- 1Radiology Department of the Second Affiliated Hospital and Yuying Children's Hospital, Wenzhou Medical University, Wenzhou, China
- 2The Key Laboratory of Biomedical Information Engineering, Ministry of Education, Department of Biomedical Engineering, School of Life Science and Technology, Xi' an Jiaotong University, Xi' an, China
- 3Neurosurgery Department of the Second Affiliated Hospital and Yuying Children's Hospital of Wenzhou Medical University, Wenzhou, China
The main objective of this study was to evaluate sex differences in cortical thickness after acute mild traumatic brain injury (mTBI) and its associations with clinical outcomes. Thirty-two patients with mTBI at acute phase (2.4 ± 1.3 days post-injury) and 30 healthy controls were enrolled. All the participants underwent comprehensive neurocognitive assessments and MRI to assess cortical thickness. Significant sex differences were determined by using variance analysis of factorial design. Relations between the cortical thickness and clinical assessments were measured with the Spearman Correlation. Results revealed that patients with mTBI had significantly reduced cortical thickness in the left entorhinal cortex while increased cortical thickness in the left precuneus cortex and right lateral occipital cortex, compared with healthy controls. The interaction effect of the group × sex on cortical thickness was significant. Female patients had significant thicker cortical thickness in the left caudal anterior cingulate cortex (ACC) than male patients and had higher scores on Posttraumatic stress disorder Checklist—Civilian Version (PCL-C). Spearman correlational analysis showed a significantly positive correlations between the cortical thickness of the left caudal ACC and PCL-C ratings in female patients. Sex differences in cortical thickness support its potential as a neuroimaging phenotype for investigating the differences in clinical profiles of mild TBI between women and men.
Introduction
Traumatic brain injury (TBI) is an important global health issue, of which 75–90% are classified as mild TBI (mTBI) (1). Although most patients with mTBI become asymptomatic within days to weeks, some develop a series of persistent symptoms that have been called as “persistent post-concussive syndrome.” There are several factors associated with those various outcomes, but one of the most controversial and interesting factors is sex (2). Sex differences in outcomes after mTBI have been addressed in lots of studies, of which some found females have a poorer outcome than males (3–8). Another study have found that females have a higher risk of developing epilepsy, suicide, and use more health care services and males have a higher risk for schizophrenia after mTBI (9). While other studies have shown no substantial difference in outcome with regard to sex (10, 11). The effect of sex on outcome after mTBI is less clear. Observation studies may be confounded by many factors, including different symptoms reporting among women and men (12, 7). Therefore, a more objective measurement, such as imaging findings, is crucial in avoiding such bias. Understanding sex differences of brain injury mechanism after mild TBI may change the future diagnostic work-up in patients with mTBI and lead to separate management strategies for patients of different sex.
Sex differences in brain activities after TBI have been reported in recent years. An fMRI study in veterans with TBI reveals that males showed increased functional connectivity between the left orbitofrontal cortex (OFC) and the right mid frontal cortex compared with females. In the meantime, a significant negative association is found on the overall score on the Buss Perry Aggression Questionnaire with functional connectivity between the left OFC and left angular region in male veterans (13). Diffusion tensor imaging (DTI) shows that male patients with mTBI have decreased fractional anisotropy (FA) in the uncinate fasciculus (UF) compared with female patients and was negatively correlated with time to symptom resolution (14). However, there is lack of findings on the cortical thickness alternations following mild TBI. Cortical thickness is an intrinsic biological parameter and should be independent of external factors such as the MRI scanner type, imaging sequence, spatial resolution and/or field strength (15, 16). Sex differences in cortical thickness have been well-documented in healthy adults (17). Cortical thickness alternations are also reported in the chronic phase of various TBI (from vary mild, moderate to more serve TBI) (18–20), there is no study focused on the sex differences in cortical thickness following acute mTBI. Moreover, gender difference in outcomes, favoring females as endogenous neuroprotectants, has been documented in TBI. However, a consistent finding in the research literature on general traumatic experience is that women exhibit twice the rate of the disorder as men, in spite of men experiencing greater lifetime exposure to traumatic events overall (21–23). We hypothesized that there was a significant interaction effect of gender and diagnosis in the traumatic complaints and associated cortical thickness alternations following mTBI.
