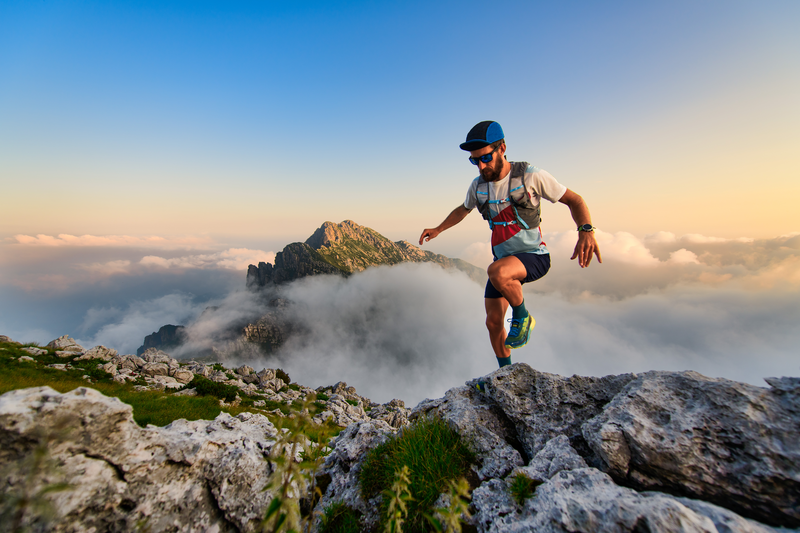
94% of researchers rate our articles as excellent or good
Learn more about the work of our research integrity team to safeguard the quality of each article we publish.
Find out more
CLINICAL STUDY PROTOCOL article
Front. Neurol. , 07 June 2018
Sec. Stroke
Volume 9 - 2018 | https://doi.org/10.3389/fneur.2018.00198
Background: There is increasing interest in stem cell therapy as another treatment modality in stroke, particularly for patients who are unable to receive endovascular clot retrieval or thrombolysis therapies, or for whom standard treatment has failed. We have recently shown that human amniotic epithelial cells (hAECs) are effective in reducing infarct volume in different animal models of ischemic stroke, including in non-human primates. hAEC therapy attenuated infarct growth and/or promoted functional recovery, even when administered 1–3 days after the onset of stroke.
Methods: We now propose an open label Phase 1 dose escalation trial to assess the safety of allogeneic hAECs in stroke patients with a view to providing an evidence platform for future Phase 2 efficacy trials. We propose a modified 3 + 3 dose escalation study design with additional components for measuring magnetic resonance signal of efficacy as well as the effect of hAECs on immunosuppression after stroke.
Result: The trial will commence in 2018. The findings will be published in a peer-reviewed journal.
Conclusion: The trial is registered with ANZCTR (ACTRN12618000076279p).
Prior to 2015, the standard clinical approach for reducing disability and death in ischemic stroke was Stroke Unit admission, antiplatelet therapy, and treatment with recombinant tissue plasminogen activator (TPA), within 4.5 h from onset in selected patients (1). However, the rate of vessel re-opening when there is a large obstructive clot is low (12%), even with TPA (2). Since 2015, there has been considerable optimism about the place of endovascular clot retrieval (ECR), for large clot removal, as a new and transformative therapy (1). However, even with this extended time window (up to 24 h), the number of eligible patients for ECR is less than 15% (1, 3). Further, a substantial proportion of those patients in the trial who received these treatments was left with moderate to severe disability or died (72% of patients who received TPA and 48% of those who received TPA and ECR) (2). In short, there remain enormous challenges in achieving the effective management of stroke across all patients. There is thus an urgent need to explore alternative therapeutic strategies that may be both suitable for more stroke patients and be more effective in improving outcome after stroke.
