- 1Faculty of Medicine, Tehran University of Medical Sciences, Tehran, Iran
- 2Students’ Scientific Research Center, Tehran University of Medical Sciences, Tehran, Iran
Impulse control disorders (ICDs) are relatively frequent in patients with Parkinson’s disease (PD), although it is still unclear whether an underlying pathological process plays a significant role in the development of ICD in PD apart from dopaminergic replacement therapy. In this study, we have investigated alterations of white matter tract in drug-naïve PD patients with ICDs via diffusion MRI connectometry. Our results showed that disrupted connectivity in the complex network of dynamic connections between cerebellum, basal ganglia, cortex, and its spinal projections serves as the underlying neuropathology of ICD in PD not interfered with the contribution of dopaminergic replacement therapy. These findings provide the first evidence on involved white matter tracts in the neuropathogenesis of ICD in drug-naïve PD population, supporting the hypothesis that neural disturbances intrinsic to PD may confer an increased risk for ICDs. Future studies are needed to validate the attribution of the impaired corticocerebellar network to impulsivity in PD.
Introduction
Impulse control disorders (ICDs) are repetitive, excessive, and compulsive behaviors, disrupting a person’s function in major areas of life (1). Prevalence of ICDs is higher among patients with Parkinson’s disease (PD) compared to normal population affecting 6–15.5% of PD patients while hitting 1.1–1.6% of the general adult population (2). Major ICDs distressing PD patients, include pathological gambling, hypersexuality, compulsive buying, and binge eating (3). In addition, other disorders have been reported in the impulsive–compulsive spectrum in PD patients, such as dopamine dysregulation syndrome, dopamine dependency syndrome, dopamine deficiency syndrome (4), punding (stereotyped, repetitive, aimless behaviors), hobbyism (e.g., artistic endeavors, excessive writing) (5), and excessive hoarding (6).
It is now well established that ICDs can be triggered by dopaminergic drugs (7). Therefore, previous studies have mainly attributed the emergence of ICDs in PD patients to the side effect of dopaminergic replacement therapy. Preliminary comparison studies have shown that ICDs are more common in PD patients on dopamine agonists than healthy controls (HC) (8–11), and untreated de novo PD patients manifest these behavioral phenotypes not more than general population (12, 13). However, not all PD patients on dopaminergic drugs suffer ICD. Besides other possible contributing variables, such as younger age, being unmarried, cigarette smoking, male sex, and positive family history (10, 14), it is not yet clear whether neural disturbances intrinsic to PD may confer an increased risk for ICDs. Although prevalence studies have not reached to this notion, there exist some supportive evidence. Milenkova et al. showed that PD patients without ICD perform more impulsively irrelevant of on/off treatment status (15). In addition, disinhibition failure in treated PD was revealed to be related to cortical atrophy in fronto-striatal areas (16), the key regions of the hallmark mesocorticolimbic network responsible of impulsive–compulsive behaviors (17). Similar phenotypic manifestations and neural underpinnings of ICD in PD and non-PD population are apparent in subsequent studies. Different neuroimaging studies in treated PD patients with ICD have shown various dysfunctions in the brain networks involved in decision making and risk processing, such as disconnection between anterior cingulate cortex and the striatum, increased monoaminergic activity in the medial orbitofrontal cortex, an abnormal resting-state dysfunction of the mesocorticolimbic network, etc (18–23). Consequently, it is suggested that ICD should be considered as a distinct endophenotype in PD, resulting from neuroanatomical abnormalities in impulse control regions of the brain, which would be provoked mainly by dopaminergic replacement therapy (24). However, all these studies have been conducted on PD patients already on dopaminergic treatment, so it is impossible to distinguish these findings as a reflection of treatment (25) or potential biomarkers of ICD in PD. A recent functional MRI study was designed to explore neural markers of upcoming ICD in drug-naïve early PD patients after initiation of the dopaminergic therapy. The results demonstrate that altered connectivity in salience, executive, and default-mode networks in baseline visits predict the development of ICD triggered by dopaminergic treatment (26).
In order to examine whether an underlying neuropathological process apart from medication-related effects plays a remarkable role in the establishment of ICD in PD, we investigated alterations of white matter tract in drug-naïve early PD patients with ICDs (PD-ICD) compared to PD patients without ICD (PD-nICD) and healthy controls (HC) via diffusion MRI connectometry.
