- Laboratory of Sensorimotor Research, National Eye Institute, NIH, Bethesda, MD, United States
Despite extensive research, the functions of the basal ganglia (BG) in movement control have not been fully understood. Eye movements, particularly saccades, are convenient indicators of BG function. Here, we review the main oculomotor findings reported in Parkinson’s disease (PD) and genetic parkinsonian syndromes. PD is a progressive, neurodegenerative disorder caused by dopaminergic cell loss within the substantia nigra pars compacta, resulting in depletion of striatal dopamine and subsequent increased inhibitory BG output from the internal globus pallidus and the substantia nigra pars reticulata. Eye movement abnormalities are common in PD: anomalies are more evident in voluntary than reflexive saccades in the initial stages, but visually guided saccades may also be involved at later stages. Saccadic hypometria (including abnormally fragmented saccades), reduced accuracy, and increased latency are among the most prominent deficits. PD patients show also unusually frequent and large square wave jerks and impaired inhibition of reflexive saccades when voluntary mirror saccades are required. Poor convergence ability and altered pursuit are common. Inherited parkinsonisms are a heterogeneous group of rare syndromes due to gene mutations causing symptoms resembling those of PD. Eye movement characteristics of some parkinsonisms have been studied. While sharing some PD features, each syndrome has a distinctive profile that could contribute to better define the clinical phenotype of parkinsonian disorders. Moreover, because the pathogenesis and the underlying neural circuit failure of inherited parkinsonisms are often well defined, they might offer a better prospect than idiopathic PD to understand the BG function.
Introduction
The basal ganglia (BG) are subcortical nuclei located at the base of the forebrain and extensively connected directly and indirectly with all cortical and subcortical structures. The BG promote the initiation of goal-directed movement by removing sustained inhibition of the desired movement and suppressing unwanted movements. Despite considerable advancements in understanding the BG anatomy and function, their complex role in modulating motor behavior, remains far from fully elucidated. The saccadic system offers unique advantages in studying the BG because the neural circuits underlying it are relatively well understood and their functional corticobasal loops are likely similar to those involved in regulating other movements (1). Moreover, saccades can be easily and accurately measured.
Eye movements, and particularly the saccadic system, allow to test a distributed network involving cortical (mainly frontal and parietal) and subcortical (BG, midbrain, brain stem, thalamus, and cerebellum) structures. Other than clinically assessed, eye movements can be quantified through electro-oculography, scleral search coil system, and video-oculography. While electrooculography is the only system allowing recording of eye movement with closed eyes, and search coil contact lenses provide the best temporal and spatial resolution, video-oculography is the most used technique given its non-invasiveness (2).
The saccadic system is usually explored by testing reflexive saccades (pro-saccades) toward a visual stimulus that suddenly appears simultaneously, after (gap paradigm), or overlapping (overlap paradigm), the offset of a fixation point exposure. With respect to the simultaneous condition, latency is usually shorter with a gap and longer with an overlap (3). The antisaccade paradigm, in which the saccade is directed to the opposite direction than the stimulus, is used to test voluntary eye movements and inhibition of reflexive movements. The dorsolateral prefrontal cortex is supposed to be involved in the suppression of the unwanted reflexive movement and, with the posterior-parietal cortex, in the generation of the correct mirror movement, while the frontal eye field (FEF) is associated with antisaccade latency (4). Fixation, memory guided saccades toward previously briefly exposed stimuli, and smooth pursuits are also commonly applied to the evaluation of BG function.
Specific eye movement abnormalities follow BG dysfunction (5, 6). Therefore, eye movements are often analyzed to differentiate Parkinson’s disease (PD) from other parkinsonian syndromes. Indeed, ocular motor abnormalities of idiopathic neurodegenerative parkinsonisms such as progressive supranuclear palsy, multisystem atrophy, corticobasal syndrome, and dementia with Lewy bodies, have been extensively studied and are well known by clinicians (2, 6). Yet, eye movements in genetic parkinsonisms are seldom investigated. Nonetheless, eye movement features might support the differential diagnosis of genetic syndromes. Moreover, inherited diseases with known pathogenesis and neurodegenerative progression might offer a better prospect than idiopathic PD to delineate the neural circuits underlying specific failures of the BG.
Here, we report the main findings of oculomotor studies in PD and genetic parkinsonian syndromes (Table 1).
Parkinson’s Disease
Parkinson’s disease is a progressive, neurodegenerative disorder. Classic clinical manifestations are tremor at rest, muscular rigidity, akinesia (or bradykinesia), and postural instability (7, 8). Included in the typical features of PD are flexed posture and freezing of gait. Non-motor symptoms such as cognitive impairment, apathy, depression, anosmia, dysautonomia, and sleep disorder are also common.
Parkinson’s disease motor manifestations are caused by dopaminergic cell loss within the substantia nigra pars compacta (SNc), resulting in dysfunction of the BG. A cardinal neuro-pathological feature is the development of intracytoplasmic aggregates of α-synuclein, termed Lewy bodies. Because the dopaminergic neurons in SNc project to the striatum (caudate and putamen), SNc cell loss results in depletion of striatal dopamine (9). PD motor symptoms are recognized when 60% of SNc cells are lost, corresponding to 80% depletion of striatal dopamine.