One such strategy is to move from the current time-based approach to an imaging tissue-based approach where treatment is based on the presence of salvageable ischemic tissue (3, 4). However, reperfusion therapies shift the peri-infarct zone toward the ischemic core but do not address the local and systemic immune response cascades that separate from the ischemic insult itself, promoting further tissue damage surrounding the infarct (5). The body’s intended effect of post-stroke immunosuppression appears to be the dampening of the “autoaggressive” Th1 immune response within the brain (6), but the unintended consequence is an increased risk of infection such as pneumonia (7), and this phenomenon is associated with high mortality and poor functional outcomes. These infiltrating inflammatory cells are located in the peri-infarct area, which has been referred to as the “inflammatory penumbra,” which denotes an area susceptible to further injury from the inflammatory cascade (5). Recognition of the need to manage the inflammatory response as part of stroke therapy has led to a call for future therapies that also focus on mitigating injurious events in the peri-infarct zone and not just on reperfusion therapy (5). In this regard, stem cell therapy has emerged as one potentially attractive option because of its ability to modulate inflammatory pathways at multiple sites appropriate to the changing pathophysiological state over time (8). By contrast, an example of a unimodal neuroprotectant drug target that would be unsuitable for modulating the inflammatory cascade is matrix metalloproteinase (MMP-9). In the early phase of stroke, MMP-9 damages the blood–brain barrier (BBB) (9) and contributes to vasogenic edema, whereas in later stages, it contributes to remodeling around the peri-infarct areas, and consequently targeting MMP-9 at this time can be harmful (5).
The amniotic membrane is the inner layer of the fetal sac and consists of epithelium, basement membrane, and stroma. Amnion epithelial cells are therefore adult cells that express surface markers common to those of embryonic stem cells, primordial germ cells, and somatic stem cells including neural crest cells (10). Human amniotic epithelial cells (hAECs) express nonpolymorphic, nonclassical human leukocyte antigen (HLA) G (11) and have “low” immunogenicity (12) due to their role in protecting the fetus from immune rejection. There are various mechanisms by which hAECs might exert therapeutic effects following stroke. First, hAECs could secrete neurotrophic factors that promote the recovery of damaged cells in the penumbra. Such factors could also promote synaptogenesis to re-innervate lost connections. Second, hAECs could differentiate into a neuronal phenotype and replace damaged or dead cells. Third, hAECs could act as “biological minipumps” within the central nervous system, secreting necessary cytokines, growth factors, hormones, and/or neurotransmitters to restore cellular function. Fourth, hAECs could improve stroke outcome by dampening of the innate and adaptive immune systems (suppression of pro-inflammatory cytokines and secretion of factors that inhibit chemotactic activity of neutrophils and macrophages) that contributes to brain injury, which would include protection of neurons from immune cell-mediated apoptosis (10, 13). Lastly, systemically administered hAECs could attenuate the severity of post-stroke immunosuppression involving marked leukopenia and atrophy of secondary lymphoid tissues. Figure 1 provides a schematic of the potential effects of hAECs on post-stroke inflammation and immunosuppression.
We have evaluated the utility of an intravenous route for hAEC therapy in a series of experiments involving different animal models of stroke (C57Bl6 mice and marmoset monkeys; both sexes) (14). These experiments showed that hAECs can reduce infarct volume and functional deficit when given acutely or as long as 3 days after stroke onset (14). hAECs were detected in the brain (infarct) and spleen of animals subjected to stroke, but not in sham-operated animals that received hAEC (14), indicating that hAEC migrate to tissues impacted by stroke. Key effects at the peri-infarct zone and infarct core occurred via reductions in (1) apoptosis, (2) inflammation (i.e., reduced expression of inflammasome protein, NLRP3 at 24 h and dampened entry of neutrophils, CD8+ T cells, B cells, and pro-inflammatory macrophages into the infarct by 72 h), and (3) post-stroke immunosuppression (14). Over 8 weeks, there was a decreased spread of gliosis into the adjacent border in hAEC-treated mice. Further, long-term functional recovery was still augmented in either young or aged mice of both sexes even if hAEC administration was delayed until 1 or 3 days post stroke (14).
We have designed an open label Phase 1 dose escalation trial of hAECs in patients with ischemic stroke with the primary aim of determining the maximal tolerated dose (MTD). Assessment of adverse events will be undertaken blinded to cell dose. Fifteen patients will be recruited for a dose escalation trial in 2018. The final follow-up for the 15th patient in the hAEC arm will be done 12 months after cell delivery. The trial will be submitted to the Monash Health Human Research Ethics Committee.
