- 1Department of Neurology, Carl Gustav Carus University Hospital, Technische Universität Dresden, Dresden, Germany
- 2Center for Rare Diseases, Children’s Hospital, Carl Gustav Carus University Hospital, Technische Universität Dresden, Dresden, Germany
- 3Division of Health Care Sciences, Dresden International University, Dresden, Germany
- 4Department of Neurology, Charite University Medicine, Berlin, Germany
- 5Department of Neurology, Beth Israel Deaconess Medical Center, Harvard Medical School, Boston, MA, United States
The vasomotor axon reflex can be evoked in peripheral epidermal nociceptive C-fibers to induce local vasodilation. This neurogenic flare response is a measure of C-fiber functional integrity and therefore shows impairment in patients with small fiber neuropathy. Laser Doppler flowmetry (LDF) and laser Doppler imaging (LDI) are both techniques to analyze vasomotor small fiber function by quantifying the integrity of the vasomotor-mediated axon reflex. While LDF assesses the flare response following acetylcholine iontophoresis with temporal resolution at a single defined skin point, LDI records flare responses with spatial and temporal resolution, generating a two-dimensional map of superficial blood flow. LDF is characterized by a high intra- and interindividual measurement variability, which is smaller in LDI due to its spatial resolution. Nevertheless, LDI still lacks standardized methods for image analysis. Consequently, use of the technique currently remains on an experimental level. Here, we sought to review the current literature on laser Doppler assessment of vasomotor function and discuss potential future applications of established techniques as well as those that are still experimental.
Introduction
In small fiber neuropathy, small somatic fibers and/or autonomic fibers are affected. Small fibers are unmyelinated C-fibers or thinly myelinated A-delta fibers that mediate thermal sensation, pain, and autonomic function, such as blood vessel and sweat gland control. A selective damage to these peripheral nerve fibers can be caused by a number of conditions and therefore comprises a variety of pathophysiologically heterogenous disorders, among them diabetes, neurodegenerative diseases, or paraneoplastic syndromes. Small fiber neuropathy is associated with increased cardiovascular morbidity and mortality, therefore early diagnosis and continued assessment of disease progression is important (1, 2). Assessment of cutaneous vasomotor function is a promising way to diagnose and monitor small fiber neuropathy. However, only few techniques are available.
Based on the assessment of the Doppler shift of backscattered laser light by moving red blood cells (3), change in cutaneous blood flow due to vasoconstrictive or vasodilating stimuli can be quantified using single-point laser Doppler Flowmetry (LDF) or the two-dimensional laser Doppler imaging (LDI). To test the neurogenic control of cutaneous blood vessels, these techniques are combined with iontophoresis of a cholinergic agent, such as acetylcholine, to allow assessment of the integrity of the neurogenic axon reflex as a way to investigate small nerve fiber function (4, 5).
Laser Doppler flowmetry investigates vasomotor axon reflex response with temporal resolution in fixed points of the skin. It is widely used in research but its clinical use is limited due to a high intra- and interindividual variability. LDI assesses the vasodilatory response after acetylcholine iontophoresis using spatial and temporal resolution, thereby reducing variability. However, it has not attained widespread use, and only few clinical studies have used this technique to assess neurovascular dysfunction (Table 1).
This review provides a summary of the current literature on laser Doppler assessment of vasomotor function and critically discusses the utility and drawbacks of available and experimental techniques in research and clinical practice as well as potential future implications.
Assessment of Vasomotor Axon Reflex Response
The Vasomotor Axon Reflex
Specific examination of quality and integrity of peripheral autonomic nerve fibers can be performed with vasomotor (neurovascular endothelial function) and sudomotor (sweat gland function) tests that are usually only performed in specialized academic centers. In the beginning of the twentieth century, the term “axon reflex” has been described in vasomotor fibers that were studied and investigated afterward in different clinical settings (6, 7). The axon reflex can be evoked directly by pharmacological (iontophoresis of 10% acetylcholine), thermal, electrical, or mechanical stimuli. The topical stimulation depolarizes unmyelinated C-fibers in the skin and results in afferent action potentials that are conducted orthodromically toward the spinal cord. At branching points of the nerve, the action potential continues antidromically, ending at dermal blood vessels adjacent to the initial stimulation point. This triggers the release of vasoactive substances, such as substance P and calcitonin gene-related peptide, from nerve fiber terminals and leads to a vasodilatory response (7–9) (Figure 1). The extended area of cutaneous vasodilation following acetylcholine iontophoresis is mediated indirectly through the axon reflex, indicating the functional integrity of vasomotor C-fibers (7, 8).