While partly challenged by more recent findings, the classical model depicts two parallel pathways connecting the BG nuclei (Figure 1) (10). The striatum receives input from the FEF, supplementary eye field, DLPFC, and the parietal eye field (PEF). In the direct pathway, dopaminergic projections from the SNc target striatal neurons expressing D1 receptors; D1 neurons send direct inhibitory projections to the BG output nuclei: the internal globus pallidus (GPi) and the substantia nigra pars reticulata (SNr). In the indirect pathway, D2 expressing neurons receive projections from the SNc and connect indirectly to the GPi/SNr through the external globus pallidus (GPe) and the subthalamic nucleus (STN) (11). The SNr inhibits the superior colliculus (SC). The SC is a crucial structure for both voluntary and reflexive saccades. The SC, indeed, integrates visual, somato-sensory, and auditory stimuli in a spatial map and produces a motor saccade command that is sent to the brain stem saccade generators. The cortical-BG-SC pathway is supposed to be particularly important in selecting the most appropriate or most rewarding movement when multiple internal and/or external inputs compete to orient the body, or the eyes, to different locations (12, 13).
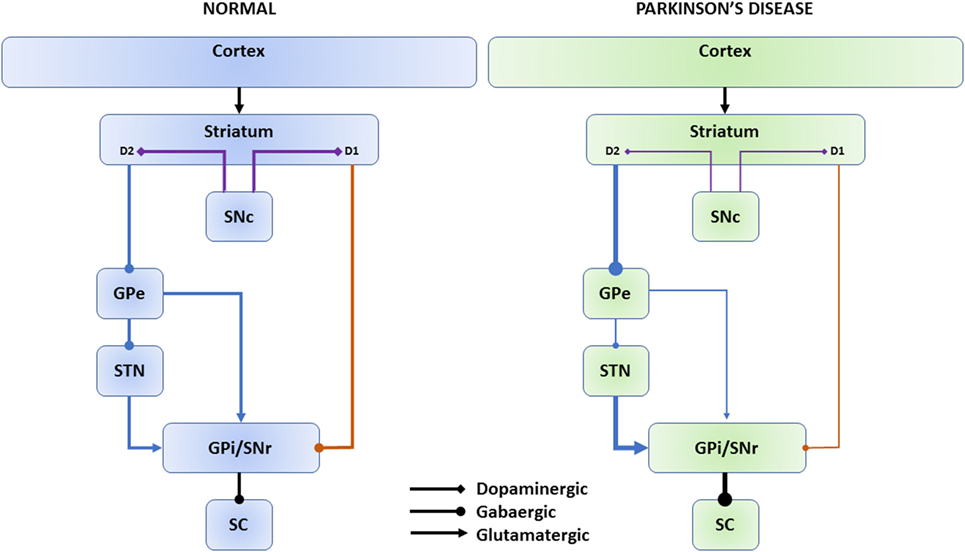
Figure 1. Direct and indirect basal ganglia pathways. The cerebral cortex sends input to the striatum. Dopaminergic projections from the SNc (violet connectors) target striatal neurons expressing D1 or D2 receptors. Direct pathway (orange connectors): D1 neurons send direct inhibitory projections to the SNr/GPi. Indirect pathway (blue connectors): D2 neurons connect indirectly to the GPi/SNr through the GPe and STN. The SNr inhibits the SC. In Parkinson’s disease, dopaminergic depletion leads to reduced inhibitory direct pathway output (thin lines) and increased excitatory indirect pathway output (thick lines) onto the GPi/SNr and, consequently, increased SNr inhibition onto the SC as net effect. SNc, substantia nigra pars compacta; GPe, external globus pallidus; STN, subthalamic nucleus; GPi, internal globus pallidus; SNr, substantia nigra pars reticulata; SC, superior colliculus.
In PD, dopaminergic depletion leads to reduced inhibitory direct pathway output and increased excitatory indirect pathway output onto the GPi/SNr (8, 14). The hyperactivated SNr induces excessive inhibition of the SC, which is considered to be responsible for the typical eye movement abnormalities in PD (15).
However, voluntary saccade generation involves a cortex-BG-SC pathway, whereas reflexive saccades can be generated by direct projections from the parietal cortex onto saccade-related neurons in the intermediate layer of the SC (16). This processing is supposed to largely bypass the BG circuit and it is considered the reason why reflexive saccades are mostly preserved in PD, particularly at early stages of the disease, while voluntary saccades are more severely affected (15, 17). According to this picture, a recent fMRI study, while failing to find differences in saccadic metrics between PD patients and controls, found that PD showed left frontal underactivation during horizontal prosaccades and right parietal overactivation during horizontal and vertical prosaccades and horizontal antisaccades (18).
One of the most prominent features of eye movement abnormality in PD is saccade hypometria (15, 19). As expected, hypometria is more severe in voluntary saccades, particularly in memory-guided saccades, where a subject is required to make a saccade to a remembered target location. Reflexive saccades, usually preserved in the initial stages of PD, can become hypometric in later stages (2). Reflexive saccade hypometria is thought to result solely from excessive SC inhibition, compared to hypometric voluntary saccades that are supposed to be caused by both increased SC inhibition and reduced pre-oculomotor drive due to dysfunctional frontal cortex-BG-SC circuit (20). An alternative explanation for hypometria in PD involves a dysfunction of the cerebellum which is hyperactivated in PD (21). However, a fundamentally preserved saccadic adaptive ability in PD suggests normal cerebellar function, at least during the early stage of the disease (22).