Patients are eligible if (1) they have ischemic stroke in the territory of the large main artery (middle cerebral artery) (15), (2) present within 24 h of stroke onset and are not eligible for TPA or clot retrieval, (3) age is between 18 and 85 years old, (4) have National Institute of Health Stroke Scale/NIHSS (tool used in clinical trials for measuring stroke severity) between 6 and 15, and (5) patient or person responsible (on behalf of participant) must sign consent form after explanation of the trial.
Patients are excluded if (1) eligible for TPA and/or ECR; (2) there is evidence of autoimmune disease, organ transplant, malignancy, splenectomized individuals, or have infection at the time of stroke; (3) neurodegenerative disease such as dementia or Parkinson’s disease; (4) pregnancy; (5) have contraindications for magnetic resonance (MR) imaging such as pacemaker; (6) patients with initial infarct [on diffusion weighted imaging (DWI)] volume of ≤5 or >100 mL will also be excluded (14, 16). This is a common strategy used in many studies to exclude infarcts with very small volume (such as lacunar infarct); very large infarcts are excluded as these patients have a high probability of developing malignant middle cerebral artery infarct and death; (7) patients with mild stroke (NIHSS <6) or very severe stroke (NIHSS >15); (8) blood sugar level of ≤3 or ≥20 mmol/L; and (9) significant lung disease requiring oxygen.
Treatment doses are of stage-wise dose escalation: 2, 4, 8, 16, and 32 million cells/kg.
Patients will be given hAECs by intravenous infusion for 1 h, previously shown to be safe in preterm babies. A recent study with multipotent adult progenitor cells showed that 1,200 million cells were infused up for 1 h (17). The maximal dose used in neural stem cell trial was 20 million cells/kg given by direct intracerebral injection (18).
This trial is designed as a balance between finding the MTD and finding the effective dose (19). The dose escalation and de-escalation stages are performed similar to the classic 3 + 3 scheme in a Phase 1 trial whereby the sample size is increased in small increments of 3. For a 5-dose escalation, the estimated sample size is 15. The Bayesian Optimal Interval Design (BOIN) (20) used here weighs the dose escalation by the lower boundary of the target toxicity rate (safety rate = 0.6 × target) and de-escalation by the upper boundary (toxicity rate = 1.4 × target). This method recommends setting the default target rate at 0.3 rather than a lower value because of the small sample size typical for Phase 1 trial (20). This default is higher than 90-day mortality of 15.7% for patients at the Monash Medical Centre with NIHSS between 6 and 15. The map of this approach is provided in Figure 2 and Table 1. The rows show that the decision for escalating or de-escalating the dose can be performed by counting the number of patients with the observed toxicity.
Descriptive statistics will be used to describe patient demographics, safety, and SAE of hAECs. The dose–response curve for estimating the MTD is performed using the BOIN package (20). This method computes the probability of toxicity (>0.3) given data base on the list of the number of patients treated at each dose and the number of toxicity at each dose. The MTD is denoted by a marked rise in the probability of toxicity from baseline.
The DSMB will be composed of stroke physicians with experience in clinical trials. Similar to other stem cell trial, after the last subject in cohort 1 (dose 2 million cells/kg) has been observed for 7 days after infusion, the DSMB will evaluate the dose-limiting toxicity (DLT) and other safety information for the eight subjects to determine whether it is safe to proceed to cohort 2.
The Cell Therapies and Regenerative Medicine Platform (CTRMP) at the Monash Health Translation Precinct has a cryobank of isolated, screened, and stored hAECs for clinical trial use (21). These cells are routinely obtained from healthy women with a healthy singleton pregnancy undergoing an elective cesarean section at term and donating their placenta for clinical trial purposes with informed consent. The donor-screening process involves a two-page medical history questionnaire and viral serology testing. The hAECs are isolated in compliance with current Good Manufacturing Practices using the Biospherix Xvivo cell isolator located at the CTRMP. The manufacturing process is undertaken free of animal-based products, and the isolated cells are cryopreserved without culture expansion. An aliquot of the isolated cells is subjected to microbial and endotoxin testing as part of the cell release protocol (below).
