported adequately (21).
Results
Study Characteristics
Initially, we identified 157 articles, 86 of which remained after removal of duplicates. A total of 20 full-text articles were assessed for eligibility, 5 randomized controlled studies and 3 non-randomized controlled trials were included in our meta-analysis at the end. Totally, 822 patients were included, among which 453 had been implanted with STN DBS, 369 with GPi DBS. The characteristics of the studies were presented in Table 1.
Study Quality
Study quality of RCTs was evaluated by Cochrane collaboration's tool, Two RCTs were classified as high quality (16, 25), and the other three RCTs were classified as moderate quality (17, 18, 22). Quality assessment results were presented in Figure 2. For the other three cohort studies evaluated by Methodological Index for Non-randomized Studies (MINORS), two studies (24, 26) scored 16 points and one study (23) scored 18 points, which could be regarded as at moderate-quality (Table 2). Thus, all included studies were deemed to be of the moderate or high quality. Most RCTs lost points because of the lack of blinding and allocation concealment. While most cohort studies lost points because of a statement of the outcome of interest at the beginning and non-blind outcome assessment.
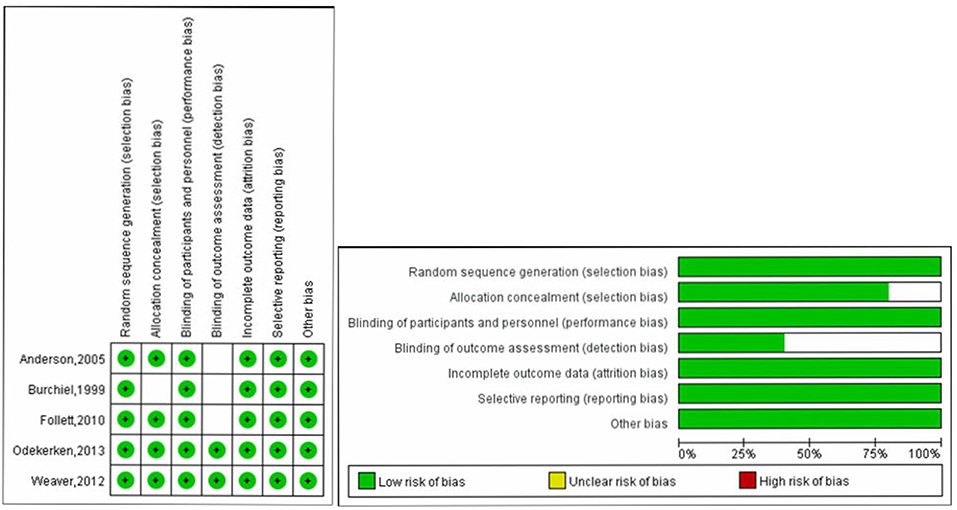
Figure 2. Quality assessment of RCTs using Cochrane collaboration's tool for assessing risk of bias.
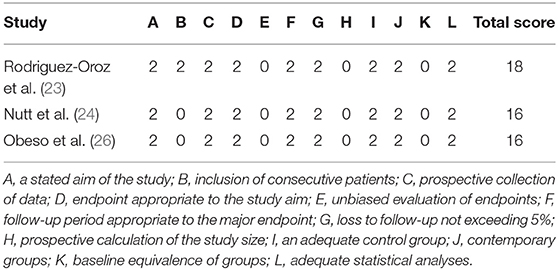
Table 2. Risk of bias results assessed with methodological index for non-randomized studies (MINORS).
Profile Comparison
Meta-analysis results of pretreatment profiles were shown in Table 3. Significant heterogeneity was detected in duration of disease (I2 = 87%) and dyskinesia (I2 = 46%). The heterogeneity was greatly reduced (I2 = 0%) when two studies (24, 26) were excluded. A significant difference in pretreatment age was observed in STN DBS group compared with GPI DBS group, with an overall pooled MD of 1.34 (95% CI = [0.12, 2.56]), indicating that the patients with STN DBS were generally older than the patients with GPi DBS. There were no significant differences and heterogeneity in the other comparisons of pretreatment profiles. Forest plots of each comparison were presented in Supplementary Data (S1–S6).