Materials and Methods
Participants
Participants involved in this research were recruited from Parkinson’s Progression Markers Initiative (PPMI, http://www.ppmi-info.org/) (27). The study was approved by the institutional review board of all participating sites. Written informed consent was obtained from all participants before study enrollment. The study was performed in accordance with relevant guidelines and regulations. The participants’ PD status was confirmed by Movement Disorder Society-Unified Parkinson’s Disease Rating Scale (MDS-UPDRS) and the loss of dopaminergic neurons was observed on DAT scans. Patients were tested and confirmed negative for any neurological disorders apart from PD. Subjects were only excluded if imaging failed specific quality control criteria. 113 cases divided into three groups, (21 PD-ICD, 68 PD-nICD, and 23 HC) were recruited from baseline available diffusion imaging data from PPMI project. ICD was assessed using the Questionnaire for Impulsive–Compulsive Disorders (QUIP), which is a validated screening tool in PD patients (28). Participants in each category were matched for age, sex, and years of education. PD patients of two groups did not differ in terms of disease duration, motor severity (total UPDRS and Hoehn and Yahr stage), motor subtype (tremor versus postural instability gait difficulty), cognitive status (Montreal Cognitive Assessment), and other non-motor symptoms (REM sleep behavior disorder, excessive daytime sleepiness, and olfaction dysfunction). Although neither group showed depressive symptoms based on geriatric depression scale (29), nICD group showed significantly higher scores than ICD group. However, in the following connectometry analysis, PD-nICD did not show lower connectivity in any white matter pathways compared to PD-ICD. PD patients differed from HC in motor impairment and also only in olfactory dysfunction and depressive symptoms among all non-motor symptoms surveyed. Demographic and clinical data are represented in Table 1.
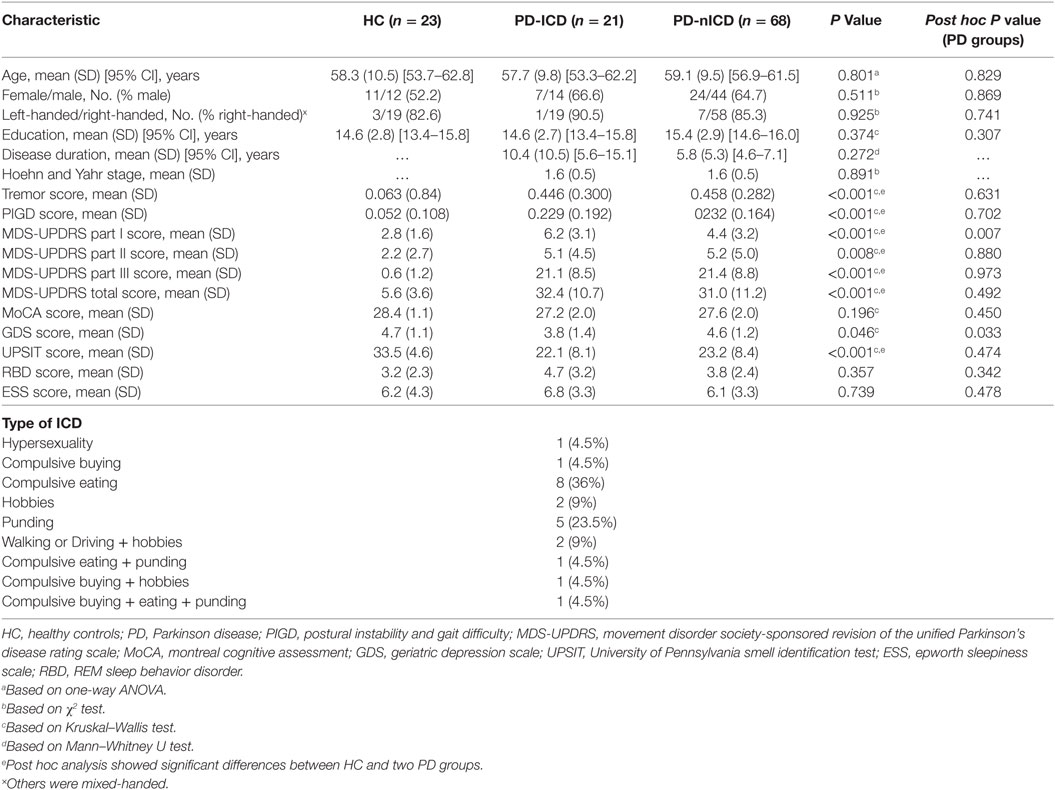
Table 1. Demographic information and comparison of clinical outcomes between HC and patients with PD.
Data Acquisition
Data used in the preparation of this article were obtained from the PPMI database (www.ppmi-info.org/data) (27). This dataset was acquired on a 3 T Siemens scanner, producing 64 diffusion MRI (repetition time = 7,748 MS, echo time = 86 ms; voxel size: 2.0 mm × 2.0 mm × 2.0 mm; field of view = 224 mm × 224 mm) at b = 1,000 s/mm2 and one b0 image along with a 3D T1-weighted structural scan (repetition time = 8.2 ms, echo time = 3.7 ms; flip angle = 8°, voxel size: 1.0 mm × 1.0 mm × 1.0 mm; field of view = 240 mm, acquisition matrix = 240 × 240).
Diffusion MRI Processing
The diffusion MRI data were corrected for subject motion and eddy current distortions using Explore DTI toolbox, which reorients the B-matrix in the stage of realigning the images to preserve the orientational information correctly (30). Orienting B-matrix is a simple, but indeed essential step in avoiding bias in diffusion measures especially in PD patients who are susceptible to move during the scans (31). We also performed quality control analysis on the subject’s signals based on the goodness-of-fit value given in q-space diffeomorphic reconstruction (QSDR) of fibers (32). Each QSDR reconstruction file has a goodness-of-fit value quantified by R2. For example, an R82 indicates a goodness-of-fit between of the subject and template of total 0.82. We excluded cases in which the R2 value did not reach a threshold of 0.6 otherwise.