Materials and Methods
Participants
All consecutive patients with non-contrast head CT due to acute head trauma enrolling from the local emergency department (ED) formed the initial population. Inclusion criteria for all mTBI patients were based on the World Health Organization's Collaborating Center for Neurotrauma Task Force (24): (i) Glasgow Coma Scale (GCS) score of 13–15, (ii) one or more/any of the following: loss of consciousness (LOC) for < 30 min, posttraumatic amnesia (PTA) for 24 or less hours, and/or other transient neurological abnormalities such as focal signs, seizure, and intracranial lesions not requiring surgery, (iii) within 1 week after onset of a mTBI, (iv) were aged 16 years or older. Mild TBI patients were excluded for: (1) a history of a previous brain injury, neurological disease, long-standing psychiatric condition, concurrent substance, or alcohol abuse, (2) a structural abnormality on neuroimaging (CT and MRI), (3) intubation and/or presence of a skull fracture and administration of sedatives, (4) the manifestation of mTBI due to medications by other injuries (e.g., systemic injuries, facial injuries, or spinal cord injury), (5) other problems (e.g., psychological trauma, language barrier, or coexisting medical conditions), (6) caused by penetrating craniocerebral injury.
Thirty-two patients with mTBI (18 males) and 30 sex-, age-, and education-matched healthy controls (14 males) without neurologic impairment or psychiatric disorders participated in the study. Participants were all right-handed according to the Edinburgh Handedness Inventory (25). All the subjects gave written, informed consent in person approved by a local institutional review board and conducted in accordance with the Declaration of Helsinki.
Image Acquisition
A non-contrast CT scan was performed on all consecutive patients following acute head injury with a 64-row CT scanner (GE, Lightspeed VCT). All the patients with mTBI went through the MRI scans the day they were recruited in the group. The MRI scans were acquired with the use of 3T MRI scanner (GE 750). A custom-built head holder was used to prevent head movements. The MRI protocol involved the high-resolution T1-weighted 3D BRAVO sequence (echo time = 3.4 ms, repetition time = 7.7 ms, flip angle = 9°, slice thickness = 1 mm, field of view = 256 × 256 mm, matrix size = 256 × 256). The presence of focal lesions and cerebral microbleeds was independently determined by experienced clinical neuroradiologists (with 9 and 10 years' experience) who assessed multiple modalities of neuroimaging data acquired at baseline (T1-flair, T2-flair, T2, susceptibility weighted imaging). Any disagreement between these two observers was resolved by consensus. None of patients were with visible contusion lesions using conventional neuroimaging techniques or exhibited cerebral micro-bleeds on SWI.
Clinical Assessments
Clinical assessments were performed within 48 h of MR imaging for all the participants. Based on these previous publications, a limited set of neuropsychological tests were analyzed in the current study, to reduce multiple testing issues. This selection was based on our previous work demonstrating sensitivity to TBI-related alterations to brain structure (26, 27). The following tests were assessed: (i) Trail-Making Test Part A and Digit Symbol coding score from the Wechsler Adult Intelligence Scale III (WAIS-III) to examine cognitive information processing speed (28); (ii) Forward Digit Span and Backward Digit Span from the WAIS-III to assess immediate auditory span, working memory, and executive function (29); (iii) Verbal Fluency Test to assess verbal fluency including language ability, semantic memory and executive function (30); (iv) Posttraumatic stress disorder Checklist—Civilian Version (31). In addition, post concussive symptoms (PCS) were measured with the Rivermead Post-Concussion Symptom Questionnaire (RPCS) (32).
Cortical Thickness Analyses
We used FreeSurfer version 5.3.0 (33) (https://surfer.nmr.mgh.harvard.edu/fswiki) to extract surface-based features from the high-resolution T1-weighted images. The reliability of obtaining cortical thickness measurements from MRI scans has been well validated (15, 34, 35). The high- resolution T1-weighted MR volume for each participant was bias corrected, skull stripped, and segmented into white matter, gray matter, and cerebrospinal fluid before surface-based morphometry (15). Then, we conducted to tessellate the gray-white boundary, perform automated topology correction, and perform surface deformation to locate the gray-white and gray-pial boundaries (36, 37). Cortical thickness was calculated as the closet distance between the gray-white matter boundary and the pial mesh at each vertex on the tessellated surface (37). The surface was then anatomically parcellated by using the Desikan-Killiany atlas into 66 structures (33 structures for each hemisphere) (38). Accuracy for automated processing was inspected by an expert (with 4 years of experience in editing data from more than 200 examinations and trained in this field) and manual corrections were applied if necessary.
