- 1Department of Neurology, Raúl Carrea Institute for Neurological Research (FLENI), Buenos Aires, Argentina
- 2Department of Diagnostic Imaging, Raúl Carrea Institute for Neurological Research (FLENI), Buenos Aires, Argentina
- 3Center for Research on Neuroimmunological Diseases (CIEN), Raúl Carrea Institute for Neurological Research (FLENI), Buenos Aires, Argentina
- 4Department of Neurology, Partners Multiple Sclerosis Center, Brigham and Women's Hospital, Harvard Medical School, Boston, MA, United States
- 5Laboratory for Neuroimaging Research, Departments of Neurology and Radiology, Brigham and Women's Hospital, Boston, MA, United States
- 6Ann Romney Center for Neurologic Diseases, Department of Neurology, Brigham and Women's Hospital, Harvard Medical School, Boston, MA, United States
- 7The Broad Institute of MIT and Harvard, Cambridge, MA, United States
Background: In MS patients, hypertension is associated with a delayed diagnosis and an increased risk of progression. Understanding the mechanisms of this association could potentially lead to improved prevention of disease progression. We aimed to establish whether high blood pressure contributes to white-matter injury and brain atrophy in MS.
Methods: Cross-sectional study of 95 patients with RRMS. Estimates of fractional anisotropy, gray-matter volume and lesion load were obtained from 3T MRI. We used fractional anisotropy voxel-based statistics to establish the effect of blood pressure on white matter tracts. Additionally, we used voxel-based morphometry (VBM) to study the effect on gray matter integrity.
Results: Only 29.5% had normal blood pressure levels, with 52.6% suffering from prehypertension and 17.9% with hypertension. Increasing systolic blood pressure was associated with damage to posterior white-matter tracts as well as greater levels of gray matter atrophy, in particular in the frontal cortex. Age-adjusted linear regression indicated that neither lesion volume (β = 0.002, 95%CI: 0.02–0.02; p = 0.85) or lesion number (β = −0.004, 95%CI: 0.03–0.02; p = 0.74) were associated with systolic blood pressure.
Conclusions: Prehypertension and hypertension are frequent in MS. Increased blood pressure is related to white- and gray-matter integrity, both related to MS disability outcomes. These findings suggest attention to the control of blood pressure in MS patients.
Introduction
Multiple sclerosis (MS) is a chronic autoimmune disease characterized by the destruction of myelin and other antigens in the central nervous system (CNS) (1). Brain, spinal cord, and optic nerve lesions arise from the infiltration of autoreactive mononuclear peripheral blood cells (1). Lesions are typically located in the white matter (WM) of periventricular, juxtacortical, infratentorial, and spinal cord areas of the CNS (2). Additionally, gray matter (GM) lesions and brain atrophy are observed commonly during the course of the disease (3). Brain atrophy reflects tissue loss and represents a global measure of both severe demyelination and axonal loss in MS (3). The pathogenesis and course of the disease are thought to result from complex interactions between genetic and environmental factors (4, 5). Of note, substantial heterogeneity exists in disease severity and several comorbidities have been proposed that may account for some of the observed differences in disease course among individuals (6).
Recently, epidemiological findings suggest a relationship between MS and several cardiovascular comorbidities, including obesity (7), insulin resistance (7, 8), dyslipidemia (9), and hypertension (8, 10); the latter being the most frequent cardiovascular comorbidity reported in MS (11). In chronic medical conditions, cardiovascular comorbidities are associated with decreased quality of life and increased mortality (11). In MS in particular, these comorbidities are associated with a delayed diagnosis, worse magnetic resonance imaging (MRI) outcomes (12), and increased risk of disease progression (13). For example, a direct relationship between cardiovascular risk factors and clinical status as measured by the Expanded Disability Status Scale (EDSS) has been reported (14). Furthermore, higher low-density lipoprotein (LDL) cholesterol and total cholesterol levels were associated with inflammatory MRI measures (9). Additionally, a large MS cohort study reported that hypertension and heart disease were associated with brain atrophy and that obesity, was associated with T1 lesion volumes (12). Finally, we recently reported that a high sodium intake, a regulating factor of blood pressure (BP), is linked to MS disease activity (15). Understanding the distribution and mechanisms of cardiovascular comorbidities in MS could then potentially lead to better management of patients and improved diagnosis and prevention of disease progression.