Between Groups Analysis
The diffusion data were reconstructed in the MNI space using QSDR to obtain the spin distribution function (SDF), to detect the differences between groups (PD-ICD, PD-nICD, and HC).
Connectometry (33) is a novel approach in the analysis of diffusion MRI signals that simply tracks the difference of white matter tracts between groups, or correlation of white matter fibers with a variable of interest. Connectometry approach extracts the SDF in a given fiber orientation, as a measure of water density along that direction. There is a multitude of diffusion indices derived from spin density, i.e., SDF, quantitative anisotropy (QA) being one of them. QA of each fiber orientation gives the peak value of water density in that direction. We used diffusion MRI connectometry to identify white matter tracts in which QA was significantly different between three groups. Resulting uncorrected output was corrected for multiple comparisons by false discovery rate (FDR). A deterministic fiber tracking algorithm (34) was conducted along the core pathway of the fiber bundle to connect the selected local connectomes. Tracts with QA > 0.1, angle threshold lesser than 40o, and tract length greater than 40 mm were included. To estimate the FDR, a total of 2,000 randomized permutation was applied to the group label to obtain the null distribution of the track length. Permutation testing allows for estimating and correcting the FDR of type-I error inflation due to multiple comparisons. The analysis was conducted using publicly available software DSI Studio (http://dsi-studio.labsolver.org).
Statistical Analysis
Demographic and clinical data were analysed using SPSS version 22 (IBM Corp., Armonk, NY, USA). P values less than 0.05 were considered to be statistically significant. Pearson’s chi-square was used to assess nominal variables across groups. Mann–Whitney U test was used to assess differences between two groups, and Kruskal–Wallis test or one-way ANOVA was used for multiple comparisons for three groups.
Results
PD-ICD Patients Versus PD-nICD Patients
The group differences between PD-ICD patients and PD-nICD are shown in Figure 1. Compared with PD-nICD patients, PD-ICD patients showed decreased connectivity in the left and right cortico-thalamic tract, the left and right cortico-pontine tract, the left and right corticospinal tract (CST), the left and right superior cerebellar peduncle (SCP), and the left and right middle cerebellar peduncle (MCP) (FDR = 0.008).
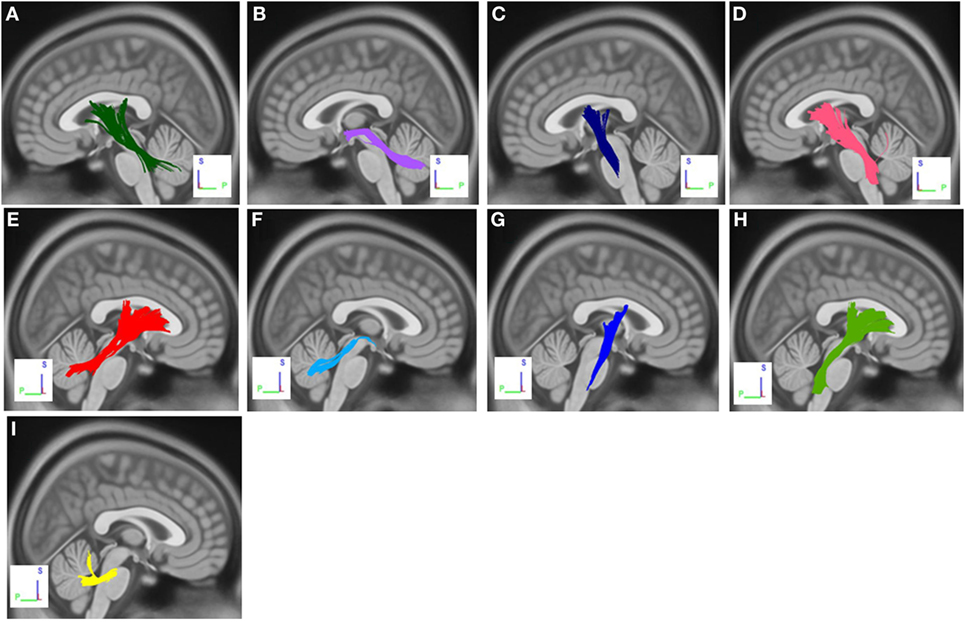
Figure 1. White matter pathways with significantly reduced anisotropy in PD-ICD patients compared to Parkinson’s disease-nICD [false discovery rate = 0.008]. (A) Left cortico-thalamic tract, (B) left superior cerebellar peduncle (SCP), (C) left corticospinal tract (CST), (D) left cortico-pontine tract, (E) right cortico-thalamic tract, (F) right SCP, (G) right CST, (H) right cortico-pontine tract, and (I) middle cerebellar peduncle. The results are overlaid on ICBM152 (mni_icbm152_t1) from the McConnell Brain Imaging Centre using DSI-STUDIO software.