Changes in Dyskinesia Scores
Based on the results of meta-analysis, GPi DBS did not yield any significant improvement in the dyskinesia score over STN DBS, with a change score of 0.13 (95% CI = −0.01 to 0.27, P = 0.006; Figure 3). No significant differences in heterogeneity was observed between treatment groups (X2 = 4.33, df = 7, p = 0.74, I2 = 0%). We conducted subgroup analyses according to follow-up periods. A greater reduction of dyskinesia was observed in GPi DBS group compared with STN DBS group at 12 months after surgery, with an overall pooled SMD of 0.32 (95% CI = 0.06 to 0.59, P = 0.02; Figure 4), with evidence of no heterogeneity (X2 = 1.62, df = 4, p = 0.81, I2 = 0%). However, no significant differences and heterogeneity were observed in the other follow-up periods.
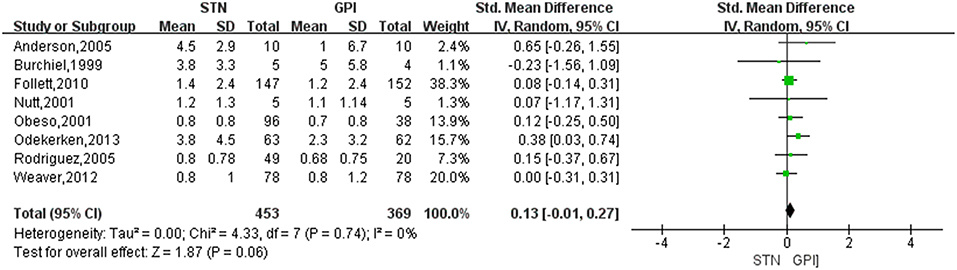
Figure 3. Forest plot of mean difference of dyskinesia score in the on-medication/on-stimulation state between STN DBS and GPi DBS. GPi, globus pallidus interna; STN, subthalamic nucleus; IV, inverse variance; CI, confidence interval; Std, standardized.
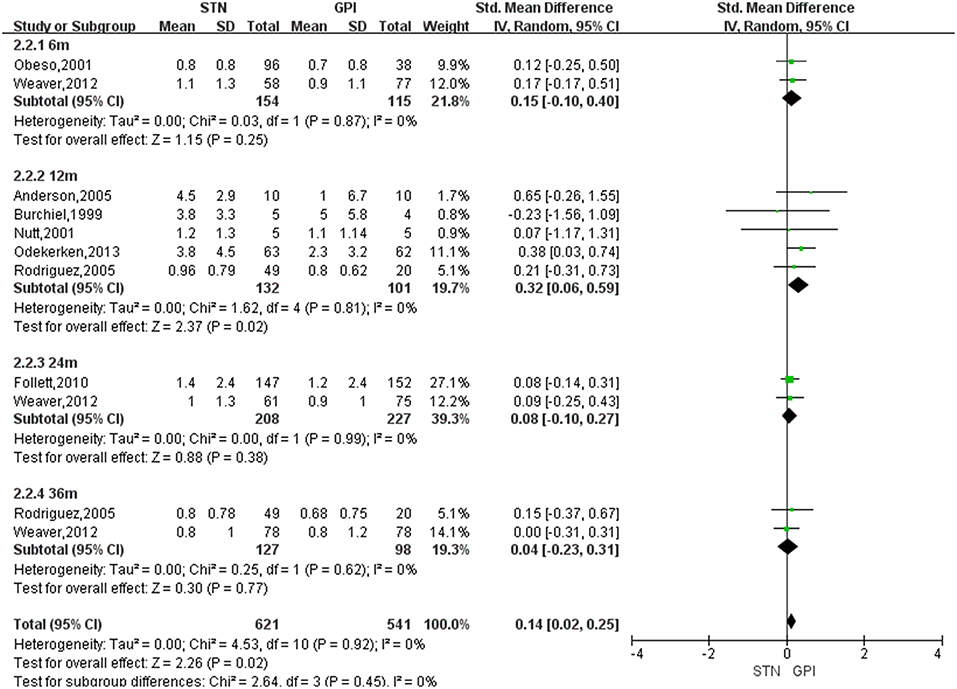
Figure 4. Forest plot: subgroup analyses were conducted according to follow-up periods in dyskinesia score between STN DBS and GPi DBS. GPi, globus pallidus interna; STN, subthalamic nucleus; IV, inverse variance; CI, confidence interval; Std, standardized.