Despite the strong epidemiological evidence, there is a paucity of studies exploring pathophysiological mechanisms to explain the association between MS and cardiovascular comorbidities. In particular, the mechanisms linking MS severity and elevated BP are poorly understood. Hypertension is associated with both cerebrovascular and cardiovascular diseases and is the greatest risk factor for mortality in those conditions (16). Moreover, elevated systolic BP has been previously linked to brain atrophy, WM injury and increased blood-brain barrier permeability in a range of conditions other than MS (17–19).
We thus hypothesize that elevated BP contributes to MS progression by affecting WM and GM integrity. To address this question, we evaluated the relationship between BP and a sensitive brain MRI measure of structural WM change (fractional anisotropy-FA) in a cross-sectional cohort of patients with MS. We additionally tested for an association between elevated BP and MS cerebral lesion load as well as GM atrophy. Establishing whether BP contributes to structural brain changes in MS would highlight the potential relevance of aggressive screening and management of hypertension.
Methods
Study Design and Patients
This study was carried out in accordance with the recommendations of the Ethics guidelines of the Raúl Carrea Institute for Neurological Research Ethics Committee. The protocol was approved by the Raúl Carrea Institute for Neurological Research Ethics Committee. All subjects gave written informed consent in accordance with the Declaration of Helsinki. One hundred consecutive patients with RRMS according to the 2010 International Panel criteria (2) who were seen at the MS clinic of the Raúl Carrea Institute for Neurological Research were recruited from November 2013 to July 2015. Patients needed to be 18 years old or older to be included. We excluded pregnant women and patients with a previous history of other neurological disorders that may affect MRI assessment. We assessed the patient's current medications, with particular attention to antihypertensive agents.
Blood Pressure Measurement
Blood pressure was measured in one session at the time of enrollment. Classification of BP for adults was based on the 7th Report of the Joint National Committee on prevention, detection, evaluation, and treatment of high BP (16). Categories were as follows: Normal (systolic-SBP < 120 and diastolic-DBP < 80), Prehypertension (SBP 120-139 or DBP 80-89), Stage 1 hypertension (SBP 140-159 or DBP 90-99) and Stage 2 hypertension (SBP ≥ 160 or ≥ 100). Due to our patients' age range we expected none or few patients in Stage 2 hypertension; therefore, we used a pooled hypertension group by collapsing Stage 1 and 2. After the finalization and image analysis of our study, a new set of recommendations were published: the 8th Report of the Joint National Committee on prevention, detection, evaluation, and treatment of high BP (20) and later the 2017 ACC/AHA/AAPA/ABC/ACPM/AGS/APhA/ASH/ASPC/NMA/PCNA Guideline for the Prevention, Detection, Evaluation, and Management of High Blood Pressure in Adults (21). In this guideline, BP was categorized as normal (< 120/80 mm Hg), elevated (120–129/ < 80 mm Hg), stage 1 hypertension (130–139/80–89 mm Hg), or stage 2 hypertension (≥140/90 mm Hg). Since the majority of the analysis were done with lineal uncategorized values of BP, the update on guidelines definition did not modify our original analysis.
For BP recordings, patients were requested to sit in an empty office for at least 5 min in a chair with their feet on the floor and arm supported at the level of the heart. BP measurement was delayed if the patient smoked, consumed caffeine, or practiced exercise within 30 min of measurement. The BP measurement was made by trained non-medical personnel to avoid the “white coat effect.” An automatic BP monitor was used (Microlife WatchBP Office Blood Pressure Monitor, Microlife, Switzerland) following these steps: first, the dominant arm was identified, then three measurements separated by 60 s intervals were made and the average of those results were considered as the final value.
Image Acquisition and Analysis
Brain MRI images were acquired on a Signa HDxt 3T scanner (General Electric, Milwaukee, IL) using an 8-channel head coil. Both 3-dimensional (3D) T1-weighted and FLAIR pulse sequences were used to measure lesion load and brain volumes. We acquired a sagittal 3D fast spoiled gradient-echo T1-weighted sequence (512 × 512 matrix; field of view = 25 cm; slice thickness = 1.1 mm; TR = 7,400 ms; TE = 2,400; TI = 450 ms; flip angle = 15°) and isotropic 3D FLAIR (512 × 512 matrix; field of view = 25 cm; slice thickness = 1 mm; TR = 8,200 ms; TE = 136 ms; TI = 2,200 ms).
Lesions were segmented by the fully automated lesion growth algorithm (22) as implemented in the LST toolbox version 2.0.15 (https://www.applied-statistics.de/lst.html) for SPM12. This algorithm is able to segment T2-hyperintense lesions from a combination of T1 and FLAIR images. It first segments the T1 image into the three main tissue classes [cerebrospinal fluid (CSF), GM, and WM]. This information is then combined with the FLAIR intensities in order to calculate lesion maps. Using this method, lesion number and volumes was estimated.