Abnormally fragmented saccades, called multistep or staircase saccades, have been described in PD. These movements, where the target is reached by several hypometric saccades, are observed also in normal subjects (23), but they are more frequent when PD patients execute memory-guided or self-paced saccades (24). Their exact mechanism is still not understood. Gaze fragmentation is supposed to reflect an inappropriate inhibition of the saccade generator (25, 26). Therefore, multistep saccades in PD might be an expression of saccadic hypometria or improper reactivation of omnipause neurons due to SC dysfunction (27), but they could also just reflect a general facilitation in the execution of small saccades (20).
Latency of voluntary saccades is usually delayed in PD, indicating difficulty in initiating volitional eye movements. Latency of reflexive saccades can be spared during the early stages of the disease, when PD patients may produce saccades even faster than normal (express saccades), particularly for small target eccentricities. This facilitation disappears at advanced stages when latency of reflexive saccades also increases, particularly for large target eccentricities (20).
Parkinson’s disease patients show also impairment of inhibition of reflexive saccades to a visual cue when a voluntary mirror saccade is required (so-called antisaccades) (28). Dysfunction of the suppression of unwanted saccades in the DLPFC following dopaminergic depletion in the prefrontal cortex, “leaky” suppression of the SC from the BG, and cognitive impairment have all been associated with impaired saccadic inhibition and “hyper-reflexivity” in PD (4, 15, 29). Antisaccades can reveal deficits of executive functions even in early stages of PD (30). Recently, antisaccade errors have been related to freezing of gait, and increased antisaccade latency in PD has been correlated with impaired postural control (31, 32).
Square wave jerks are saccadic intrusions (usually 0.5–5°) that move the eye from and back to the fixation point with an intersaccadic interval of about 200 ms. Abnormally frequent and large square wave jerks in PD patients have been ascribed to compensatory increased activity in the FEF (19, 26). PD patients show other abnormal eye movements such as poor convergence ability and altered pursuit (33). Pursuit is often saccadic in PD patients who can also show increased pursuit latency and reduced gain and impaired preparation and execution of cue-dependent memory-based smooth-pursuits (34, 35).
A long debate has characterized the finding of ocular tremor in PD patients (36–38). Indeed, while some researchers support the presence of pervasive ocular tremor in PD (39, 40), others consider it the simple consequence of the vestibulo-ocular reflex induced by head movement (41, 42).
Eye movement abnormalities are supposed to impair some behaviors of PD patients. Deficient generation of voluntary saccades, for example, might explain the visual search pattern of PD: patients scan smaller areas than normal with fewer, hypometric saccades, which could lead to a mild degree of visuospatial neglect in PD patients (43). Facilitation of small saccades might underlie reading difficulties (44). Impairment in the generation of voluntary saccades can also affect stability and walking (6).
While impaired oculomotor performance is attributed to dopamine depletion, studies on the effect of dopaminergic treatment on eye movement have given inconsistent results (45, 46).
Finally, PD patients show several oculo-visual dysfunctions (i.e., hallucinations and impairment of visual acuity, color and contrast sensitivity, motion perception, stereopsis), but whether and how they impact eye movement is difficult to establish (47, 48).
Parkinson Disease 1 (PARK1)
Parkinson disease 1 (PARK1, OMIM number #168601) is the first genetically identified parkinsonism. Its associated autosomal dominant mutation in gene SNCA was initially isolated in Italian families (49). SNCA encodes a presynaptic protein, α-synuclein, involved in neuronal plasticity. Intra-neuronal aggregates of α-synuclein are the hallmark of neurodegenerative synucleinopathies, being the major component of Lewy bodies in PD and Lewy body dementia. Tau-inclusions may be also frequent. Neuronal loss is more severe in the brain stem, hippocampus, dorsal motor nucleus of the vagus, SNc, nucleus basalis of Meynert, and locus coeruleus, but it involves also cortical areas (49, 50). The clinical phenotype of patients with mutations in PARK1 resembles typical sporadic PD, except for earlier onset, rapid progression, and frequent cognitive decline. Eye movement recording from two patients with mutated PARK1 showed increased latency of reflexive and voluntary saccades, more frequent multistep saccades, but normal average gain and precision, and normal saccade velocity and duration; patients made more directional errors at the antisaccade task, but corrected as frequently as normal (51).
Parkinson Disease 2 (PARK2)
Parkinson disease 2 (PARK2, #60016) is due to homozygous and compound heterozygous mutations in Parkin (PRKN) and it is the most common genetic parkinsonism. Parkin defects interfere with the ubiquitin-mediated proteolytic pathway and cause accumulation of 22-kD glycosylated α-synuclein leading to neurodegeneration with more involvement of the SNc than the locus coeruleus with respect to idiopathic PD (50). Phenotype is similar to that of sporadic PD.
Recording of eye movements in symptomatic patients with PARK2 mutations showed hypometric saccades with normal latency, antisaccades with normal latency but increased error rate, and reduced gain of smooth pursuit (52, 53).