The release criteria for the hAECs are as follows (22):
1. Absence of microbial contamination and endotoxins.
2. >96% epithelial cells—determined by flow cytometric analysis of EpCAM expression.
3. <1% mesenchymal stromal cells and leukocytes—determined by flow cytometric analysis of CD90, CD105, and CD45.
4. >80% post-thaw viability.
5. >50% reduction in T cell proliferation index.
6. >25% reduction in M1 (CD86+) macrophages and >10-fold increase in M2 (CD206+) macrophages—determined by macrophage polarization assay.
The primary clinical outcome is safety or frequency of SAEs. The secondary clinical outcome is the patients’ stroke severity (measured by NIHSS) and the level of disability (measured by modified Rankin scale) at 1 week and 90 days. The modified Rankin scale is a widely used tool for measuring outcome in stroke trials. A score of 0 indicates no disability and 6 indicates death. Neurological impairment and functional scores will be measured by a neurologist. The NIHSS will be assessed before the acute study, then again at 24 h, at time points coinciding with MRI scanning (1 week, 1 month, and 12 months), and at any other time during the hospital stay if there is a clinical deterioration. The neurological and safety outcome assessment will therefore be performed by a blinded observer. This observer will not be involved in patient recruitment.
We define a treatment-emergent adverse effect/AE (TEAE) as any event not present before the initiation of treatment or any event already present that worsened in either intensity or frequency after exposure to the study treatment (23). We classify TEAEs as mild, moderate, severe, or life threatening according to standard procedures (23). DLT is defined here to be equivalent to SAE and includes any untoward effect such as death, life threatening, requires hospitalization (for outpatient), prolonged hospitalization (for inpatients), or requires intervention to prevent permanent damage.
The primary imaging outcome is the volume of vasogenic edema at 1 week versus that before treatment. This analysis is performed with a view to assessing safety. The secondary imaging outcome is the change in the infarct expansion ratio (IER) between the outcome post-treatment (T2 FLAIR) infarct volume and the initial DWI infarct volume (see Image Analysis) (16), and symptomatic intracerebral hemorrhage on MR or CT (4). Symptomatic intracerebral hemorrhage is defined by Safe Implementation of Thrombolysis in Stroke Monitoring Study (SIT-MOST) criteria as parenchymal hematoma type 2 (PH2) occurring within 3–6 hours of treatment, combined with neurological deterioration leading to an increase of four points on the NIHSS from baseline, or the lowest NIHSS value after baseline to 24 h (24).
Patients will have MR imaging performed prior to receiving hAECs and at 1 week, 1 month, and at 12 months. The sequences include volumetric 3D T1 images, T2 weighted FLAIR images, DWI (measures infarct volume) and tensor imaging/DTI (assesses integrity of white matter tracts), MR angiography (assesses blood vessel patency), arterial spin labeling (assesses cerebral blood flow), and susceptibility weighted imaging (detection of blood products). The choice of these MR sequences is based on consensus recommendations from an expert panel: Stem Cells as an Emerging Paradigm in Stroke (STEPS) 3 (8).
Images will be de-identified prior to analysis. The analysis will therefore be performed blinded to the dosing regiments. Segmentation of infarct will be performed using in-house purpose-written semi-automated software. In a previous analysis from our group, the IER in the control arm occurred in 75% of patients (16). Hyperintense signal on FLAIR images consists of the infarct (cytotoxic edema) and surrounding vasogenic edema. By contrast, the lesion on DWI consists of cytotoxic but not vasogenic edema. Vasogenic edema is therefore defined as the difference between the hyperintense signal on FLAIR sequence at day 7 minus the hyperintense signal on the DWI sequence (all sequences will be co-registered to the same coordinate space for analysis). The DTI images will be used to characterize longitudinal change in the fractional anisotropy (white matter integrity) around the infarct. Used in this way, it will provide another measure of peri-infarct injury and/or recovery.