In addition to this, estimated lesion masks were then automatically filled using an internal filling method where candidate region voxels where replaced by random intensities from a Gaussian distribution generated from the normal-appearing WM intensities and then filtered to reintroduce the original spatial variation in WM (23). We filled MS lesions in order to increase the accuracy of brain volumes estimates as previously described (24).
For brain volumes, we processed the T1-weighted images using the CAT12 toolbox (http://www.neuro.uni-jena.de) in SPM12 (http://www.fil.ion.ucl.ac.uk/spm) running MATLAB 8.5.0. Brain parenchymal fraction, WM fraction, and GM fraction were calculated as previously described (25). These global volumes were used for descriptive purposes and not considered as main predictor variables.
We acquired diffusion tensor imaging (DTI) data using the following parameters: 55 gradient directions, b-value 1,000 s/mm2, 256 × 256 matrix; field of view = 24 cm; slice thickness = 3.5 mm; in plane voxel size (pixel size) = 0.71 × 0.78 mm, TR = 10,000 ms; TE = 88.7 ms. To estimate the impact of BP in WM integrity, we performed FA voxel-based statistics using the ACID Toolbox written in MATLAB (version R2016b, Mathworks, USA), as previously described (26). DTI data were visually inspected and found to be free of artifacts using the residual error map of the tensor fit to detect outliers. The DTI data were corrected for motion and eddy current effects using ECMOCO from the ACID Toolbox. All resulting maps were of good quality. FA values were generated from the pre-processed DTI data. The default settings of the SPM12 normalization software were used for each registration approach. The b0 image was first co-registered using an affine transformation to the standard SPM12 EPI template. The same transformation was subsequently applied to the corresponding FA image and to the DW images. Finally, images were smoothed prior to model specification and analysis.
To estimate differences in regional brain volume according to BP, a voxel-based morphometry (VBM) approach was used (27), a technique previously used to estimate WM and GM atrophy in MS patients (28, 29). First, lesion-filled 3D T1-weighted images were segmented in GM, WM and CSF, as described above. Then, GM and WM segmented images of all subjects, were used to produce GM and WM templates and drive the deformation to the templates. At each iteration, the deformations, calculated using the DARTEL registration method (30), were applied to GM and WM, with an increasingly good alignment of subject morphology, to produce templates. Spatially normalized images were then modulated to ensure that the overall amount of each tissue class was not altered by the spatial normalization procedure. To better align the final template with the Montreal Neurologic Institute (MNI) space, an affine registration between the customized GM template and the statistical parametric mapping GM template (in the MNI space) was also calculated. The same transformation was applied to the WM customized template. The images were then smoothed with an 8 mm full width at half maximum (FWHM) Gaussian kernel. Finally, we overlaid the T maps with the probabilistic fiber map included in SPM12 to provide a post-hoc description of the area of the GM significant voxels.
Statistical Analysis
We aimed to establish if systolic BP (SBP) was associated with increased WM injury (as measured by FA, primary outcome). Our secondary outcomes were to assess if SBP was related to lesion load and regional GM volumes. For our primary outcomes, we used FA measures in a linear model as the dependent variable and the following covariates: SBP, age, smoking status (smoker, non-smoker), gender, hypertension treatment (treatment vs. no treatment), MS disease-modifying treatment, vitamin D level (continuous). The T maps obtained for the covariates were assessed for significance with threshold-free cluster enhancement with an α of 0.001.
To achieve our secondary analysis, we used a linear regression with log-transformed WM lesion volume as the dependent variable and continuous SBP as the independent variable, adjusting for the same co-variates as above. In exploratory analysis, only systolic BP (our variable of interest) and age demonstrated significance to be included in our model, and all other variables were discarded after assessing for confounding and effect modification. The same analysis was also carried out for lesion number. For GM volumes, a linear model was generated after VBM and T maps were analyzed as mentioned above.
P < 0.05 were considered significant. Unless otherwise noted, mean ± standard deviation is reported. All statistical procedures were performed with Stata version 12.1 (Statacorp).
Results
Prevalence of Elevated Blood Pressure
One hundred patients were initially recruited in our study. Two patients later refused BP recordings and 3 patients cancelled MRI. Thus, 95 patients were finally included, 57 of whom were female (60%). Patients were aged 19-66 (median 32, IQR 32-45). Regarding BP, 28 (29%) were normal, 50 (53%) had pre-hypertension, and 17 (18%) had hypertension. According to the 2017 guidelines, 29% were normal, 18% had elevated BP, and 53% were hypertense. Patient characteristics are shown in Table 1 (including a breakdown according to BP levels).