We mapped these structures onto a spherical space to achieve point-to-point correspondence for each subject (39). The final segmentation of surface-based labeling was based on both a subject-independent probabilistic atlas and on subject-specific measured values. Combining the cortical-thickness map and surface-based labels, we computed the average cortical thickness for each region.
Statistical Analysis
The Shapiro–Wilk W-test was used to test for normality distribution of all continuous variables. The independent two-sample t-test and the Mann–Whitney test were used to compare group differences based on data normality, respectively. Chi-square analyses were applied to assess categorical variables. P < 0.05 were considered to indicate a significant difference. Effect sizes (Cohen's d) were computed to demonstrate the magnitude of observed differences. Two-sample t-tests were used to explore cortical thickness differences between groups from each native-surface region of interest (ROIs), and results were assessed for significance after controlling the false-discovery rate (FDR) at < 0.05 to correct for multiple comparisons. The 2 × 2 (Group × Sex) mixed measures ANOVAs were performed to test the interactions and group effects, respectively. P < 0.05 were considered to indicate a significant difference. Simple effect was restricted to the ROIs showing significant interaction effect of group and sex. All regional results were Bonferroni-corrected by a factor of number of ROIs (N) showing significant interaction effect, corresponding to a corrected α of P < 0.05/N after controlling for age and education level. Spearman's correlations were conducted between clinical assessments and the region-of-interest variables, since the data were not normally distributed.
Results
Demographic and Clinical Characteristics
Thirty-seven patients with mTBI participated in this study. Of which, data from five patients were excluded because of poor MR imaging quality (n = 3), and excessive head motions (n = 2). Finally, 32 patients (18 male) were included. Thirty matched healthy controls (14 male) were also recruited. No significant difference was showed between patients with mTBI and healthy controls regarding age and education level. The average age was 33.4 years (range 14–54 years) in healthy controls, and was 31.0 years (range 13–59 years) in patients with mTBI [F(1, 60) = 0.62, P = 0.43, Cohen' s d = −0.20]. The average education level was 11.8 years (range 1–18 years) in healthy controls, and was 9.6 years (range 1–16 years) in patients with mTBI [F(1, 60) = 2.88, P = 0.1, Cohen' s d = −0.43]. No significant difference was in sex (χ2 = 0.57, P = 0.45). No significant difference was found in age and education level among the four groups (i.e., male and female controls and male and female patients with mTBI) [for age: F(3, 58) = 1.98, P = 0.13; for education level: F(3, 58) = 1.32, P = 0.27]. A detailed demographic data and clinical characteristics were summarized in the Tables 1, 2. The major mechanism of trauma was a motor vehicle accident (11 of 18 male patients [61%], 10 of 14 female patients [71%]), followed by an assault (two of 18 male patients [11%], two of 14 female patients [14%]) (P = 0.4).
ANCOVAs (Group × Sex) analysis on the clinical assessments were conducted. The interaction effect of the group × sex was significant for the PCL-C [F(1, 58) = 5.99, P = 0.017] with simple effect testing suggested that females presented more complaints in the PCL-C compared with male counterparts only in the patient group but not in the control group (P = 0.001 after Bonferroni-correction) (Table 2). For patients, more complaints on the PCL-C was presented in both female (P < 0.001) and male (P = 0.012) compared with their corresponding controls.
Cortical Thickness Results
Patients with mTBI presented prominently reduced cortical thickness than healthy controls in the left entorhinal cortex, while significantly increased cortical thickness in the left precuneus cortex and right lateral occipital cortex (P < 0.05, FDR corrected, Figure 1). The interaction effect of the group × sex on cortical thickness was significant in the left caudal anterior cingulate cortex (caudal ACC) [F(1, 57) = 5.976, P = 0.018], fusiform cortex [F(1, 57) = 10.13, P = 0.002], insula cortex [F(1, 57) = 7.35, P = 0.009] and superior frontal cortex (SFC) [F(1, 57) = 5.131, P = 0.027] (Figure 2). Simple effect testing indicated that female patients had significant increased cortical thickness than male patients in the left caudal ACC (P = 0.004). Increased cortical thickness in the left SFC and fusiform cortex (all for P = 0.005) were also presented in female controls compared with male controls. Female patients had non-significant tendency of increased cortical thickness than male patients in the insula cortex (P = 0.036). Other comparison did not obtain the significance.