We will perform studies to evaluate the effects of the hAECs on the immune response post stroke. Assessments will be made before hAEC administration, 1 h after hAEC infusion, and at 24 h, 3 days, and 1 week. Venepuncture will be performed using EDTA tubes at the time of enrollment and transported to the laboratory. We will measure the following:
1. Lymphocytes: circulating T lymphocytes (CD4+, CD8+), CD56+ natural killer cells, CD19+ B cells, and T regulatory cells.
2. Key cytokines and markers of inflammation: IFNγ, TNFα, IL-10, IL-17, IL-6, high-sensitivity C-reactive protein.
3. BBB integrity: MMP-9 (9).
4. Immunogenicity: HLA typing of hAEC and recipient.
The expected outcome from Aim 1 is the MTD to inform the dose to be used in a Phase 2 trial. A smaller vasogenic edema volume, a decrement in infarct expansion, and a decrement in fractional anisotropy volume with dose escalation will provide another assessment of safety and efficacy. It is expected that at the MTD, infarct expansion will be lower than at the preceding doses and in the control group. The immunological assays should parallel our findings in animal experiments (e.g., dampening of the pro-inflammatory cytokines). The findings of the study will be submitted for publication in peer-reviewed journals. Following this, the application will be made to the National Health Medical Research Council to obtain funding for Phase 2 trial.
If successful, stem cell therapy in the acute phase of ischemic stroke will be transformative for patients who miss out on clot-busting drugs or clot-retrieval therapies, in reducing disability and death. In line with recommendation by the STEPS3 experts, we have avoided including patients who have received TPA or ECR as these therapies would confound the effect of hAECs (8). Apart from allogenic multipotent adult progenitor cells, hAEC is the only other stem cell tested at present in the first 48 h (1, 7). This therapy is attractive as hAECs are readily sourced from healthy term placentae, typically, for logistical ease, after elective cesarean section. There are about 45,000 term elective cesarean section births in Australia alone each year, after which the placenta is discarded. Each term placenta can provide 200–300 million hAECs, sufficient for one stroke patient (70–80 kg) at a dose of 4 million cells per kg. At higher cell doses, pooled cells from two to three placentae would be required for each adult patient. Unlike other forms of stem cell therapy, such as immortalized human neural stem cells derived from 12-week-old human fetuses (18), there are no significant ethical concerns with the collection and therapeutic use of hAECs (25). The risk of tumorigenesis with hAECs is also extremely low due to their lack of expression of telomerase (26).
We have chosen the intravenous route of administration over direct intracerebral delivery as it is less invasive (does not require brain surgery). In our animal experiments, hAECs have action on other organs (such as spleen) affected by stroke. This modulation of the immune response does not require direct intracerebral injection.
From a safety aspect, we have also recently completed a Phase 1 safety trial of intravenously administered hAECs in six preterm infants with established bronchopulmonary dysplasia, using a dose of 1 million cells/kg (ACTRN 12614000174684). No severe adverse events were found to be associated with hAEC treatment. A Phase 1 trial of hAECs in adults with compensated cirrhosis is also underway in our institution (21) (ACTRN12616000437460).
In summary, hAEC-based therapy is a highly innovative alternative to current reperfusion and neuroprotection strategies. In experimental models, hAECs appear to exert effects by modulating the immune response post stroke to limit the ongoing injury at the peri-infarct interface or the “inflammatory penumbra.” If ultimately proven to be successful in Phases 2 and 3, this therapy will have a greater proportion (80–90%) of eligible patients than current reperfusion therapies. It is anticipated that in future trials, we will test the additional benefit of hAECs with reperfusion therapies. This trial is registered with ANZCTRN (ACTRN12618000076279p).
This trial has been approved by the Monash Health Human Research Ethics Committee.
Steering Committee: TP, HM, RL, EW, and CS. Writing group: TP, HM, RL, EW, and CS. Data Safety Monitoring Board: Geoffrey Donnan and Velandai Srikanth.
The authors declare that the research was conducted in the absence of any commercial or financial relationships that could be construed as a potential conflict of interest.
This protocol has been submitted for NHMRC project grant in 2018.