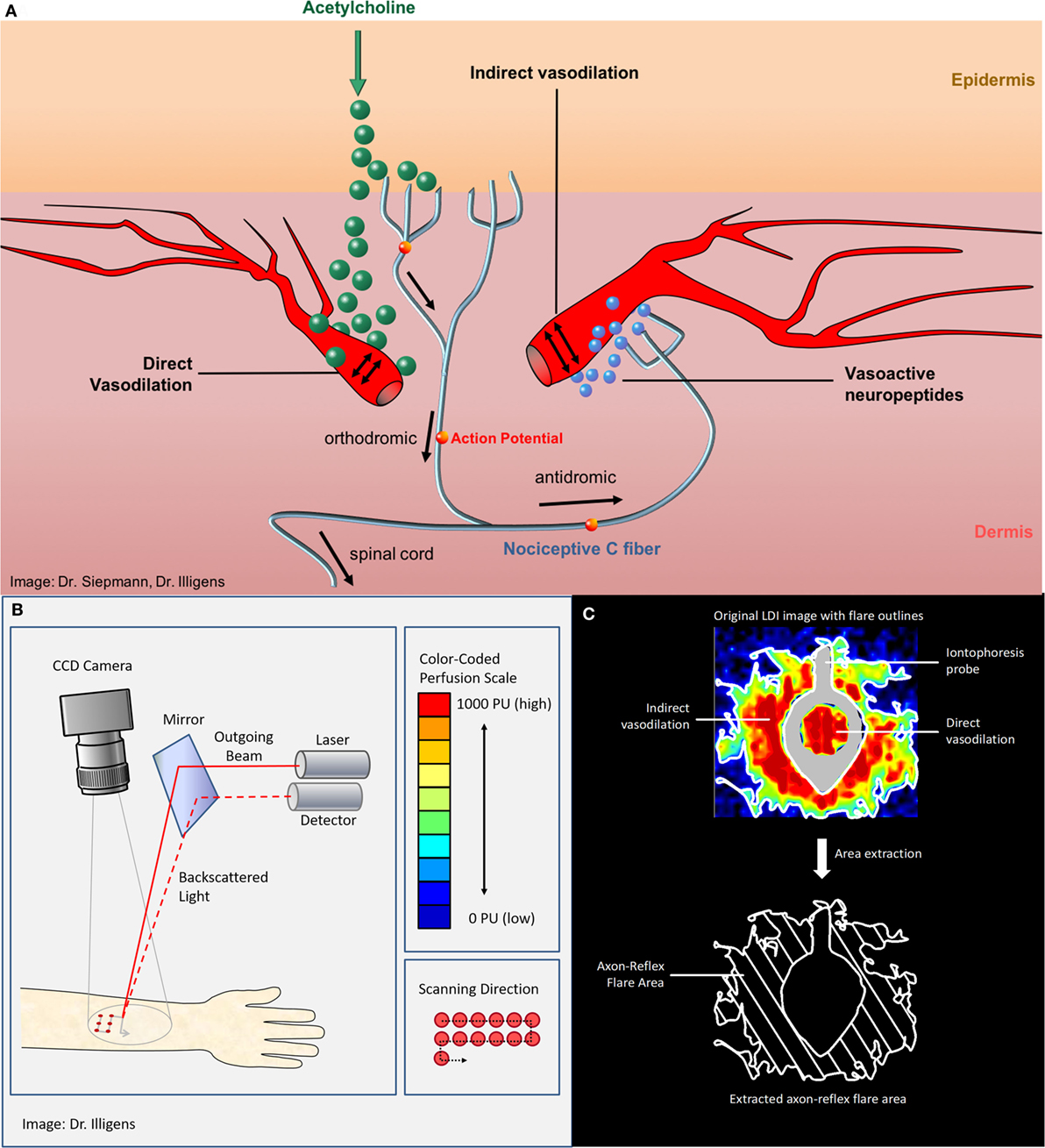
Figure 1. (A) The vasomotor axon reflex: iontophoresis of acetylcholine results in stimulation of peripheral epidermal nociceptive C-fibers and thereafter indirectly results in local vasodilatation. The topical stimulation depolarizes unmyelinated C-fibers in the skin and results in afferent action potentials that are conducted orthodromically toward the spinal cord. At branching points of the nerve, the impulse continues antidromically, ending at arterial dermal vessels adjacent to the initial stimulation point. There, the axon reflex induces the release of vasoactive substances, such as substance P and calcitonin gene-related peptide, from nerve terminals and leads to vasodilation. (B) Laser Doppler imager setup and principle of measurement. (C) The upper panel shows the color-coded laser Doppler imaging perfusion data with iontophoresis probe framing direct vasodilation induced by direct stimulation as well as axon reflex-mediated enhancement of blood flow (“flare”) in an adjacent skin area surrounding the probe (“indirect flare”). The lower panel shows the extracted axon reflex flare, which provides a measure of functional integrity of those nociceptive C-fibers that mediate the vasomotor axon reflex upon activation through acetylcholine.
Iontophoresis of Acetylcholine to Induce Axon Reflex-Mediated Blood Flow
Iontophoresis of vasoactive substances via a drug-delivering capsule is a common technique for studying pharmacological aspects of the microvascular function (10). Particularly iontophoresis of acetylcholine is used to evoke axon reflex–mediated increase in blood flow, also referred to as flare response (7, 11). Iontophoresis of acetylcholine stimulates endothelium-dependent production of substance P, calcitonin gene-related peptide, and nitric oxide leading to microvascular vasodilation. Therefore, the detected flux beneath the iontophoresis capsule is referred to as direct response (12, 13). In contrast, the detected flux next to the iontophoresis capsule, called indirect response, is axon reflex mediated and therefore represents a measure of small nerve fiber function (7, 11, 14).
During the past 10 years, iontophoresis technique has been used in various experimental settings but to date, there are no consistent iontophoresis protocols throughout the vasomotor studies (15, 16). Current density, dose, and acetylcholine concentration differ between studies, making the comparison of study results difficult (17–19).