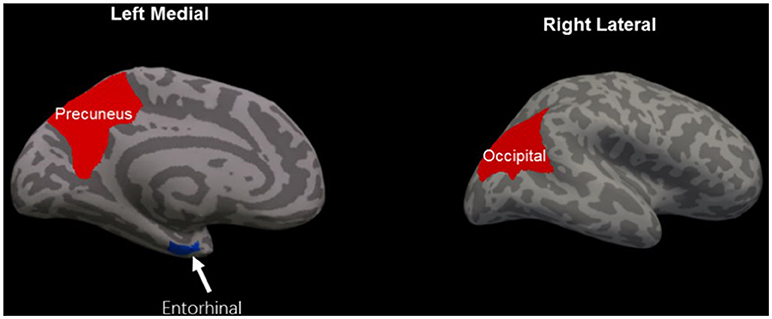
Figure 1. Regions of significant cortical thinning (blue) and thickening (red) in mild traumatic brain injury patients, compared with healthy controls. Regions in the left entorhinal cortex, left precuneus cortex, and right lateral occipital cortex were significant at P < 0.05 after FDR correction.
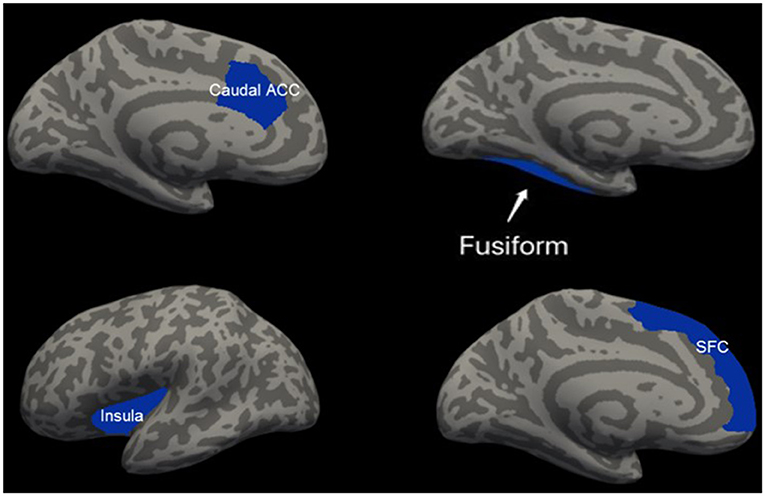
Figure 2. Regions of significant increased cortical thickness (blue) in female participants, compared with male participants. Left column represents in patients with mTBI and right column represents in healthy controls. Regions in the caudal anterior cingulate cortex (caudal ACC), fusiform cortex and superior frontal cortex (SFC) in the left hemisphere were significant at P < 0.0125 after Bonferroni-correction. Region in the left insula cortex was not significant at P = 0.036 after Bonferroni-correction.
Correlation Analysis Results
Spearman correlation analysis was only restricted into clinical assessment (PCL-C) and regional cortical variable showing a significant interaction effect. There was a significantly positive correlations between the cortical thickness of the left caudal ACC and PCL-C ratings only in female patients (r = 0.594, P = 0.011). No other correlation was presented in either female, male patients or whole patient group.
Discussion
To our knowledge, this is the first study to examine sex differences in cortical thickness during the very early acute post-injury of mTBI. The present study indicated a salient modulatory effect of sex on both self-reported symptomatology (PCL-C) and regional cortical thickness following acute mTBI. Female patients had significant increased cortical thickness than male patients in the left caudal ACC. The increased cortical thickness in the left caudal ACC was positively related with more complaints in the PCL-C ratings only in female patients. These findings may provide the clue to the management strategies for mTBI patients of different sexes.
Previous studies about sex differences in outcomes of mTBI revealed confounded results (4–6, 11, 13). A prior research suggested that it may be easier to admit their concussion symptoms after TBI for female athletes than male (12). Similarly, male concussion athletes presented more difficult to have a willing to report their symptoms than female athletes because of social norms (40) and the pressure to return to sports (41). Outcome differences in patients with mTBI could be masked by the subjective assessments. Our study avoids it by evaluating an objective measurement of the underlying neuroimaging detected injury using brain cortical thickness. To date, sex differences in cortical thickness have been reported in healthy individuals in several researches though no such findings were reported in mTBI (41). We found that the female healthy controls had significant increased cortical thickness than male controls in the left fusiform cortex, while this difference disappears modulated by the mild TBI (42). In addition, interaction effect of group and sex in the cortical thickness were primarily located in the four regions, including the left caudal ACC, fusiform cortex, insula cortex, and SFC. Based on these findings, sex difference in cortical thickness may be modulated by the injury.