Parkinson Disease 6 (PARK6)
Parkinson disease 6 (PARK6, #605909) results from mutations in PINK1 coding a mitochondrial protein (PTEN-induced putative kinase 1), causing increased susceptibility to cellular stress and apoptosis (54). Neurodegeneration affects the SNc, brain stem reticular formation, and nucleus basalis of Meynert and it is associated with cortical and brain stem Lewy bodies (50). Patients present with parkinsonism, gait disturbances, and psychosis. A cohort of PINK1 mutation carriers showed increased latency of horizontal prosaccades with normal gain and velocity, higher rate of multistep saccades, normal error rate in the antisaccade task; homozygous (but not heterozygous) patients showed also hypometric memory guided saccades (55).
Parkinson Disease 9 (PARK9)
Parkinson disease 9 (PARK9 or Kufor-Rakeb syndrome, #606693) is a rare autosomal recessive juvenile-onset levodopa-responsive parkinsonism due to mutations in ATP13A2 (PARK9) encoding a lysosomal P-type ATP-ase (56). Mutation of PARK9 leads to α-synuclein accumulation and increased manganese toxicity (57), causing cortical and subcortical neurodegeneration. Beside progressive parkinsonism patients present also with pyramidal signs, facial-faucial-finger mini-myoclonus, and cognitive decline (58).
Neuroimaging shows reduced dopamine transporter activity and reduced gray matter in the motor cortex, prefrontal cortex, somatosensory association cortex, cingulate, caudate, thalamus, and cerebellum. Accumulation of iron in the BG has been detected in some cases.
Typical eye movement abnormalities are vertical supranuclear gaze palsy, slowing of vertical and horizontal saccades, and saccadic pursuit (58, 59). Eye movement recordings from three patients showed hypometric and slow saccades with worse precision, increased or normal latency of horizontal saccades and increased latency of vertical saccades (51, 60). Target was often reached by multistep saccades. Antisaccades directional error rate was increased and patients never corrected the errors (51).
Hypermanganesemia with Dystonia, Polycythemia, and Cirrhosis (HMNDYT1)
Hypermanganesemia with dystonia, polycythemia, and cirrhosis (HMNDYT1, #613280) is a parkinsonism due to recessive mutations in SLC30A10 (61). Loss of function of the encoded manganese transporter leads to a primary metabolic disorder causing hypermanganesemia. Manganese accumulation induces cell toxicity in the liver, bone marrow, and nervous system. Brain MRI T1 hyperintense lesions are present in the caudate and lentiform nuclei, thalamus, corticospinal tract, substantia nigra, posterior pons, and bulbar olives, cerebellum and cerebello-rubro-thalamic pathways.
Eye movement recording from two affected patients showed increased latency of reflexive and voluntary saccades, normal gain and velocity, but worse precision, and increased frequency of multistep saccades. Antisaccade errors were increased, but they were corrected as frequently as normal (51).
Manganese is also a known cause of environmental intoxication upon overexposure in workers and drug abusers (62, 63). In these cases, manganese accumulates in the globus pallidus more than in other brain structures, causing parkinsonism and oculomotor abnormalities. A detailed description of the oculomotor abnormalities is available only for subjects with ephedrone-induced parkinsonism (62). Ephedrone (also called methcathinone or α-methylamino-propiophenone) is a home-made drug obtained by oxidation of ephedrine or pseudoephedrine with potassium permanganate and acetic acid (62). Ephedrone addicts can face manganese intoxication leading to severe, rapidly progressive, non-levodopa responsive parkinsonism and dystonia. Patients showed hypometric and slow horizontal saccades with normal latency, and increased latency of vertical saccades; antisaccades showed normal latency, but increased error rate with normal correction frequency. These findings differ with respect to those shown by subjects with genetic hypermanganesemia, perhaps indicating that the slow manganese accumulation in HMNDYT1 might allow for some adaptation.
Neurodegeneration with Brain Iron Accumulation
Neurodegeneration with brain iron accumulation is a genetically heterogenous disorder causing progressive accumulation of iron in the BG and other brain regions, most cases being associated with mutations in PANK2 and PLA2G6. Pantothenate kinase-associated neurodegeneration (PKAN, formerly Hallervorden-Spatz syndrome, #234200) is a recessive disease associated with mutations in PANK2. Patients present with cognitive dysfunction and extrapyramidal features such as parkinsonism and dystonia. Eye movement abnormalities include hypometric and slow vertical saccades, normal horizontal saccades, saccadic pursuit, impaired vestibulo-ocular reflex suppression, poor convergence, square-wave jerk saccadic intrusions, and abnormal vertical optokinetic reflex (64). Patients with PLA2G6 mutations (#603604) can present with up gaze palsy, poor convergence, saccadic intrusions, and saccadic pursuit (65).
Gaucher Disease
Gaucher disease (#230800, #230900, #231000) is the most common autosomal recessive lysosomal storage disorder and it is due to mutations in GBA leading to deficit of glucocerebrosidase and intracellular accumulation of glucosylceramide. Typical features are hepatosplenomegaly and pancytopenia. Several patients with Gaucher disease presented with parkinsonism. In these patients, neuronal loss occurred in SNc, hippocampus, and cortex (50). Ocular motor abnormalities have been proposed as an early marker to detect neurological impairment in type 3 Gaucher disease, distinguishing it from the subtype without neurological involvement (type1) (66, 67).