PD-nICD Versus HC
The group differences between PD-nICD patients and HC are shown in Figure 2. The differences were that connectivity in HC was higher than that in PD-nICD in the left inferior longitudinal fasciculus (ILF), the left and right CST, and the left and right cingulum (FDR = 0.001).
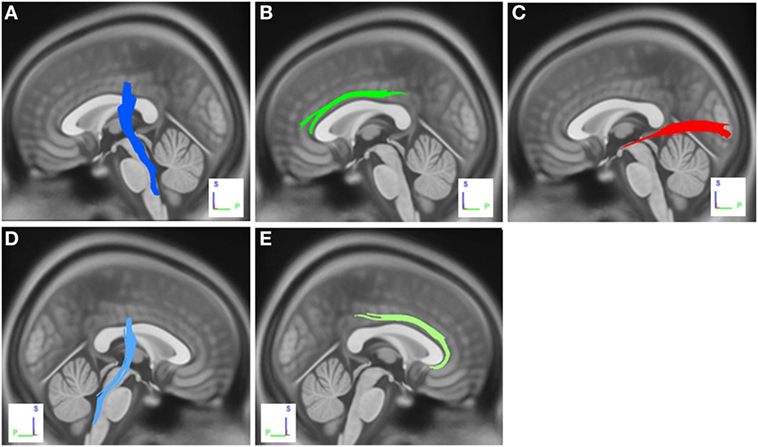
Figure 2. White matter pathways with significantly reduced anisotropy in Parkinson’s disease-nICD compared to healthy controls (false discovery rate = 0.001). (A) Left corticospinal tract, (B) left cingulum, (C) left inferior longitudinal fasciculus, (D) right corticospinal tract, and (E) right cingulum. The results are overlaid on ICBM152 (mni_icbm152_t1) from the McConnell Brain Imaging Centre using DSI-STUDIO software.
PD-ICD Patients Versus HC
The differences were that connectivity in HC was higher than that in PD-ICD patients in the left and right ILF, genu and body of the corpus callosum (CC), the left and right CST, the left SCP, and the left and right cingulum (FDR = 0.002).
Discussion
This study revealed that compared to HC, drug-naïve PD patients have microstructural changes in the CST, ILF, and cingulum. PD-ICD patients also showed additional pathways, i.e., genu and body of CC and SCP compared to HC. These tracts are commonly presented in the literature in relation to various motor and non-motor symptoms of PD, such as olfaction dysfunction, mood and sleep dysregulations, and cognitive decline [reviewed in Hall et al. (35)].
Neural contributions of impulsivity in PD have recently grabbed attention, and some studies have investigated white tract alterations in PD patients with ICDs. In a DTI study, Canu et al. compared white matter microstructure of PD patients with and without punding, at the time when they were on dopaminergic medication. They showed that punding in PD patients is associated with the disconnection between midbrain, limbic, and white matter tracts projecting to the frontal cortex (36). Yoo et al. also indicated some structural alterations in PD-ICD patients, especially in the CC (22). Another study using DTI and resting-state fMRI showed that PD-ICD patients had more severe involvement of frontal, mesolimbic, and motor circuits (23). These results suggest that ICD might be the result of a disconnection between sensorimotor, associative, and cognitive networks in PD patients (23).
fMRI studies showed that ventral striatum and anterior cingulate might be associated with risk and reward-related behaviors and decision making (37, 38). In a risk-taking task, PD-ICD patients showed decreased anterior cingulate and orbitofrontal cortex activity in comparison to PD-nICD. Moreover, pharmacological manipulation (using dopamine agonists) resulted in decreased ventral striatal activity in PD-ICD group, compared with PD-nICD group (38). An experiment with gambling-related visual cue showed that in PD patients with pathological gambling, there is altered activity in the ventral striatum, anterior cingulate cortex, and frontal gyri (39). Resting-state fMRI studies also indicated a functional disconnection between a striatal associative area (the left putamen) and cortical associative (inferior temporal) and limbic regions (anterior cingulate) in PD patients with ICD compared to PD-nICD group (40).
Regarding gray matter (GM), studies are not consistent. Some studies showed that PD patients with ICD had a reduction in cortical thickness of fronto-striatal regions when compared to other PD patients (41). Moreover, Biundo et al. indicated that the level of GM alterations is associated with the severity of ICDs in PD patients (42). Interestingly, Tessitore et al. had completely different results. They indicated that PD-ICD patients have thicker orbitofrontal and anterior cingulate cortices, in comparison to PD-nICD. They also showed that these abnormalities were positively correlated with ICD severity (26). Finally, another study showed relatively preserved GM in PD patients with ICD when compared to PD patients without such disorder (43).
Most studies have compared brain alterations of PD patients with and without ICD at the time they were on dopaminergic medication (44). However, evidence from our study on white matter microstructural alterations in drug-naïve PD patients supports the hypothesis that these abnormalities may be due to neurodegenerative processes intrinsic to PD. These changes might be an independent risk factor for developing ICDs in PD patients and may interact with chronic treatment with dopamine agonists. Other studies have shown that decreased dopamine transporter availability might predict the risk of future ICD behaviors in drug-naïve PD patients who are going to take dopamine replacement therapy in the future (45). Variend et al. also showed that lower level of dopamine transporters in striatal regions might predate the incidence of ICDs in PD patients after the beginning of dopaminergic treatment and may be an independent risk factor for punding behaviors (46). These results highlight the fact that PD itself may play a significant role in developing ICDs in parkinsonian patients.