In addition, the axon reflex can be evoked by a wide range of physiologic stimuli such as heat, cold, mechanical distension, and UV light (20, 21). However, in clinical research, iontophoresis of acetylcholine has been widely established due to its precision in delivering a predefined dosage of the compound. Moreover, the technique may be superior to others due to its capacity to mediate a neurogenic blood flow response which has been shown to be mediated neither by phenomena secondary to iontophoresis nor endothelium-mediated vasodilation (22–25).
Laser Doppler Assessment
Axon reflex-based tests of peripheral small nerve fiber function assess neurogenic response of cutaneous blood vessels to vasodilatory stimuli and can be quantified by LDF and LDI (14).
Single-Point LDF
Laser Doppler flowmetry uses a single-frequency (monochromatic), coherent laser light source on a fixed area of the skin. Processing the frequency distribution of the backscattered light allows an estimation of the microvascular blood perfusion (3). LDF quantifies the vasodilator response following acetylcholine iontophoresis using temporal resolution at a single and defined skin point, with a predefined distance from the delivery capsule of acetylcholine (4). The test thereby allows detecting cutaneous perfusion differences between patients with peripheral neuropathy and healthy individuals (1).
Two-dimensional LDI
Laser Doppler imaging constitutes a newer axon reflex-based measurement method, which records the vasomotor axon reflex-mediated flare response with spatial and temporal resolution and generates a two-dimensional map of the cutaneous blood flow (26). The imaging instrument scans the desired, predefined skin area with a laser beam at a fixed distance from the surface of the skin, allowing to generate a perfusion map of a heterogeneous tissue (2, 27). In this technique, the principal task of the laser Doppler imager is to record images, as a map of single-point cell data (2). These images reflect relative values of each measured cell, measured in perfusion units. When a cell’s perfusion unit exceeds a threshold, fixed at an arbitrary level, a flare response is registered. These baseline characteristics do not change across analysis techniques, but the definition of the appropriate threshold have been different between each analysis method (2). The presence of different analysis techniques to measure the extent of LDI axon reflex-induced flare area limits its consistent application and generalization of results. The search for the best technique is, therefore, objective of ongoing clinical studies.