Changes in LED Scores
Treatment of STN DBS was associated with a greater reduction of LED compared with GPi DBS, with a change score of −320.55 (95% CI = −401.36 to −239.73, P < 0.00001; Figure 5). Based on the Chi-square and I-square analyses, there was small difference in heterogeneity between treatment groups (X2 = 4.47, df = 4, p = 0.35, I2 = 10%). The heterogeneity was greatly reduced (I2 = 0%) when two studies (17, 24) were exclude [Figure 6, for example, the study by Follett et al. (17) was excluded]. However, even after excluding one or the other of those studies, LED were still reduced to a greater extent after STN DBS than GPi DBS.
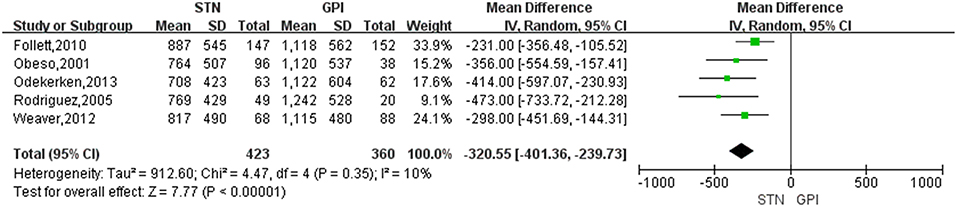
Figure 5. Forest plot of standardized mean difference of levodopa equivalent doses between STN DBS and GPi DBS. GPi, globus pallidus interna; STN, subthalamic nucleus; IV, inverse variance; CI, confidence interval.
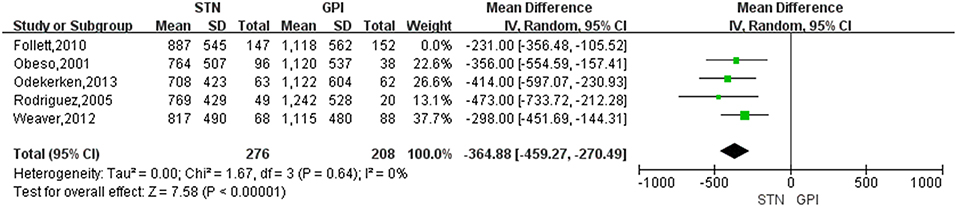
Figure 6. Forest plot: sensitivity analysis. GPi, globus pallidus interna; STN, subthalamic nucleus; IV, inverse variance; CI, confidence interval.
Publication Bias
Publication bias was estimated by funnel plots. No obvious asymmetry was identified in funnel plots, indicating that there was no publication bias (Supplementary Data S7).
Discussion
The current meta-analysis provides a review of the efficacy of STN and GPi DBS in the dyskinesia in the treatment of advanced PD. Eight controlled clinical trials were included in this meta-analysis. Changes in dyskinesia scores and LED scores from baseline values after DBS were used to assess improvements in dyskinesia and medication use in patients with PD. Our findings revealed that there was a greater reduction of dyskinesia scores from GPi DBS compared with STN DBS at 12 months follow-up. There were two randomized clinical trials that revealed that GPi stimulation was superior in dyskinesia reduction to STN stimulation at 12 months after surgery (16, 22). However, there was no statistically significant difference between GPi DBS and STN DBS in the other follow-up periods, which was consistent with the VA Cooperative Study (25). Furthermore, STN DBS allowed for medication dosages to be reduced to lower levels than GPi DBS. Therefore, our results indicated that GPi DBS offered a greater reduction of dyskinesia than STN DBS at 12 months after surgery.