Elevated Blood Pressure and White-Matter Integrity
To establish the impact of SBP on WM integrity, we performed a voxel-based regression analysis with measures of FA as the dependent variables and SBP as the main predictor. As shown in Figure 1 and Table 2, SBP was independently associated with bilateral decreased FA in voxels located in the precuneus, and middle and posterior cingulate gyrus (Figure 1 and Table 2). Thus, high levels of SBP were associated with reduced integrity of WM.
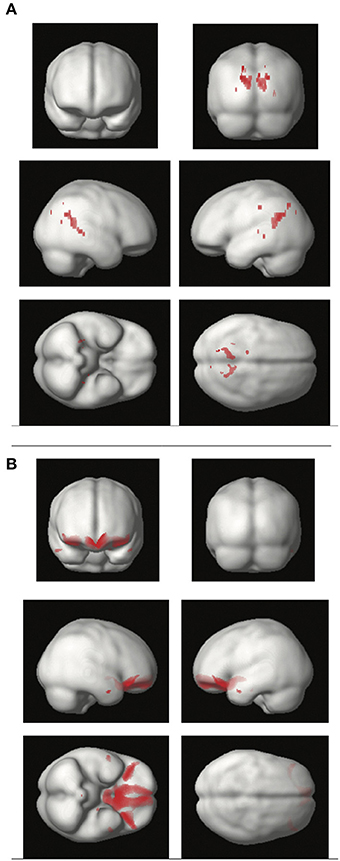
Figure 1. Regions of the cerebral white matter (A) and gray matter (B) in which systolic blood pressure is inversely associated with tissue integrity. (A) Brain render depicting white matter areas associated with decreased fractional anisotropy. Voxel-based regression included fractional anisotropy as the dependent variable and systolic blood pressure as the independent variable. Age, gender, and smoking were added as covariates. (B) Brain render showing gray matter areas with reduced volume associated with increasing systolic blood pressure. Voxel-based morphometry included gray matter as the dependent variable, with systolic blood pressure as the independent variable. Age, gender, smoking, and total intracranial volume were covariates.
Elevated Blood Pressure and Brain Atrophy
As shown in Figure 1 and Table 2, SBP was inversely associated with regional VBM-derived GM volumes bilaterally in the posterior orbital gyrus, the medial frontal cortex and the subcallosal area. Thus, SBP was associated with brain atrophy in frontal areas.
Relationship Between White-Matter Areas Affected by SBP and Disability
To test whether WM areas found to be affected by SBP had an impact in disability, we performed an ordinal regression analysis with EDSS score as the dependent variable and measures of FA as the predictors after adjustment by age, gender and smoking. We found a positive correlation between FA values in the left and right cingulate gyrus (P = 0.04 and P = 0.013, respectively). We did not detect a significant association between areas in which greater atrophy linked to higher BP and EDSS. Thus, in our cohort, only WM areas linked to SBP contributed to MS disability as measured by EDSS.
Blood Pressure and Lesion Size or Number
Elevated BP can have a significant impact on WM damage and is associated with the appearance of WM hyperintensities in locations similar to MS (17). Thus, we hypothesized that elevated BP may contribute to WM lesion size or number.
We built a linear model with lesion volume as the dependent variable and age and systolic BP as the predictors. Age-adjusted linear regression indicated that neither lesion volume (β = 0.002, 95% CI: −0.02 to 0.02; p = 0.85) nor lesion number (β = −0.004, 95% CI: −0.03 to 0.02; p = 0.74) was associated with systolic BP. Thus, BP was not related to overt MS lesions.
Discussion
In patients with RRMS, increasing SBP was associated with WM injury and greater brain atrophy. Although no direct comparison to healthy controls was made, our results indicate that elevated SBP is frequent in MS. Moreover, damage in some areas of WM affected by SBP correlated with higher physical disability on the EDSS.
Extensive work has been done in healthy controls to establish the impact of SBP and other vascular factors in brain aging (17, 31). In those studies, a clear association between SBP and WM injury and brain atrophy has been established. Although, initially, the findings referred to a link between SBP and WM hyperintensities, damage was later linked to more subtle measures of WM integrity such as FA, even in mid-life individuals, such as the cohort included in our study (17). Our work shows that raised SBP contributes to WM damage and brain atrophy in young and mid-life MS patients. A recent MS cohort study addressing the impact of cardiovascular factors (12), did not detected a significant impact in WM by hypertension, but found a similar impact on cortical atrophy. This discrepancy on WM results might be explained by the more sensitive (FA) method used in our study, of particular relevance in young cohorts.