LDI Axon Reflex Flare Area Test
Laser Doppler imaging measures the microvascular blood flow sequentially over a defined skin area, representing skin blood flow two-dimensionally over time (28). Thus, LDI allows creation of a topographical map of skin perfusion by estimating the extent of the axon reflex-based flare area following the vasodilator response after acetylcholine iontophoresis (9). Using standardized conditions, the laser Doppler perfusion imager obtains over a predefined area of interest baseline images to quantify the resting blood flow, followed by images taken during and after iontophoresis. Obtained perfusion images are analyzed using a color perfusion code, where dark blue represents the lowest perfusion value, and red the highest perfusion value displayed (4). Compared to the single-point blood flow measurement of LDF, LDI allows visualization of the size, intensity, and shape of the axon reflex-induced hyperemic flare area and calculation of the average perfusion (14, 29). The subtraction of the direct dilation area gives a measure of the LDI detected flare area (Figure 1). Since the flare depends on an intact axon reflex response in peripheral C-fibers, patients with neuropathy show a decreased flare area as measured by LDI (14). Typically, the axon reflex-mediated local blood flow presents an irregular shape, rather than a homogeneous circle. This is a challenge when using LDF, since probes are placed at a fixed distance from the iontophoresis capsule, and therefore can be easily placed over a less innervated skin area resulting in a large coefficient of variation (7, 11, 14, 30). Thus, LDI might be more suitable for clinical evaluation of small fiber neuropathies (14, 31, 32).
Available Blood Flow Analysis Techniques
There are several techniques available to analyze the axon reflex-induced vasodilatory response following acetylcholine iontophoresis. All analyzing techniques have in common that a baseline perfusion threshold is predetermined initially and used as reference for subsequent perfusion measurements.
Flare Area Method. The flare area method sets the threshold by averaging the maximum perfusion reaction of all cells. Thereafter, the axon reflex flare is determined by the number of cells above the set threshold after acetylcholine iontophoresis subtracted from the number of cells above the threshold before the iontophoresis (2):
Baseline Perfusion Method. The baseline perfusion method defines the blood flow threshold as two standard deviations of the baseline perfusion. Thereafter, the total axon reflex-mediated flare area is calculated based on the total number of cells exceeding the defined threshold (2).
Resting Blood Flow Method. After acetylcholine iontophoresis the axon reflex-mediated flare response is represented by the measurement of the total number of cells exceeding the previous identified blood flow threshold (2, 14). Compared to resting blood flow, axon reflex-mediated flare response was defined as an increase of three times of the baseline blood flow value (14).
Limitations and Drawbacks
Both LDF and LDI should be applied under constant temperature, humidity, and light conditions to ensure reliable measurements. The distance to the tested skin area should be constant as well in all measurements as it influences the outcome of the imaging processes of both techniques. A calibration of each device before starting the measurement is recommended. Therefore, the use of both methods in bedside testing is challenging from the clinical point of view. Moreover, the LDI as well as the LDF technique require a thorough preparation of the studied skin area. Hairs should be removed from the skin, and skin scales should be removed carefully to avoid any disturbances in the imaging processes. The use of both methods on irritated skin is not recommended. In addition, for LDI, movement of the scanned area has to be avoided since this will introduce movement artifacts in the recording, limiting quality of the data obtained.
Laser Doppler flowmetry assessment is characterized by a high intra- and interindividual variability and is limited by technically demanding settings. Since most investigators agree that it lacks sensitivity to detect vasomotor neuropathy in individuals during clinical routine diagnosis, LDF is being limited to specialized clinical centers with specific demands and research studies (26, 29, 33–35). Furthermore, LDF cannot determine the extent of the flare area, since the blood flow change is measured in single points at a predetermined distance from the site of iontophoresis (4, 23, 33, 34).