DBS has been established as an important therapeutic strategy to relieve motor symptoms in advanced PD patients when motor symptoms are no longer managed adequately with levodopa treatment (27). STN and GPi are the two most commonly selected targets. Moreover, mounting evidence has confirmed similar effect of the two targets stimulation on improvement of motor function and dyskinesia observed in several meta-analyses of RCTs involved in DBS therapy (28–30). Nevertheless, the mechanisms of dyskinesia reduction in STN and GPi DBS are fundamentally different. GPi stimulation improved dyskinesia through direct stimulation effects on dopaminergic pathways to inhibit abnormal electrical activity of GPi (22, 31, 32), while STN stimulation reduced dyskinesia by lowering greater dopaminergic medication to minimize dyskinesia (16, 33). Further investigations are needed to focus on the exact mechanisms of dyskinesia changes after stimulation of the two targets.
Targets election for DBS should be assessed based on the patient's specific characteristics and goals. Deep brain stimulation of the STN is advantageous if the main goal is dopaminergic medication reduction. However, medication reduction may aggravate depression and apathy, even increase suicidal ideation (34). GPi stimulation rather than STN stimulation can be considered in patients with cognitive decline or mood changes (25). In patients with prominent gait disorder, axial symptoms, or falls, GPi DBS may be preferable (35). Successful GPi DBS was also applied in cases of persistent or severe dyskinesia, especially if they were unable to sufficiently reduce dopaminergic treatment (36).
The changes in LED observed in our analysis were consistent with the results of the meta-analysis (29) and the outcome of other recent studies, which indicated that medication was markedly reduced after STN DBS compared with GPi DBS. Although medication reduction is not the primary goal of surgery, dopaminergic requirements are reduced, with the additional advantageous of decreased fluctuations in “on” and “off” state, drug-induced dyskinesia, and other complications of medications (28–30). However, the reduction in medication should be managed cautiously, neurosurgeons have to avoid aggressive medication reduction after STN DBS, since apathy, depressive symptoms, and increased suicidal ideation may occur once levodopa was rapidly withdrawn (34). Previous study demonstrated that the loss of prior positive effects of STN stimulation in the medication “on” phase especially for gait and balance was related to a reduction in dopaminergic medication, not observed in GPi-DBS patients which retained stable scores (37). This contributed to various thoughts such as the desirability of medication reduction in the absence of side effects, the relationship between medications and stimulation.
Some limitations should be considered in our study. First, three studies lacked LED data (18, 22, 24). The involved studies were conducted with various implantation techniques, stimulators, stimulation parameters, and postoperative management. Therefore, potential risks of significant heterogeneity were undefined. Both randomized and non-randomized studies were included in the same analysis, which might result in potential bias. However, even after excluding the non-randomized studies, the outcomes were still stable (Supplementary Data S8). Second, the analyzed domains about dyskinesia involved multiple testing instruments, and the measurements in those studies of our meta-analysis were performed in different times after surgery, which might cause bias. Finally, we only included studies published in English, which might result in potential bias.
Conclusion
GPi DBS is superior to reduce dyskinesia than STN DBS at 12 months after surgery for advanced PD patients, and the mechanisms of dyskinesia reduction in STN and GPi DBS are fundamentally different. STN DBS allowed for significant dopaminergic medication reduction. Further studies should focus on the different mechanism for dyskinesia reduction by GPi or STN DBS.