We observed that the cortical thickness of female patients in the left caudal anterior cingulate cortex was positively related with the PCL-C scores. Previous work has shown that caudal anterior cingulate cortex is involved in motor control (43). For instance, it was reported that post-traumatic stress disorder (PTSD) is an anxiety disorder associated with the anterior cingulate cortex (ACC) (44). Results exhibited that decreased functional connectivity was observed between the caudal ACC and the precentral gyrus in veterans with PTSD compared to healthy controls. So we supposed that the thickened cortical thickness in left caudal anterior cingulate cortex may affect functional connectivity in female survivors. This possibility needs to be tested by future study to focus on dynamic structural and functional changes after mTBI. We have also found non-significant trend of thicker thickness in the left insula in the female patients, compared with male patients. Simple effect testing suggested that female patients had significant increased cortical thickness in this region than female controls that didn't found in the male groups (P = 0.016) after Bonferroni-correction. Such finding may be limited by the relatively small sample sizes. So we still speculated that it may due to the significant increased cortical thickness in female patients than male patients, compared with respective controls.
We did not explore the mechanisms that may contribute to cortical thickening in the current study, but several possibilities in this context can be considered. In animal studies, regional micro-edema has been found in thickened cortical regions within a day after cortical impact (45). Gray matter changes were in the form of increased, not decreased cortical thickness, which may have resulted from neuroinflammatory or other trophic process related to endocrine changes or functional compensation (46). Acute cerebral inflammatory reactions have been found to recover within months after injury and animal studies revealed thickened cortical regions became thinning over days with reduction of micro-edema (45). Unfortunately, we still need to conform such changes in cortical thickness after mTBI in a more chronical follow-up.
There are several limitations to our study. Post-injury time may be not long enough to observe cortical changing at acute phase and longitudinal analysis needs to be involved using following-up data. Furthermore, we did not evaluate the heterogeneity of injury, future studies should use additional outcome measures, including diffusion tensor imaging for structure integrity, resting state functional connectivity study for dynamic changes in functional networks, perfusion of cerebral blood flow (CBF) for brain metabolism using Arterial Spin Labeling Technology (ASL), which may be helpful in understanding the underlying pathophysiology and causes of sex differences in mTBI. Considering the selection of control may influence the detected injury pattern following mTBI, further study needs to enroll both orthopedically-injured patients and healthy subjects as different control group for comparison.
Conclusions
In conclusion, the study presented the abnormal cortical thickness changes related to sex in patients with mTBI, which correlated with the more possibility to develop PTSD and impairments in the information processing speed. Thus, our results indicated a role for cortical thickness as a metric for evaluating the sex difference of mTBI injuries and may predicting subsequent clinical outcome.
Author Contributions
MS, LB, ZY, BY, and GB contributed to the conception of the study. MS, JC, and LB contributed significantly to analysis and manuscript preparation. MS, JC, LB, and SW performed the data analyses and wrote the manuscript. WH, SW, CS, SG, LY, DZ, CG, and LH helped perform the analysis with constructive discussions.
Funding
This research was supported by the Natural Science Foundation of China under Grant Nos. 81571752 and 81771914, the National key research and development plan of China, 2016YFC0100300, the Natural Science Foundation of Zhejiang Province (nos. LY16H180009 and LY15H090016).
Conflict of Interest Statement
The authors declare that the research was conducted in the absence of any commercial or financial relationships that could be construed as a potential conflict of interest.