Using the LDI technique in clinical or research setting requires training. It is recommended to set up the LDI equipment before acetylcholine iontophoresis. The LDI needs to be placed at an appropriate distance over the iontophoresis capsule parallel to the skin before starting the imaging process. The presence of different analysis techniques to measure the extent of LDI axon reflex-induced flare area limits its consistent application and generalization of results. Most importantly, to improve utility standardization of image analyses presets is necessary. Therefore, studies to define parameters relevant to diagnostic discrimination such as sensitivity and specificity are needed. Especially, a set of normative data gained in studies in large populations of healthy subjects compared with patients with neuropathy should form the basis for future application. Furthermore, technical independence from environmental factors such as light, temperature, and humidity would improve its application. Until now, application of LDI axon reflex flare area test remains on an experimental level in clinical diagnostics and is limited to specialized centers (2, 14, 26, 31, 32).
Laser Doppler-Based Assessment of Neurovascular Function in Clinical Research
Even though clinical studies assessing these tests are still rare, some studies have been published proving their utility. It has been suggested that LDI is a simple method to detect early neuropathy in subjects with type 2 diabetes and may have some value as an endpoint to assess therapeutic interventions aiming to prevent or reverse C-fiber dysfunction (4). Furthermore, laser Doppler devices used to measure C-fiber axon reflex showed impairment in both patients with type 1 and type 2 diabetes (8). It is possible that functional abnormalities in diabetic peripheral neuropathy are not homogeneous, however whether C-fiber subclasses are differentially involved in type 1 and type 2 diabetes mellitus remain speculative and warrant further studies (5).
Laser Doppler imaging has shown a high sensitivity for diabetic mixed fiber neuropathy detection and could be performed with immediate results (36). Its reproducibility was also confirmed in healthy subjects (37). LDF had been studied in familial dysautonomia and has helped to understand the relationship between receptors and innervation in disorders of autonomic dysfunction (38). It has also been used to detect the onset of small fiber neuropathy also in transthyretin-related familial amyloid polyneuropathy (39).
In neurodegenerative disorders, reduced laser Doppler blood flow in patients with Huntington’s disease supports the hypothesis that autonomic nervous system dysfunction is part of the pathogenesis of Huntington’s disease (40). In patients with Alzheimer’s disease, LDI has shown a significantly attenuated skin vascular response following iontophoresis of acetylcholine, indicating abnormalities in receptor/signal transduction, supporting the idea that Alzheimer’s disease might be a systemic disorder (41). Recently, reduced vasoconstrictive response of cutaneous blood vessels of patients with Parkinson’s disease compared to healthy subjects has been shown using LDF, indicating a significant impairment of vasomotor function in these patients as a manifestation of cutaneous autonomic neuropathy (42, 43). Importantly, severity of impaired vasoconstrictive response increased in advanced disease stages (44).
Conclusion and Perspective
Assessment of microvascular function in peripheral small fiber neuropathy is useful to diagnose vasomotor dysfunction and supplements other well-established autonomic function tests, such as tilt-table testing and the measurement of heart rate variability. Hence, vasomotor axon reflex-mediated flare measurement techniques have been gaining importance in recent years not only in research studies but also in specialized academic and clinical centers.
Laser Doppler imaging presents increased accuracy and reproducibility when compared to the single-point LDF technique by using temporal and spatial resolution. However, standardized blood flow image analysis warrants further validation in larger study groups before the technique can be established in clinical practice.
Author Contributions
MK: conception and drafting the manuscript. AK, JP, SB, and KB: reviewing the manuscript for intellectual content. BI: creating figures and reviewing the manuscript for intellectual content. TS: conception, creating figures, and reviewing the manuscript for intellectual content.
Conflict of Interest Statement
The authors declare that the research was conducted in the absence of any commercial or financial relationships that could be construed as a potential conflict of interest.
Acknowledgments
The authors thank Professor Heinz Reichmann and Professor Roy Freeman for their general support.
Funding
Publication costs were funded by the Open Access Publication Funds of the Technische Universität Dresden.