Author Contributions
YL and ZX: conception and design, drafting the article. QH and LC: acquisition of data. YL, FL, and HL: analysis and interpretation of data. ZX: approved the final version of the manuscript on behalf of all authors. ZX, WZ, YC, LC, and YL: study supervision. All authors critically revising the article, reviewed submitted version of manuscript.
Funding
This work was supported by Key Project of Medical Science and technology development Foundation, Nanjing Department of Health (Grants No. ZKX15032) and Jiangsu Provincial key research and development program (Grants No. BE2016614).
Conflict of Interest Statement
The authors declare that the research was conducted in the absence of any commercial or financial relationships that could be construed as a potential conflict of interest.
Supplementary Material
The Supplementary Material for this article can be found online at: https://www.frontiersin.org/articles/10.3389/fneur.2019.00151/full#supplementary-material
References
1. Connolly BS, Lang AE. Pharmacological treatment of Parkinson disease: a review. JAMA (2014) 311:1670–83. doi: 10.1001/jama.2014.3654
2. Evans JR, Mason SL, Williams-Gray CH, Foltynie T, Brayne C, Robbins TW, et al. The natural history of treated Parkinson's disease in an incident, community based cohort. J Neurol Neurosurg Psychiatry (2011) 82:1112–8. doi: 10.1136/jnnp.2011.240366
3. Ahlskog JE, Muenter MD. Frequency of levodopa-related dyskinesias and motor fluctuations as estimated from the cumulative literature. Mov Disord. (2001) 16:448–58. doi: 10.1002/mds.1090
4. Hely MA, Morris JG, Reid WG, Trafficante R. Sydney multicenter study of Parkinson's disease: non-L-dopa-responsive problems dominate at 15 years. Mov Disord. (2005) 20:190–9. doi: 10.1002/mds.20324
5. Iansek R, Centre K, Victoria C. Pharmacological management of Parkinson's disease. J Pharm Pract Res. (2004) 34:229–32. doi: 10.1002/jppr2004343229
6. Rieck M, Schumacher-Schuh AF, Callegari-Jacques SM, Altmann V, Schneider Medeiros M, Rieder CR, et al. Is there a role for ADORA2A polymorphisms in levodopa-induced dyskinesia in Parkinson's disease patients? Pharmacogenomics (2015) 16:573–82. doi: 10.2217/pgs.15.23
7. Kumar N, Van Gerpen JA, Bower JH, Ahlskog JE. Levodopa-dyskinesia incidence by age of Parkinson's disease onset. Mov Disord. (2005) 20:342–4. doi: 10.1002/mds.20360
8. Zappia M, Annesi G, Nicoletti G, Arabia G, Annesi F, Messina D, et al. Sex differences in clinical and genetic determinants of levodopa peak-dose dyskinesias in Parkinson disease: an exploratory study. Arch Neurol. (2005) 62:601–5. doi: 10.1001/archneur.62.4.601
9. Pechevis M, Clarke CE, Vieregge P, Khoshnood B, Deschaseaux-Voinet C, Berdeaux G, et al. Effects of dyskinesias in Parkinson's disease on quality of life and health-related costs: a prospective European study. Eur J Neurol. (2005) 12:956–63. doi: 10.1111/j.1468-1331.2005.01096.x
11. Rascol O, Goetz C, Koller W, Poewe W, Sampaio C. Treatment interventions for Parkinson's disease: an evidence based assessment. Lancet (2002) 359:1589–98. doi: 10.1016/S0140-6736(02)08520-3
12. Fasano A, Daniele A, Albanese A. Treatment of motor and non-motor features of Parkinson's disease with deep brain stimulation. Lancet Neurol. (2012) 11:429–42. doi: 10.1016/S1474-4422(12)70049-2