1. Saver JL, Goyal M, van der Lugt A, Menon BK, Majoie CB, Dippel DW, et al. Time to treatment with endovascular thrombectomy and outcomes from ischemic stroke: a meta-analysis. JAMA (2016) 316:1279–88. doi:10.1001/jama.2016.13647
2. Campbell BC, Mitchell PJ, Kleinig TJ, Dewey HM, Churilov L, Yassi N, et al. Endovascular therapy for ischemic stroke with perfusion-imaging selection. N Engl J Med (2015) 372:1009–18. doi:10.1056/NEJMoa1414792
3. Nogueira RG, Jadhav AP, Haussen DC, Bonafe A, Budzik RF, Bhuva P, et al. Thrombectomy 6 to 24 hours after stroke with a mismatch between deficit and infarct. N Engl J Med (2017) 378:11–21. doi:10.1056/NEJMoa1706442
4. Ma H, Parsons MW, Christensen S, Campbell BC, Churilov L, Connelly A, et al. A multicentre, randomized, double-blinded, placebo-controlled phase iii study to investigate extending the time for thrombolysis in emergency neurological deficits (extend). Int J Stroke (2012) 7:74–80. doi:10.1111/j.1747-4949.2011.00730.x
5. Lo EH. A new penumbra: transitioning from injury into repair after stroke. Nat Med (2008) 14:497–500. doi:10.1038/nm1735
6. Dirnagl U, Klehmet J, Braun JS, Harms H, Meisel C, Ziemssen T, et al. Stroke-induced immunodepression: experimental evidence and clinical relevance. Stroke (2007) 38:770–3. doi:10.1161/01.STR.0000251441.89665.bc
7. Hannawi Y, Hannawi B, Rao CP, Suarez JI, Bershad EM. Stroke-associated pneumonia: major advances and obstacles. Cerebrovasc Dis (2013) 35:430–43. doi:10.1159/000350199
8. Savitz SI, Cramer SC, Wechsler L, Consortium S. Stem Cells as an Emerging Paradigm in Stroke 3: enhancing the development of clinical trials. Stroke (2014) 45:634–9. doi:10.1161/STROKEAHA.113.003379
9. Barr TL, Latour LL, Lee KY, Schaewe TJ, Luby M, Chang GS, et al. Blood–brain barrier disruption in humans is independently associated with increased matrix metalloproteinase-9. Stroke (2010) 41:e123–8. doi:10.1161/STROKEAHA.109.570515
10. Castillo-Melendez M, Yawno T, Jenkin G, Miller SL. Stem cell therapy to protect and repair the developing brain: a review of mechanisms of action of cord blood and amnion epithelial derived cells. Front Neurosci (2013) 7:194. doi:10.3389/fnins.2013.00194
11. Houlihan JM, Biro PA, Harper HM, Jenkinson HJ, Holmes CH. The human amnion is a site of MHC class ib expression: evidence for the expression of HLA-E and HLA-G. J Immunol (1995) 154:55665–74.
12. Bailo M, Soncini M, Vertua E, Signoroni PB, Sanzone S, Lombardi G, et al. Engraftment potential of human amnion and chorion cells derived from term placenta. Transplantation (2004) 78:1439–48. doi:10.1097/01.TP.0000144606.84234.49
13. Broughton BR, Lim R, Arumugam TV, Drummond GR, Wallace EM, Sobey CG. Post-stroke inflammation and the potential efficacy of novel stem cell therapies: focus on amnion epithelial cells. Front Cell Neurosci (2012) 6:66. doi:10.3389/fncel.2012.00066
14. Evans MA, Lim R, Kim H-A, Chu HX, Gardiner-Mann CV, Taylor KWE, et al. Acute or delayed systemic administration of human amnion epithelial cells improves outcomes in experimental stroke. Stroke (2018) 49:700–9. doi:10.1161/STROKEAHA.117.019136
15. Phan TG, Donnan GA, Srikanth V, Chen J, Reutens DC. Heterogeneity in infarct patterns and clinical outcomes following internal carotid artery occlusion. Arch Neurol (2009) 66:1523–8. doi:10.1001/archneurol.2009.259
16. MR Stroke CG, Phan TG, Donnan GA, Davis SM, Byrnes G. Proof-of-principle phase II MRI studies in stroke: sample size estimates from dichotomous and continuous data. Stroke (2006) 37:2521–5. doi:10.1161/01.STR.0000239696.61545.4b