References
1. Freeman R. Autonomic peripheral neuropathy. Lancet (2005) 365(9466):1259–70. doi:10.1016/S0140-6736(05)74815-7
2. Illigens BMW, Siepmann T, Roofeh J, Gibbon CH. Laser Doppler imaging in the detection of peripheral neuropathy. Auton Neurosci (2013) 177(2):286–90. doi:10.1016/j.autneu.2013.06.006
3. Frerichs KU, Feuerstein GZ. Laser-Doppler flowmetry. Mol Chem Neuropathol (1990) 12:55. doi:10.1007/BF03160057
4. Krishnan STM, Rayman G. The LDIflare: a novel test of C-fiber function demonstrates early neuropathy in type 2 diabetes. Diabetes Care (2004) 27(12):2930–5. doi:10.2337/diacare.27.12.2930
5. Berghoff M, Kilo S, Hilz MJ, Freeman R. Differential impairment of the sudomotor and nociceptor axon-reflex in diabetic peripheral neuropathy. Muscle Nerve (2006) 33(4):494–9. doi:10.1002/mus.20497
6. Langley JN. On axon-reflexes in the pre-ganglionic fibres of the sympathetic system. J Physiol (1900) 25(5):364–98. doi:10.1113/jphysiol.1900.sp000803
7. Berghoff M, Kathpal M, Kilo S, Hilz MJ, Freeman R. Vascular and neural mechanisms of ACh-mediated vasodilation in the forearm cutaneous microcirculation. J Appl Physiol (2002) 92(2):780–8. doi:10.1152/japplphysiol.01167.2000
8. Kilo S, Berghoff M, Hilz M, Freeman R. Neural and endothelial control of the microcirculation in diabetic peripheral neuropathy. Neurology (2000) 54(6):1246–52. doi:10.1212/WNL.54.6.1246
9. Siepmann T, Penzlin AI, Illigens BMW. [Autonomic neuropathies – diagnosis and evidence based treatment]. Dtsch Med Wochenschr (2013) 138(30):1529–32. doi:10.1055/s-0033-1343270
10. Dixit N, Bali V, Baboota S, Ahuja A, Ali J. Iontophoresis – an approach for controlled drug delivery: a review. Curr Drug Deliv (2007) 4(1):1–10. doi:10.2174/1567201810704010001
11. Caselli A, Rich J, Hanane T, Uccioli L, Veves A. Role of C-nociceptive fibers in the nerve axon reflex-related vasodilation in diabetes. Neurology (2003) 60(2):297–300. doi:10.1212/01.WNL.0000040250.31755.F9
12. Morris SJ, Shore AC, Tooke JE. Responses of the skin microcirculation to acetylcholine and sodium nitroprusside in patients with NIDDM. Diabetologia (1995) 38(11):1337–44. doi:10.1007/BF00401767
13. Morris SJ, Shore AC. Skin blood flow responses to the iontophoresis of acetylcholine and sodium nitroprusside in man: possible mechanisms. J Physiol (1996) 496(2):531–42. doi:10.1113/jphysiol.1996.sp021704
14. Green AQ, Krishnan ST, Rayman G. C-fiber function assessed by the laser Doppler imager flare technique and acetylcholine iontophoresis. Muscle Nerve (2009) 40(6):985–91. doi:10.1002/mus.21333
15. Hamdy O, Abou-Elenin K, LoGerfo FW, Horton ES, Veves A. Contribution of nerve-axon reflex-related vasodilation to the total skin vasodilation in diabetic patients with and without neuropathy. Diabetes Care (2001) 24(2):344–9. doi:10.2337/diacare.24.2.344
16. Henricson J, Tesselaar E, Persson K, Nilsson G, Sjöberg F. Assessment of microvascular function by study of the dose–response effects of iontophoretically applied drugs (acetylcholine and sodium nitroprusside)—methods and comparison with in vitro studies. Microvasc Res (2007) 73(2):143–9. doi:10.1016/j.mvr.2006.10.004
17. Droog EJ, Sjöberg F. Nonspecific vasodilatation during transdermal iontophoresis—the effect of voltage over the skin. Microvasc Res (2003) 65(3):172–8. doi:10.1016/S0026-2862(03)00002-5