13. Williams A, Gill S, Varma T, Jenkinson C, Quinn N, Mitchell R, et al. Deep brain stimulation plus best medical therapy versus best medical therapy alone for advanced Parkinson's disease (PD SURG trial): a randomised, open-label trial. Lancet Neurol. (2010) 9:581–91. doi: 10.1016/S1474-4422(10)70093-4
14. Weaver FM, Follett K, Stern M, Hur K, Harris C, Marks WJ Jr, et al. Bilateral deep brain stimulation vs best medical therapy for patients with advanced Parkinson disease: a randomized controlled trial. JAMA (2009) 301:63–73. doi: 10.1001/jama.2008.929
15. Tan ZG, Zhou Q, Huang T, Jiang Y. Efficacies of globus pallidus stimulation and subthalamic nucleus stimulation for advanced Parkinson's disease: a meta-analysis of randomized controlled trials. Clin Interv Aging (2016) 11:777–86. doi: 10.2147/CIA.S105505
16. Odekerken VJ, van Laar T, Staal MJ, Mosch A, Hoffmann CF, Nijssen PC, et al. Subthalamic nucleus versus globus pallidus bilateral deep brain stimulation for advanced Parkinson's disease (NSTAPS study): a randomised controlled trial. Lancet Neurol. (2013) 12:37–44. doi: 10.1016/S1474-4422(12)70264-8
17. Follett KA, Weaver FM, Stern M, Hur K, Harris CL, Luo P, et al. Pallidal versus subthalamic deep-brain stimulation for Parkinson's disease. N Engl J Med. (2010) 362:2077–91. doi: 10.1056/NEJMoa0907083
18. Burchiel KJ, Anderson VC, Favre J, Hammerstad JP. Comparison of pallidal and subthalamic nucleus deep brain stimulation for advanced Parkinson's disease: results of a randomized, blinded pilot study. Neurosurgery (1999) 45:1375–82. doi: 10.1097/00006123-199912000-00024
19. Moher D, Liberati A, Tetzlaff J, Altman DG. Preferred reporting items for systematic reviews and meta-analyses: the PRISMA statement. PLoS Med. (2009) 6:e1000097. doi: 10.1371/journal.pmed.1000097
20. Higgins JP, Green S. Cochrane handbook for systematic reviews of interventions. Naunyn-Schmiedebergs Arch Exp Pathol Pharmakol. (2011) 5:S38.
21. Slim K, Nini E, Forestier D, Kwiatkowski F, Panis Y, Chipponi J. Methodological index for non-randomized studies (minors): development and validation of a new instrument. ANZ J Surg. (2003) 73:712–6. doi: 10.1046/j.1445-2197.2003.02748.x
22. Anderson VC, Burchiel KJ, Hogarth P, Favre J, Hammerstad JP. Pallidal vs subthalamic nucleus deep brain stimulation in Parkinson disease. Arch Neurol. (2005) 62:554–60. doi: 10.1001/archneur.62.4.554
23. Rodriguez-Oroz MC, Obeso JA, Lang AE, Houeto JL, Pollak P, Rehncrona S, et al. Bilateral deep brain stimulation in Parkinson's disease: a multicentre study with 4 years follow-up. Brain (2005) 128:2240–9. doi: 10.1093/brain/awh571
24. Nutt JG, Rufener SL, Carter JH, Anderson VC, Pahwa R, Hammerstad JP, et al. Interactions between deep brain stimulation and levodopa in Parkinson's disease. Neurology (2001) 57:1835–42. doi: 10.1212/WNL.57.10.1835
25. Weaver FM, Follett KA, Stern M, Luo P, Harris CL, Hur K, et al. Randomized trial of deep brain stimulation for Parkinson disease: thirty-six-month outcomes. Neurology (2012) 79:55–65. doi: 10.1212/WNL.0b013e31825dcdc1
26. Obeso JA, Olanow CW, Rodriguez-Oroz MC, Krack P, Kumar R, Lang AE. Deep-brain stimulation of the subthalamic nucleus or the pars interna of the globus pallidus in Parkinson's disease. N Engl J Med. (2001) 345:956–63. doi: 10.1056/NEJMoa000827