Cerebellum participates in higher order functions of cognition and emotion by means of bidirectional communications to limbic and paralimbic regions and neocortex, especially prefrontal and posterior parietal areas (47–50). Several behavioral disorders such as impulsive actions are reported following cerebro-cerebellar circuitry damage (51, 52). Disruption of the parieto-ponto-cerebellar loop through lower connectivity in MCP and cerebello-basal ganglia-thalamo-cortical loop via lower connectivity in SCP was demonstrated in relation to ICD in our cohort of PD patients. These loops process information in cognitive, emotional, and behavioral domains. In this complicated network, cerebellum, cortex, and basal ganglia have integrated roles in reinforcement learning anchored to reward predictions of dopamine signals in the striatum (53). The interplay between these structures underlies the complex motor and cognitive functions. Evidence regarding disruption of this system is multitude with respect to motor and cognitive features of PD (53). In particular, the cerebellum is strongly connected to the striatum via output projections of SCP to the thalamus (54). Since striatum as a part of mesocorticolimbic network plays the central role in the pathology of misbehaviors such as addiction and impulsion–compulsion linked to reward learning (17), it seems that cerebellar corroboration in this scenario is often neglected. Although the vast network of cerebro-cerebellar communications is often assumed to be confined to multi-synaptic pathways by means of pontine and thalamic nuclei, simultaneous activation of corticospinal fibers plays a definitive role in relaying feedbacks to the learning processes (53). The contribution of the multi-synaptic cortico-cerebellar network as underlying neuropathology of ICD in early PD without the interference of dopaminergic drugs is a novel and promising result that should be more addressed in future studies.
Some methodological limitations should be considered when interpreting our results, such as small sample size of participants, no-follow up assessments, and not to take into account other risk factors attributed to ICD such as previous histories of addiction and family histories of ICD. Although PD-ICD and PD-nICD patients did not differ in terms of motor and non-motor symptoms, PD patients showed worse scores in screening tests of olfaction function and depressive symptoms compared to healthy controls. This may account for observed alterations in neural connectivity comparing PD-ICD with HC. Future studies are needed to validate if the presented white matter tracts by this preliminary study serve as possible neural markers of ICD in PD. Measurement of the correlation of severity of ICD symptoms with MRI parameters will add valuable information.
In conclusion, this is the first study that investigates the alteration of white matter tracts relative to impulsive–compulsive behaviors in drug-naïve PD patients. Our results showed that disrupted connectivity in the complex network of dynamic connections between cerebellum, basal ganglia, cortex, and its spinal projections serves as the underlying neuropathology of ICD in PD not interfered with the contribution of dopaminergic replacement therapy. Association of these novel pathways provides a potential explanation of why dopamine agonists can lead to an unconscious bias toward risk in some individuals suffering PD. Further studies can evaluate this hypothesis and bring about more evidence, to diagnose ICDs in early stages of PD.
Ethics Statement
All procedures performed here, including human participants were in accordance with the ethical standards of the institutional research committee and with the 1964 Helsinki declaration and its later amendments or comparable ethical standards. Informed consent: informed consent was obtained from all individual participants included in the study.
Author Contributions
MZ, AA-G, FS and MA contributed to the conception and design of the study. MZ, MH, FS, and MA contributed to data collection and analysis. MZ, AA-G, FS, and MA contributed to writing the manuscript.
Conflict of Interest Statement
The authors declare that the research was conducted in the absence of any commercial or financial relationships that could be construed as a potential conflict of interest.
Acknowledgments
The dataset of this work was funded by grants from the Michael J. Fox Foundation for Parkinson’s Research, the W. Garfield Weston Foundation, and the Alzheimer’s Association, the Canadian Institutes for Health Research, and the Natural Sciences and Engineering Research Council of Canada. We thank Christian Beckmann and Simon Eickhoff for their advice on data analysis. Data used in this article were obtained from the Parkinson’s Progression Markers Initiative (PPMI) database (www.ppmi-info.org/data). For up-to-date information on the study, visit www.ppmi-info.org. PPMI is sponsored and partially funded by the Michael J. Fox Foundation for Parkinson’s Research and funding partners, including AbbVie, Avid Radiopharmaceuticals, Biogen, Bristol-Myers Squibb, Covance, GE Healthcare, Genentech, GlaxoSmithKline (GSK), Eli Lilly and Company, Lundbeck, Merck, Meso Scale Discovery (MSD), Pfizer, Piramal Imaging, Roche, Servier, and UCB (www.ppmi-info.org/fundingpartners).