References
1. Mondello S, Schmid K, Berger RP, Kobeissy F, Italiano D, Jeromin A, et al. The challenge of mild traumatic brain injury: role of biochemical markers in diagnosis of brain damage. Med Res Rev. (2014) 34:503–31. doi: 10.1002/med.21295
2. Laker SR. Epidemiology of concussion and mild traumatic brain injury. PM R (2011) 3(10 Suppl. 2), S354–8. doi: 10.1016/j.pmrj.2011.07.017
3. Kraus JF, Peek-Asa C, McArthur D. The independent effect of gender on outcomes following traumatic brain injury: a preliminary investigation. Neurosurg Focus (2000) 8:e5. doi: 10.3171/foc.2000.8.1.156
4. Broshek DK, Kaushik T, Freeman JR, Erlanger D, Webbe F, Barth JT. Sex differences in outcome following sports-related concussion. J Neurosurg. (2005) 102:856–63. doi: 10.3171/jns.2005.102.5.0856
5. Preiss-Farzanegan SJ, Chapman B, Wong TM, Wu J, Bazarian JJ. The relationship between gender and postconcussion symptoms after sport-related mild traumatic brain injury. PmR (2009) 1:245–53. doi: 10.1016/j.pmrj.2009.01.011
6. Bazarian JJ, Blyth B, Mookerjee S, He H, McDermott MP. Sex differences in outcome after mild traumatic brain injury. J Neurotrauma (2010) 27:527–39. doi: 10.1089/neu.2009.1068
7. Covassin T, Bay E. Are there gender differences in cognitive function, chronic stress, and neurobehavioral symptoms after mild-to-moderate traumatic brain injury? J Neurosci Nurs. (2012) 44:124–33. doi: 10.1097/JNN.0b013e318252737d
8. Hsu HL, Chen DY, Tseng YC, Kuo YS, Huang YL, Chiu WT, et al. Sex differences in working memory after mild traumatic brain injury: a functional MR imaging study. Radiology (2015) 276:828–35. doi: 10.1148/radiol.2015142549
9. Cancelliere C, Donovan J, Cassidy JD. Is sex an indicator of prognosis after mild traumatic brain injury: a systematic analysis of the findings of the world health organization collaborating centre task force on mild traumatic brain injury and the international collaboration on mild traumatic brain injury prognosis. Arch Phys Med Rehabil. (2016) 97(2 Suppl.):S5–18. doi: 10.1016/j.apmr.2014.11.028
10. Cantu RC, Guskiewicz K, Register-Mihalik JK. A retrospective clinical analysis of moderate to severe athletic concussions. PM R (2010) 2:1088–93. doi: 10.1016/j.pmrj.2010.07.483
11. Frommer LJ, Gurka KK, Cross KM, Ingersoll CD, Comstock RD, Saliba SA. Sex differences in concussion symptoms of high school athletes. J Athl Train (2011) 46:76–84. doi: 10.4085/1062-6050-46.1.76
12. Farace E, Alves WM. Do women fare worse? A metaanalysis of gender differences in outcome after traumatic brain injury. Neurosurg Focus (2000) 8:e6. doi: 10.3171/foc.2000.8.1.152
13. McGlade E, Rogowska J, Yurgelun-Todd D. Sex differences in orbitofrontal connectivity in male and female veterans with TBI. Brain Imaging Behav. (2015) 9:535–49. doi: 10.1007/s11682-015-9379-3
14. Fakhran S, Yaeger K, Collins M, Alhilali L. Sex differences in white matter abnormalities after mild traumatic brain injury: localization and correlation with outcome. Radiology (2014) 272:815–23. doi: 10.1148/radiol.14132512
15. Han X, Jovicich J, Salat D, van der Kouwe A, Quinn B, Czanner S, et al. Reliability of MRI-derived measurements of human cerebral cortical thickness: the effects of field strength, scanner upgrade and manufacturer. Neuroimage (2006) 32:180–94. doi: 10.1016/j.neuroimage.2006.02.051
16. Wonderlick JS, Ziegler DA, Hosseini-Varnamkhasti P, Locascio JJ, Bakkour A, van der Kouwe A, et al. Reliability of MRI-derived cortical and subcortical morphometric measures: effects of pulse sequence, voxel geometry, and parallel imaging. Neuroimage (2009) 44:1324–33. doi: 10.1016/j.neuroimage.2008.10.037
17. Sowell ER, Peterson BS, Kan E, Woods RP, Yoshii J, Bansal R, et al. Sex differences in cortical thickness mapped in 176 healthy individuals between 7 and 87 years of age. Cereb Cortex (2007) 17:1550–60. doi: 10.1093/cercor/bhl066
18. Wilde EA, Merkley TL, Bigler ED, Max JE, Schmidt AT, Ayoub KW, et al. Longitudinal changes in cortical thickness in children after traumatic brain injury and their relation to behavioral regulation and emotional control. Int J Dev Neurosci. (2012) 30:267–76. doi: 10.1016/j.ijdevneu.2012.01.003
19. Palacios EM, Sala-Llonch R, Junque C, Fernandez-Espejo D, Roig T, Tormos JM, et al. Long-term declarative memory deficits in diffuse TBI: correlations with cortical thickness, white matter integrity and hippocampal volume. Cortex (2013) 49:646–57. doi: 10.1016/j.cortex.2012.02.011
20. Michael AP, Stout J, Roskos PT, Bolzenius J, Gfeller J, Mogul D, et al. Evaluation of cortical thickness after traumatic brain injury in military veterans. J Neurotrauma (2015) 32:1751–8. doi: 10.1089/neu.2015.3918
21. Breslau N, Chilcoat HD, Kessler RC, Davis GC. Previous exposure to trauma and PTSD effects of subsequent trauma: results from the Detroit Area Survey of Trauma. Am J Psychiatry (1999) 156:902–7. doi: 10.1176/ajp.156.6.902