Patients affected by type 3 Gaucher disease showed slow velocity (affecting horizontal more than vertical saccades), saccadic hypometria, and increased horizontal saccade latency (68). Saccadic pursuit and oculomotor apraxia were also reported.
Recently, heterozygous GBA mutation carriers have been found to be at higher risk to develop PD and Lewy body dementia (69, 70). The phenotype of these patients can be indistinguishable from that of idiopathic PD.
Conclusion
Studies of eye movements in inherited parkinsonian syndromes are often limited by small sample sizes and by inconsistent examination techniques and paradigms. Nevertheless, these reports support distinct pictures of eye movement disorders for each genetic syndrome, highlighting some features as possible markers for differential diagnosis and for evaluation of disease extension and progression. Still, some characteristics tend to recur across syndromes and could be more informative about BG functions: saccades with increased latency but normal velocity suggest a role of BG in motor initiation rather than execution, so that bradykinesia in parkinsonism should be interpreted more as a delayed motor onset than a slow movement. Also, increased error rate in the antisaccade task, with often normal frequency of correction, might indicate that BG participate more in selecting than planning the proper movement among competitive movements. Other less frequent features are usually associated with more severe phenotypes, possibly indicating more profound BG damage or degenerative involvement of other brain structures.
More studies, applying the same saccadic paradigms and integrating clinical, genetic, neuroimaging, and neuropathological data, are needed for better picturing the oculomotor features associated with BG dysfunctions in PD and parkinsonisms and their relationship with other motor and non-motor symptoms. Clarifying these aspects might provide clinical/diagnostic indications to guide the evaluation of patients with parkinsonism, including its genetic variants. Also, it will help in understanding the modulatory role of BG in behavior.
Author Contributions
EP and LO: design of the work, acquisition and interpretation of data for the work, drafting the work, and final approval of the article.
Conflict of Interest Statement
The authors declare that the research was conducted in the absence of any commercial or financial relationships that could be construed as a potential conflict of interest.
Funding
Study funded by the Intramural Research Programs of NEI.
References
1. Watanabe M, Munoz DP. Probing basal ganglia functions by saccade eye movements. Eur J Neurosci (2011) 33(11):2070–90. doi:10.1111/j.1460-9568.2011.07691.x
2. Gorges M, Pinkhardt EH, Kassubek J. Alterations of eye movement control in neurodegenerative movement disorders. J Ophthalmol (2014) 2014:658243. doi:10.1155/2014/658243
3. Saslow MG. Latency for saccadic eye movement. J Opt Soc Am (1967) 57(8):1030–3. doi:10.1364/JOSA.57.001024
4. Crawford TJ, Bennett D, Lekwuwa G, Shaunak S, Deakin JF. Cognition and the inhibitory control of saccades in schizophrenia and Parkinson’s disease. Prog Brain Res (2002) 140:449–66. doi:10.1016/S0079-6123(02)40068-4
5. Kennard C, Lueck CJ. Oculomotor abnormalities in diseases of the basal ganglia. Rev Neurol (Paris) (1989) 145(8–9):587–95.
6. Anderson TJ, MacAskill MR. Eye movements in patients with neurodegenerative disorders. Nat Rev Neurol (2013) 9(2):74–85. doi:10.1038/nrneurol.2012.273
7. Jankovic J. Parkinson’s disease: clinical features and diagnosis. J Neurol Neurosurg Psychiatry (2008) 79(4):368–76. doi:10.1136/jnnp.2007.131045
8. Rodriguez-Oroz MC, Jahanshahi M, Krack P, Litvan I, Macias R, Bezard E, et al. Initial clinical manifestations of Parkinson’s disease: features and pathophysiological mechanisms. Lancet Neurol (2009) 8(12):1128–39. doi:10.1016/S1474-4422(09)70293-5
9. Shulman JM, De Jager PL, Feany MB. Parkinson’s disease: genetics and pathogenesis. Annu Rev Pathol (2011) 6:193–222. doi:10.1146/annurev-pathol-011110-130242
10. Calabresi P, Picconi B, Tozzi A, Ghiglieri V, Di Filippo M. Direct and indirect pathways of basal ganglia: a critical reappraisal. Nat Neurosci (2014) 17(8):1022–30. doi:10.1038/nn.3743
11. DeLong MR, Wichmann T. Circuits and circuit disorders of the basal ganglia. Arch Neurol (2007) 64(1):20–4. doi:10.1001/archneur.64.1.20
12. Hikosaka O, Takikawa Y, Kawagoe R. Role of the basal ganglia in the control of purposive saccadic eye movements. Physiol Rev (2000) 80(3):953–78.