18. Droog EJ, Henricson J, Nilsson GE, Sjöberg F. A protocol for iontophoresis of acetylcholine and sodium nitroprusside that minimises nonspecific vasodilatory effects. Microvasc Res (2004) 67(2):197–202. doi:10.1016/j.mvr.2003.12.003
19. Gibbons CH, Freeman R, Veves A. Diabetic neuropathy: a cross-sectional study of the relationships among tests of neurophysiology. Diabetes Care (2010) 33(12):2629–34. doi:10.2337/dc10-0763
20. Steinhoff M, Ständer S, Seeliger S, Ansel JC, Schmelz M, Luger T. Modern aspects of cutaneous neurogenic inflammation. Arch Dermatol (2003) 139(11):1479–88. doi:10.1001/archderm.139.11.1479
21. Hassan AA, Rayman G, Tooke JE. Effect of indirect heating on the postural control of skin blood flow in the human foot. Clin Sci (1986) 70:577–82. doi:10.1042/cs0700577
22. LeVasseur SA, Gibson SJ, Helme RD. The measurement of capsaicin-sensitive sensory nerve fiber function in elderly patients with pain. Pain (1990) 41:19–25. doi:10.1016/0304-3959(90)91104-Q
23. Walmsley D, Wiles PG. Assessment of the neurogenic flare response as a measure of nociceptor C fibre function. J Med Eng Technol (1990) 14(5):194–6. doi:10.3109/03091909009009960
24. Westerman RA, Widdop RE, Hogan C, Zimmet P. Non-invasive tests of neurovascular function: reduced responses in diabetes mellitus. Neurosci Lett (1987) 81:177–82. doi:10.1016/0304-3940(87)90361-2
25. Westerman RA, Widdop RE, Hannaford J, Hogan C, Roberts R, Zimmet P. Non-invasive tests of neurovascular function. Clin Exp Neurol (1987) 24:129–37.
26. Krämer HH, Schmelz M, Birklein F, Bickel A. Electrically stimulated axon reflexes are diminished in diabetic small fiber neuropathies. Diabetes (2004) 53(3):769–74. doi:10.2337/diabetes.53.3.769
27. Rajan V, Varghese B, van Leeuwen TG, Steenbergen W. Review of methodological developments in laser Doppler flowmetry. Lasers Med Sci (2009) 24(2):269–83. doi:10.1007/s10103-007-0524-0
28. Gibbons CH, Illigens BMW, Centi J, Freeman R. QDIRT: quantitative direct and indirect test of sudomotor function. Neurology (2008) 70(24):2299–304. doi:10.1212/01.wnl.0000314646.49565.c0
29. Low PA, Caskey PE, Tuck RR, Fealey RD, Dyck PJ. Quantitative sudomotor axon reflex test in normal and neuropathic subjects. Ann Neurol (1983) 14(5):573–80. doi:10.1002/ana.410140513
30. Parkhouse N, Le Quesne PM. Quantitative objective assessment of peripheral nociceptive C fibre function. J Neurol Neurosurg Psychiatry (1988) 51(1):28–34. doi:10.1136/jnnp.51.1.28
31. Siepmann T, Illigens BMW, Reichmann H, Ziemssen T. [Axon-reflex based nerve fiber function assessment in the detection of autonomic neuropathy]. Nervenarzt (2014) 85(10):1309–14. doi:10.1007/s00115-014-4120-9
32. Bickel A, Krämer HH, Hilz MJ, Birklein F, Neundörfer B, Schmelz M. Assessment of the neurogenic flare reaction in small-fiber neuropathies. Neurology (2002) 59(6):917–9. doi:10.1212/WNL.59.6.917
33. Parkhouse N, Le Quesne PM. Impaired neurogenic vascular response in patients with diabetes and neuropathic foot lesions. N Engl J Med (1988) 318(20):1306–9. doi:10.1056/NEJM198805193182005
34. Benarroch EE, Low PA. The acetylcholine-induced flare response in evaluation of small fiber dysfunction. Ann Neurol (1991) 29(6):590–5. doi:10.1002/ana.410290604
35. Caselli A, Spallone V, Marfia GA, Battista C, Pachatz C, Veves A, et al. Validation of the nerve axon reflex for the assessment of small nerve fibre dysfunction. J Neurol Neurosurg Psychiatry (2006) 77(8):927–32. doi:10.1136/jnnp.2005.069609