A wide range of biological factors are thought to contribute to clinical and MRI outcomes in patients with MS, creating a complex disease pathophysiology (1). Previous studies show that high sodium intake is associated with greater clinical and MRI activity in both MS animal models and in patients with the disease (15, 32). The mechanisms of this association remain largely unknown. High sodium intake is also associated with hypertension, which, in turn, is one of the leading causes of cerebrovascular disease and mortality worldwide (16). Thus, in addition to the immunological mechanisms previously described linking sodium to MS pathophysiology (32, 33), high sodium intake could also affect MS severity indirectly through increases in SBP. One can consider possible direct mechanisms explaining the relationship between elevated BP and MS such as subtle myelin injury and increased blood brain barrier (BBB) permeability. Microvascular damage due to hypertension can increase arterial stiffness and generate decreased oxygen loads, with subsequent chronic myelin injury (34). This mechanism however does not explain the differential affection of posterior WM tracts and anterior GM. Of interest, although other tractography and VBM studies in MS patients have found similar areas affected (28), studies in healthy controls show that SBP impacts more on anterior WM tracts. This may suggest a differential interaction between SBP and autoimmunity. The second potential mechanism relates to increases in BBB permeability due to elevated SBP. Previous reports in animal models suggest that raises in SBP increase BBB leakage, and may thus facilitate the entry of immune cells and other inflammatory mediators into the brain parenchyma (19). In addition to this, Angiotensin II, a key component of the renin-angiotensin system (RAS) can also enter the brain during hypertension and influence the immune system through the activation of Angiotensin type 1 receptor (AT1R) in astrocytes and microglia (35), of great relevance to MS progression (36). A final potential mechanism could be that hypertension leads to small vessel occlusive disease and therefore to hypoperfusion and subtle brain damage (37). This fails to explain, however, the lack of association between SBP and lesion load, but could account for additional chronic damage to the brain relevant to MS progression.
Regardless of the potential mechanisms, comorbidities are an increasing concern for MS practitioners. Recently, an international workshop on comorbidities in MS was held (6). In a meta-analysis, the panel identified hypertension as one of the most frequent comorbidities in MS. In line with previous reports, they highlighted that hypertension, as well as other comorbidities, may be associated with worse outcomes. Moreover, they generated recommendations for future research, including exploring the mechanisms of the effects of comorbidity on MS as a means of identifying potential approaches to mitigating their impact. Our study goes along with this recommendation by identifying structural correlates of the effect of hypertension on cerebral MS related pathology, raising the possibility that elevated BP may contribute to disease progression.
There are several limitations of our study worthy of comment. First, our sample consisted of a cohort of white Hispanics from a single center, and may not be generalizable to the worldwide MS population. Second, although every consecutive patient was invited, participation was voluntary, and healthier, less disabled patients were more likely to accept invitation. This selection bias may have been negligible due to the relative young age and low disability of our cohort. Third, we did not include a comparison healthy control cohort, which may have shed some more information regarding the differential affection in MS and healthy controls. Further studies are required to compare both groups. The estimated prevalence in Argentina of prehypertension and hypertension is 35 and 12.2%, respectively, for a similar age group (38). Therefore, it appears that hypertension is more prevalent in MS. However, because blood pressure and hypertension were measured and defined differently, we were very cautious to avoid any misleading comparison. Fourth, we studied our patients in a cross-sectional fashion; longitudinal studies are required to further elucidate the long-term impact of BP on clinical and MRI-defined MS progression. Despite these limitations, our study has a clear impact: there is an unmet need to address the early detection and treatment of hypertension in patients with MS. Furthermore, additional research is warranted to fully characterize the role of hypertension in contributing to the pathophysiology of MS.
In conclusion, our study shows that prehypertension and hypertension are common among patients with MS. Moreover, elevated BP is associated with reduced brain integrity. Since WM and GM integrity are associated with disability outcomes during disease progression, it is of importance to avoid additional factors injuring the brain. These findings further suggest the importance of aggressive, early management of hypertension as a preventive strategy, not only for cardiovascular and cerebrovascular events, but also potentially, to limit MS disease progression. Our results, suggests that MS practitioners should measure and aim for optimum control of BP.