13. Hikosaka O. Basal ganglia mechanisms of reward-oriented eye movement. Ann N Y Acad Sci (2007) 1104:229–49. doi:10.1196/annals.1390.012
14. DeLong M, Wichmann T. Update on models of basal ganglia function and dysfunction. Parkinsonism Relat Disord (2009) 15(Suppl 3):S237–40. doi:10.1016/S1353-8020(09)70822-3
15. Terao Y, Fukuda H, Ugawa Y, Hikosaka O. New perspectives on the pathophysiology of Parkinson’s disease as assessed by saccade performance: a clinical review. Clin Neurophysiol (2013) 124(8):1491–506. doi:10.1016/j.clinph.2013.01.021
16. May PJ. The mammalian superior colliculus: laminar structure and connections. Prog Brain Res (2006) 151:321–78. doi:10.1016/S0079-6123(05)51011-2
17. Gaymard B. Cortical and sub-cortical control of saccades and clinical application. Rev Neurol (Paris) (2012) 168(10):734–40. doi:10.1016/j.neurol.2012.07.016
18. Lemos J, Pereira D, Almendra L, Rebelo D, Patricio M, Castelhano J, et al. Distinct functional properties of the vertical and horizontal saccadic network in Health and Parkinson’s disease: an eye-tracking and fMRI study. Brain Res (2016) 1648(Pt A):469–84. doi:10.1016/j.brainres.2016.07.037
19. White OB, Saint-Cyr JA, Tomlinson RD, Sharpe JA. Ocular motor deficits in Parkinson’s disease. II. Control of the saccadic and smooth pursuit systems. Brain (1983) 106(Pt 3):571–87. doi:10.1093/brain/106.3.571
20. Terao Y, Fukuda H, Yugeta A, Hikosaka O, Nomura Y, Segawa M, et al. Initiation and inhibitory control of saccades with the progression of Parkinson’s disease – changes in three major drives converging on the superior colliculus. Neuropsychologia (2011) 49(7):1794–806. doi:10.1016/j.neuropsychologia.2011.03.002
21. Wu T, Hallett M. The cerebellum in Parkinson’s disease. Brain (2013) 136(Pt 3):696–709. doi:10.1093/brain/aws360
22. MacAskill MR, Anderson TJ, Jones RD. Adaptive modification of saccade amplitude in Parkinson’s disease. Brain (2002) 125(Pt 7):1570–82. doi:10.1093/brain/awf168
23. Kimmig H, Haussmann K, Mergner T, Lucking CH. What is pathological with gaze shift fragmentation in Parkinson’s disease? J Neurol (2002) 249(6):683–92. doi:10.1007/s00415-002-0691-7
24. Blekher T, Weaver M, Rupp J, Nichols WC, Hui SL, Gray J, et al. Multiple step pattern as a biomarker in Parkinson disease. Parkinsonism Relat Disord (2009) 15(7):506–10. doi:10.1016/j.parkreldis.2009.01.002
25. Optican LM, Rucker JC, Keller EL, Leigh RJ. Mechanism of interrupted saccades in patients with late-onset Tay–Sachs disease. Prog Brain Res (2008) 171(5):567–70. doi:10.1016/S0079-6123(08)00681-X
26. Shaikh AG, Xu-Wilson M, Grill S, Zee DS. ‘Staircase’ square-wave jerks in early Parkinson’s disease. Br J Ophthalmol (2011) 95(5):705–9. doi:10.1136/bjo.2010.179630
27. Paul K, Gnadt JW. Activity of omnipause neurons during “staircase saccades” elicited by persistent microstimulation of the superior colliculus. Vision Res (2006) 46(20):3430–42. doi:10.1016/j.visres.2006.05.014
28. Rivaud-Pechoux S, Vidailhet M, Brandel JP, Gaymard B. Mixing pro- and antisaccades in patients with parkinsonian syndromes. Brain (2007) 130(Pt 1):256–64. doi:10.1093/brain/awl315
29. van Stockum S, MacAskill M, Anderson T, Dalrymple-Alford J. Don’t look now or look away: two sources of saccadic disinhibition in Parkinson’s disease? Neuropsychologia (2008) 46(13):3108–15. doi:10.1016/j.neuropsychologia.2008.07.002
30. Antoniades CA, Demeyere N, Kennard C, Humphreys GW, Hu MT. Antisaccades and executive dysfunction in early drug-naive Parkinson’s disease: the discovery study. Mov Disord (2015) 30(6):843–7. doi:10.1002/mds.26134
31. Walton CC, O’Callaghan C, Hall JM, Gilat M, Mowszowski L, Naismith SL, et al. Antisaccade errors reveal cognitive control deficits in Parkinson’s disease with freezing of gait. J Neurol (2015) 262(12):2745–54. doi:10.1007/s00415-015-7910-5
32. Ewenczyk C, Mesmoudi S, Gallea C, Welter ML, Gaymard B, Demain A, et al. Antisaccades in Parkinson disease: a new marker of postural control? Neurology (2017) 88(9):853–61. doi:10.1212/WNL.0000000000003658
33. Almer Z, Klein KS, Marsh L, Gerstenhaber M, Repka MX. Ocular motor and sensory function in Parkinson’s disease. Ophthalmology (2012) 119(1):178–82. doi:10.1016/j.ophtha.2011.06.040