17. Hess DC, Wechsler LR, Clark WM, Savitz SI, Ford GA, Chiu D, et al. Safety and efficacy of multipotent adult progenitor cells in acute ischaemic stroke (masters): a randomised, double-blind, placebo-controlled, phase 2 trial. Lancet Neurol (2017) 16:360–8. doi:10.1016/S1474-4422(17)30046-7
18. Kalladka D, Sinden J, Pollock K, Haig C, McLean J, Smith W, et al. Human neural stem cells in patients with chronic ischaemic stroke (PISCES): a phase 1, first-in-man study. Lancet (2016) 388:787–96. doi:10.1016/S0140-6736(16)30513-X
19. O’Quigley J, Pepe M, Fisher L. Continual reassessment method: a practical design for phase 1 clinical trials in cancer. Biometrics (1990) 46:33–48. doi:10.2307/2531628
20. Liu S, Yuan Y. Bayesian optimal interval designs for phase I clinical trials. J R Stat Soc C (2015) 64:507–23. doi:10.1111/rssc.12089
21. Lim R, Hodge A, Moore G, Wallace EM, Sievert W. A pilot study evaluating the safety of intravenously administered human amnion epithelial cells for the treatment of hepatic fibrosis. Front Pharmacol (2017) 8:549. doi:10.3389/fphar.2017.00549
22. Murphy SV, Kidyoor A, Reid T, Atala A, Wallace EM, Lim R. Isolation, cryopreservation and culture of human amnion epithelial cells for clinical applications. J Vis Exp (2014). doi:10.3791/52085
23. Steinberg GK, Kondziolka D, Wechsler LR, Lunsford LD, Coburn ML, Billigen JB, et al. Clinical outcomes of transplanted modified bone marrow-derived mesenchymal stem cells in stroke: a phase 1/2a study. Stroke (2016) 47:1817–24. doi:10.1161/STROKEAHA.116.012995
24. Wahlgren N, Ahmed N, Davalos A, Ford GA, Grond M, Hacke W, et al. Thrombolysis with alteplase for acute ischaemic stroke in the safe implementation of thrombolysis in stroke-monitoring study (sits-most): an observational study. Lancet (2007) 369:275–82. doi:10.1016/S0140-6736(07)60149-4
25. Murphy S, Rosli S, Acharya R, Mathias L, Lim R, Wallace E, et al. Amnion epithelial cell isolation and characterization for clinical use. Curr Protoc Stem Cell Biol (2010) Chapter 1:Unit 1E6. doi:10.1002/9780470151808.sc01e06s13
Keywords: stroke, stem cell, amnion, phase 1, trial
Citation: Phan TG, Ma H, Lim R, Sobey CG and Wallace EM (2018) Phase 1 Trial of Amnion Cell Therapy for Ischemic Stroke. Front. Neurol. 9:198. doi: 10.3389/fneur.2018.00198
Received: 08 February 2018; Accepted: 13 March 2018;
Published: 07 June 2018
Edited by:
Marc Ribo, Hospital Universitari Vall d’Hebron, SpainReviewed by:
María Gutiérrez Fernández, Hospital Universitario La Paz, SpainCopyright: © 2018 Phan, Ma, Lim, Sobey and Wallace. This is an open-access article distributed under the terms of the Creative Commons Attribution License (CC BY). The use, distribution or reproduction in other forums is permitted, provided the original author(s) and the copyright owner are credited and that the original publication in this journal is cited, in accordance with accepted academic practice. No use, distribution or reproduction is permitted which does not comply with these terms.
*Correspondence: Thanh G. Phan, dGhhbmgucGhhbkBtb25hc2guZWR1
Disclaimer: All claims expressed in this article are solely those of the authors and do not necessarily represent those of their affiliated organizations, or those of the publisher, the editors and the reviewers. Any product that may be evaluated in this article or claim that may be made by its manufacturer is not guaranteed or endorsed by the publisher.
Research integrity at Frontiers
Learn more about the work of our research integrity team to safeguard the quality of each article we publish.