Disclosures
RB has received consulting fees from EMD Serono, Genentech, Sanofi-Genzyme, and Novartis and research support from Biogen, EMD-Serono, Novartis, and Sanofi-Genzyme. FQ serves on the editorial board for Systems Biomedicine, Inmunologia, American Journal of Clinical and Experimental Immunology, is an associate editor for Immunology (UK), is an advisory board member for Seminars in Immunopathology; received research support from Harvard Medical School, BADERC, NMSS. JC is a board member of Merck-Serono Argentina,Novartis Argentina,Genzyme LATAM,Genzyme global, Biogen-Idec LATAM, and Merck-Serono LATAM. JC has received reimbursement for developing educational presentations for Merck-Serono Argentina, Merck- Serono LATAM, Biogen-Idec Argentina, Genzyme Argentina, Novartis Argentina, Novartis LATAM, Novartis Global, and TEVA Argentina as well as professional travel/accommodations stipends. MF has received travel accommodations from Teva, Merck-Serono, Biogen-Idec and Novartis. Dr. Farez has also received research funds from Biogen-Idec and Novartis Argentina.
Author Contributions
DD, HC, EH, SR, and FV: acquisition of data, analysis, and interpretation. RB, FQ, and JC: critical revision of the manuscript for important intellectual content. MF: study concept and design, data analysis and interpretation, manuscript writing, study supervision.
Funding
This study was funded by Novartis Argentina and the Center for Research on Neuroimmunological Diseases (CIEN), Raúl Carrea Institute for Neurological Research (FLENI).
Conflict of Interest Statement
The authors declare that the research was conducted in the absence of any commercial or financial relationships that could be construed as a potential conflict of interest.
References
1. Sospedra M, Martin R. Immunology of multiple sclerosis. Annu Rev Immunol. (2005) 23:683–747. doi: 10.1146/annurev.immunol.23.021704.115707
2. Polman CH, Reingold SC, Banwell B, Clanet M, Cohen JA, Filippi M, et al. Diagnostic criteria for multiple sclerosis: 2010 revisions to the McDonald criteria. Ann Neurol. (2011) 69:292–302. doi: 10.1002/ana.22366
3. Mahad DH, Trapp BD, Lassmann H. Pathological mechanisms in progressive multiple sclerosis. Lancet Neurol. (2015) 14:183–93. doi: 10.1016/S1474-4422(14)70256-X
4. Farez MF, Mascanfroni ID, Méndez-Huergo SP, Yeste A, Murugaiyan G, Garo LP, et al. Melatonin contributes to the seasonality of multiple sclerosis relapses. Cell (2015) 162:1338–52. doi: 10.1016/j.cell.2015.08.025
5. Handel AE, Giovannoni G, Ebers GC, Ramagopalan S V. Environmental factors and their timing in adult-onset multiple sclerosis. Nat Rev Neurol. (2010) 6:156–66. doi: 10.1038/nrneurol.2010.1
6. Marrie RA, Miller A, Sormani MP, Thompson A, Waubant E, Trojano M, et al. Recommendations for observational studies of comorbidity in multiple sclerosis. Neurology (2016) 86:1446–53. doi: 10.1212/WNL.0000000000002474
7. Oliveira SR, Colado Simão AN, Kallaur AP, Delicato de Almeida ER, Morimoto HK, Lopes J, et al. Disability in patients with multiple sclerosis: influence of insulin resistance, adiposity, and oxidative stress. Nutrition (2014) 30:268–73. doi: 10.1016/j.nut.2013.08.001
8. Marrie RA, Rudick R, Horwitz R, Cutter G, Tyry T, Campagnolo D, et al. Vascular comorbidity is associated with more rapid disability progression in multiple sclerosis. Neurology (2010) 74:1041–7. doi: 10.1212/WNL.0b013e3181d6b125
9. Weinstock-Guttman B, Zivadinov R, Horakova D, Havrdova E, Qu J, Shyh G, et al. Lipid profiles are associated with lesion formation over 24 months in interferon-β treated patients following the first demyelinating event. J Neurol Neurosurg Psychiatry (2013) 84:1186–91. doi: 10.1136/jnnp-2012-304740
10. Dagan A, Gringouz I, Kliers I, Segal G. Disability progression in multiple sclerosis is affected by the emergence of comorbid arterial hypertension. J Clin Neurol. (2016) 12:345. doi: 10.3988/jcn.2016.12.3.345