References
1. Weintraub D, Stacy M, David AS, Grant JE, Evans AH. Clinical spectrum of impulse control disorders in Parkinson’s disease. Mov Disord (2015) 30(2):121–7. doi:10.1002/mds.26016
2. Callesen MB, Scheel-Kruger J, Kringelbach ML, Moller A. A systematic review of impulse control disorders in Parkinson’s disease. J Parkinsons Dis (2013) 3(2):105–38. doi:10.3233/JPD-120165
3. Voon V, Fox SH. Medication-related impulse control and repetitive behaviors in Parkinson disease. Arch Neurol (2007) 64(8):1089–96. doi:10.1001/archneur.64.8.1089
4. Wolters EC, van der Werf YD, van den Heuvel OA. Parkinson’s disease-related disorders in the impulsive-compulsive spectrum. J Neurol (2008) 255(5):48–56. doi:10.1007/s00415-008-5010-5
5. Weintraub D, Claassen DO. Impulse control and related disorders in Parkinson’s disease. Int Rev Neurobiol (2017) 133:679–717. doi:10.1016/bs.irn.2017.04.006
6. O’Sullivan SS, Djamshidian A, Evans AH, Loane CM, Lees AJ, Lawrence AD. Excessive hoarding in Parkinson’s disease. Mov Disord (2010) 25(8):1026–33. doi:10.1002/mds.23016
7. Claassen DO, Kanoff K, Wylie SA. Dopamine agonists and impulse control disorders in Parkinson’s disease. US Neurol (2013) 9(1):13–6. doi:10.17925/USN.2013.09.01.13
8. Avanzi M, Baratti M, Cabrini S, Uber E, Brighetti G, Bonfà F. Prevalence of pathological gambling in patients with Parkinson’s disease. Mov Disord (2006) 21(12):2068–72. doi:10.1002/mds.21072
9. Giladi N, Weitzman N, Schreiber S, Shabtai H, Peretz C. New onset heightened interest or drive for gambling, shopping, eating or sexual activity in patients with Parkinson’s disease: the role of dopamine agonist treatment and age at motor symptoms onset. J Psychopharmacol (2007) 21(5):501–6. doi:10.1177/0269881106073109
10. Weintraub D, Koester J, Potenza MN, Siderowf AD, Stacy M, Voon V, et al. Impulse control disorders in Parkinson disease: a cross-sectional study of 3090 patients. Arch Neurol (2010) 67(5):589–95. doi:10.1001/archneurol.2010.65
11. Ambermoon P, Carter A, Hall WD, Dissanayaka NN, O’Sullivan JD. Impulse control disorders in patients with Parkinson’s disease receiving dopamine replacement therapy: evidence and implications for the addictions field. Addiction (2011) 106(2):283–93. doi:10.1111/j.1360-0443.2010.03218.x
12. Antonini A, Siri C, Santangelo G, Cilia R, Poletti M, Canesi M, et al. Impulsivity and compulsivity in drug-naïve patients with Parkinson’s disease. Mov Disord (2011) 26(3):464–8. doi:10.1002/mds.23501
13. Weintraub D, Papay K, Siderowf A, Parkinson’s Progression Markers Initiative. Screening for impulse control symptoms in patients with de novo Parkinson disease: a case-control study. Neurology (2013) 80(2):176–80. doi:10.1212/WNL.0b013e31827b915c
14. Poletti M, Logi C, Lucetti C, Del Dotto P, Baldacci F, Vergallo A, et al. A single-center, cross-sectional prevalence study of impulse control disorders in Parkinson disease: association with dopaminergic drugs. J Clin Psychopharmacol (2013) 33(5):691–4. doi:10.1097/JCP.0b013e3182979830
15. Milenkova M, Mohammadi B, Kollewe K, Schrader C, Fellbrich A, Wittfoth M, et al. Intertemporal choice in Parkinson’s disease. Mov Disord (2011) 26(11):2004–10. doi:10.1002/mds.23756
16. O’Callaghan C, Naismith SL, Hodges JR, Lewis SJ, Hornberger M. Fronto-striatal atrophy correlates of inhibitory dysfunction in Parkinson’s disease versus behavioural variant frontotemporal dementia. Cortex (2013) 49(7):1833–43. doi:10.1016/j.cortex.2012.12.003
17. Lopez AM, Weintraub D, Claassen DO. Impulse control disorders and related complications of Parkinson’s disease therapy. Semin Neurol (2017) 37(2):186–92. doi:10.1055/s-0037-1601887
18. Cilia R, Siri C, Marotta G, Isaias IU, De Gaspari D, Canesi M, et al. Functional abnormalities underlying pathological gambling in Parkinson disease. Arch Neurol (2008) 65(12):1604–11. doi:10.1001/archneur.65.12.1604
19. Cilia R, Cho SS, van Eimeren T, Marotta G, Siri C, Ko JH, et al. Pathological gambling in patients with Parkinson’s disease is associated with fronto-striatal disconnection: a path modeling analysis. Mov Disord (2011) 26(2):225–33. doi:10.1002/mds.23480