34. Shibasaki H, Tsuji S, Kuroiwa Y. Oculomotor abnormalities in Parkinson’s disease. Arch Neurol (1979) 36(6):360–4. doi:10.1001/archneur.1979.00500420070009
35. Fukushima K, Fukushima J, Barnes GR. Clinical application of eye movement tasks as an aid to understanding Parkinson’s disease pathophysiology. Exp Brain Res (2017) 235(5):1309–21. doi:10.1007/s00221-017-4916-5
36. Duval C, Beuter A, Gauthier S. Fluctuations in tremor at rest and saccadic eye movements in subjects with Parkinsons disease. Parkinsonism Relat Disord (1997) 3(4):195–205. doi:10.1016/S1353-8020(97)00019-9
37. Duval C, Beuter A. Fluctuations in tremor at rest and eye movements during ocular fixation in subjects with Parkinson’s disease. Parkinsonism Relat Disord (1998) 4(2):91–7. doi:10.1016/S1353-8020(98)00023-6
38. Duval C. Ocular tremor in Parkinson’s disease: the debate is not over. Mov Disord (2013) 28(6):713–4. doi:10.1002/mds.25514
39. Gitchel GT, Wetzel PA, Baron MS. Pervasive ocular tremor in patients with Parkinson disease. Arch Neurol (2012) 69(8):1011–7. doi:10.1001/archneurol.2012.70
40. Baron MS, Gitchel GT, Wetzel PA. Scientific data support that ocular tremor is genuine and unrelated to head movement. Parkinsonism Relat Disord (2014) 20(12):1449–50. doi:10.1016/j.parkreldis.2014.09.032
41. Kaski D, Saifee TA, Buckwell D, Bronstein AM. Ocular tremor in Parkinson’s disease is due to head oscillation. Mov Disord (2013) 28(4):534–7. doi:10.1002/mds.25342
42. Leigh RJ, Martinez-Conde S. Tremor of the eyes, or of the head, in Parkinson’s disease? Mov Disord (2013) 28(6):691–3. doi:10.1002/mds.25478
43. Matsumoto H, Terao Y, Furubayashi T, Yugeta A, Fukuda H, Emoto M, et al. Small saccades restrict visual scanning area in Parkinson’s disease. Mov Disord (2011) 26(9):1619–26. doi:10.1002/mds.23683
44. Yu CY, Lee T, Shariati MA, Santini V, Poston K, Liao YJ. Abnormal eye movement behavior during reading in Parkinson’s disease. Parkinsonism Relat Disord (2016) 32:130–2. doi:10.1016/j.parkreldis.2016.08.008
45. Reilly JL, Lencer R, Bishop JR, Keedy S, Sweeney JA. Pharmacological treatment effects on eye movement control. Brain Cogn (2008) 68(3):415–35. doi:10.1016/j.bandc.2008.08.026
46. Srivastava A, Sharma R, Sood SK, Shukla G, Goyal V, Behari M. Saccadic eye movements in Parkinson’s disease. Indian J Ophthalmol (2014) 62(5):538–44. doi:10.4103/0301-4738.133482
47. Armstrong RA. Oculo-visual dysfunction in Parkinson’s disease. J Parkinsons Dis (2015) 5(4):715–26. doi:10.3233/JPD-150686
48. Weil RS, Schrag AE, Warren JD, Crutch SJ, Lees AJ, Morris HR. Visual dysfunction in Parkinson’s disease. Brain (2016) 139(11):2827–43. doi:10.1093/brain/aww175
49. Golbe LI, Di Iorio G, Bonavita V, Miller DC, Duvoisin RC. A large kindred with autosomal dominant Parkinson’s disease. Ann Neurol (1990) 27(3):276–82. doi:10.1002/ana.410270309
50. Poulopoulos M, Levy OA, Alcalay RN. The neuropathology of genetic Parkinson’s disease. Mov Disord (2012) 27(7):831–42. doi:10.1002/mds.24962
51. Pretegiani E, Rosini F, Federico A, Optican LM, Rufa A. Eye movements in genetic parkinsonisms affecting the α-synuclein, PARK9, and manganese network. Clin Neurophysiol (2017). doi:10.1016/j.clinph.2017.09.107
52. Helmchen C, Schwekendiek A, Pramstaller PP, Hedrich K, Klein C, Rambold H. Blink amplitude but not saccadic hypometria indicates carriers of Parkin mutations. J Neurol (2006) 253(8):1071–5. doi:10.1007/s00415-006-0168-1
53. Machner B, Klein C, Sprenger A, Baumbach P, Pramstaller PP, Helmchen C, et al. Eye movement disorders are different in Parkin-linked and idiopathic early-onset PD. Neurology (2010) 75(2):125–8. doi:10.1212/WNL.0b013e3181e7ca6d
54. Valente EM, Abou-Sleiman PM, Caputo V, Muqit MM, Harvey K, Gispert S, et al. Hereditary early-onset Parkinson’s disease caused by mutations in PINK1. Science (2004) 304(5674):1158–60. doi:10.1126/science.1096284
55. Hertel S, Sprenger A, Klein C, Kompf D, Helmchen C, Kimmig H. Different saccadic abnormalities in PINK1 mutation carriers and in patients with non-genetic Parkinson’s disease. J Neurol (2009) 256(7):1192–4. doi:10.1007/s00415-009-5092-8