11. Marrie RA, Patten SB, Tremlett H, Wolfson C, Warren S, Svenson LW, et al. Sex differences in comorbidity at diagnosis of multiple sclerosis. Neurology (2016) 86:1279–86. doi: 10.1212/WNL.0000000000002481
12. Kappus N, Weinstock-Guttman B, Hagemeier J, Kennedy C, Melia R, Carl E, et al. Cardiovascular risk factors are associated with increased lesion burden and brain atrophy in multiple sclerosis. J Neurol Neurosurg Psychiatry (2016) 87:181–7. doi: 10.1136/jnnp-2014-310051
13. Marrie RA, Horwitz R, Cutter G, Tyry T, Campagnolo D, Vollmer T. Comorbidity delays diagnosis and increases disability at diagnosis in MS. Neurology (2009) 72:117–24. doi: 10.1212/01.wnl.0000333252.78173.5f
14. Moccia M, Lanzillo R, Palladino R, Maniscalco GT, De Rosa A, Russo C, et al. The Framingham cardiovascular risk score in multiple sclerosis. Eur J Neurol. (2015) 22:1176–83. doi: 10.1111/ene.12720
15. Farez MF, Fiol MP, Gaitán MI, Quintana FJ, Correale J. Sodium intake is associated with increased disease activity in multiple sclerosis. J Neurol Neurosurg Psychiatry (2015) 86:26–31. doi: 10.1136/jnnp-2014-307928
16. Chobanian A V, Bakris GL, Black HR, Cushman WC, Green LA, Izzo JL, et al. Seventh report of the joint national committee on prevention, detection, evaluation, and treatment of high blood pressure. Hypertension (2003) 42:1206–52. doi: 10.1161/01.HYP.0000107251.49515.c2
17. Maillard P, Seshadri S, Beiser A, Himali JJ, Au R, Fletcher E, et al. Effects of systolic blood pressure on white-matter integrity in young adults in the Framingham Heart Study: a cross-sectional study. Lancet Neurol. (2012) 11:1039–47 doi: 10.1016/S1474-4422(12)70241-7
18. CD, Murphy DGM, Tranh M, Grady CL, Haxby JV, Gillette JA, et al. The effect of white matter hyperintensity volume on brain structure, cognitive performance, and cerebral metabolism of glucose in 51 healthy adults. Neurology (1995)45:2077–84.
19. Biancardi VC, Son SJ, Ahmadi S, Filosa JA, Stern JE. Circulating angiotensin II gains access to the hypothalamus and brain stem during hypertension via breakdown of the blood-brain barrier. Hypertension (2014) 63:572–9. doi: 10.1161/HYPERTENSIONAHA.113.01743
20. James PA, Oparil S, Carter BL, Cushman WC, Dennison-Himmelfarb C, Handler J, et al. 2014 Evidence-based guideline for the management of high blood pressure in adults. JAMA (2014) 311:507. doi: 10.1001/jama.2013.284427
21. Whelton PK, Carey RM, Aronow WS, Casey DE, Collins KJ, Dennison Himmelfarb C, et al. 2017 ACC/AHA/AAPA/ABC/ACPM/AGS/APhA/ASH/ASPC/NMA/PCNA Guideline for the prevention, detection, evaluation, and management of high blood pressure in adults: a report of the American College of Cardiology/American Heart Association Task Force on Clinical Practice Guidelines. Hypertension (2018) 71:1269–324. doi: 10.1161/HYP.0000000000000066
22. Schmidt P, Gaser C, Arsic M, Buck D, Förschler A, Berthele A, et al. An automated tool for detection of FLAIR-hyperintense white-matter lesions in Multiple Sclerosis. Neuroimage (2012) 59:3774–83. doi: 10.1016/j.neuroimage.2011.11.032
23. Valverde S, Oliver A, Roura E, Pareto D, Vilanova JC, Lluís R-T, et al. Quantifying brain tissue volume in multiple sclerosis with automated lesion segmentation and filling. NeuroImage Clin. (2015) 9:640–7. doi: 10.1016/j.nicl.2015.10.012
24. Valverde S, Oliver A, Lladó X. A white matter lesion-filling approach to improve brain tissue volume measurements. Neuroimage Clin. (2014) 6:86–92. doi: 10.1016/j.nicl.2014.08.016
25. Dell'Oglio E, Ceccarelli A, Glanz BI, Healy BC, Tauhid S, Arora A, et al. Quantification of global cerebral atrophy in multiple sclerosis from 3T MRI using SPM: the role of misclassification errors. J Neuroimaging (2015) 25:191–9. doi: 10.1111/jon.12194