20. Joutsa J, Martikainen K, Niemela S, Johansson J, Forsback S, Rinne JO, et al. Increased medial orbitofrontal [18F]fluorodopa uptake in Parkinsonian impulse control disorders. Mov Disord (2012) 27(6):778–82. doi:10.1002/mds.24941
21. Carriere N, Lopes R, Defebvra L, Delmaire C, Dujardin K. Impaired corticostriatal connectivity in impulse control disorders in Parkinson disease. Neurology (2015) 84(21):2116–23. doi:10.1212/WNL.0000000000001619
22. Yoo HB, Lee JY, Lee JS, Kang H, Kim YK, Song IC, et al. Whole-brain diffusion-tensor changes in parkinsonian patients with impulse control disorders. J Clin Neurol (2015) 11(1):42–7. doi:10.3988/jcn.2015.11.1.42
23. Imperiale F, Agosta F, Canu E, Markovic V, Inuggi A, Jacmenica-Lukic M, et al. Brain structural and functional signatures of impulsive-compulsive behaviours in Parkinson’s disease. Mol Psychiatry (2018) 23(2):459–66. doi:10.1038/mp.2017.18
24. Voon V, Dalley JW. Parkinson disease: impulsive choice-Parkinson disease and dopaminergic therapy. Nat Rev Neurol (2011) 7(10):541. doi:10.1038/nrneurol.2011.139
25. Voon V, Napier TC, Frank MJ, Sgambato-Faure V, Grace AA, Rodriguez-Oroz M, et al. Impulse control disorders and levodopa-induced dyskinesias in Parkinson’s disease: an update. Lancet Neurol (2017) 16(3):238–50. doi:10.1016/S1474-4422(17)30004-2
26. Tessitore A, Santangelo G, De Micco R, Vitale C, Giordano A, Raimo S, et al. Cortical thickness changes in patients with Parkinson’s disease and impulse control disorders. Parkinsonism Relat Disord (2016) 24:119–25. doi:10.1016/j.parkreldis.2015.10.013
27. Parkinson Progression Marker Initiative. The Parkinson Progression Marker Initiative (PPMI). Prog Neurobiol (2011) 95(4):629–35. doi:10.1016/j.pneurobio.2011.09.005
28. Weintraub D, Hoops S, Shea JA, Lyons KE, Pahwa R, Driver-Dunckley ED, et al. Validation of the questionnaire for impulsive-compulsive disorders in Parkinson’s disease. Mov Disord (2009) 24(10):1461–7. doi:10.1002/mds.22571
29. Torbey E, Pachana NA, Dissanayaka NN. Depression rating scales in Parkinson’s disease: a critical review updating recent literature. J Affect Disord (2015) 184:216–24. doi:10.1016/j.jad.2015.05.059
30. Leemans A, Jeurissen B, Sijbers J, Jones D. ExploreDTI: a graphical toolbox for processing, analyzing, and visualizing diffusion MR data. Proc Int Soc Mag Reson Med (2009) 17:3536.
31. Leemans A, Jones DK. The B-matrix must be rotated when correcting for subject motion in DTI data. Magn Reson Med (2009) 61(6):1336–49. doi:10.1002/mrm.21890
32. Yeh FC, Tseng WY. NTU-90: a high angular resolution brain atlas constructed by q-space diffeomorphic reconstruction. Neuroimage (2011) 58(1):91–9. doi:10.1016/j.neuroimage.2011.06.021
33. Yeh FC, Badre D, Verstynen T. Connectometry: a statistical approach harnessing the analytical potential of the local connectome. Neuroimage (2016) 125:162–71. doi:10.1016/j.neuroimage.2015.10.053
34. Yeh F-C, Verstynen TD, Wang Y, Fernández-Miranda JC, Tseng W-YI. Deterministic diffusion fiber tracking improved by quantitative anisotropy. PLoS One (2013) 8(11):e80713. doi:10.1371/journal.pone.0080713
35. Hall JM, Martens KAE, Walton CC, O’Callaghan C, Keller PE, Lewis SJ, et al. Diffusion alterations associated with Parkinson’s disease symptomatology: a review of the literature. Parkinsonism Relat Disord (2016) 33:12–26. doi:10.1016/j.parkreldis.2016.09.026
36. Canu E, Agosta F, Markovic V, Petrovic I, Stankovic I, Imperiale F, et al. White matter tract alterations in Parkinson’s disease patients with punding. Parkinsonism Relat Disord (2017) 43:85–91. doi:10.1016/j.parkreldis.2017.07.025
37. Voon V, Pessiglione M, Brezing C, Gallea C, Fernandez HH, Dolan RJ, et al. Mechanisms underlying dopamine-mediated reward bias in compulsive behaviors. Neuron (2010) 65(1):135–42. doi:10.1016/j.neuron.2009.12.027
38. Voon V, Gao J, Brezing C, Symmonds M, Ekanayake V, Fernandez H, et al. Dopamine agonists and risk: impulse control disorders in Parkinson’s disease. Brain (2011) 134(Pt 5):1438–46. doi:10.1093/brain/awr080
39. Frosini D, Pesaresi I, Cosottini M, Belmonte G, Rossi C, Dell’Osso L, et al. Parkinson’s disease and pathological gambling: results from a functional MRI study. Mov Disord (2010) 25(14):2449–53. doi:10.1002/mds.23369