56. Ramirez A, Heimbach A, Grundemann J, Stiller B, Hampshire D, Cid LP, et al. Hereditary parkinsonism with dementia is caused by mutations in ATP13A2, encoding a lysosomal type 5 P-type ATPase. Nat Genet (2006) 38(10):1184–91. doi:10.1038/ng1884
57. Gitler AD, Chesi A, Geddie ML, Strathearn KE, Hamamichi S, Hill KJ, et al. Alpha-synuclein is part of a diverse and highly conserved interaction network that includes PARK9 and manganese toxicity. Nat Genet (2009) 41(3):308–15. doi:10.1038/ng.300
58. Williams DR, Hadeed A, al-Din AS, Wreikat AL, Lees AJ. Kufor Rakeb disease: autosomal recessive, levodopa-responsive parkinsonism with pyramidal degeneration, supranuclear gaze palsy, and dementia. Mov Disord (2005) 20(10):1264–71. doi:10.1002/mds.20511
59. Behrens MI, Bruggemann N, Chana P, Venegas P, Kagi M, Parrao T, et al. Clinical spectrum of Kufor-Rakeb syndrome in the Chilean kindred with ATP13A2 mutations. Mov Disord (2010) 25(12):1929–37. doi:10.1002/mds.22996
60. Machner B, Sprenger A, Behrens MI, Ramirez A, Bruggemann N, Klein C, et al. Eye movement disorders in ATP13A2 mutation carriers (PARK9). Mov Disord (2010) 25(15):2687–9. doi:10.1002/mds.23352
61. Quadri M, Federico A, Zhao T, Breedveld GJ, Battisti C, Delnooz C, et al. Mutations in SLC30A10 cause parkinsonism and dystonia with hypermanganesemia, polycythemia, and chronic liver disease. Am J Hum Genet (2012) 90(3):467–77. doi:10.1016/j.ajhg.2012.01.017
62. Bonnet C, Rusz J, Megrelishvili M, Sieger T, Matouskova O, Okujava M, et al. Eye movements in ephedrone-induced parkinsonism. PLoS One (2014) 9(8):e104784. doi:10.1371/journal.pone.0104784
63. O’Neal SL, Zheng W. Manganese toxicity upon overexposure: a decade in review. Curr Environ Health Rep (2015) 2(3):315–28. doi:10.1007/s40572-015-0056-x
64. Egan RA, Weleber RG, Hogarth P, Gregory A, Coryell J, Westaway SK, et al. Neuro-ophthalmologic and electroretinographic findings in pantothenate kinase-associated neurodegeneration (formerly Hallervorden-Spatz syndrome). Am J Ophthalmol (2005) 140(2):267–74. doi:10.1016/j.ajo.2005.03.024
65. Khan AO, AlDrees A, Elmalik SA, Hassan HH, Michel M, Stevanin G, et al. Ophthalmic features of PLA2G6-related paediatric neurodegeneration with brain iron accumulation. Br J Ophthalmol (2014) 98(7):889–93. doi:10.1136/bjophthalmol-2013-304527
66. Harris CM, Taylor DS, Vellodi A. Ocular motor abnormalities in Gaucher disease. Neuropediatrics (1999) 30(6):289–93. doi:10.1055/s-2007-973507
67. Capablo JL, Saenz de Cabezon A, Fraile J, Alfonso P, Pocovi M, Giraldo P, et al. Neurological evaluation of patients with Gaucher disease diagnosed as type 1. J Neurol Neurosurg Psychiatry (2008) 79(2):219–22. doi:10.1136/jnnp.2006.111518
68. Benko W, Ries M, Wiggs EA, Brady RO, Schiffmann R, Fitzgibbon EJ. The saccadic and neurological deficits in type 3 Gaucher disease. PLoS One (2011) 6(7):e22410. doi:10.1371/journal.pone.0022410
69. Clark LN, Ross BM, Wang Y, Mejia-Santana H, Harris J, Louis ED, et al. Mutations in the glucocerebrosidase gene are associated with early-onset Parkinson disease. Neurology (2007) 69(12):1270–7. doi:10.1212/01.wnl.0000276989.17578.02
Keywords: saccades, basal ganglia, α-synuclein, PARK, manganese, Gaucher disease, brain iron accumulation, parkinsonism
Citation: Pretegiani E and Optican LM (2017) Eye Movements in Parkinson’s Disease and Inherited Parkinsonian Syndromes. Front. Neurol. 8:592. doi: 10.3389/fneur.2017.00592
Received: 10 April 2017; Accepted: 23 October 2017;
Published: 09 November 2017
Edited by:
Elena H. Martínez-Lapiscina, Hospital Clínic de Barcelona, SpainReviewed by:
Heather Mack, Eye Surgery Associates, AustraliaGunnar P. H. Dietz, Schwabe Pharma Deutschland, Germany
Cecilia García Cena, Universidad Politécnica de Madrid (UPM), Spain
Copyright: © 2017 Pretegiani and Optican. This is an open-access article distributed under the terms of the Creative Commons Attribution License (CC BY). The use, distribution or reproduction in other forums is permitted, provided the original author(s) or licensor are credited and that the original publication in this journal is cited, in accordance with accepted academic practice. No use, distribution or reproduction is permitted which does not comply with these terms.
*Correspondence: Elena Pretegiani, ZWxlbmEucHJldGVnaWFuaUBuaWguZ292