36. Abraham A, Alabdali M, Alsulaiman A, Breiner A, Barnett C, Katzberg HD, et al. Laser Doppler flare imaging and quantitative thermal thresholds testing performance in small and mixed fiber neuropathies. PLoS One (2016) 11(11):e0165731. doi:10.1371/journal.pone.0165731
37. Nieuwenhoff MD, Wu Y, Huygen FJPM, Schouten AC, van der Helm FCT, Niehof SP. Reproducibility of axon reflex-related vasodilation assessed by dynamic thermal imaging in healthy subjects. Microvasc Res (2016) 106:1–7. doi:10.1016/j.mvr.2016.03.001
38. Weiser M, Hilz MJ, Bronfin L, Axelrod FB. Assessing microcirculation in familial dysautonomia by laser Doppler flowmeter. Clin Auton Res (1998) 8(1):13–23. doi:10.1007/BF02267599
39. Obayashi K, Ando Y. Diagnosis of small-fiber neuropathy using various autonomic function tests. Rinsho Shinkeigaku (2014) 54(12):1044–6. doi:10.5692/clinicalneurol.54.1044
40. Melik Z, Kobal J, Cankar K, Strucl M. Microcirculation response to local cooling in patients with Huntington’s disease. J Neurol (2012) 259(5):921–8. doi:10.1007/s00415-011-6279-3
41. Algotsson A, Nordberg A, Almkvist O, Winblad B. Skin vessel reactivity is impaired in Alzheimer’s disease. Neurobiol Aging (2016) 16(4):577–82. doi:10.1016/0197-4580(95)00077-R
42. Siepmann T, Frenz E, Penzlin AI, Goelz S, Zago W, Friehs I, et al. Pilomotor function is impaired in patients with Parkinson’s disease: a study of the adrenergic axon-reflex response and autonomic functions. Parkinsonism Relat Disord (2016) 31:129–34. doi:10.1016/j.parkreldis.2016.08.001
43. Shindo K, Iida H, Watanabe H, Ohta E, Nagasaka T, Shiozawa Z. Sympathetic sudomotor and vasoconstrictive neural function in patients with Parkinson’s disease. Parkinsonism Relat Disord (2008) 14(7):548–52. doi:10.1016/j.parkreldis.2007.12.004
Keywords: laser Doppler, imaging, flowmetry, skin, neuropathy, assessment, axon
Citation: Kubasch ML, Kubasch AS, Torres Pacheco J, Buchmann SJ, Illigens BM, Barlinn K and Siepmann T (2017) Laser Doppler Assessment of Vasomotor Axon Reflex Responsiveness to Evaluate Neurovascular Function. Front. Neurol. 8:370. doi: 10.3389/fneur.2017.00370
Received: 04 January 2017; Accepted: 14 July 2017;
Published: 14 August 2017
Edited by:
Luke Henderson, University of Sydney, AustraliaReviewed by:
Dale E. Bjorling, University of Wisconsin-Madison, United StatesAnusha Mishra, Oregon Health & Science University, United States
Copyright: © 2017 Kubasch, Kubasch, Torres Pacheco, Buchmann, Illigens, Barlinn and Siepmann. This is an open-access article distributed under the terms of the Creative Commons Attribution License (CC BY). The use, distribution or reproduction in other forums is permitted, provided the original author(s) or licensor are credited and that the original publication in this journal is cited, in accordance with accepted academic practice. No use, distribution or reproduction is permitted which does not comply with these terms.
*Correspondence: Timo Siepmann, timo.siepmann@uniklinikum-dresden.de
†Present address: Anne Sophie Kubasch, Center for Rare Diseases, Children’s Hospital, Carl Gustav Carus University Hospital, Technische Universität Dresden, Dresden, Germany;
Sylvia J. Buchmann, Department of Neurology, Charite University Medicine, Berlin, Germany