22. Breslau N. Gender differences in trauma and posttraumatic stress disorder. J Gend Specif Med. (2002) 5:34–40.
23. Nemeroff CB, Bremner JD, Foa EB, Mayberg HS, North CS, Stein MB. Posttraumatic stress disorder: a state-of-the-science review. J Psychiatr Res. (2006) 40:1–21. doi: 10.1016/j.jpsychires.2005.07.005
24. Holm L, Cassidy JD, Carroll LJ, Borg J, Neurotrauma Task Force on Mild Traumatic Brain Injury of the WHO Collaborating Centre. Summary of the WHO collaborating centre for neurotrauma task force on mild traumatic brain injury. J Rehabil Med. (2005) 37:137–41. doi: 10.1080/16501970510027321
26. Kinnunen KM, Greenwood R, Powell JH, Leech R, Hawkins PC, Bonnelle V, et al. White matter damage and cognitive impairment after traumatic brain injury. Brain (2011) 134:449–63. doi: 10.1093/brain/awq347
27. Fagerholm ED, Hellyer PJ, Scott G, Leech R, Sharp DJ. Disconnection of network hubs and cognitive impairment after traumatic brain injury. Brain (2015) 138:1696–709. doi: 10.1093/brain/awv075
28. Arnett JA, Labovitz SS. Effect of physical layout in performance of the trail making test. Psychol Assess. (1995) 7:220–1. doi: 10.1037/1040-3590.7.2.220
29. Harman-Smith YE, Mathias JL, Bowden SC, Rosenfeld JV, Bigler ED. Wechsler Adult Intelligence Scale-Third Edition profiles and their relationship to self-reported outcome following traumatic brain injury. J Clin Exp Neuropsychol. (2013) 35:785–98. doi: 10.1080/13803395.2013.824554
30. Joy S, Kaplan E, Fein D. Speed and memory in the WAIS-III Digit Symbol–Coding subtest across the adult lifespan. Arch Clin Neuropsychol. (2004) 19:759–67. doi: 10.1016/j.acn.2003.09.009
31. Ruggiero KJ, Del Ben K, Scotti JR, Rabalais AE. Psychometric properties of the PTSD Checklist-Civilian Version. J Trauma Stress (2003) 16:495–502. doi: 10.1023/A:1025714729117
32. King NS, Crawford S, Wenden FJ, Moss NEG, Wade DT. The rivermead post concussion symptoms questionnaire - a measure of symptoms commonly experienced after head-injury and its reliability. J Neurol. (1995) 242:587–92. doi: 10.1007/BF00868811
33. Fischl B, Sereno MI, Dale AM. Cortical surface-based analysis - II: inflation, flattening, and a surface-based coordinate system. Neuroimage (1999) 9:195–207. doi: 10.1006/nimg.1998.0396
34. Dickerson BC, Fenstermacher E, Salat DH, Wolk DA, Maguire RP, Desikan R, et al. Detection of cortical thickness correlates of cognitive performance: reliability across MRI scan sessions, scanners, and field strengths. Neuroimage (2008) 39:10–8. doi: 10.1016/j.neuroimage.2007.08.042
35. Jovicich J, Czanner S, Han X, Salat D, van der Kouwe A, Quinn B, et al. MRI-derived measurements of human subcortical, ventricular and intracranial brain volumes: reliability effects of scan sessions, acquisition sequences, data analyses, scanner upgrade, scanner vendors and field strengths. Neuroimage (2009) 46:177–92. doi: 10.1016/j.neuroimage.2009.02.010
36. Dale AM, Fischl B, Sereno MI. Cortical surface-based analysis. I Segmentation and surface reconstruction. Neuroimage (1999) 9:179–94. doi: 10.1006/nimg.1998.0395
37. Fischl B, Dale AM. Measuring the thickness of the human cerebral cortex from magnetic resonance images. Proc Natl Acad Sci USA. (2000) 97:11050–5. doi: 10.1073/pnas.200033797