40. Petersen K, Van Wouwe N, Stark A, Lin YC, Kang H, Trujillo-Diaz P, et al. Ventral striatal network connectivity reflects reward learning and behavior in patients with Parkinson’s disease. Hum Brain Mapp (2018) 39(1):509–21. doi:10.1002/hbm.23860
41. Cerasa A, Salsone M, Nigro S, Chiriaco C, Donzuso G, Bosco D, et al. Cortical volume and folding abnormalities in Parkinson’s disease patients with pathological gambling. Parkinsonism Relat Disord (2014) 20(11):1209–14. doi:10.1016/j.parkreldis.2014.09.001
42. Biundo R, Weis L, Facchini S, Formento-Dojot P, Vallelunga A, Pilleri M, et al. Patterns of cortical thickness associated with impulse control disorders in Parkinson’s disease. Mov Disord (2015) 30(5):688–95. doi:10.1002/mds.26154
43. Biundo R, Formento-Dojot P, Facchini S, Vallelunga A, Ghezzo L, Foscolo L, et al. Brain volume changes in Parkinson’s disease and their relationship with cognitive and behavioural abnormalities. J Neurol Sci (2011) 310(1–2):64–9. doi:10.1016/j.jns.2011.08.001
44. Weintraub D, Nirenberg MJ. Impulse control and related disorders in Parkinson’s disease. Neurodegener Dis (2013) 11(2):63–71. doi:10.1159/000341996
45. Smith KM, Xie SX, Weintraub D. Incident impulse control disorder symptoms and dopamine transporter imaging in Parkinson disease. J Neurol Neurosurg Psychiatry (2016) 87(8):864–70. doi:10.1136/jnnp-2015-311827
46. Vriend C, Nordbeck AH, Booij J, van der Werf YD, Pattij T, Voorn P, et al. Reduced dopamine transporter binding predates impulse control disorders in Parkinson’s disease. Mov Disord (2014) 29(7):904–11. doi:10.1002/mds.25886
47. Strick PL, Dum RP, Fiez JA. Cerebellum and nonmotor function. Annu Rev Neurosci (2009) 32:413–34. doi:10.1146/annurev.neuro.31.060407.125606
48. Roldan Gerschcovich E, Cerquetti D, Tenca E, Leiguarda R. The impact of bilateral cerebellar damage on theory of mind, empathy and decision making. Neurocase (2011) 17(3):270–5. doi:10.1080/13554791003730618
49. Rosenbloom MH, Schmahmann JD, Price BH. The functional neuroanatomy of decision-making. J Neuropsychiatry Clin Neurosci (2012) 24(3):266–77. doi:10.1176/appi.neuropsych.11060139
50. Bostan AC, Dum RP, Strick PL. Cerebellar networks with the cerebral cortex and basal ganglia. Trends Cogn Sci (2013) 17(5):241–54. doi:10.1016/j.tics.2013.03.003
51. Schmahmann JD, Sherman JC. The cerebellar cognitive affective syndrome. Brain (1998) 121(4):561–79. doi:10.1093/brain/121.4.561
52. Mariën P, D’aes T. “Brainstem cognitive affective syndrome” following disruption of the cerebrocerebellar network. Cerebellum (2015) 14(2):221–5. doi:10.1007/s12311-014-0624-x
53. Caligiore D, Pezzulo G, Baldassarre G, Bostan AC, Strick PL, Doya K, et al. Consensus paper: towards a systems-level view of cerebellar function: the interplay between cerebellum, basal ganglia, and cortex. Cerebellum (2017) 16(1):203–29. doi:10.1007/s12311-016-0763-3
Keywords: impulse control disorders, Parkinson’s disease, diffusion MRI, connectometry, drug-naïve
Citation: Mojtahed Zadeh M, Ashraf-Ganjouei A, Ghazi Sherbaf F, Haghshomar M and Aarabi MH (2018) White Matter Tract Alterations in Drug-Naïve Parkinson’s Disease Patients With Impulse Control Disorders. Front. Neurol. 9:163. doi: 10.3389/fneur.2018.00163
Received: 15 December 2017; Accepted: 05 March 2018;
Published: 20 March 2018
Edited by:
Angelo Antonini, Università degli Studi di Padova, ItalyReviewed by:
Rachel Paes Guimarães, Universidade Estadual de Campinas, BrazilAntonella Conte, Sapienza Università di Roma, Italy
Copyright: © 2018 Mojtahed Zadeh, Ashraf-Ganjouei, Ghazi Sherbaf, Haghshomar and Aarabi. This is an open-access article distributed under the terms of the Creative Commons Attribution License (CC BY). The use, distribution or reproduction in other forums is permitted, provided the original author(s) and the copyright owner are credited and that the original publication in this journal is cited, in accordance with accepted academic practice. No use, distribution or reproduction is permitted which does not comply with these terms.
*Correspondence: Amir Ashraf-Ganjouei, YS1nYW5qb3VlaUBzdHVkZW50LnR1bXMuYWMuaXI=;
Mohammad Hadi Aarabi, bW9oYW1tYWRoYWRpYXJhYmlAZ21haWwuY29t
†These authors have contributed equally to this work.