26. Mohammadi S, Keller SS, Glauche V, Kugel H, Jansen A, Hutton C, et al. The influence of spatial registration on detection of cerebral asymmetries using voxel-based statistics of fractional anisotropy images and TBSS. PLoS ONE (2012) 7:e36851. doi: 10.1371/journal.pone.0036851
27. Whitwell JL. Voxel-based morphometry: an automated technique for assessing structural changes in the brain. J Neurosci. (2009) 29:9661–4. doi: 10.1523/JNEUROSCI.2160-09.2009
28. Riccitelli G, Rocca MA, Pagani E, Martinelli V, Radaelli M, Falini A, et al. Mapping regional grey and white matter atrophy in relapsing–remitting multiple sclerosis. Mult Scler J. (2012) 18:1027–37. doi: 10.1177/1352458512439239
29. Prinster A, Quarantelli M, Lanzillo R, Orefice G, Vacca G, Carotenuto B, et al. A voxel-based morphometry study of disease severity correlates in relapsing– remitting multiple sclerosis. Mult Scler J. (2010) 16:45–54. doi: 10.1177/1352458509351896
30. Ashburner J. A fast diffeomorphic image registration algorithm. Neuroimage (2007) 38:95–113. doi: 10.1016/j.neuroimage.2007.07.007
31. Jeerakathil T, Wolf PA, Beiser A, Massaro J, Seshadri S, D'Agostino RB, et al. Stroke risk profile predicts white matter hyperintensity volume: the Framingham Study. Stroke (2004) 35:1857–61. doi: 10.1161/01.STR.0000135226.53499.85
32. Wu C, Yosef N, Thalhamer T, Zhu C, Xiao S, Kishi Y, et al. Induction of pathogenic TH17 cells by inducible salt-sensing kinase SGK1. Nature (2013) 496:513–7. doi: 10.1038/nature11984
33. Kleinewietfeld M, Manzel A, Titze J, Kvakan H, Yosef N, Linker RA, et al. Sodium chloride drives autoimmune disease by the induction of pathogenic TH17 cells. Nature (2013) 496:518–22. doi: 10.1038/nature11868
34. Knopman DS, Mosley TH, Catellier DJ, Sharrett AR. Cardiovascular risk factors and cerebral atrophy in a middle-aged cohort. Neurology (2005) 65:876–81. doi: 10.1212/01.wnl.0000176074.09733.a8
35. Lanz T V, Ding Z, Ho PP, Luo J, Agrawal AN, Srinagesh H, et al. Angiotensin II sustains brain inflammation in mice via TGF-β. J Clin Invest. (2010) 120:2782–94. doi: 10.1172/JCI41709
36. Farez MF, Quintana FJ, Gandhi R, Izquierdo G, Lucas M, Weiner HL. Toll-like receptor 2 and poly(ADP-ribose) polymerase 1 promote central nervous system neuroinflammation in progressive EAE. Nat Immunol. (2009) 10:958–64. doi: 10.1038/ni.1775
37. D'haeseleer M, Hostenbach S, Peeters I, Sankari S El, Nagels G, De Keyser J, et al. Cerebral hypoperfusion: a new pathophysiologic concept in multiple sclerosis? J Cereb Blood Flow Metab. (2015) 35:1406–10. doi: 10.1038/jcbfm.2015.131
Keywords: hypertension, systolic blood pressure, multiple sclerosis, white-matter integrity, fractional anisotrophy
Citation: Dossi DE, Chaves H, Heck ES, Rodriguez Murúa S, Ventrice F, Bakshi R, Quintana FJ, Correale J and Farez MF (2018) Effects of Systolic Blood Pressure on Brain Integrity in Multiple Sclerosis. Front. Neurol. 9:487. doi: 10.3389/fneur.2018.00487
Received: 08 March 2018; Accepted: 05 June 2018;
Published: 25 June 2018.
Edited by:
Scott S. Zamvil, University of California, San Francisco, United StatesReviewed by:
Bruno Gran, Nottingham University Hospitals NHS Trust, United KingdomHans Lassmann, Center for Brain Research, Austria
Copyright © 2018 Dossi, Chaves, Heck, Rodriguez Murúa, Ventrice, Bakshi, Quintana, Correale and Farez. This is an open-access article distributed under the terms of the Creative Commons Attribution License (CC BY). The use, distribution or reproduction in other forums is permitted, provided the original author(s) and the copyright owner are credited and that the original publication in this journal is cited, in accordance with accepted academic practice. No use, distribution or reproduction is permitted which does not comply with these terms.
*Correspondence: Mauricio F. Farez, mfarez@fleni.org.ar
†These authors have contributed equally to this work.