38. Desikan RS, Segonne F, Fischl B, Quinn BT, Dickerson BC, Blacker D, et al. An automated labeling system for subdividing the human cerebral cortex on MRI scans into gyral based regions of interest. Neuroimage (2006) 31:968–80. doi: 10.1016/j.neuroimage.2006.01.021
39. Fischl B, van der Kouwe A, Destrieux C, Halgren E, Segonne F, Salat DH, et al. Automatically parcellating the human cerebral cortex. Cereb Cortex (2004) 14:11–22. doi: 10.1093/cercor/bhg087
40. Covassin T, Elbin RJ. The female athlete: the role of gender in the assessment and management of sport-related concussion. Clin Sports Med. (2011) 30:125–31. doi: 10.1016/j.csm.2010.08.001
41. Colvin AC, Mullen J, Lovell MR, West RV, Collins MW, Groh M. The role of concussion history and gender in recovery from soccer-related concussion. Am J Sports Med. (2009) 37:1699–704. doi: 10.1177/0363546509332497
42. Im K, Lee JM, Lee J, Shin YW, Kim IY, Kwon JS, et al. Gender difference analysis of cortical thickness in healthy young adults with surface-based methods. Neuroimage (2006) 31:31–8. doi: 10.1016/j.neuroimage.2005.11.042
43. Dum RP, Strick PL. The origin of corticospinal projections from the premotor areas in the frontal lobe. J Neurosci. (1991) 11:667–89. doi: 10.1523/JNEUROSCI.11-03-00667.1991
44. Kennis M, Rademaker AR, van Rooij SJ, Kahn RS, Geuze E. Resting state functional connectivity of the anterior cingulate cortex in veterans with and without post-traumatic stress disorder. Hum Brain Mapp. (2015) 36:99–109. doi: 10.1002/hbm.22615
45. Lewen A, Fredriksson A, Li GL, Olsson Y, Hillered L. Behavioural and morphological outcome of mild cortical contusion trauma of the rat brain: influence of NMDA-receptor blockade. Acta Neurochir. (1999) 141:193–202. doi: 10.1007/s007010050286
Keywords: mild traumatic brain injury, cortical thickness, gender difference, interaction effect, clinical outcomes
Citation: Shao M, Cao J, Bai L, Huang W, Wang S, Sun C, Gan S, Ye L, Yin B, Zhang D, Gu C, Hu L, Bai G and Yan Z (2018) Preliminary Evidence of Sex Differences in Cortical Thickness Following Acute Mild Traumatic Brain Injury. Front. Neurol. 9:878. doi: 10.3389/fneur.2018.00878
Received: 11 June 2018; Accepted: 27 September 2018;
Published: 17 October 2018.
Edited by:
Nada Andelic, Oslo University Hospital, NorwayReviewed by:
Paul Perrin, Virginia Commonwealth University, United StatesRao P. Gullapalli, University of Maryland, United States
Copyright © 2018 Shao, Cao, Bai, Huang, Wang, Sun, Gan, Ye, Yin, Zhang, Gu, Hu, Bai and Yan. This is an open-access article distributed under the terms of the Creative Commons Attribution License (CC BY). The use, distribution or reproduction in other forums is permitted, provided the original author(s) and the copyright owner(s) are credited and that the original publication in this journal is cited, in accordance with accepted academic practice. No use, distribution or reproduction is permitted which does not comply with these terms.
*Correspondence: Guanghui Bai, YmdodTc5QDEyNi5jb20=
Zhihan Yan, emhpaGFueWFud3pAMTYzLmNvbQ==
†These authors have contributed equally to this work