27. Okun MS. Deep-brain stimulation for Parkinson's disease. N Engl J Med. (2013) 368:483–4. doi: 10.1056/NEJMc1214078
28. Sako W, Miyazaki Y, Izumi Y, Kaji R. Which target is best for patients with Parkinson's disease? A meta-analysis of pallidal and subthalamic stimulation. J Neurol Neurosurg Psychiatry (2014) 85:982–6. doi: 10.1136/jnnp-2013-306090
29. Liu Y, Li W, Tan C, Liu X, Wang X, Gui Y, et al. Meta-analysis comparing deep brain stimulation of the globus pallidus and subthalamic nucleus to treat advanced Parkinson disease. J Neurosurg. (2014) 121:709–18. doi: 10.3171/2014.4.JNS131711
30. Xu H, Zheng F, Krischek B, Ding W, Xiong C, Wang X, et al. Subthalamic nucleus and globus pallidus internus stimulation for the treatment of Parkinson's disease: a systematic review. J Int Med Res. (2017) 45:1602–12. doi: 10.1177/0300060517708102
31. Lozano AM, Lang AE, Levy R, Hutchison W, Dostrovsky J. Neuronal recordings in Parkinson's disease patients with dyskinesias induced by apomorphine. Ann Neurol. (2000) 47:S141–6.
32. Wu YR, Levy R, Ashby P, Tasker RR, Dostrovsky JO. Does stimulation of the GPi control dyskinesia by activating inhibitory axons? Mov Disord. (2001) 16:208–16. doi: 10.1002/mds.1046
33. Sankar T, Lozano AM. Surgical approach to l-dopa-induced dyskinesias. Int Rev Neurobiol. (2011) 98:151–71. doi: 10.1016/B978-0-12-381328-2.00006-7
34. Wagle Shukla A, Okun MS. Surgical treatment of Parkinson's disease: patients, targets, devices, and approaches. Neurotherapeutics (2014) 11:47–59. doi: 10.1007/s13311-013-0235-0
35. Ramirez-Zamora A, Ostrem JL. Globus pallidus interna or subthalamic nucleus deep brain stimulation for Parkinson disease: a review. JAMA Neurol. (2018) 75:367–72. doi: 10.1001/jamaneurol.2017.4321
36. Krishnan S, Pisharady KK. Surgical treatment of levodopa-induced dyskinesia in Parkinson's disease. Ann Indian Acad Neurol. (2017) 20:199–206. doi: 10.4103/aian.AIAN_244_17
Keywords: Parkinson's disease, deep brain stimulation, subthalamic nucleus, globus pallidus interna, dyskinesia
Citation: Liu Y, Li F, Luo H, He Q, Chen L, Cheng Y, Zhang W and Xie Z (2019) Improvement of Deep Brain Stimulation in Dyskinesia in Parkinson's Disease: A Meta-Analysis. Front. Neurol. 10:151. doi: 10.3389/fneur.2019.00151
Received: 04 December 2018; Accepted: 05 February 2019;
Published: 25 February 2019.
Edited by:
Antonio Pisani, University of Rome Tor Vergata, ItalyReviewed by:
Santiago Perez-Lloret, Consejo Nacional de Investigaciones Científicas y Técnicas (CONICET), ArgentinaAngel Sesar, Complejo Hospitalario Universitario de Santiago, Spain
Copyright © 2019 Liu, Li, Luo, He, Chen, Cheng, Zhang and Xie. This is an open-access article distributed under the terms of the Creative Commons Attribution License (CC BY). The use, distribution or reproduction in other forums is permitted, provided the original author(s) and the copyright owner(s) are credited and that the original publication in this journal is cited, in accordance with accepted academic practice. No use, distribution or reproduction is permitted which does not comply with these terms.
*Correspondence: Wenbin Zhang, wenbinzhang@njmu.edu.cn
Zongyi Xie, zyxieneuro2013@yahoo.com